ABSTRACT
The adaptive immune system is responsible for generating immunological response and immunological memory. Regulation of adaptive immunity including B cell and T cell biology was mainly understood from the protein and microRNA perspective. However, long non-coding RNAs (lncRNAs) are an emerging class of non-coding RNAs (ncRNAs) that influence key factors in lymphocyte biology such as NOTCH, PAX5, MYC and EZH2. LncRNAs were described to modulate lymphocyte activation by regulating pathways such as NFAT, NFκB, MYC, interferon and TCR/BCR signalling (NRON, NKILA, BCALM, GAS5, PVT1), and cell effector functions (IFNG-AS1, TH2-LCR). Here we review lncRNA involvement in adaptive immunity and the implications for autoimmune diseases (multiple sclerosis, inflammatory bowel disease, rheumatoid arthritis) and T/B cell leukaemias and lymphomas (CLL, MCL, DLBCL, T-ALL). It is becoming clear that lncRNAs are important in adaptive immune response and provide new insights into its orchestration.
Introduction
RNAs are versatile molecules capable of determining phenotypes, performing catalytic activities, and storing genetic information. Transcriptome analysis has revealed a complex RNA network encoded by a large portion of the human genome that orchestrate gene expression. Over time, many ‘housekeeping’ ncRNAs have been described such as ribosomal (rRNAs), transfer RNAs (tRNAs), small nuclear RNAs (snRNAs), and small nucleolar RNAs (snoRNAs). More recently, new classes of short RNAs with regulatory functions have been discovered, namely microRNAs (miRNAs) and piwi RNAs (piRNAs). Yet, next-generation sequencing technology has revealed a large amount of additional longer ncRNAs further coined as lncRNAs. LncRNAs are arbitrarily defined as having a length of >200 nucleotides with minimal or no potential to encode proteins [Citation1]. The human genome currently contains tens of thousands of annotated lncRNAs (NONCODE database contains ~100,000 entries for lncRNAs), and new classifications for these molecules are emerging as the numbers of characterized lncRNAs increase [Citation2–4]. The biological relevance of lncRNAs is supported by their highly tissue-specific expression patterns, which is a phenomenon noted in early miRNA studies [Citation5]. LncRNAs have been shown to play a role in biological processes such as hormone signalling, reproduction, adipogenesis, neuronal growth, muscle growth, and haemoglobin expression [Citation6–9]. On the cellular level, lncRNAs participate in differentiation, epigenetic regulation, cell proliferation, apoptosis, migration, and other processes [Citation10–14]. The first well-described lncRNA discovered in the early 90s is the X–inactive specific transcript (XIST), which is required for chromosome X inactivation in female cells.
LncRNAs have recently been shown to be functionally relevant in both branches of the immune system i.e. innate and adaptive immunity. Here we focus on reviewing the role of lncRNAs in the physiology of adaptive immune cells and the mechanisms by which they impact immune response. A rapidly accumulating body of evidence suggests that lncRNAs, similar to miRNAs and proteins, have some decisive and indispensable functions for B and T cell physiology which, in turn, has implications in the pathophysiology of autoimmune diseases and malignancies.
Structure and features of lncRNAs
Early on, it has been shown that lncRNAs can interact with miRNAs to indirectly regulate mRNA translation. This network of ‘competing endogenous RNAs’ (ceRNAs) creates regulatory feedback-loops that also can lead to the dysregulation of classical oncogenes or tumour suppressors [Citation15]. However, lncRNAs were described as also acting independently of miRNAs via several mechanisms, such as: i) signalling, ii) scaffolding for proteins, iii) guidance of transcription factors, iv) decoying transcription factors () [Citation13]. LncRNAs typically have low evolutionary conservation and due to this, it is presumed that their ‘function’ is determined by relatively short stretches of sequence or by their secondary/tertiary structure. These short sequences can influence lncRNAs biogenesis, subcellular localization, and interaction partners [Citation16]. Importantly, localizing a particular lncRNA in cytoplasm versus the nucleus can help to decipher its role as a cis versus trans regulator. LncRNA trafficking to cytoplasm suggests a trans activity such as lincRNA-p21 which modulates translation [Citation17], while nuclear localization indicates a potential cis activity, either by topological chromatin changes or regulating neighbouring genes. The genomic position can also uncover lncRNA features, such as in the case of antisense lncRNAs (lncRNA-AS). lncRNA-AS are transcribed from the opposite strand of an mRNA and commonly acting in cis by regulating the overlapping gene(s) [Citation1]. On the other hand, long intergenic non-coding RNAs (lincRNAs) are more alike to mRNAs when it comes to their transcription regulation, biogenesis, and splicing [Citation18].
Figure 1. Schematic overview of lncRNAs’ mechanisms of action. LncRNA can a) promote or disrupt signal transduction by interacting with proteins, b) serve as a scaffold to proteins, c) act as competing endogenous RNAs (ceRNA) and bind miRNAs, d) guide or e) decoy transcription factors
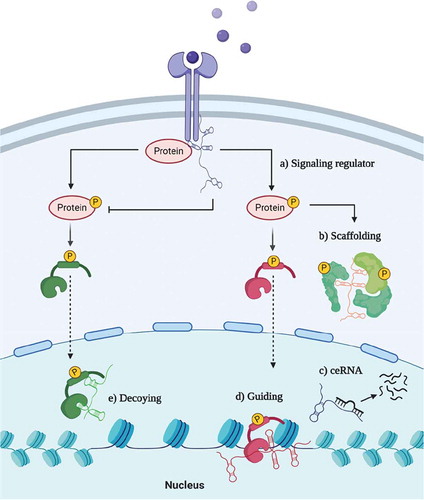
Furthermore, lncRNAs’ transcription is tightly regulated by epigenetic marks, and the presence of specific marks distinguishes chromatin signatures across different intergenic lncRNA classes [Citation19]. LncRNAs with high amounts of H3K4me3 histone mark on the transcription start site (TSS) and H3K4me1 across the gene are similar to protein-coding genes and were coined as promoter-associated lncRNAs (pRNAs). On the contrary, lncRNAs with a higher H3K4me1 content across the gene but low to no H3K4me3 at TSS are likely enhancer-associated RNAs (eRNAs) [Citation19,Citation20]. A lncRNA with epigenetic eRNA marks is more likely to regulate the neighbouring gene(s) in cis, and this guides experimental designs. eRNAs are difficult to silence through RNA interference techniques due to activity near the origin of transcription [Citation21]. Additionally, ectopic eRNA overexpression may lead to a false-negative result when exogenous RNA is far from the place of activity [Citation21]. On the contrary, pRNAs might act as trans-activators, in which case their function is independent of the genomic position.
Both nuclear and cytoplasmic lncRNAs and those acting in cis or trans have been shown to influence the physiology of cells required for adaptive immunity, and in the following chapters, we review this. Identifying lncRNAs’ function remains technically challenging, and these molecules are likely to have many more functions waiting to be discovered.
LncRNAs in lymphocyte development
Lymphocytes develop from haematopoietic stem cells (HSCs) in a tightly controlled multistep process. LncRNAs were shown to regulate the fundamental self-renewal process in HSCs and cell commitment to myeloid versus lymphoid lineage. LncHSC-1 participates in myeloid commitment, while lncHSC-2 is involved in lymphocyte commitment [Citation22,Citation23]. In the beginning, HSCs need to differentiate into common lymphoid progenitors and pass through different microenvironment stimuli to decide on B and T cell lineage commitment. This process has been shown to be closely regulated by protein-coding genes and miRNAs [Citation24], but its complexity also involves lncRNAs.
In 2015, a study revealed the ‘landscape’ of 9444 lncRNAs expressed in B and T cell lineages, of which 35% had not been previously annotated [Citation25]. In B cells, lncRNA expression correlated with the expression of characteristic B cell markers such as CD19, BLNK, and pre-BCR and underwent similar modulations during the stages of B cell maturation [Citation26,Citation27]. One of the classical transcription factors of the early B cell stage, PAX5 (regulates CD19, BLNK, VpreB, and VDJ recombination), was shown to bind and regulate over 100 lncRNAs in pro- and mature mouse B cells [Citation26].
Differently from B cells, T cell lineage development takes place in the thymus, and the immature precursors are known as CD4−CD8− double-negative cells. Analogically to PAX5 during B cell maturation, Notch1 plays a fundamental role in T cell development [Citation28]. Notch1 itself seems to be regulated by a lncRNA named ‘Notch1-associated lncRNA in T-ALL’ (NALT1). NALT1 is a nuclear lncRNA localized ~100 nucleotides from the Notch1 gene. Due to this proximity, Wang et al hypothesized that NALT1 acts in cis and regulates its neighbouring gene. In their study, shRNA against NALT1 decreased Notch1 levels and impaired T cell proliferation, but on the other hand, ectopic NALT1 overexpression wasn’t able to rescue Notch1 expression. These experiments revealed a role dependent on the genomic position, suggesting that NALT1 regulates Notch1 through a cis-regulation mechanism () [Citation29].
Table 1. Summary of lncRNAs involved in T cell biology
The Notch pathway tunes a network of proteins responsible for thymocyte physiology. In particular, BCL11B is tightly regulated by Notch and is also essential for T cell development [Citation30,Citation31]. Double-negative cells orchestrate chromatin folding and compartmentalization to support BCL11B expression. An lncRNA named ThymoD (thymocyte differentiation factor) participates in re-organizing chromatin architecture, moving BCL11B enhancer from the lamina to the nuclear interior and thereby repositioning the BCL11B promoter and enhancer into a single loop domain [Citation30]. Additionally, thymocytes require the last step of T cell receptor (TCR) recombination to gain a mature TCR. It has been revealed that T early α promoter in Tcra locus is transcribed into a non-coding transcript (>50 kbs) supporting chromatin remodelling to promote Jα recombination [Citation32]. Vα-to-Jα recombination is rearranged from Tcra locus, and this step is crucial to produce a mature TCR αβ expressed by single-positive mature T cells.
Lymphocyte development contains multiple sequential events in both B and T cell lineage. The main transcriptional regulators from both lineages have been shown to regulate lncRNAs, and these likely serve to fine-tune their transcriptional programs. The evidence supporting such roles in differentiating and activating T/B cells is discussed in the next chapters.
