Abstract
There is evidence for immunotoxicity of aflatoxin B1 (AFB1) in chronic animal feeding studies; however, little information is available as to the effects of inhalation exposure. This study evaluated the acute affects of aerosolized AFB1 on systemic immune function of female C57BL/6N mice following a single aerosol exposure. Mice were exposed in nose-only inhalation tubes to 0, 2.86, 6.59 and 10 μg AFB1 aerosol/L air for 90 minutes. A negative control group of untreated mice and a positive control group of cyclophosphamide-treated mice were included to account for day to day variation. Three days following exposure, mice were sacrificed and body, liver, lung, thymus and spleen weights, and complete blood counts and white blood cell differentials were measured. Splenocytes were isolated for flow cytometric analysis of CD4+ and CD8+ lymphocytes, CD19+ B-cells and natural killer cells (NK 1.1+). The effect of AFB1 on humoral immunity was assessed by measuring serum anti-keyhole limpet hemocyanin (KLH) IgM levels. Of the tissues examined, only the thymus weight of AFB1 exposed mice decreased significantly compared to naïve mice; however, the decrease was not dose related and was also observed in the 0 AFB1 aerosol control group. A decrease in the mean white blood cell count of treated vs. naïve mice was observed at all dose levels but was clearly not dose related and was statistically significant only in the 0 and 2.86 μg/L groups. Red blood cell and platelet counts and white blood cell differentials were not significantly affected by AFB1. The number of CD4+ (helper T-cells), CD8+ (cytotoxic T-cells) and CD19+ (B-cells) decreased in spleens of AFB1 aerosol exposed mice compared to naïve mice; however, the decrease was not dose-related and was also observed in the 0 AFB1 exposure group. Dose-related changes in the CD4+/CD8+ T-lymphocyte ratios were not observed. The IgM response to KLH was not significantly different in AFB1 compared to naïve mice, suggesting that AFB1 did not effect antigen-specific antibody production. Based on the results of this study, a single AFB1 inhalation exposure up to 10 μg/L for 90 minutes (CxT = 900 μg ·min/L) did not significantly alter the immune parameters measured in this study. The aerosol vehicle (ethanol) and/or stress could have masked subtle AFB1-dependent changes in thymus and spleen weights, and in splenic lymphocyte subpopulations. However, for other immunological parameters, such as the IgM response to KLH, there was clearly no significant effect of AFB1 aerosol exposure.
INTRODUCTION
Aflatoxin B1 (AFB1), a metabolite of the fungus Aspergillus flavus, is a potent hepatocarcinogen in experimental animals and humans (Groopman, Citation1988). Aflatoxin (AF) contaminated food has been implicated as a major cause of liver cancer in many parts of Africa and Asia (National Toxicology Program, Citation2002). Dietary AF has been implicated as a factor in outbreaks of infectious disease in domesticated animals (Pier et al., Citation1980; Pier, Citation1981; Pier and McLoughlin, Citation1985; Panangala et al., Citation1986; Padmanaba, Citation1989). In addition, there is experimental evidence indicating that AF reduces resistance to bacterial, viral, fungal, and parasitic infections in swine, rabbits, and chickens (Michael et al., Citation1973; Richard et al., Citation1973; Cysewski et al., Citation1975; Miller et al., Citation1978; Ghoneimy et al., Citation2000).
A number of studies have documented the immunotoxic potential of dietary AF in several species including laboratory and domestic animals (reviewed in Sharma, Citation1993; Bondy and Pestka, Citation2000). Studies in domesticated animals have shown that AF ingestion causes thymus and bursal hypertrophy in chickens (Thaxton et al., Citation1974), immunosuppression in turkeys (Pier and Heddleston, Citation1970; Pier et al., Citation1972; Richard et al., Citation1973), impaired bovine lymphocyte mitogenesis (Paul et al., Citation1977), suppressed antibody response to Salmonella in chickens (Giambrone et al., Citation1978), suppressed cell-mediated immunity in chickens (Ghosh et al., Citation1990), and delayed-type hypersensitivity in chickens (Kadian et al., Citation1988). In pigs, AF decreased mitogen-induced lymphocyte proliferation, complement titers, macrophage activation, and the delayed hypersensitivity response (Miller et al., Citation1978; Silvotti et al., Citation1997; Mocchegiani et al., Citation1998). Oral doses of AF decreased the number of lymphoid cells and reduced peritoneal macrophage phagocytosis in weanling rats (Raisuddin et al., Citation1990), reduced lymphocyte blastogenesis and antibody response in mice (Reddy et al., Citation1987), impaired alveolar macrophage phagocytosis in rabbits (Richard and Thurston, Citation1975), and reduced complement activity in guinea pigs (Thurston et al., Citation1972, Citation1974). Low dose AF reduced the humoral immune response induced by Mycoplasma agalactiae (Marin et al., Citation2002).
AF ingestion does not appear to alter immunoregulatory cytokine production (Dugyala and Sharma, Citation1996; Watzl et al., Citation1999; Marin et al., Citation2002) or the humoral response to sheep red blood cells (van Heugten et al., Citation1994) and Erysipelothrix rhusiopathiae (Panangala et al., Citation1986). Weanling pigs fed low doses of AF for 4 weeks, displayed no alterations in the relative numbers of blood cells, globulin, albumins, serum protein concentration, or phytohemagglutinin (PHA)-induced expression of IL-2 or IL-4 using whole blood, indicating that there was no effect of AF on the cell-mediated immune response (Marin et al., Citation2002).
