Abstract
Moringa oleifera Lam. is a small tree cultivated throughout India. We have investigated the effect of ethanolic extract of seeds of Moringa oleifera (MOEE, an herbal remedy) on the potential prevention of immune-mediated inflammatory responses in toluene diisocyanate (TDI as antigen)-induced asthma in Wistar rats. Rats were divided into five different groups (n = 8/group): Group-I = unsensitized control; Group-II = TDI control/vehicle; Group-III = dexamethasone (DXM) 2.5 mg/kg; and, Groups IV and V = 100 mg/kg and 200 mg/kg body weight [BW] of MOEE, respectively. All rats (except unsensitized controls) were sensitized by intranasal application of 10% TDI to induce airway hypersensitivity. Animals in Groups II–V were given their respective drug treatment per os from 1 wk prior to initiation of sensitization until the day of final provocation with 5% TDI. After this last challenge, all rats were examined for hyperreactivity symptoms and then sacrificed to determine their total and differential leucocytes in blood and bronchoalveolar (BAL) fluid and levels of TNF ∝, IL-4, and IL-6 in their BAL and serum. Homogenates of one lung lobe from each animal were utilized to assess oxidative stress; a separate lobe underwent histologic examination to assess airway inflammatory status. The results suggest that asthmatic symptoms were found in TDI control rats only, while both MOEE- and DXM-treated rats did not manifest any airway abnormality. In MOEE- and DXM-treated rats, neutrophil and eosinophil levels in the blood were decreased significantly; levels of total cells and each different cell in their BAL fluid were markedly decreased as compared to those in TDI controls. TNF α, IL-4, and IL-6 were predominant in serum as well as in BAL fluids in TDI controls, but these levels were reduced significantly by MOEE treatment. The antioxidant activity in relation to antiinflammatory activity of the extract and histopathological observations also reflected a protective effect. Based on the above findings and observations, it can be concluded that Moringa oleifera may possess some beneficial properties that act against chemically stimulated immune-mediated inflammatory responses that are characteristic of asthma in the rat.
Keywords :
INTRODUCTION
Asthma is characterized by reversible airflow obstruction, airway hyperresponsiveness and/or hyperreactivity, and chronic inflammation characterized by an influx and activation of inflammatory cells like eosinophils (Wardlaw et al., Citation1988), lymphocytes (Sergio, Citation2000) macrophages/monocytes (Bousquet et al., Citation2000), and neutrophils (Sur et al., Citation1993). Activation of these cells leads to release of inflammatory mediators including cytokines and generation of reactive oxygen species (ROS) (Kinnula et al., Citation1995). Many cytokines or their mRNA have been detected in increased quantity in bronchial biopsies or bronchoalveolar lavage fluid from asthmatic subjects (Broide et al., Citation1992). Specifically, tumor necrosis factor (TNF)-α (Coker and Laurent, Citation1998), interleukin (IL)-4 (Gharaee-Kermani et al., Citation2001), and IL-6 (Elias et al., Citation1997) have been detected in human airways (Robinson et al., Citation1992).
It has been shown that inflammation driven by increased oxidative stress occurs in the airways of patients with asthma, (reviewed by Dworski, Citation2000). In addition, it has been proposed that ROS can initiate inflammatory responses in the lungs (Guyton et al., Citation1996; Rahman and MacNee, Citation1998) and produced by phagocytes that have been recruited to sites of inflammation is a major cause of the cell and tissue damage associated with many chronic inflammatory lung diseases including asthma (Hatch, Citation1995; Rahman and MacNee, Citation1996, Citation1999, Citation2000; Dworski, Citation2000).
Toluene diisocyanate (TDI), a highly reactive industrial chemical, is one of the leading causes of occupation related asthma in industrialized countries. In modern times, well over 200 causative agents have now been associated with asthma in the workplace (Berstein et al., Citation1992). It is estimated that 2–15% chronically exposed workers exhibit symptoms of asthma (Becklake, Citation1993; Chan-Yeung and Malo, Citation1993). To date, no scientific study has been reported that has examined alternative therapies in connection with the development or persistence of TDI-induced asthma in rats. However, the use of complementary and alternative medicines for asthma treatment is of great interest in patient care (Lane and Lane, Citation1991; Graham and Blaiss, Citation2000). To this goal, we have selected this herbal plant to reveal its scientific exploration for the treatment of asthma.