LncRNAs in T cell activation and differentiation
Mature T cells have their activation triggered by the TCR when recognizing foreign antigen-derived epitopes presented in the context of MHC molecules. The TCR-mediated activation is tuned by co-stimulatory or co-inhibitory receptors engaged by ligands provided by the cells in the microenvironment. During TCR activation, the lncRNA PVT1 is upregulated and provokes an increase in glycolytic capacity (, )[Citation33,Citation34]. PVT1 influences aerobic glycolysis during T cells expansion which, most of the time, remain as quiescent cells utilizing oxidative phosphorylation.
Figure 2. Summary of lncRNAs’ regulatory networks involved in T cell biology. LncRNAs influence T cell activation and inhibition by participating in TCR cascade and T cell inhibition through co-inhibitory receptor signals (centre of the figure top and bottom). LncRNAs are also involved in differentiating TH subsets and their effector functions (such as TH17, TREG, TH1, TH2; see corners of the figure for each subset). These lncRNAs can dictate TH polarization upon TCR activation and contribute to T cell response. LncRNAs are indicated in bold boxes, miRNAs in rectangles, and proteins or functions in other boxes. Arrows indicate positive regulation, blunt-ended lines indicate negative regulation, and straight lines indicate interaction. Gene names in red indicate typical markers for each subset of the TH population
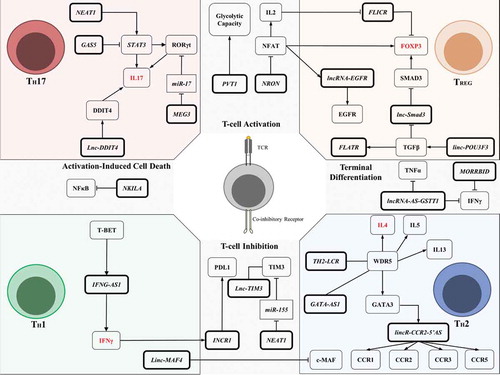
The TCR signalling pathway is also known to activate NFAT to induce IL2 transcription and synthesis [Citation35]. A lncRNA named ”Noncoding repressor of NFAT” (NRON) was found to impair NFAT activity in resting T cell by forming a scaffold that modulates NFAT trafficking to the nucleus by interacting with IQ motif containing GTPase activating protein (IQGAP), casein kinase 1 (CK1), glycogen synthase kinase 3 (GSK3), and dual-specificity tyrosine phosphorylation regulated kinase (DYRK) (, ) [Citation36–38].
Once T cells are activated, they face a challenge to overcome the activation state by surviving a process called activation-induced cell death (AICD). During AICD, T cells upregulate pro-apoptotic factors such as FasL, TRAIL, and TNF, increasing the propensity to apoptosis, which results in the T cell clearance [Citation39]. The NFκB Interacting lncRNA (NKILA) is upregulated during T cell activation and represses NFκB activity favouring the AICD [Citation40]. The mechanism underlying this process involves TCR signal amplification by the calcium-calmodulin pathway and chromatin changes in the NKILA promoter, which allows STAT1 to mediate its transcription (, ) [Citation40].
LncRNAs were also shown to regulate IFNγ levels, an essential proinflammatory/anti-tumour cytokine released in high amounts by cytotoxic (CD8+ cells) and T helper 1 (TH1) cells. The discovery came from an interesting observation made in two mice strains with different susceptibility to Theiler’s virus infection [Citation41,Citation42]. The Tmevp3 locus was defined as being responsible for genetic resistance to the virus and further characterized to contain lncRNA IFNG-AS1 [Citation41,Citation42]. IFNG-AS1 (also known as NeST or Tmevpg1) was shown to physically interact with WDR5, mediating H3K4 methylation and consequently enhancing IFNγ production [Citation42]. Another study using T cells argued that IFNG-AS1 indeed helps to organize chromatin to regulate IFNG but in cis, without affecting other genes (, ) [Citation43]. Altogether, there is strong evidence that IFNG-AS1 plays a role against pathogens such as Salmonella enterica [Citation42], Theiler’s virus [Citation42], and Toxoplasma gondii [Citation43], and this is linked to IFNγ activity.
A small fraction of CD8+T cells overcome AICD to become memory T cells. Activated T cells follow the differentiation into stem memory T cells (TSCM), central memory T cells (TCM) and effector memory T cells (TEM), which are all important in adaptive immunity response. LncRNA expression dynamics in naïve and memory T cells was studied in mice challenged with lymphocytic choriomeningitis virus (LCMV) infection, which is a model previously used to study protein-coding genes [Citation44]. Affinity propagation clustering revealed that some lncRNAs have a similar expression to CD244 and IFNG, suggesting their involvement in memory T cell populations [Citation44]. CD244 is a co-inhibitory receptor expressed in terminal effector T cells and exhausted T cells inhibiting IFNγ and TNFα production [Citation44,Citation45]. Antisense lncRNA ‘glutathione S-transferase theta-1’ (lncRNA-AS-GSTT1) levels were found to positively correlate with CD244 expression [Citation46]. This lncRNA physically interacts with EZH2 and regulates histone methylation marks to repress IFNG and TNFA transcription (, ) [Citation46]. Adoptively transferred CD8+ cells with depleted lncRNA-AS-GSTT1 efficiently reduced Mycobacterium tuberculosis infection when compared to mice transplanted with wild-type CD8+ T cells [Citation46].
IFNG-AS1 was the first well-described lncRNA which enhances IFNγ production in T cells. On the other hand, lncRNA Morrbid has been shown to impair IFNγ production in CD8+ effector T cells [Citation47]. Morrbid is induced by TCR signals, upregulates a proapoptotic gene bcl2l11, and tunes the PI3K-AKT pathway during IFN type I stimulation [Citation47]. Strikingly, Morrbid-less T cells produce higher amounts of IFNγ, TNFα, and Granzyme B during LCMV infection () [Citation47]. IFNG-AS1, lncRNA-AS-GSTT1, and Morrbid functions suggest an important role for lncRNAs in CD8+ T cell biology.
LncRNAs were also described as having an impact on T helper cell polarization. TH cells can differentiate in T cell subsets depending on the cytokines and microenvironment stimuli. The term ‘polarized’ refers to CD4+ T cell differentiation into TH1 or T helper type 2 (TH2), which canonically produce IFNγ and IL4, respectively [Citation48]. Linc-MAF4 seems to be a lncRNA dictating classical polarization. Linc-MAF4 is inversely correlated with c-MAF levels [Citation49,Citation50]. linc-MAF4 epigenetically represses c-MAF expression and contributes to TH1 differentiation [Citation49], but on the other hand, downregulating linc-MAF4 facilitates differentiation towards TH2 (, ) [Citation49,Citation50].
TH1 commitment is driven by JAK-STAT components such as STAT1 and STAT4, triggered mainly by IL12 and IFNγ. These signals control the main TH1 transcription regulator T-bet. Above, we have described that IFNγ production is affected by lncRNA IFNG-AS1, and this was also expected to play a role in TH1 cells. IFNG-AS1 is selectively expressed in TH1 and not in TH2, and accordingly is dependent on STAT4 and T-bet activity [Citation51]. In STAT4-/- or T-bet-/- transgenic mice, TH1 cells down-modulated IFNG-AS1 and IFNG expression. IFNG expression was only rescued by T-bet with IFNG-AS1 in cooperation [Citation51]. Further, T-bet was confirmed to bind the IFNG-AS1 promoter and epigenetically modify it to recruit other transcription factors, namely NFκB and Ets1 [Citation52]. This might explain the role of TCR stimulation in IFNG-AS1 expression and how IFNG-AS1 is crucial for T cell effector functions and TH1 polarization.
TH2 cells use GATA3 as the main transcription factor, which also regulates several lncRNAs to reach the TH2 phenotype. Hu et al first investigated the lncRNAs regulated in GATA3 wild-type TH2 vs GATA3-/- TH2 cells and discovered the lncRNA named lincR-Crr2-5ʹAS [Citation14]. LincR-Crr2-5ʹAS is located between Ccr2 and Ccr3 and it seems to enhance Ccr1, Ccr2, Ccr3, and Ccr5 chemokine receptor expression (). LincR-Crr2-5ʹAS knockdown impaired TH2 migration to the lungs, matching with a chemokine related function [Citation14]. Additionally, an antisense RNA in GATA3 locus was found to be selectively expressed in TH2 [Citation53]. GATA3-AS1 has a controversial mechanism of action regarding cis or trans activity since it seems to bind WDR5 to promote methylation of its gene locus and in the shared promoter with GATA3 [Citation54]. TH2-LCR is another specific lncRNA from TH2 cells that also binds to WDR5 to promote chromatin remodelling during TH2 polarization [Citation55]. GATA3-AS1 and TH2-LCR seem to lead the IL4, IL5, and IL13 expression regulation through chromatin changes, which are essential for TH2 effector function (, ) [Citation54,Citation55]. In a nutshell, IFNG-AS1 was described as being functionally relevant for the TH1 population while lincR-Crr2-5ʹAS, GATA3-AS1, and TH2-LCR cooperate to induce the TH2 phenotype. Additionally, linc-MAF4 has been shown to dictate the classical polarization. However, the TH polarization is not limited by these two populations and additional TH cell subsets have already been described.