Five studies with aerosol exposure to AF have been reported (Louria et al., Citation1974; Northrup et al., Citation1975; Richard et al., Citation1982; Zarba et al., Citation1992); however, only one study examined the immunotoxicity of AF (Jakab et al., Citation1994). Jakab et al. (Citation1994) observed suppression of alveolar macrophage Fc-receptor mediated phagocytosis following aerosol inhalation of AFB1 in rats. Suppression of systemic immune function, as determined by splenic antibody response to sheep red blood cells and suppression of peritoneal macrophage phagocytosis, was observed with intratracheal instillation of AFB1 at 10-fold higher doses compared to inhalation.
Inhalation of AFB1 from grain dust may be a risk factor for a number of human diseases (Zarba et al., Citation1992). AFB1 levels of 12 to 700 ppb were measured in airborne dust during handling and processing of peanut and corn grain (Burg et al., Citation1981; Sorenson et al., Citation1981, Citation1984). In addition, there are numerous studies identifying increased incidences of total and respiratory cancer in aflatoxin-exposed workers (Hayes et al., Citation1984 and NTP, 2002). Since immune surveillance is an important protective mechanism against the development of cancer cells, suppression of the immune response could be at least partially responsible for increased risk of carcinogenicity. In this study we investigated the effects of AFB1 aerosol on immune parameters in female C57BL6 mice at three dose levels. Analyses included characterization of cells and organs of the immune system, hematology, spleen cell immunophenotyping, and T-cell dependent IgM response to KLH.
MATERIALS AND METHODS
Animals
Female C57BL/6N mice, approximately 7–9 weeks old on the day of dosing, were obtained from Charles Rivers (Wilmington, MA) and housed in a controlled environment with a 12-h light/dark cycle. Food and water were provided ad libitum. The study adhered to the Guide for the Care and Use of Laboratory Animals prepared by the Institute of Laboratory Animal Resources, National Research Council (CitationNational Academy Press, 1996).
Test Chemical
Aflatoxin B1 (FW 312.3) from Aspergillus flavus (99% purity by TLC) was purchased from Sigma Chemical Co. (St. Louis, MO). The identity of the stock AFB1 was verified by LC/MS. A 10 μ L aliquot of a 1 μ g/mL solution of AFB1 in methanol was injected on a 150 × 3.2 mm Allure C-18 column with a MicroMass Quattro MS/MS detector. The LC column was eluted for 16 min at 0.4 mL/min using with a linear gradient from 20% to 80% methanol containing 2 mM formic acid and 2 mM ammonium formate followed by a 0.1 min ramp back to 20% methanol containing 2 mM formic acid and 2 mM ammonium formate and holding for 4 min. The eluent was analyzed by MS in the ESP+ mode by scanning from mass/charge ratio (m/z) 10 to m/z 500 in 1.5 sec. LC/MS of the stock AFB1 gave a single chromatographic peak for m/z 313.2 at 17.8 min. MS analysis of this peak gave a major ion at 313 (MH+) and 335 (M+Na)+. Nebulizer solutions of AFB1 (0.05, 0.20 or 0.60 mg AFB1/mL ethanol) were prepared on the day of use.
Other Chemicals
Cyclophosphamide monohydrate, ethanol (200 proof, spectrophotometric grade, > 99% purity), bovine serum albumin (BSA), paraformaldehyde, sodium azide, red blood cell lysing buffer, and DNase and RNase free water were obtained from Sigma-Aldrich (St. Louis, MO). Modified Eagle medium (MEM) with 100 mM sodium pyruvate and RPMI medium 1640 with Glutamax I were obtained from Invitrogen (Carlsbad, CA). 10X Calcium- and magnesium-free phosphate-buffered saline, pH 6.7–6.9 (10X CMF PBS) was obtained from In Vitro Life Technologies (Carlsbad, CA). Penicillin-streptomycin (10,000 units penicillin, 10 mg/mL streptomycin) was obtained from Amresco (Solon, OH). Keyhole limpet hemocyanin (KLH), lyophilized powder, was obtained from Pierce (Rockford, IL) and was regenerated in sterile distilled water (Sigma). Horseradish peroxidase (HRP)-conjugated rat anti-mouse IgM monoclonal antibody was obtained from BD Biosciences Pharmingen (San Diego, CA). Mouse IgM was obtained from Caltag (Burlingame, CA). The monoclonal antibodies used for spleen cell phenotyping were rat anti-mouse CD3-R-phycoerythrin (R-PE), rat anti-mouse CD4-fluorescein isothiocyanate (FITC), rat anti-mouse CD8a-FITC, rat anti-mouse CD19-FITC (Caltag), and mouse anti-mouse (NK1.1-FITC) (BD Biosciences Pharmingen). The matched isotype control antibody conjugates used to determine background staining were anti-mouse IgG2a isotype control-R-PE and anti-mouse IgG2a isotype control-FITC (Caltag), and anti-mouse IgG2a isotype control-FITC (BD Biosciences Pharmingen).
Experimental Design
There were four aerosol exposure groups in this study; 0, 2.86, 6.59, and 10.0 μ g AFB1/L air (). Each aerosol treatment group contained 21 mice that were exposed once to the aerosol for 90 min. Each aerosol treatment group was exposed on a different day. To account for day-to-day variation, an additional group of 21 naïve mice (Untreated Control) was processed and analyzed simultaneously with samples from the AFB1 aerosol exposed animals on each day of exposure. On each day of aerosol exposure an additional group of 21 naïve animals was treated with cyclophosphamide, a known immunosuppressant, intraperitoneally (IP) at 225 mg/kg body weight (BW); these animals served as positive controls for the immunological analyses.
TABLE 1 Experimental design
Of the 21 mice in each group, 7 were used for analysis of the IgM response to KLH and the other 14 mice for the other analyses. For analyses of splenocytes, the spleens from 14 mice were harvested and processed in sets of two (n = 7).