The known TH repertoire was expanded into follicular TH cells (TFH), TH type 3 (TH3), TH type 9 (TH9), TH type 17 (TH17), TH type 22 (TH22), and T regulatory cells (TREG) [Citation56,Citation57]. TH17 and TREG cells could be considered antagonists in the immune response context. TH17 cells produce IL17 and promote proinflammatory effects, while TREG cells are characterized by Foxp3 expression and promote immunosuppression [Citation57]. A dysregulation between TH17 and TREG ratio has been associated with a variety of diseases such as asthma, autoimmune diseases, and problems during allogeneic haematopoietic stem cell transplantation. LncRNA MEG3 was shown to regulate TH17/TREG balance in asthmatic patients by acting as ceRNA for miR-17 [Citation58]. Asthmatic patients suffer from chronic airway inflammation and have low miR-17 levels in the CD4+ T cell population [Citation58]. This miRNA was further described to repress RORγt, the key transcription factor in TH17 [Citation59]. RORγt is induced by STAT3 and both transcription factors cooperate together to achieve full TH17 differentiation and effector function [Citation60]. STAT3 half-life is regulated by lncRNAs in the TH cells. NEAT1 binds STAT3 to promote its stabilization while GAS5 binds STAT3 to promote its degradation, and this indirectly modulates RORγt via STAT3 activity [Citation61,Citation62]. Lnc-DDIT4 was also shown to impact effector function by cis-activating DDIT4 and enhancing the mTOR pathway [Citation63]. Altogether, MEG3, NEAT1, GAS5, and lnc-DDIT4 are the main lncRNAs described to tune RORγt and impact the TH17 effector function (). A properly tuned RORγt is crucial to dictate TH17/TREG balance once its activity antagonizes Foxp3, preventing TREG polarization even though both transcriptional regulators are stimulated by TGFβ [Citation59].
Curiously, lncRNA Flatr expression precedes Foxp3 expression during TREG polarization in the presence of TGFβ in vitro, and the Flatr-deficient mice have a delayed TREG induction in vivo (, ) [Citation64]. The TGFβ pathway itself is enhanced by linc-POU3F3 [Citation65] and its cascade upregulates Smad2/3 through several epigenetic events that tune TREG polarization (). The accessibility to the Smad3 promoter is negatively regulated by lnc-Smad3 which binds histone deacetylase 1 (HDAC1) to epigenetically suppress Smad3 transcription (, ). Upon TGFβ stimulation, lnc-Smad3 is downregulated and TH cells recruit methyltransferase Ash1l to accumulate activate marks in Smad2/3 promoter regions [Citation66]. Hence, Smad3 is upregulated and indirectly enhances Foxp3 expression by binding regulatory regions [Citation67]. Foxp3 is a TREG program master regulator and a specific marker for this population. Foxp3 expression seems to be a critical checkpoint to differentiate TH cells into TREG cells and promote immunosuppression. ‘Foxp3 long intergenic noncoding RNA’ (Flicr) was shown to negatively cis-regulate Foxp3 and paradoxically is specifically expressed in TREG. Flicr is presumably fine-tuning the regulation of Foxp3, together with TGFβ and IL2 signalling (, ) [Citation68]. TCR-activated CD4+ cells under TGFβ stimuli modulate NFAT trafficking to the nucleus and cooperate with Smad3 to sustain Foxp3 expression [Citation67]. Flicr is repressed upon IL2 exposure and NFAT is probably the key transcription factor to orchestrate important feedback loops with the TGFβ pathway to sustain TREG polarization.
NFAT cooperates with Foxp3 in a network circuit to convert activated T cells into TREG cells. NFAT promotes strong AP-1 interaction and indirectly enhances the EGFR pathway, recently linked with the TREG phenotype [Citation69,Citation70]. NFAT starts a forward feed-loop to upregulate lncRNA-EGFR which binds EGFR to avoid cCBL degrading it and contributing to the TREG phenotype (, ) [Citation70]. Although NFAT is the canonical transcription factor inducing IL2 production while Foxp3 has been shown to repress IL2, both transcription factors cooperate to induce CD25 (Interleukin-2 receptor alpha chain). This may seem contradictory, as TREG cells are characterized as CD4+ CD25+ Foxp3+ and are highly responsive to IL2. But as we can infer, CD25 is a fundamental receptor to ensure fine-tuned lncRNA control related to Foxp3 regulation through feedback loops triggered by TGFβ, IL2, and EGFR ligands. Thus, lnc-Smad3, Flicr, and lncRNA-EGFR are the main lncRNAs known to be directly involved in the transcriptional programs required to achieve full TREG polarization and effector function ().
Altogether, these studies support the lncRNAs’ crucial participation in differentiating and specializing T cell subsets (, ). It remains unknown whether lncRNAs are involved in other subsets of T helper cells, and this will likely be a fruitful area of future research.
LncRNAs in B cell activation and differentiation
The adaptive immune system also depends on proper B cell function. Mature B cells are characterized by the expression of IgM and IgD on the membrane, which are assembled with Igα and Igβ to form a mature B cell receptor (BCR) [Citation71]. The signal trigged by BCR is crucial to maintain the B cells in the peripheral blood through tonic activation and to drive further differentiation in order to become antibody-producing cells [Citation71]. Wang et al studied B cells in the chronic graft-versus-host disease and found lncRNAs co-expressing with mRNAs related to the BCR pathway, however, the functional relevance of those lncRNAs for BCR signalling remains unclear [Citation72]. Recently, Pyfrom et al discovered a cytoplasmic lncRNA named BCALM to be involved in BCR-mediated Ca+ signalling. BCALM interacts with AKAP-associated proteins to phosphorylate phospholipase D 1 (PLD1). PLD1 produces phosphatidic acid which activates SHP1 phosphatase, a negative BCR signalling regulator, resulting in a negative feedback loop (, ) [Citation73].
Table 2. Summary of lncRNAs involved in B cell biology
Figure 3. Summary of lncRNAs’ involvement in B cell biology. a) BCALM and DLEU1/2 are involved in B cell activation. BCALM drives a negative feedback loop with SHP1 to decrease BCR-mediated activation while DLEU1/2 cis-regulates NFκB-related genes. b) LncRNAs can promote malignant B cell proliferation by interacting with EZH2 and by being a part of MYC’s pro-proliferative program. c) FAS-AS1 promotes membrane Fas isoform generation. FAS-AS1 is epigenetically repressed in B cell lymphomas, leading to soluble Fas generation and cell death evasion. d) NEAT1 and lincRNA-p21 are regulated by p53 during DNA damage response. e) During germinal centre (GC) reaction, AID interacts with xTSS-RNAs that influence genome integrity. AID also binds transcripts from the S region to drive class switch recombination and lncRNA-CSRIgA specifically induces an IgA class switch. f) FAM30A is an antisense RNA from the IgH locus that positively correlates with high antibody titres. Plasma cells generate high levels of circRNAs from Ig genes. LncRNAs are indicated in bold boxes, miRNAs in rectangles, and proteins or pathways in other boxes. Arrows indicate positive regulation, blunt-ended lines indicate negative regulation, and straight lines indicate interaction. DSB, double-strand break; CSR, class switch recombination
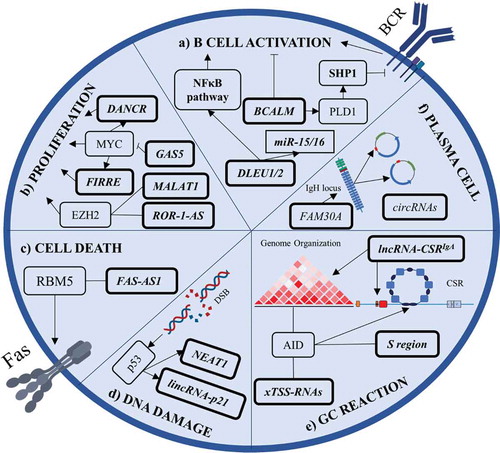
Upon BCR activation, B cells undergo a germinal centre (GC) reaction in order to become plasma cells or memory B cells responsible for immunoglobulin (Ig) production and immunological memory, respectively [Citation74]. During all these steps of differentiation, lncRNAs seem to be tightly regulated with specific profiles in different stages of B cell maturation, just as it is with protein-coding genes [Citation26,Citation27,Citation75]. In fact, ~30% of the human genome is transcribed when taking together all the different stages of B cell differentiation and the number of expressed lncRNAs exceeds the number of protein-coding genes [Citation75]. B cell maturation stages not only differ in gene expression but also in chromatin organization. The lncRNA XIST is expressed during the female B cell maturation steps, with slight differences in its levels [Citation76]. Naïve female lymphocytes were the first example of somatic cells where XIST RNA does not co-localize with inactive X (Xi) chromosome [Citation77]. In such B cells, the RNA molecules are dispersed in the nuclei and they don’t form clusters on Xi as in other cells. Interestingly, the XIST localization seems to be modulated during B cell maturation and activation. XIST RNA signals in the nuclei are abolished in pro-B cells, while GC cells and plasma cells have a small fraction of XIST localizing in detectable ‘clouds’ not linked to Xi [Citation76]. Interestingly, female mature B cells have a physiologically permissive gene expression localized in the chrX.q13 and chrX.p22 regions, which contain immune-related genes [Citation77]. Still, B cell activation with lipopolysaccharide (LPS) or CpG induces dramatic changes in the XIST RNA signal to co-localize to the X chromosome, while this is less prominent during BCR activation [Citation76]. This suggests dynamic chromatin regulation in X chromosome by XIST during B cell activation, but the functional significance remains a matter of debate. XIST was shown to bind the EZH2 subunit of the Polycomb complex (PRC2) to place histone marks (H3K27me3) needed to inactivate the X chromosome [Citation76,Citation77], and EZH2 is well known to be involved in GC cell biology ().
In GC cells, LINC00487, LINC00877, and RP11-203B7.2 expression is highly correlated with mRNAs typical for this stage of B cell maturation [Citation27] and also GC cells have a high abundance of eRNAs compared to the naïve B cells [Citation75]. These eRNAs could participate in the intense chromatin changes that GC cells undergo. RP11-132N15.3 is localized upstream from BCL6 and its levels strongly correlate with BCL6 expression suggesting a cis-regulation () [Citation27]. The upstream region from BCL6 is essential to re-organize genome architecture and coordinate the transcription program [Citation78]. BCL6 presents as a hotspot locus for topological re-arrangements and gene loops [Citation78] but also contains divergent and convergent lncRNAs which may contribute to activation-induced cytidine deaminase (AID) activity and its off-target effects in this region, such as mutations or translocations, typically observed in B cell lymphomas [Citation79]. BCL6 has a sequence motif recognized by AID in intron 1 and interestingly, an antisense lncRNA RP11-211G3.3.1–1 is located in the region where ~90% of the translocations occur [Citation80]. This would indicate that lncRNAs might influence the B cells’ genome integrity and the occurrence of translocation involving oncogenes and immunoglobulin genes.
Activation of AID is a hallmark of a GC reaction and acts to promote somatic hypermutations and class switch recombination (CSR); generating other classes of immunoglobulins beyond IgM and IgD [Citation81]. During CSR, the molecular machinery causes double-strand breaks (DSB) in the switch (S) region of each constant region (CH) and repairs using the non-homologous end joining (NHEJ) pathway resulting in a different CH cluster. The S regions were shown to be transcribed into lncRNAs and promote R-loops to facilitate CSR () [Citation82]. Nascent RNAs seem to be processed by an RNA exosome complex [Citation83], which interacts with several ncRNAs to recruit AID to specific sites and supports DNA-RNA hybrid structures focusing AID activity on these spots [Citation84]. These RNAs can come from promoters and enhancers transcribed in divergent directions at the TSS. Pefanis et al referred to them as TSS-associated transcripts (xTSS-RNAs) [Citation84]. It is suggested xTSS-RNAs may boost off-target AID activity, but also facilitate CSR () [Citation85]. These are examples of how lncRNAs can directly affect AID activity. However, lncRNAs can also promote topological chromatin changes in immunoglobulin loci to favour CSR. Recently, an eRNA was identified to favour IgA CSR [Citation86]. LncRNA-CSRIgA facilitates molecular machinery recruitment near CTCF sites and promotes interaction between the locus sites to induce an IgA switch (, ) [Citation87]. The presence of lncRNA influencing switching to other immunoglobulin classes remains unclear. These studies suggest the possibility of a complex relationship between the triad DNA:ncRNA:protein during CSR and lncRNAs’ role in coordinating cell differentiation for a proper humoral response.
Given these observations, it is reasonable to hypothesize that lncRNAs might have a role in various B cell subtypes and their physiology. Indeed, human plasma cells express a higher amount of circular RNA (circRNA) and the majority of these circRNAs came from Ig genes, suggesting that rearranged Ig genes generate specific RNA pieces representing plasma cell clonality () [Citation75]. This resembles our previous observations describing the rearrangement of specific immunoglobulin light chain variable subgenes in B cells leading to miRNA production from the immunoglobulin transcript [Citation88–90]. This has consequences for the biology of these cells, but it is unclear how this developed during immunoglobulin light chain gene evolution. It is plausible that the strongly expressed transcripts for heavy or light BCR Ig give rise to some ncRNAs, and this helps to tune B cell physiology with productively rearranged immunoglobulins. Furthermore, a study identified lncRNAs LINC00525, GUSBP11, and FAM30A as strongly correlated with plasma cell response during periodontitis () [Citation91]. The lncRNA FAM30A is an antisense lncRNA from IgH locus and its levels positively correlated with antibody titres during a vaccination study () [Citation92].
Overall, these findings suggest during B cell maturation, a complex orchestra of RNAs interacting with DNA and proteins helps to shape the chromatin and ensure important checkpoints for B cell differentiation (, ). It seems that understanding lncRNAs is progressing more rapidly in T cell biology, and this opens opportunities for interesting research and investigations into lncRNAs in B cells.
LncRNAs in autoimmune diseases
Autoimmune diseases affect 5–10% of people worldwide [Citation93] and 90% of single nucleotide polymorphisms (SNPs) associated with these diseases are located in non-coding regions of the human genome [Citation94]. SNPs may hypothetically contribute to (dys)regulating lncRNAs, affect lncRNA secondary structure, or determine their splicing [Citation95]. Recently, a susceptibility loci SNP rs7134599 within the lncRNA IFNG-AS1 was described in inflammatory bowel disease (IBD) and curiosity arose about its impact on patients’ health [Citation96]. Patients with IBD suffer from chronic inflammation in their digestive tract, and Rankin et al have shown that colon cells also express a specific IFNG-AS1 isoform, which correlates with severe colitis [Citation96].
Hosseini et al also argued that high IFNG levels correlate with high IFNG-AS1 levels in the peripheral blood mononuclear cells of patients with relapsed multiple sclerosis (MS) [Citation97], suggesting a potential role in this disease too. Notably, conditioned medium from T cells overexpressing IFNG-AS1 was able to hyper-stimulate primary immune cells [Citation96]. These observations suggest that SNP dysregulating IFNG-AS1 or other lncRNA involved in cytokine release could boost TH cell activation and start a vicious circle of pro-inflammatory cytokine production.
Additionally, autoimmune diseases such as IBD and MS show disrupted TH subpopulation balance. They often are enriched in proinflammatory TH cells like TH1 and TH17 while tend to suppress the TREG population, and this is mirrored or caused by dysregulating lncRNAs related to TH polarization as discussed above. For example, high linc-MAF4 levels in MS patients’ CD4+ cells support the polarization towards TH1 cells [Citation50], which is critical for producing IFNγ. Downregulating lncRNA MALAT1 was also associated with the peak of disease in an MS mice model and an increase in the size of TH17 population [Citation98].
TH17 cell enrichment is also relevant in rheumatoid arthritis (RA) and these patients have upregulation of TH17-related lncRNAs (MEG3, Rmrp, NEAT1, and lnc-DDIT4) () [Citation61]. RA is a chronic inflammatory disease that mainly affects synovial joints where the disease evaluation relies on the number of involved joints, inflammatory clinical parameters, symptom duration, serologic rheumatoid factor, or anti-citrullinated antibody (anti-CCP) [Citation99]. The clinical parameters and anti-CCP antibody production have been associated with multiple SNPs in the lncRNAs loci encoding ANRIL, lnc-DC, MALAT1, ZFAS1, and MEG3 [Citation100,Citation101]. Some of these lncRNAs are expressed in T cells and as we have mentioned, could potentially hyper-stimulate T cells.
However, in contrast to IBD and MS, which are mainly T cell-dependent diseases, RA patients’ clinical outcome is related to the levels of autoreactive antibodies, such as anti-CCP antibody. Preliminary efforts to associate lncRNAs with autoantibody production found a negative correlation between serum MEG3 expression and anti-CCP, while high IFNG-AS1 expression positively correlated with anti-CCP antibody levels [Citation102]. This positive correlation between autoantibody production and IFNG-AS1 levels was also observed in Sjögren’s syndrome [Citation103] and Hashimoto disease to a certain extent [Citation104].
In this context, a surprising and somehow counterintuitive observation was made for myasthenia gravis (MG). Patients with MG have lower IFNG-AS1 levels than healthy donors and this negatively correlates with anti-nicotinic acetylcholine receptors antibody (anti-AChR) levels [Citation105]. The downregulation of IFNG-AS1 leads to HLA-DRB1 and HLA-DOB upregulation [Citation105]. These genes are a part of the MHC class II beta family crucial for antigen-presenting cells (APC) in order to carry peptides and engage in immune response with T cells. Paradoxically, HLA-DRB1 is associated with other autoimmune diseases such as RA [Citation106], MS [Citation107], and type 1 diabetes [Citation108]. The mechanisms by which IFNG-AS1 could influence HLA-DRB1 and HLA-DOB expression are still unknown, but this axis potentially represents an important piece in the puzzle to understand autoimmune disease biology. Ultimately, in clinical practice nowadays, autoimmune disease patients are treated irrespective of the TH cell population balance and there is a lack of a biomarker to identify the probability of disease progression versus patients that are ‘good’ responders. For instance, IFNG-AS1 was elevated in good responders after prednisone treatment but remained unchanged in non-responders in MG [Citation105]. The data suggest that the elevating IFNG-AS1 in good responders leads to the downregulation of HLA-DRB1 and HLA-DOB affecting T cell activation in MG. This was shown by the decrease in CD40L and T-bet expression in CD4+ T cells from MG cells transfected with constructs for IFNG-AS1 overexpression [Citation105]. CD40L is essential to prime B cells to produce anti-AChR IgG and for the onset of autoimmune myasthenia gravis, suggesting an important cross-talk interaction between the T cells and B cells in its pathogenesis [Citation109].
Although antibody production and CSR are highly dependent on CD40L provided by T cells, BAFF seems to replace this role in a CD40L-independent fashion in some autoimmune diseases [Citation110,Citation111]. BAFF is produced by innate immune cells from the microenvironment and its overexpression is associated with Sjögren’s syndrome, RA, and systemic lupus erythematosus (SLE) [Citation112,Citation113]. SLE patients are known to have active IFN type I signalling in B cells, which correlates to high anti-dsDNA titers. Dong et al noticed that the percentage of myeloid-derived suppressor cells (MDSCs) directly influenced SLE disease progression and also the autoantibody production in a mouse model [Citation114]. NEAT1 was found to be overexpressed in granulocyte MDSCs and further described to enhance BAFF production. This NEAT1-BAFF axis was also confirmed to be elevated in SLE patients followed by an IFN type I signalling signature compared to healthy donors [Citation114]. These studies also argue for a possible link in lncRNAs with autoantibody production driven by innate immune cells reflecting disease progression dependent on the microenvironment.
LncRNAs are expressed in different cells and impact the circuits of pro- and anti-inflammatory cytokines that prime adaptive immune response. LncRNAs’ involvement in the dysbalance of TH subpopulations is becoming evident even though it remains unclear if this is the cause or the consequence in autoimmune diseases. Besides that, studies reported correlations in lncRNAs’ expression from T cells with autoantibody levels in autoimmune diseases, but the biological relevance of this still remains a matter of debate. The field is still lacking strong mechanistic and functional proof related to lncRNAs’ causal role in autoimmune disease pathophysiology, and this underscores the need for further research.
LncRNAs in B and T cell malignancies
The role of lncRNAs in cancer development is an expanding field of study, however, evidence for their direct participation in cancer pathogenesis is still limited. A pioneering publication by the Pandolfi group has shown that BRAF pseudogene (Braf-rs1) overexpression in transgenic mice induces B cell lymphoma development [Citation115]. Braf-rs1 promotes regulatory networks’ dysregulation by sponging multiple miRNAs () [Citation115]. Esposito’s group studied another pseudogene related to human malignancies but not present in the mouse genome [Citation116]. They were able to demonstrate that transgenic mice overexpressing human HMGA1P7 also develop B cell lymphomas. HMGA1P7 overexpression caused the dysregulation of several pathways that contributed to lymphomagenesis [Citation116], such as JAK/STAT, NFκB, and MYC (). This study helped to support the notion that even lncRNAs that do not have a homolog among relatively closely related species, can act as oncogenes or tumour suppressors. LncRNAs have also been shown to act in association with canonically known oncogenes such as the MYC, which binds lncRNA PVT1 [Citation34,Citation117–119].