AFB1 Aerosol Exposure
Female C57BL/6N mice were divided randomly by weight into three groups: aerosol-treated, untreated, and cyclophosphamide-treated mice. Mice were acclimated to exposure tubes for 0.5 and 1 h on the two days prior to aerosol exposure.
Mice were exposed once for 90 min to an atmosphere of 0, 2.86, 6.59, or 10.0 μ g AFB1 aerosol/L air. The exposure atmosphere also contained ethanol vapor due to solubilization of the AFB1in ethanol for nebulization. The AFB1 aerosol exposure was generated by nebulization of solutions of 0, 0.05, 0.20, or 0.60 mg AFB1/mL ethanol using a three jet Collison nebulizer and was delivered to rodents via a nose-only inhalation system (CH Technologies, Westwood, NJ). Total air-flow through the system was regulated to give approximately 10 L/min through the chamber. Homogeneity of an aerosol in the exposure chamber was verified with 0.7–2.9 μm polystyrene latex beads (PSL) at aerosol concentrations of 50,000 to 500,000 particles/L. PSL concentration values at all levels of the exposure chamber were within 10% of the mean. Since the AFB1 aerosol has a smaller particle size than the standard PSL particles; it can be safely assumed to distribute in a similar homogeneous manner throughout the system.
The aerosol atmosphere was sampled through two serial 0.2 μ m filters. The aerosol particle size was estimated as the mass median aerodynamic diameter (MMAD) using a PIXE cascade impactor, Model I-1, PIXE International Corp. (Tallahassee, FL). Filters and slides from the cascade impactor were extracted with 200-proof ethanol and analyzed by UV spectroscopy at 260 nm. A standard curve was prepared using a standard solution of AFB1 in 200-proof ethanol. The aerosol concentration in the exposure chamber was calculated from the amount of AFB1 collected on filters as:
Following exposure, the animals were placed in cages (2–4 per cage) and provided food and water ad libitum until sacrifice, at 3–5 days following exposure. All animals were weighed at randomization (3 or 4 days prior to exposure) and at sacrifice (3 days after aerosol exposure). Clinical observations were performed twice daily from the quarantine period through the day of termination.
IgM Response to KLH
Animals (7 per treatment group) were injected IP with KLH (0.2 mL of 0.5 mg KLH/mL sterile phosphate-buffered saline, pH 7.2) 1 day following AFB1 aerosol or cyclophosphamide dosing. Untreated (control) animals were injected the same day. Five days following exposure (4 days after KLH injection), a blood sample was collected by retro-orbital bleed (or heart puncture if insufficient blood was obtained) under halothane anesthesia into a serum collection tube. Sera was stored at ≤ −70°C and analyzed within 4 days of collection for the concentration of anti-KLH IgM.
Body Weight, Hematology, and Organ Weights
Three days following aerosol exposure, 14 aerosol-treated, 14 cyclophosphamide-treated, and 14 untreated mice were weighed, and a blood sample was collected by retro-orbital bleed under CO2 anesthesia into a potassium-EDTA tube for hematology. Blood from individual animals was analyzed for white blood cell count (WBC), red blood cell count (RBC), hemoglobin (HGB), hematocrit (HCT), mean corpuscular volume (MCV), mean corpuscular hemoglobin (MCH), mean corpuscular hemoglobin concentration (MCHC), red cell distribution width (RDW), platelet count (PLT), mean platelet volume (MPV), and white blood cell differentials.
The mice were euthanized while under anesthesia by cervical dislocation. The body of the rodent was washed with 95% ethanol and the spleen was removed aseptically, placed in a sterile container, and weighed. The liver, thymus, and lung were removed and weighed individually.
Isolation of Splenocytes
Spleens were transferred to dishes (two per dish) containing 5–10 mL of growth medium (RPMI 1640 containing 10% FBS, 10 μ g/mL gentamycin). Each spleen was macerated between the frosted ends of two sterile glass slides. Splenocytes were collected and transferred to sterile conical tubes. Samples were centrifuged for 5 min at 350 × g at room temperature to pellet the cells. The supernatant was removed and red blood cells were lysed using Red Blood Cell Lysing Buffer. Splenocytes were pelleted, washed with growth medium and resupended in 2 mL of fresh growth medium. Total splenocyte counts and viability were determined using a hemocytometer. A portion of each cell solution was diluted to 1.0 × 107 cells/mL and used for flow cytometric analysis. The average cell viability was > 94% for all groups of animals (range of 87.3–99.8%) except the cyclophosphamide treated mice which showed a slightly lower mean viability of 91.4% (range of 80.0–98.9%) These results indicate that the isolated splenocytes retained viability and were suitable for subsequent analyses.
Immunophenotyping
Immunostaining for CD3, CD4, CD8, CD19 and NK 1.1 antigenic markers was performed on the day of splenocyte isolation. Briefly, 20 μ L aliquots of the appropriate antibody solutions were added to the wells of a 96-well microplate. The volume of each well was brought to 100 μ L using PBS/BSA/azide wash buffer (0.2% bovine serum albumin and 0.2% sodium azide in 1X-CMF PBS). An aliquot (100 μ L) of a solution containing 1.0 × 107 splenocytes/mL was added to each assay well for a final well volume of 200 μ L. The cells were incubated for 1 hr at 37°C in the dark. The plate was centrifuged at 210 × g for 3 min and washed twice with PBS/BSA/azide buffer. The cell suspension in PBS/BSA/azide was added to an equal volume of 2% paraformaldehyde and stored at 2–8°C protected from light until analyzed. Analysis of splenic lymphocyte populations was performed on a FACScan (Becton Dickinson, San Jose, CA) using Cell Quest software (Becton Dickinson, San Jose, CA). Splenocytes were gated by means of forward and side scatter, and approximately 10,000 gated cells were analyzed.
Statistical Analysis
Comparisons between treated and untreated mice were made using the Student's 2-tailed t-test with a p value of ≤ 0.05 being considered significant.
RESULTS
AFB1 Aerosol Exposures
The aerosol concentrations for the 90 min exposures were 0, 2.86, 6.59 and 10.0 μ g AFB1/L air () equivalent to C × T exposures of 0, 257, 593, and 900 μ g·min/L air. The amount of AFB1 inhaled in the four exposures was estimated to be 0, 5.1, 11.9 and 18.0 μ g assuming a mouse minute volume of 20 mL/ min. Regression analysis of impactor data indicated that the aerosol had a MMAD range of 0.04–0.2 μm over the three AFB1 exposures. This particle size ensures significant alveolar deposition of the AFB1 aerosol. The estimated exposure concentration of the vehicle, ethanol, was similar for all aerosol exposures and ranged from 50.4–67.8 mg/L air.
TABLE 2 Exposure concentration of AFB1 aerosol
Body Weights
There was no effect of AFB1 exposure on body weight (data not shown). The cyclophosphamide-treated mice showed a slight decrease in average weight gain compared to untreated mice; however, this was statistically significant on only one of the four exposure days.
Organ Weights
A summary of the effect of treatment on organ weights and organ/body weight ratios are shown in and , respectively. Although some apparent statistical differences in liver weight and liver/body weight ratio were observed in AFB1 treated versus untreated mice, these were within 10% and were not dose related. Although lung weight and lung/body weight ratio were significantly increased (18%) compared to untreated mice in the mid-dose group (6.59 μ g/L), this increase was not dose related; the high-dose AFB1-treated mice had the same mean lung weight as the untreated mice. Thymus weights in aerosol exposed mice decreased significantly (20–30%) compared to untreated mice, however, the decrease was similar at all AFB1 exposure levels (including the 0 AFB1 level) indicating that this effect was not due to AFB1, but most likely due to either ethanol exposure (Budec et al., Citation1992, Budec, Citation1996) and/or a stress response caused by restraint of animals in nose-only exposure tubes. A similar decrease in spleen weight was observed for aerosol exposed mice, but was only statistically significant at the 0 AFB1 exposure level, indicating that the effect was not due to AFB1.
TABLE 3 Summary of organ weights
TABLE 4 Summary of organ/body weight ratios
Cyclophosphamide affected the mean liver weight slightly, and increased the mean lung weight 17–37%. Cyclophosphamide consistently decreased thymus (54–68% decrease) and spleen (47–56%) weights.
Hematology
Although statistically different only at the 0 and 2.86 μ g/L level, the WBC count was lower than that of naïve mice at all AFB1 aerosol concentrations (). This decrease in WBC is not due to AFB1 since the same decrease is seen in the 0 AFB1 (vehicle) exposure. There was no effect of aerosol exposures on blood RBC count, HCT, HBG, MCH, MCHC, MCV, or RDW (data not shown). Significant changes in platelet count and mean platelet volume related to aerosol exposures were not observed. There was no consistent AFB1 aerosol exposure related change in percent lymphocytes, monocytes, neutrophils, eosinophils, or basophils (data not shown).
TABLE 5 White blood cell count
Cyclophosphamide decreased the WBC count by 81–90% () and caused significant decreases in HCT (11–15%), HBG (10–18%), and RDW on all exposure days and a decrease in platelet count on three of the four exposure days. The percentage of neutrophils and eosinophils was significantly increased by cyclophosphamide.
Spleen Cell Immunophenotype
Flow cytometry was used to evaluate lymphocyte subpopulations in the spleen. Total T-cells (CD3+), T-helper cells (CD4+), cytotoxic T-cells (CD8+), B-cells (CD19+), and natural killer (NK) cells (CD3−/NK1.1+) are expressed as total cells per spleen (). A statistically significant decrease in total T- and B-lymphocytes per spleen was observed at 0 μ g AFB1 /L air as well as at 6.59 and 10.0 μ g AFB1 /L air indicating that this effect was not due to AFB1 exposure. Levels of NK cells were not significantly affected by AFB1 exposure. Cyclophosphamide caused a decrease in all subpopulations of lymphocytes.
FIG. 1 Splenocytes were isolated from mouse spleen three days following exposure to aerosol containing 0 μ g AFB1/L (aerosolized vehicle, ethanol; A), 2.86 μ g AFB1/L (B), 6.59 μ g AFB1/L (C) or 10.0 μ g AFB1/L (D). The quantities of different lymphocyte subpopulations for aerosol-exposed animals is shown by the solid bar. Lymphocyte subpopulations of splenocytes isolated from untreated mice and cyclophosphamide-treated mice on the same day are illustrated by the open and dotted bars, respectively. Antigenic markers were used to identify and quantitate T-cells (CD3+), helper T-cells (CD3+/CD4+), cytotoxic T-cells (CD3+/CD8+), B cells (CD19+) and natural killer cells (NK1.1+) from the isolated splenocytes by fluorescent-activated cell sorting (FACS) as described in the Methods. An asterisk indicates that the value is significantly different from the untreated mice (open bar) using a Student's 2-tailed t-test at p ≤ 0.05.
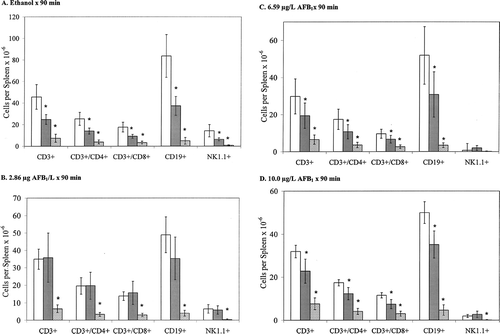
The CD4+/CD8+ ratio was statistically decreased in the 6.59 μ g AFB1/L group and increased in the 10.0 μ g AFB1/L group compared to untreated mice from the same day (). These changes in the CD4+/CD8+ ratio were within the day-to-day variation observed with untreated mice, suggesting that this finding was not related to AFB1 aerosol exposure.