Malignant cells frequently acquire genetic aberrations and/or dysregulated network pathways that hold the cells in a certain stage of differentiation. Mutations in the Notch gene for example are reported in ~50% of patients with T cell acute lymphoblastic leukaemia (T-ALL) and are considered an important transformation event [Citation120]. T-ALL originates from thymocytes and data suggest that many lncRNAs are regulated by Notch1 signalling in normal and T-ALL lymphocytes. However, only only LUNAR1 (lnc-IGF1R-1) and lnc- FAM120AOS-1 seem to be differentially expressed in Notch1 wild-type vs Notch1 mutated T-ALL [Citation121]. LUNAR1 is a nuclear lncRNA cis-activating the insulin-like growth factor 1 receptor (IGF1R) localized approximately 50 kilobases from LUNAR1 () [Citation122].
In B cell acute lymphoblastic leukaemia (B-ALL), oncogene translocations are an important genetic hit used by clinicians to assess patients prognosis based on cytogenetics. Fernando and colleagues showed that lncRNA expression profiles can segregate between the well-known cytogenetic B-ALL groups [Citation123]. Similarly, lncRNAs were found to be differentially expressed in prognostic subgroups of chronic lymphocytic leukaemia (CLL) and associated with IGHV status and presence of chromosome aberrations [Citation124,Citation125]. This resembles the situation with miRNAs that were also initially described as CLL subtypes’ discriminators that later turned out to be functionally relevant for cell proliferation, apoptosis, and microenvironmental interactions such as BCR signalling [Citation88,Citation126–128].
CLL originates from the mature B cells and was one of the first diseases directly linked to both short and long non-coding RNAs’ dysregulation. The most common genetic hit observed in CLL is the deletion of the chromosome 13q region containing two lncRNAs (DLEU1 and DLEU2). DLEU2 is recognized as the host gene for miR-15 and miR-16, however, DLEU2 also seems to affect in cis the genes involved in the NFkB pathway (, ) [Citation129]. CLL is a generally indolent disease but can undergo transformation into an aggressive lymphoma-like Richter’s syndrome (RS), and similar histological transformation is also observed in other indolent B cell malignancies such as follicular lymphoma. One of the most frequent aberrations described in transformed CLL and follicular lymphoma is the gain in MYC activity [Citation130,Citation131]. MYC is a potent proto-oncogene frequently dysregulated in B/T cell leukaemias and lymphomas. FIRRE and DANCR are lncRNAs found to be part of the proliferative program regulated by MYC in B cell lymphomas. FIRRE promotes proliferation by activating Wnt/β-catenin signalling while DANCR promotes proliferation by p21 inhibition (, ) [Citation132,Citation133]. MYC is also guided by lncRNA PDIA3P to bind the G6PD promoter, thus boosting G6PD expression and pentose phosphatase pathway (, ) [Citation134]. Due to the MYC’s huge impact on cell physiology, it needs to be tightly regulated. MYC is directly targeted by miR-34-5p, but MYC also promotes lncRNA RMRP transcription to sponge miR-34-5p [Citation135]. LncRNA GAS5, on the other hand, works as a tumour suppressor interacting with eIF4E to repress MYC translation [Citation136]. Besides that, MYC is constantly degraded in the cell when phosphorylated on Thr58, and lncRNA PVT1 interacts with MYC to impair this phosphorylation and thus increases MYC activity [Citation34,Citation119]. Notably, aggressive lymphomas recurrently harbour PVT1 rearrangements [Citation137], and an SNP (rs2608053) in PVT1 locus has been shown to impact progression-free survival in Hodgkin lymphoma [Citation138].
MYC also directly binds miRNA promotors to shut down tumour-suppressor miRNAs (i.e. miR-150, miR-15/16, miR-26, miR-29) or recruits EZH2 to enhance the proliferative program through epigenetic changes [Citation139,Citation140]. LncRNAs such as ROR1-AS1 and MALAT1 were shown to bind EZH2 to promote mantle cell lymphoma cell proliferation (, ) [Citation141,Citation142]. Interestingly, EZH2 is frequently mutated in multiple non-Hodgkin’s lymphomas and it remains to be determined if that could affect binding to lncRNAs that contribute to chromatin modifications. EZH2 was also linked to evading cell death by suppressing an lncRNA. This mechanism was described in B cell lymphomas where an alternative splicing variant of Fas was found. This alternative isoform skips exon 6, producing a soluble variant instead of being bound on the cell membrane, which renders cells resistant to apoptosis. The lncRNA FAS-AS1 was discovered to bind RBM5 and inhibit exon 6 exclusion, promoting the generation of the membrane isoform (, ). Interestingly, FAS-AS1 is downregulated in lymphomas via EZH2, and this affects apoptosis susceptibility [Citation143]. Besides evading apoptosis, cells respond to stress and accumulate DNA damage during their lifetime.
The p53 is a ‘genome guard’ protein, induced by DNA damage and responsible for a multitude of physiological functions, including cell senescence. For this reason, p53 is frequently mutated in cancer cells and, when activated, it upregulates several genes to mediate cell cycle arrest and apoptosis. In fact, the lncRNAs NEAT1 and lincRNA-p21 were demonstrated to be induced by p53 during the DNA damage and presumably mediate apoptosis. The upregulation of these lncRNAs was impaired in p53 aberrant B cells (, ) [Citation144] resembling the situation with miR-34a, which was shown to be a direct p53 target [Citation127].
Evading adaptive immune response is another crucial mechanism of cancer progression, and for this purpose, malignant cells use cytokines or trigger co-inhibitory signals via PD-1, CTLA-4, LAG-3, and TIM-3 [Citation145]. Expressing these receptors or their ligands can affect adaptive immune response. TIM-3 is regulated by lncRNAs in T cells not only on the posttranscriptional level but also on the signal transduction level. NEAT1 is highly expressed in T cells infiltrating hepatocellular carcinomas and was shown to sequester miR-155. This miRNA targets TIM-3 and therefore, upregulating NEAT1 induces TIM-3 expression and impairs CD8+ cells activity against the tumour () [Citation146]. On the signal transduction level, lnc-TIM-3 is a recently described cytoplasmatic lncRNA that directly binds TIM-3 to disrupt TCR signalling and promote T cell inhibition () [Citation147]. T cells attack malignant cells releasing IFNγ and perforin, but when exhausted or inhibited, the immune response can be evaded. Malignant cells often upregulate ligands to trigger T cell co-inhibitory receptors to inhibit T cell response. Under INFγ stimulation, cancer cells counteract by expressing an ‘IFN-stimulated non-coding RNA 1ʹ (INCR1) to enhance PD-L1 expression [Citation148]. INCR1 is an antisense RNA from the PD-L1/PD-L2 locus enabling PD-L1 expression via HNRNPH1 sequestration (). INCR1 strongly correlates with PD-L1 expression in several solid tumours and INCR1 knockdown enhanced the efficacy of chimeric antigen receptor (CAR) T cell therapy [Citation148].
LncRNAs are associated with dysregulated pathways and prognostic groups in multiple T/B cell malignancies, which underscores their ability to affect the biology of these immune cells. This also suggests that lncRNAs might serve as novel therapeutic targets, which is supported by recent major advancements in the use of therapeutic oligonucleotides [Citation149,Citation150].
Conclusion and considerations
LncRNAs are an emerging component of adaptive immunity and participate in regulatory circuits dictating lymphocyte development, activation, and differentiation. The key mediators of lymphocyte development regulate many lncRNAs, suggesting their importance in T/B cell maturation steps. IFNG-AS1, Morrbid, lncRNA-AS-GSTT1 are examples of lncRNAs that directly affect T cell response and besides that, lncRNAs also have been shown to influence TH polarization. LncRNAs cooperate with the key transcription factors responsible for T cell polarization and enhance cytokine production required for cell effector functions (). LncRNA dysregulation may also lead to an imbalance in TH populations in the context of autoimmune diseases.
Producing antibodies has been associated with lncRNA expression in B cells, just as lncRNAs expressed by other cells in the microenvironment can influence antibody production by B cells, as suggested by the NEAT1-BAFF axis. Moreover, lncRNAs are important players in the GC reaction by supporting AID functions and promoting class switch recombination (). LncRNAs also impact general B cell biology by regulating survival signals during B cell activation. Dysregulating lncRNAs can impact several pathways and drive lymphomagenesis (i.e. Braf-rs1 and HMGA1P7), while other lncRNAs can cooperate with classical oncogenes such as MYC in malignant B cells (). The genetic aberrations in haematological malignancies also associate with specific lncRNA expression signatures that can potentially be used to distinguish disease subtypes. Genomic aberrations and other alterations found in haematological malignancies are likely to dysregulate lncRNA expression, and this has been demonstrated in some cases (such as p53 aberrations).
In summary, lncRNAs participate in numerous steps required during both cellular and humoral immune responses. Similar to the discovery of miRNAs’ functional role, studying lncRNAs can decipher new molecular mechanisms. The classical literature on adaptive immunity will need to be revisited from this new perspective of lncRNAs.
Authorship contributions
P.Z. and M.M. wrote the manuscript.
Conflict-of-interest disclosure
The authors declare no competing financial interests.
Acknowledgments
This work was supported by the Ministry of Health of the Czech Republic, grant nr. NU20-03-00292. All rights reserved. We would like to thank Laura Ondrisova (CEITEC MU) for the critically reading the manuscript and discussions. The (in part) were created with BioRender.com.
Disclosure statement
No potential conflict of interest was reported by the authors.
Additional information
Funding
References
- Quinn JJ, Chang HY. Unique features of long non-coding RNA biogenesis and function. Nat Rev Genet. 2016 Jan;17(1):47–62.