TABLE 6 Effect of AFB1 on CD4+/CD8+ ratio of mouse splenocytes
IgM Response to Keyhole Limpet Hemocyanin (KLH)
A significant IgM response to KLH was detected in all groups of mice. There was no significant immunosuppressive effect of the aerosol vehicle (0 AFB1) or any of the AFB1 aerosol concentrations tested on the anti-KLH IgM response of mice (). Although not always statistically significant due to variability in the individual mouse response, cyclophosphamide caused a decrease of the mean anti-KLH IgM response on all exposure days.
TABLE 7 IgM response to keyhole limpet hemocyanin (KLH)
DISCUSSION AND CONCLUSIONS
Exposure of female C57BL/6N mice to aerosolized AFB1 at concentrations of 2.9 to 10 μ g/L for 90 min had no significant effect on the immune parameters analyzed in this study. AFB1 did not affect measures of acute toxicity such as organ and body weight, including organs specifically involved in the immune system, such as thymus and spleen. Hematology and white blood cell differentials, a measure of the circulating levels of immune cells also were unaffected by AFB1. Splenic T-cell (CD4+ and CD8+), B-cell (CD19+) and natural killer cell (CD3−/NK1.1+) populations were unaffected by AFB1 aerosol exposure as determined by flow cytometric analysis. AFB1 did not affect the T-cell-dependent antibody response of mice as shown by a lack of inhibition of the IgM response to KLH. Based on comparison to the aerosol control (0 AFB1), the lymphocyte subpopulation profile (white blood cell differential) was not altered by AFB1 exposure. These results indicate that a single, acute exposure to AFB1 aerosol at concentrations up to 10 μ g/L for 90 min is unlikely to cause appreciable systemic immunotoxicity in the short term. Higher concentrations of AFB1 and/or multiple exposures may be needed to observe the immunotoxic effects reported in previous feeding studies.
In this study, some measures of immune parameters were inhibited by aerosol exposure in the presence or absence of AFB1 indicating that the immunosuppression was either due to the vehicle (ethanol vapor; 0 AFB1), the stress response caused by restraint of mice in nose-only exposure tubes, or a combination of the two. High levels of ethanol consumption are known to affect the immune system (Szabo, Citation1999). Previous studies have reported that an oral dose of ethanol (4 to 7 g/kg) to B6C3F1 mice slightly decreased the number of nucleated cells in the spleen but did not effect the percentage of CD4+ and CD8+ cells (Pruett et al., Citation2003). These results are similar to those observed in this study using ethanol vapor. Assuming a minute volume of 20 mL/min, mouse body weight of 0.02 kg, ethanol vapor concentration of 60 mg/L, and 50–100% absorption of the inhaled dose, the maximum ethanol dose to mice in this study was 2.7–5.4 g/kg BW which is within the range used by Pruett et al. (Citation2003).
Although mice were acclimated to exposure tubes prior to exposures, the possibility of some degree of stress cannot be ruled out. Restraint has been shown to transiently increase serum corticosterone levels, indicative of a stress response. This stress response decreased expression of MHC Class II receptors on splenocytes and NK cell activity within 12 h of the start of restraint (Pruett et al., Citation1999, Citation2003). In addition, the IgG response to KLH at 2 weeks after challenge was inhibited by stress (Pruett et al., Citation1999, Citation2003). In contrast, we did not observe an inhibition of the IgM response to KLH measured in our study.
The estimated inhaled dose of AFB1 in this study ranged from 5–18 μ g per mouse over a 90 min exposure (). Assuming mice have the same percent alveolar deposition as rats (15%; Schlesinger, 1985), and a mouse body weight of 20 g, the highest dose level used in this study equates to an alveolar dose of 135 μ g AFB1/kg. Jakab et al. (Citation1994) reported a local immunosuppressive effect of AFB1 on alveolar macrophage phagocytosis in male Fischer 344 rats following inhalation exposure to 33.6 μ g AFB1/kg BW, however other immune functions using this route of exposure were not examined. At an approximately four-fold higher dose (135 μ g/kg BW), we observed no evidence of acute systemic AFB1 immunotoxicity following inhalation exposure. It appears that for systemic immunosuppressive effects to occur a much higher dose, such as that delivered by intratracheal instillation (Jakab et al., Citation1994), or orally (Bondy and Pestka, Citation2000), would be required. The low solubility of AFB1 in biologically compatible solvents limits achieving higher inhalation doses by nebulization of a solution.
Local immunotoxic effects of AFB1 on lymphocyte populations in the lung were not measured in this study. Since AFB1 has been shown to suppress alveolar macrophage function (Jakab et al., Citation1994), it is quite possible that there may also be effects on alveolar lymphocytes as well. Further investigation of this area is warranted, as local suppression of lung alveolar lymphocytes would be expected to increase host susceptibility to respiratory infections.
Hatori et al. (Citation1991) orally dosed male C57Bl/6 mice with AFB1 once daily for 4 weeks at doses up to 750 mg/kg BW. Consistent with our findings in female C57BL/6N mice, Hatori et al. (Citation1991) observed no effect of treatment on helper T-cells (L3T4), suppressor and cytotoxic T-cells (Lyt 2), and the L3T4/Lyt 2 ratio at the 150 μ g AFB1/kg dose level. AFB1 had no effect on PHA-stimulated proliferation, mixed lymphocyte culture response, or NK cell activity at doses up to 750 μ g/kg BW. A slight (approximately 17%) decrease in plasma anti-sheep red blood cell IgM concentration was observed at 150 and 750 μ g/kg BW (Hatori et al., Citation1991) despite 14 days of treatment compared to our study that was a single inhalation dose.