- Harrow J, Frankish A, Gonzalez JM, et al. GENCODE: the reference human genome annotation for the ENCODE project. Genome Res. 2012;22(9):1760–1774.
- Chan WL, Chang YS, Yang WK, et al. Very long non-coding RNA and human disease. Biomed. 2012;2(4):167–173.
- Fang S, Zhang L, Guo J, et al. NONCODEV5: a comprehensive annotation database for long non-coding RNAs. Nucleic Acids Res. 2018;46(D1):D308–D314.
- Cabili MN, Trapnell C, Goff L, et al. Integrative annotation of human large intergenic noncoding RNAs reveals global properties and specific subclasses. Genes Dev. 2011 Sep 15;25(18):1915–1927.
- Sun M, Kraus WL. From discovery to function: the expanding roles of long noncoding RNAs in physiology and disease. Endocr Rev. 2015;36(1):25–64.
- Perry RBT, Hezroni H, Goldrich MJ, et al. Regulation of neuroregeneration by long noncoding RNAs. Mol Cell. 2018;72(3):553–567.e5.
- Zhang ZK, Li J, Guan D, et al. Long noncoding RNA lncMUMA reverses established skeletal muscle atrophy following mechanical unloading. Mol Ther. 2018;26(11):2669–2680.
- Ivaldi MS, Diaz LF, Chakalova L, et al. Fetal g-globin genes are regulated by the BGLT3 long noncoding RNA locus. Blood. 2018;132(18):1963–1973.
- Fatica A, Bozzoni I. Long non-coding RNAs: new players in cell differentiation and development. Nat Rev Genet. 2014;15(1):7–21.
- Nagano T, Fraser P. Emerging similarities in epigenetic gene silencing by long noncoding RNAs. Mamm Genome. 2009;20(9–10):557–562.
- Stojic L, Lun ATL, Mascalchi P, et al. A high-content RNAi screen reveals multiple roles for long noncoding RNAs in cell division. Nat Commun. 2020;11(1).
- Wang KC, Chang HY. Molecular mechanisms of long noncoding RNAs. Mol Cell. 2011;43(6):904–914.
- Hu G, Tang Q, Sharma S, et al. Expression and regulation of intergenic long noncoding RNAs during T cell development and differentiation. Nat Immunol. 2013;14(11):1190–1198.
- Tay Y, Rinn J, Pandolfi PP. The multilayered complexity of ceRNA crosstalk and competition. Nature. 2014;505(7483):344–352.
- Guo C-J, Ma XK, Xing YH, et al. Distinct processing of lncRNAs contributes to non-conserved functions in stem cells. Cell. 2020;1–16.
- Yoon JH, Abdelmohsen K, Srikantan S, et al. LincRNA-p21 suppresses target mRNA translation. Mol Cell. 2012;47(4):648–655.
- Ransohoff JD, Wei Y, Khavari PA. The functions and unique features of long intergenic non-coding RNA. Nat Rev Mol Cell Biol. 2018;19(3):143–157.
- Signal B, Gloss BS, Dinger ME. Computational approaches for functional prediction and characterisation of long noncoding RNAs. Trends Genet. 2016;32(10):620–637.
- de Santa F, Barozzi I, Mietton F, et al. A large fraction of extragenic RNA Pol II transcription sites overlap enhancers. PLoS Biol. 2010;8(5):e1000384.
- Feyder M, Goff LA, Feyder M, et al. Investigating long noncoding RNAs using animal models Find the latest version: investigating long noncoding RNAs using animal models. J Clin Invest. 2016;126(8):2783–2791.
- Luo H, Zhu G, Xu J, et al. HOTTIP lncRNA promotes hematopoietic stem cell self-renewal leading to AML-like disease in mice. Cancer Cell. 2019;36(6):645–659.e8.
- Luo M, Jeong M, Sun D, et al. Long non-coding RNAs control hematopoietic stem cell function. Cell Stem Cell. 2015;16(4):426–438.
- Coffre M, Koralov SB. miRNAs in B cell development and lymphomagenesis. Trends Mol Med. 2017;23(8):721–736.
- Casero D, Sandoval S, Seet CS, et al. Long non-coding RNA profiling of human lymphoid progenitor cells reveals transcriptional divergence of B cell and T cell lineages. Nat Immunol. 2015;16(12):1282–1291.
- Brazão TF, Johnson JS, Müller J, et al. Long noncoding RNAs in B-cell development and activation. Blood. 2016;128(7):e10–e19.
- Petri A, Dybkær K, Bøgsted M, et al. Long noncoding RNA expression during human B-cell development. PLoS One. 2015;10(9):1–19.
- Wilson A, MacDonald HR, Radtke F. Notch 1-deficient common lymphoid precursors adopt a B cell fate in the thymus. J Exp Med. 2001;194(7):1003–1012.
- Wang Y, Wu P, Lin R, et al. LncRNA NALT interaction with NOTCH1 promoted cell proliferation in pediatric T cell acute lymphoblastic leukemia. Sci Rep. 2015 March;5:1–10.
- Isoda T, Moore AJ, He Z, et al. Non-coding transcription instructs chromatin folding and compartmentalization to dictate enhancer-promoter communication and T cell fate. Cell. 2017;171(1):103–119.e18.
- Dolens A, Durinck K, Lavaert M, et al. Distinct Notch1 and BCL11B requirements mediate human γδ/αβ T cell development. EMBO Rep. 2020;21(5):1–20.
- Abarrategui I, Krangel MS. Noncoding transcription controls downstream promoters to regulate T-cell receptor α recombination. Embo J. 2007;26(20):4380–4390.
- Fu J, Shi H, Wang B, et al. LncRNA PVT1 links Myc to glycolytic metabolism upon CD4+ T cell activation and Sjögren’s syndrome-like autoimmune response. J Autoimmun. 2020 November;107:102358.
- Johnsson P, Morris KV. Expanding the functional role of long noncoding RNAs. Cell Res. 2014;24(11):1284–1285.
- Hogan PG. Calcium–NFAT transcriptional signalling in T cell activation and T cell exhaustion. Cell Calcium. 2017;63:66–69.
- Willingham AT, Orth AP, Batalov S, et al. Molecular biology: a strategy for probing the function of noncoding RNAs finds a repressor of NFAT. Science. 2005;309(5740):1570–1573.
- Sharma S, Findlay GM, Bandukwala HS, et al. Dephosphorylation of the nuclear factor of activated T cells (NFAT) transcription factor is regulated by an RNA-protein scaffold complex. Proc Natl Acad Sci U S A. 2011;108(28):11381–11386.
- Tsao HW, Tai T-S, Tseng W, et al. Ets-1 facilitates nuclear entry of NFAT proteins and their recruitment to the IL-2 promoter. Proc Natl Acad Sci U S A. 2013;110(39):15776–15781.
- Krammer PH, Arnold R, Lavrik IN. Life and death in peripheral T cells. Nat Rev Immunol. 2007;7(7):532–542.
- Huang D, Chen J, Yang L, et al. NKILA lncRNA promotes tumor immune evasion by sensitizing T cells to activation-induced cell death. Nat Immunol. 2018;19(10):1112–1125.
- Vigneau S, Rohrlich P-S, Brahic M, et al. Tmevpg1, a candidate gene for the control of theiler’s virus persistence, could be implicated in the regulation of gamma interferon. J Virol. 2003;77(10):5632–5638.
- Gomez JA, Wapinski OL, Yang YW, et al. The NeST long ncRNA controls microbial susceptibility and epigenetic activation of the interferon-γ locus. Cell. 2013;152(4):743–754.
- Petermann F, Pękowska A, Johnson CA, et al. The magnitude of IFN-γ responses is fine-tuned by DNA architecture and the non-coding transcript of Ifng-as1. Mol Cell. 2019;75(6):1229–1242.e5.
- Hudson WH, Prokhnevska N, Gensheimer J, et al. Expression of novel long noncoding RNAs defines virus-specific effector and memory CD8 + T cells. Nat Commun. 2019;10(1):1–11.
- Agresta L, Hoebe KHN, Janssen EM. The emerging role of CD244 signaling in immune cells of the tumor microenvironment. Front Immunol. 2018 NOV;9:1–9.
- Wang Y, Zhong H, Xie X, et al. Long noncoding RNA derived from CD244 signaling epigenetically controls CD8+ T-cell immune responses in tuberculosis infection. Proc Natl Acad Sci U S A. 2015;112(29):E3883–E3892.
- Kotzin JJ, Iseka F, Wright J, et al. The long noncoding RNA morrbid regulates CD8 T cells in response to viral infection. Proc Natl Acad Sci U S A. 2019;116(24):11916–11925.
- Grogan JL, Locksley RM. Thelper cell differentiation: on again, off again. Curr Opin Immunol. 2002;14(3):366–372.
- Ranzani V, Rossetti G, Panzeri I, et al. The long intergenic noncoding RNA landscape of human lymphocytes highlights the regulation of T cell differentiation by linc-MAF-4. Nat Immunol. 2015;16(3):318–325.
- Zhang F, Liu G, Wei C, et al. Linc-MAF-4 regulates Th1/Th2 differentiation and is associated with the pathogenesis of multiple sclerosis by targeting MAF. Faseb J. 2017;31(2):519–525.
- Collier SP, Collins PL, Williams CL, et al. Cutting edge: influence of Tmevpg1, a long intergenic noncoding RNA, on the expression of Ifng by Th1 cells. J Immunol. 2012;189(5):2084–2088.
- Collier SP, Henderson MA, Tossberg JT, et al. Regulation of the Th1 genomic locus from ifng through Tmevpg1 by T-bet. J Immunol. 2014;193(8):3959–3965.
- Zhang H, Nestor CE, Zhao S, et al. Profiling of human CD4+ T-cell subsets identifies the T H2-specific noncoding RNA GATA3-AS1. J Allergy Clin Immunol. 2013;132(4):1005–1008.
- Gibbons HR, Shaginurova G, Kim LC, et al. Divergent lncRNA GATA3-AS1 regulates GATA3 transcription in T-helper 2 Cells. Front Immunol. 2018 October;9:2512.