In contrast to our findings, Hatori et al. (Citation1991) reported a dose-related decrease in leukocyte count, a significant (∼ 20%) decrease in helper T-cells (L3T4) at the highest dose (750 μ g/kg), and an inhibition of Con-A, LPS, and pokeweed mitogen-induced proliferation of spleen lymphocytes (150 or 750 μ g AFB1/kg). However, these mice were exposed orally, daily for 4 weeks at higher doses (up to 750 μ g/kg BW) than that achieved in our single inhalation dose study (estimate of 135 μ g/kg BW). Hatori et al. (Citation1991) reported that the oral dose of AFB1 necessary to elicit immunosuppressive effects on the blastogenic response, IL-2 production, and primary antibody production of splenic cells in male C57Bl/6 mice was higher than required in previous studies using male BALB/c and male CD-1 mice.
Consistent with our study, Dugyala and Sharma (Citation1996) reported no effect of AFB1 on liver, kidney, spleen and thymus weights, and red blood cell and white blood cell concentrations at oral doses up to 700 μ g AFB1/kg BW administered every other day for 2 weeks to male CD-1 mice. This study also observed no significant effect of AFB1 treatment on Con-A-induced production of IL-2, IFNγ, and IL-3 by splenic lymphocytes. A slight and non-dose-dependent AFB1 inhibition of LPS-induced macrophage production of IL-1, TNF, and IL-6 was observed. Dugyala et al. (Citation1994) reported a significant decrease in proliferation and differentiation of granulocyte-macrophage progenitor cells in bone marrow cultures from male CD-1 mice treated with 145 to 700 μ g AFB1/kg BW every other day for 2 weeks. A similar inhibition of granulocyte-macrophage progenitor cell proliferation was observed in vitro at approximately 10 μ M AFB1.
Raisuddin et al. (Citation1994) reported decreased thymus and spleen weights and cellularity, white blood cell and red blood cell counts, phagocytic function, mitogenesis of T- and B-cells, and delayed-type hypersensitivity responses in male Wistar rats at a dose of 300 μ g AFB1/kg BW orally for two weeks every other day. Differences from the findings of our inhalation study may be due to the higher dose, the route of exposure, and/or the difference in species (rat vs. mice).
In summary, our results indicate that a single inhalation exposure to aerosolized AFB1 at an exposure up to 900 μ g· min/L (CxT) does not result in a significant suppression of the systemic immune system. However, it should be kept in mind that subtle effects of AFB1 on some immune parameters may be masked by the effects of the aerosol vehicle (ethanol) and/or stress on some components of the immune system.
ACKNOWLEDGMENTS
The authors acknowledge L.M. Baker, P.A.S. Reuther, K.E. Gebhardt, E.B. Delpit, T. Brown, R. Ramsey, J. Truxall, P. Herr-Calomeni, G. Sparks, S. Sabourin, C. Singer, N. Coons, J. Bailey, Z. King, J. Ritchie, K. Krieg, and A. Moran for their skillful technical assistance.
REFERENCES
- Bondy G. S., Pestka J. J. Immunomodulation by fungal toxins. J. Toxicol. Environ. Health B Crit. Rev. 2000; 3: 109–143, [PUBMED], [INFOTRIEVE], [CSA]
- Budec M. Acute effect of ethanol on rat thymus: Relationship to dose. 12th International Conference on Lymphoid Tissue and Germinative Centers in Immune Reactions. Graz, Austria 1996; 225, [CSA]
- Budec M., Ciric O., Koko V., Asanin R. The possible mechanism of action of ethanol on rat thymus. Drug Alcohol Depend. 1992; 30: 181–185, [PUBMED], [INFOTRIEVE], [CSA], [CROSSREF]
- Burg W. A., Shotwell O. L., Saltzman B. E. Measurements of airborne aflatoxins during the handling of contaminated corn. Am. Ind. Hyg. Assoc. J. 1981; 42: 1–11, [PUBMED], [INFOTRIEVE], [CSA]
- Cysewski J., Wood R. L., Pier A. C., Baetz A. L. Effects of aflatoxin on the development of acquired immunity to swine erysipelas. Am. J. Vet. Res. 1975; 39: 445–448, [CSA]
- Dugyala R. R., Kim Y. W., Sharma R. P. Effects of aflatoxin B1 and T-2 toxin on the granulocyte-macrophage progenitor cells in mouse bone marrow cultures. Immunopharmacology 1994; 27: 57–65, [PUBMED], [INFOTRIEVE], [CSA], [CROSSREF]
- Dugyala R. R., Sharma R. P. The effect of aflatoxin on cytokine mRNA and corresponding protein levels in peritoneal macrophages and splenic lymphocytes. Int. J. Immunopharmacol. 1996; 18: 599–608, [PUBMED], [INFOTRIEVE], [CSA], [CROSSREF]
- Ghoneimy W. A., Hassan H. A., Soliman S. A., Gergis S. M. Study on the effect of aflatoxicosis on the immune response of rabbit to Pasteurella multocida vaccine. Assiut. Vet. Med. J. 2000; 43: 287–303, [CSA]