- Spurlock CF, Tossberg JT, Guo Y, et al. Expression and functions of long noncoding RNAs during human T helper cell differentiation. Nat Commun. 2015;6.
- Tuomela S, Lahesmaa R. Early T helper cell programming of gene expression in human. Semin Immunol. 2013;25(4):282–290.
- Chien CH, Chiang BL. Regulatory T cells induced by B cells: a novel subpopulation of regulatory T cells. J Biomed Sci. 2017;24(1):1–8.
- Ying Qiu Y, Wu Y, Jie Lin M, et al. LncRNA-MEG3 functions as a competing endogenous RNA to regulate Treg/Th17 balance in patients with asthma by targeting microRNA-17/RORγt. Biomed Pharmacother. 2018;111:386–394. Nov .
- Zhou L, Littman DR. Transcriptional regulatory networks in Th17 cell differentiation. Curr Opin Immunol. 2009;21(2):146–152.
- Ivanov II, Zhou L, Littman DR. Transcriptional regulation of Th17 cell differentiation. Semin Immunol. 2007;19(6):409–417.
- Shui X, Chen S, Lin J, et al. Knockdown of lncRNA NEAT1 inhibits Th17/CD4+ T cell differentiation through reducing the STAT3 protein level. J Cell Physiol. 2019;234(12):22477–22484.
- Li J, Tian J, Lu J, et al. LncRNA GAS5 inhibits Th17 differentiation and alleviates immune thrombocytopenia via promoting the ubiquitination of STAT3. Int Immunopharmacol. 2019;80:106127. Sept .
- Zhang F, Liu G, Li D, et al. DDIT4 and associated lncDDIT4 modulate Th17 differentiation through the DDIT4/TSC/mTOR pathway. J Immunol. 2018;ji1601689. DOI:10.4049/jimmunol.1601689.
- Brajic A, Franckaert D, Burton O, et al. The long non-coding RNA Flatr anticipates Foxp3 expression in regulatory T cells. Front Immunol. 2018 SEP;9:1–12.
- Xiong G, Yang L, Chen Y, et al. Linc-POU3F3 promotes cell proliferation in gastric cancer via increasing T-reg distribution. Am J Transl Res. 2015;7(11):2262–2269.
- Xia M, Liu J, Liu S, et al. Ash1l and lnc-Smad3 coordinate Smad3 locus accessibility to modulate iTreg polarization and T cell autoimmunity. Nat Commun. 2017;8(1). DOI:10.1038/ncomms15818.
- Tone Y, Furuuchi K, Kojima Y, et al. Smad3 and NFAT cooperate to induce Foxp3 expression through its enhancer. Nat Immunol. 2008;9(2):194–202.
- Zemmour D, Pratama A, Loughhead SM, et al. Flicr, a long noncoding RNA, modulates Foxp3 expression and autoimmunity. Proc Natl Acad Sci U S A. 2017;114(17):E3472–E3480.
- Wu Y, Borde M, Heissmeyer V, et al. FOXP3 controls regulatory T cell function through cooperation with NFAT. Cell. 2006;126(2):375–387.
- Jiang R, Tang J, Chen Y, et al. The long noncoding RNA lnc-EGFR stimulates T-regulatory cells differentiation thus promoting hepatocellular carcinoma immune evasion. Nat Commun. 2017 May;8:15129.
- Ondrisova L, Mraz M. Genetic and Non-Genetic Mechanisms of Resistance to BCR Signaling Inhibitors in B Cell Malignancies. Front Oncol. 2020 Oct 26;10:591577.
- Wang F, Luo L, Gu Z, et al. Integrative analysis of long noncoding RNAs in patients with graft-versus-host disease. Acta Haematol. 2020.
- Pyfrom SC, Quinn CC, Dorando HK, et al. BCALM (AC099524.1) Is a human B lymphocyte-specific long noncoding RNA that modulates B cell receptor–mediated calcium signaling. J Immunol. 2020 Jun;ji2000088.
- Sandoval H, Kodali S, Wang J. Regulation of B cell fate, survival, and function by mitochondria and autophagy. Mitochondrion. 2018 November;41:58–65.
- Agirre X, Meydan C, Jiang Y, et al. Long non-coding RNAs discriminate the stages and gene regulatory states of human humoral immune response. Nat Commun. 2019;10(1). DOI:10.1038/s41467-019-08679-z.
- Syrett CM, Sindhava V, Hodawadekar S, et al. Loss of Xist RNA from the inactive X during B cell development is restored in a dynamic YY1-dependent two-step process in activated B cells. PLoS Genet. 2017;13(10):1–28.
- Wang J, Syrett CM, Kramer MC, et al. Unusual maintenance of X chromosome inactivation predisposes female lymphocytes for increased expression from the inactive X. Proc Natl Acad Sci U S A. 2016;113(14):E2029–E2038.
- Bunting KL, SoongTD, Singh R, et al. Multi-tiered reorganization of the genome during B cell affinity maturation anchored by a germinal center-specific locus control region. Immunity. 2016;45(3):497–512.
- Andrews JM, Payton JE. Epigenetic dynamics in normal and malignant B cells: die a hero or live to become a villain. Curr Opin Immunol. 2019;57:15–22.
- Lu Z, Pannunzio NR, Greisman HA, et al. Convergent BCL6 and lncRNA promoters demarcate the major breakpoint region for BCL6 translocations. Blood. 2015;126(14):1730–1731.
- Yu K, Lieber MR. Current insights into the mechanism of mammalian immunoglobulin class switch recombination. Crit Rev Biochem Mol Biol. 2019;54(4):333–351.
- Yewdell WT, Chaudhuri J. A transcriptional serenAID: the role of noncoding RNAs in class switch recombination. Int Immunol. 2017;29(4):183–196.
- Basu U, Meng F-L, Keim C, et al. The RNA exosome targets the AID cytidine deaminase to both strands of transcribed duplex DNA substrates. Cell. 2011;144(3):353–363.
- Pefanis E, Wang J, Rothschild G, et al. Noncoding RNA transcription targets AID to divergently transcribed loci in B cells. Nature. 2014;514(7522):389–393.
- Casellas R, Basu U, Yewdell WT, et al. Mutations, kataegis and translocations in B cells: understanding AID promiscuous activity. Nat Rev Immunol. 2016;16(3):164–176.
- Pefanis E, Wang J, Rothschild G, et al. RNA exosome-regulated long non-coding RNA transcription controls super-enhancer activity. Cell. 2015;161(4):774–789.
- Rothschild G, Zhang W, Lim J, et al. Noncoding RNA transcription alters chromosomal topology to promote isotype-specific class switch recombination. Sci Immunol. 2020;5(44):1–14.
- Mraz M, Dolezalova D, Plevova K, et al. MicroRNA-650 expression is influenced by immunoglobulin gene rearrangement and affects the biology of chronic lymphocytic leukemia. Blood. 2012;119(9):2110–2113.
- Mraz M, Stano Kozubik K, Plevova K, et al. The origin of deletion 22q11 in chronic lymphocytic leukemia is related to the rearrangement of immunoglobulin lambda light chain locus. Leuk Res. 2013;37(7):802–808.
- Mraz M, Pospisilova S. Detection of a deletion at 22q11 locus involving ZNF280A/ZNF280B/PRAME/GGTLC2 in B-cell malignancies: simply a consequence of an immunoglobulin lambda light chain rearrangement. Br J Haematol. 2019;186(4):e91–e94.
- Wu D, Zhou P, Cao F, et al. Expression profiling and cell type classification analysis in periodontitis reveal dysregulation of multiple lncRNAs in plasma cells. Front Genet. 2020 April;11:1–10.
- de Lima DS, Cardozo LE, Maracaja-Coutinho V, et al. Long noncoding RNAs are involved in multiple immunological pathways in response to vaccination. Proc Natl Acad Sci U S A. 2019;116(34):17121–17126.
- Shapira Y, Agmon-Levin N, Shoenfeld Y. Defining and analyzing geoepidemiology and human autoimmunity. J Autoimmun. 2010;34(3):168–177.
- Hrdlickova B, Kumar V, Kanduri K, et al. Expression profiles of long non-coding RNAs located in autoimmune disease-associated regions reveal immune cell-type specificity. Genome Med. 2014;6(10).
- Castellanos-Rubio A, Ghosh S. Disease-associated SNPs in inflammation-related lncRNAs. Front Immunol. 2019 MAR;10:1–9.
- Rankin CR, Shao L, Elliott J, et al. The IBD-associated long noncoding RNA IFNG-AS1 regulates the balance between inflammatory and anti-inflammatory cytokine production after T-cell stimulation. Am J Physiol Gastrointest Liver Physiol. 2020;318(1):G34–G40.
- Hosseini A, Teimuri S, Ehsani M, et al. LncRNAs associated with multiple sclerosis expressed in the Th1 cell lineage. J Cell Physiol. 2019;234(12):22153–22162.
- Masoumi F, Ghorbani S, Talebi F, et al. Malat1 long noncoding RNA regulates inflammation and leukocyte differentiation in experimental autoimmune encephalomyelitis. J Neuroimmunol. 2018;328:50–59. Jul .
- Littlejohn EA, Monrad SU. Early diagnosis and treatment of rheumatoid arthritis. Prim Care Clin Off Pract. 2018;45(2):237–255.
- Zhang T-P, Zhu B-Q, Tao S-S, et al. Long non-coding RNAs genes polymorphisms and their expression levels in patients with rheumatoid arthritis. Front Immunol. 2019;10:2529.
- Wahba AS, Ibrahim ME, Mesbah NM, et al. Long non-coding RNA MEG3 and its genetic variant rs941576 are associated with rheumatoid arthritis pathogenesis in Egyptian patients. Arch Physiol Biochem. 2020 Jul;1–8.
- Peng H, Ren S, Liu Y, et al. Elevated expression of the long noncoding RNA IFNG-AS1 in the peripheral blood from patients with rheumatoid arthritis. J Immunol Res. 2020;2020:1–8.
- Wang J, Peng H, Tian J, et al. Upregulation of long noncoding RNA TMEVPG1 enhances T helper type 1 cell response in patients with Sjögren syndrome. Immunol Res. 2016;64(2):489–496.
- Peng H, Liu Y, Tian J, et al. The long noncoding RNA IFNG-AS1 promotes T helper type 1 cells response in patients with hashimoto’s thyroiditis. Sci Rep. 2015 June;5:1–9.
- Luo M, Liu X, Meng H, et al. IFNA-AS1 regulates CD4+ T cell activation in myasthenia gravis though HLA-DRB1. Clin Immunol. 2017;183:121–131.
- Viatte S, Plant D, Han B, et al. Association of HLA-DRB1 haplotypes with rheumatoid arthritis severity, mortality, and treatment response. J Am Med Assoc. 2015;313(16):1645–1656.
- Barcellos LF, Sawcer S, Ramsay PP, et al. Heterogeneity at the HLA-DRB1 locus and risk for multiple sclerosis. Hum Mol Genet. 2006;15(18):2813–2824.
- Singh S, Singh UG, Agrawal NK, et al. Prevalence of autoantibodies and HLA DR, DQ in type 1 diabetes mellitus. J Clin Diagn Res. 2016;10(7):EC09–EC13.
- Shi FD, He B, Li H, et al. Differential requirements for CD28 and CD40 ligand in the induction of experimental autoimmune myasthenia gravis. Eur J Immunol. 1998;28(11):3587–3593.
- Litinskiy MB, Nardelli B, Hilbert DM, et al. DCs induce CD40-independent immunoglobulin class switching through BLyS and APRIL. Nat Immunol. 2002;3(9):822–829.
- Caro-Maldonado A, Wang R, Nichols AG, et al. Metabolic reprogramming is required for antibody production that is suppressed in anergic but exaggerated in chronically BAFF-exposed B cells. J Immunol. 2014;192(8):3626–3636.
- Mariette X, Roux S, Zhang J, et al. The level of BLyS (BAFF) correlates with the titre of autoantibodies in human Sjögren’s syndrome. Ann Rheum Dis. 2003;62(2):168–171.
- Pers JO, Daridon C, Devauchelle V, et al. BAFF overexpression is associated with autoantibody production in autoimmune diseases. Ann N Y Acad Sci. 2005;1050(33):34–39.
- Dong G, Yang Y, Li X, et al. Granulocytic myeloid-derived suppressor cells contribute to IFN-I signaling activation of B cells and disease progression through the lncRNA NEAT1-BAFF axis in systemic lupus erythematosus. Biochim Biophys Acta - Mol Basis Dis. 2020;1866(1):165554.
- Karreth FA, Reschke M, Ruocco A, et al. The BRAF pseudogene functions as a competitive endogenous RNA and induces lymphoma in vivo. Cell. 2015;161(2):319–332.
- De Martino M, De Biase D, Forzati F, et al. HMGA1-pseudogene7 transgenic mice develop B cell lymphomas. Sci Rep. 2020;10(1):1–12.
- Ghafouri-Fard S, Omrani MD, Taheri M. Long noncoding RNA PVT1: a highly dysregulated gene in malignancy. J Cell Physiol. 2020;235(2):818–835.
- Cho SW, Xu J, Sun R, et al. Promoter of lncRNA gene PVT1 is a tumor-suppressor DNA boundary element. Cell. 2018;173(6):1398–1412.e22.
- Tseng YY, Moriarity BS, Gong W, et al. PVT1 dependence in cancer with MYC copy-number increase. Nature. 2014;512(1):82–86.
- Pear WS, Aster JC, Scott ML, et al. Exclusive development of T cell neoplasms in mice transplanted with bone marrow expressing activated Notch alleles. J Exp Med. 1996;183(5):2283–2291.
- Durinck K, Wallaert A, Van de Walle I, et al. The notch driven long non-coding RNA repertoire in T-cell acute lymphoblastic leukemia. Haematologica. 2014;99(12):1808–1816.
- Trimarchi T, Bilal E, Ntziachristos P, et al. Genome-wide mapping and characterization of notch-regulated long noncoding RNAs in acute leukemia. Cell. 2014;158(3):593–606.
- Fernando TR, Rodriguez-Malave NI, Waters EV, et al. LncRNA expression discriminates karyotype and predicts survival in B-lymphoblastic leukemia. Mol Cancer Res. 2015;13(5):839–851.
- Ronchetti D, Manzoni M, Agnelli L, et al. LncRNA profiling in early-stage chronic lymphocytic leukemia identifies transcriptional fingerprints with relevance in clinical outcome. Blood Cancer J. 2016;6(9):e468.
- Tschumper RC, Shanafelt TD, Kay NE, et al. Role of long non-coding RNAs in disease progression of early stage unmutated chronic lymphocytic leukemia. Oncotarget. 2019;10(1):60–75.
- Mraz M, Chen L, Rassenti LZ, et al. MiR-150 influences B-cell receptor signaling in chronic lymphocytic leukemia by regulating expression of GAB1 and FOXP1. Blood. 2014;124(1):84–94.
- Cerna K, Oppelt J, Chochola V, et al. MicroRNA miR-34a downregulates FOXP1 during DNA damage response to limit BCR signalling in chronic lymphocytic leukaemia B cells. Leukemia. 2019;33(2):403–414.
- Cui B, Chen L, Zhang S, et al. Micro RNA-155 influences B-cell receptor signaling and associates with aggressive disease in chronic lymphocytic leukemia. Blood. 2014;124(4):546–554.
- Garding A, Bhattacharya N, Claus R, et al. Epigenetic Upregulation of lncRNAs at 13q14.3 in Leukemia Is Linked to the In Cis Downregulation of a Gene Cluster That Targets NF-kB. PLoS Genet. 2013;9(4):e1003373.
- Devan J, Janikova A, Mraz M. New concepts in follicular lymphoma biology: from BCL2 to epigenetic regulators and non-coding RNAs. Semin Oncol. 2018;45(5–6):291–302.
- Filip D, Mraz M. The role of MYC in the transformation and aggressiveness of ‘indolent’ B-cell malignancies. Leuk Lymphoma. 2020;61(3):510–524.
- Shi X, Cui Z, Liu X, et al. LncRNA FIRRE is activated by MYC and promotes the development of diffuse large B-cell lymphoma via Wnt/β-catenin signaling pathway. Biochem Biophys Res Commun. 2019;510(4):594–600.
- Lu Y, Hu Z, Mangala LS, et al. MYC targeted long noncoding RNA DANCR promotes cancer in part by reducing p21 levels. Cancer Res. 2018;78(1):64–74.
- Yang X, Ye H, He M, et al. LncRNA PDIA3P interacts with c-Myc to regulate cell proliferation via induction of pentose phosphate pathway in multiple myeloma. Biochem Biophys Res Commun. 2018;498(1):207–213.
- Xiao X, Gu Y, Wang G, et al. c-Myc, RMRP, and miR-34a-5p form a positive-feedback loop to regulate cell proliferation and apoptosis in multiple myeloma. Int J Biol Macromol. 2019 Feb;122:526–537.
- Hu G, Lou Z, Gupta M. The long non-coding RNA GAS5 cooperates with the eukaryotic translation initiation factor 4E to regulate c-Myc translation. PLoS One. 2014;9(9).
- Tsutsumi Y, Chinen Y, Sakamoto N, et al. Deletion or methylation of CDKN2A/2B and PVT1 rearrangement occur frequently in highly aggressive B-cell lymphomas harboring 8q24 abnormality. Leuk Lymphoma. 2013;54(12):2760–2764.
- Ghesquières H, Larrabee BR, Casasnovas O, et al. A susceptibility locus for classical Hodgkin lymphoma at 8q24 near MYC/PVT1 predicts patient outcome in two independent cohorts. Br J Haematol. 2018;180(2):286–290.
- Zhao X, Lwin T, Zhang X, et al. Disruption of the MYC-miRNA-EZH2 loop to suppress aggressive B-cell lymphoma survival and clonogenicity. Leukemia. 2013;27(12):2341–2350.
- Musilova K, Devan J, Cerna K, et al. miR-150 downregulation contributes to the high-grade transformation of follicular lymphoma by upregulating FOXP1 levels. Blood. 2018;132(22):2389–2400.
- Hu G, Gupta SK, Troska TP, et al. Long non-coding RNA profile in mantle cell lymphoma identifies a functional lncRNA ROR1-AS1 associated with EZH2/PRC2 complex. Oncotarget. 2017;8(46):80223–80234.
- Wang X, Sehgal L, Jain N, et al. Lncrna malat1 promotes development of mantle cell lymphoma by associating with ezh2. J Transl Med. 2016;14(1):1–14.
- Sehgal L, Mathur R, Braun FK, et al. FAS-antisense 1 lncRNA and production of soluble versus membrane Fas in B-cell lymphoma. Leukemia. 2014;28(12):2376–2387.
- Blume CJ, Hotz-Wagenblatt A, Hüllein J, et al. P53-dependent non-coding RNA networks in chronic lymphocytic leukemia. Leukemia. 2015;29(10):2015–2023.
- Rabinovich GA, Gabrilovich D, Sotomayor EM. Immunosuppressive strategies that are mediated by tumor cells. Annu Rev Immunol. 2007;25(1):267–296.
- Yan K, Fu Y, Zhu N, et al. Repression of lncRNA NEAT1 enhances the antitumor activity of CD8 + T cells against hepatocellular carcinoma via regulating miR-155/Tim-3. Int J Biochem Cell Biol. 2019 January;110:1–8.
- Ji J, Yin Y, Ju H, et al. Long non-coding RNA Lnc-Tim3 exacerbates CD8 T cell exhaustion via binding to Tim-3 and inducing nuclear translocation of Bat3 in HCC. Cell Death Dis. 2018;9(5).
- Mineo M, Lyons SM, Zdioruk M, et al. Tumor interferon signaling is regulated by a lncRNA INCR1 transcribed from the PD-L1 locus. Mol Cell. 2020;78(6):1207–1223.e8.
- Levin AA. Treating disease at the RNA level with oligonucleotides. N Engl J Med. 2019;380(1):57–70.
- Musilova K, Mraz M. MicroRNAs in B-cell lymphomas: how a complex biology gets more complex. Leukemia. 2015;29(5):1004–1017.