- Ghosh R. C., Chauhan H. V., Roy S. Immunosuppression in broilers under experimental aflatoxicosis. Br. Vet. J. 1990; 146: 457–462, [PUBMED], [INFOTRIEVE], [CSA]
- Giambrone J. J., Ewert D. L., Wyatt R. D., Edison C. S. Effect of aflatoxin on the humoral and cell-mediated immune systems of the chicken. Am. J. Vet. Res. 1978; 39: 305–308, [PUBMED], [INFOTRIEVE], [CSA]
- Groopman J. D., Cain L. G., Kensler T. W. Aflatoxin exposure in human populations and relationship to cancer. CRC Crit. Rev. Toxicol. 1988; 19: 113–145, [CSA]
- Hatori Y., Sharma R. P., Warren R. P. Resistance of C57Bl/6 mice to immunosuppressive effects of aflatoxin B1 and relationship with neuroendocrine mechanisms. Immunopharmacology 1991; 22: 127–136, [PUBMED], [INFOTRIEVE], [CSA], [CROSSREF]
- Hayes R. B., van Nieuwenhuize J. P., Raatgever J. W., Ten Kate F. J. Aflatoxin exposures in the industrial setting: an epidemiological study of mortality. Food Chem. Toxicol. 1984; 22: 39–43, [PUBMED], [INFOTRIEVE], [CSA], [CROSSREF]
- Jakab G. J., Hmieleski R. R., Zarba A., Hemenway D. R., Groopman J. D. Respiratory aflatoxicosis: Suppression of pulmonary and systemic host defenses in rats and mice. Toxicol. Appl. Pharmacol. 1994; 125: 198–205, [PUBMED], [INFOTRIEVE], [CSA], [CROSSREF]
- Kadian S. K., Monga D. P., Goel M. C. Effect of aflatoxin B1 on the delayed type hypersensitivity and phagocytic activity of reticuloendothelial system in chickens. Mycopathologica 1988; 104: 33–36, [CSA], [CROSSREF]
- Louria D. B., Finkel G., Smith J. K., Buse M. Aflatoxin-induced tumors in mice. Sabouraudia 1974; 12: 371–375, [PUBMED], [INFOTRIEVE], [CSA]
- Michael G. Y., Thaxton P., Hamilton P. B. Impairment of the reticuloendothelial system of chickens during aflatoxicosis. Poultry Sci. 1973; 52: 1206–1207, [CSA]
- Miller D. M., Stuart B. P., Crowell W. A., Cole R. J., Goven A. J., Brown J. Aflatoxicosis in swine: Its effects on immunity and relationship to salmonellosis. Am. Assoc. Vet. Lat. Diagn. 1978; 21: 135–146, [CSA]
- Marin D. E., Taranu I., Bunaciu R. P., Pascale F., Tudor D. S., Avram N., Sarca M., Cureu I., Criste R. D., Suta V., Oswald I. P. Changes in performance, blood parameters, humoral and cellular immune responses in weanling piglets exposed to low doses of aflatoxin. J. Anim. Sci. 2002; 80: 1250–1257, [PUBMED], [INFOTRIEVE], [CSA]
- Mocchegiani E., Corradi A., Santarelli L., Tibaldi A., DeAngelis E., Porghetti P., Bonomi A., Fabris N., Cabassi E. Zinc thymic endocrine activity and mitogen responsiveness (PHA) in piglets exposed to maternal aflatoxicosis B1 and G1. Vet. Immunol. Immunopathol. 1998; 62: 245–260, [PUBMED], [INFOTRIEVE], [CSA], [CROSSREF]
- National Academy Press. Guide for the Care and Use of Laboratory Animals. Institute of Laboratory Animal Resources, National Resource Council. 1966
- National Toxicology Program. Aflatoxins. Rep. Carcinog. 2002; 10: 8–10, [CSA]
- Northrup S. W., McKenzie R., Thurston R., Hess R., Kilburn K. Aflatoxin effects on airway cells in rodents. Fed. Proc. 1975; 34: 839, [CSA]
- Padmanaba V. D. Role of aflatoxins as immunomodulators. J. Toxicol. Toxin Rev. 1989; 8: 239–245, [CSA]
- Panangala V. S., Giambrone J. J., Diener U. L., Davis N. D., Hoerr F. J., Mitra A., Schultz R. D., Wilt G. R. Effects of aflatoxin on the growth performance and immune responses of weanling swine. Am. J. Vet. Res. 1986; 47: 2062–2067, [PUBMED], [INFOTRIEVE], [CSA]
- Paul P. S., Johnson D. W., Mirocha C. J., Soper B. S., Thoen C. O., Muscoplat C. C., Weber A. F. In vitro stimulation of bovine peripheral blood lymphocytes: Suppression of phytomitogen and specific antigen lymphocyte responses by aflatoxin. Am. J. Vet. Res. 1977; 38: 2033–2035, [PUBMED], [INFOTRIEVE], [CSA]
- Pier A. C. Mycotoxins and animal health. Adv. Vet. Sci. Comp. Med. 1981; 25: 185–243, [PUBMED], [INFOTRIEVE], [CSA]
- Pier A. C., Heddleston K. L. Effects of aflatoxins on immunity in turkeys. I. Impairment of actively acquired resistance to bacterial challenge. Avian Dis. 1970; 14: 797–809, [PUBMED], [INFOTRIEVE], [CSA]
- Pier A. C., Heddleston K. L., Cysewski S. J., Patterson J. M. Effect of aflatoxin on immunity in turkeys. II. Reversal of impaired resistance to bacterial infection by passive transfer of plasma. Avian Dis. 1972; 16: 381–387, [PUBMED], [INFOTRIEVE], [CSA]
- Pier A. C., McLoughlin C. A. Mycotoxin suppression of immunity. Trichothecenes and Other Mycotoxins, J. Lacey. Wiley, ChichesterEngland 1985; 507–519
- Pier A. C., Richard J. L., Cysewski S. J. Implications of mycotoxins in animal disease. J. Am. Vet. Med. Assoc. 1980; 176: 719–724, [PUBMED], [INFOTRIEVE], [CSA]
- Pruett S. B., Collier S., Wu W. J., Fan R. Quantitative relationships between the suppression of selected immunological parameters and the area under the corticosterone concentration vs. time curve in B6C3F1 mice subjected to exogenous corticosterone or to restraint stress. Toxicol. Sci. 1999; 49: 272–280, [PUBMED], [INFOTRIEVE], [CSA], [CROSSREF]
- Pruett S. B., Fan R., Zheng Q., Myers L. P., Hebert P. Modeling and predicting immunological effects of chemical stressors: Characterization of a quantitative biomarker for immunological changes caused by atrazine and ethanol. Toxicol. Sci. 2003; 75: 343–354, [PUBMED], [INFOTRIEVE], [CSA], [CROSSREF]
- Raisuddin S., Singh K. P., Zaidi S. I., Ray P. K. Immunostimulating effects of Protein A in immunosuppressed aflatoxin-intoxicated rats. Int. J. Immunopharmacol. 1994; 16: 977–984, [PUBMED], [INFOTRIEVE], [CSA], [CROSSREF]
- Raisuddin S., Singh K. P., Zaidi S. I., Saxena A. K., Ray P. K. Effects of aflatoxin on lymphoid cells of weanling rat. J. Appl. Toxicol. 1990; 14: 245–250, [CSA]
- Reddy R. V., Taylor M. J., Sharma R. P. Studies of immune function of CD-1 mice exposed to aflatoxin B1. Toxicology 1987; 43: 123–132, [PUBMED], [INFOTRIEVE], [CSA], [CROSSREF]
- Richard J. L., Chevill N. F., Songer J. R., Thurston J. R. Exposure of rats to aflatoxin-containing particles. Proceedings of the VIIIth Congress of the International Society for Human and Animal Mycology, M. Baxter. Massey Univ. Press, Palmerston NorthNew Zealand 1982; 464–468
- Richard J. L., Pier A. C., Cysewski S., Graham C. Effect of aflatoxin and aspergillosis on turkey poults. Avian. Dis. 1973; 17: 111–121, [PUBMED], [INFOTRIEVE], [CSA]
- Richard J. L., Thurston J. R. Effect of aflatoxin on phagocytosis of Aspergillus fumigatus spores by rabbit alveolar macrophages. Appl. Microbiol. 1975; 30: 44–47, [PUBMED], [INFOTRIEVE], [CSA]
- Schlesinger R. B. Comparative deposition of inhaled aerosols in experimental animals and humans: A review. J. Toxicol. Environ. Health 1985; 15: 197–214, [PUBMED], [INFOTRIEVE], [CSA]
- Sharma R. P. Immunotoxicity of mycotoxins. J. Dairy Sci. 1993; 76: 892–897, [PUBMED], [INFOTRIEVE], [CSA]
- Silvotti L., Petterino C., Bonomi A., Cabassi E. Immunotoxicological effects on piglets of feeding sows diets containing aflatoxins. Vet. Rec. 1997; 141: 469–472, [PUBMED], [INFOTRIEVE], [CSA]
- Sorenson W. G., Jones W., Simpson J., Davidson J. I. Aflatoxin in respirable airborne peanut dust. J. Toxicol. Environ. Health 1984; 14: 525–533, [PUBMED], [INFOTRIEVE], [CSA]
- Sorenson W. G., Simpson J. P., Peach M. J., 3rd, Thedell T. D., Olenchock S. A. Aflatoxin in respirable corn dust particles. J. Toxicol. Environ. Health 1981; 7: 669–672, [PUBMED], [INFOTRIEVE], [CSA]
- Szabo G. Consequences of alcohol consumption on host defence. Alcohol Alcoholism 1999; 34: 830–841, [CSA], [CROSSREF]
- Thaxton J. P., Tung H. T., Hamilton P. B. Immunosuppression in chickens by aflatoxin. Poult. Sci. 1974; 53: 721–725, [PUBMED], [INFOTRIEVE], [CSA]
- Thurston J. R., Richard J. L., Cysewski S. J., Pier A. C., Graham C. K. Effect of aflatoxin on complement activity in guinea pigs. Proc. Soc. Exp. Biol. Med. 1972; 139: 300–307, [PUBMED], [INFOTRIEVE], [CSA]
- Thurston J. R., Deyoe B. L., Baetz A. L., Richard J. L., Booth G. D. Effect of aflatoxin on serum proteins, complement activity and the antibody response to Brucella abortus in guinea pigs. Am. J. Vet. Res. 1974; 35: 1097–1100, [PUBMED], [INFOTRIEVE], [CSA]
- van Heugten E., Spears J. W., Coffey M. T., Kegley E. B., Qureshi M. A. The effect of methionine and aflatoxin on immune function in weanling pigs. J. Anim. Sci. 1994; 72: 658–664, [PUBMED], [INFOTRIEVE], [CSA]
- Watzl B., Neudecker C., Hansch G. M., Rechkemmer G., Pool-Zobel B. L. Short-term moderate aflatoxin exposure has only minor effects on the gut-associated lymphoid tissue of Brown Norway rats. Toxicology 1999; 138: 93–102, [PUBMED], [INFOTRIEVE], [CSA], [CROSSREF]
- Zarba A., Hmieleski R., Hemenway D. R., Jakab G. J., Groopman J. D. Aflatoxin B1-DNA adduct formation in rat liver following exposure by aerosol inhalation. Carcinogenesis 1992; 13: 1031–1033, [PUBMED], [INFOTRIEVE], [CSA]
- Zilinskas R. A. Iraq's biological weapons. The past as future?. J. Amer. Med. Assoc. 1997; 278: 418–424