Moringa oleifera Lam. belongs to the family Moringaceae (genus Moringa), and its best known and most widely distributed species (Sengupta and Gupta, Citation1970). It is small fast growing ornamental tree grown throughout the tropics and subtropics of Asia and Africa (Michael and Horst, Citation1998). Numerous varieties of M. oleifera have been developed to meet the tastes of local populations (Rajan, Citation1986). The medicinal values of the seeds and the different parts of the plant have long been recognized in folklore medicine (Faizi et al., Citation1995). The whole plant possesses antimicrobial activity (Caceres, Citation1991) and is also used for the treatment of ascites, rheumatism, venomous bites, and for enhancing cardiac function (Nadkarni and Nadkarni, Citation1976; Chaudhri, Citation1996).
The leaves exhibit strong hypotensive, diuretic, and spasmolytic effects, and have been seen to be useful against inflammation, in anaphylaxis (Mahajan et al., Citation2006) helminthesis, and scurvy (Caceres et al., Citation1992). The roots of the plant have been used as carminatives, anthelmintics, diuretics, and in the treatment of intermittent fever, epilepsy, and chronic rheumatism (Karadi et al., Citation2006). The plant's seeds have been used as purgatives, antipyretics, and as anti-inflammatory agents (Varier, Citation1997). We have previously reported on the antiarthritic activity of the ethanolic extract of these seeds (Mahajan et al., Citation2007) and Guevara et al. (Citation1999) noted the antigenotoxic activity of these extracts. Ayurvedic practitioners used to administer the seed extract nasally in diseases like rhinitis, and the dried seeds were used as an anti-allergic medication (Chopra et al., Citation1938). Treatment with the plant is also reported to elicit good clinical responses in children suffering from upper respiratory tract infections as well as skin infections. It has been reported that one of the alkaloids from the plant closely resembles ephedrine in action and has been seen to be useful in the treatment of asthma; alkaloid Moringine relaxes bronchioles (Kirtikar and Basu, Citation1975).
MATERIALS AND METHODS
Procurement of Plant Material and Extraction Procedure
Seed kernels of M. oleifera were obtained from a commercial supplier of Ahmedabad and were identified and authenticated by Department of Pharmacognosy, L. M. College of Pharmacy, Ahmedabad, India. Voucher specimens were deposited at the Department of Pharmacognosy, Ahmedabad.
The coarse powder (500 g) of the dried seed kernels was defatted using petroleum ether and then it was exhaustively extracted using 95% ethanol in a Soxhlet extractor at 55oC for 6 hr. The extract was concentrated under reduced pressure to yield a syrupy mass. The extract was stored in airtight container in cool place and used throughout the study.
Phytochemical Analysis
Preliminary phytochemical studies of the extract were performed according to published standard methods (Peach and Tracey, Citation1955). These tests (see ) were broad in scope and sought to determine the presence of alkaloids, flavanoids, saponins, glycosides, phenols, steroids, tannins and terpenoids in the extract. For these tests, a stock solution of 10 mg MOEE/ml was prepared in distilled water. For each specific test, the appearance of any turbidity, opacity, or precipitation—indicative of the presence of the specific class of agent being tested for within each given test was then made by direct observation (not spectrophotometrically).
TABLE 1 Preliminary phytochemical study on M.oleifera seed extract
Drugs and Chemicals
All the required organic solvents were obtained from the S.D. Fine Chemicals Private Limited (Mumbai, India) and all were of analytical grade. 2,4-Toluene diisocyanate, bovine serum albumin (A-9418), Field's stain, hydrogen peroxide, trichloroacetic acid (TCA), thiobarbituric acid (TBA), Tris buffer, 5,5′-dithiobis-2-nitrobenzoic acid (DTNB), epinephrine, dexamethasone were all obtained from Sigma-Aldrich (St. Louis, MO). ELISA kits were purchased from Biosource International (Camarillo, CA).
Experimental Animals and Feeds
Wistar albino rats procured from Zydus Cadila Limited (Ahmedabad, India) were housed at ambient temperature (22 ± 1°C), relative humidity (55 ± 5%) and 12 hr light-dark cycle. Animals had free access to standard pellet diet (certified Amrut brand rodent feed, Pranav Agro Industries, Pune, India) and filtered tap water given ad libitum. Freshly prepared solutions of drugs or chemicals were used throughout the study. All the experiments complied with the University guidelines for animal experimentation. Throughout the entire study period, the rats were monitored for growth, health status and food intake capacity to be certain that they were healthy.
Antigen for Sensitization
The antigen used for sensitization was 2,4-toluene diisocyanate (TDI). TDI was prepared in ethyl acetate to a concentration of 10% for sensitization and to 5% for provocation.
Sensitization and Provocation of Animals
Adult Wistar rats, weighing 200–250 g were divided into 5 different groups of 8 animals each. Group-I: control (distilled water); Group-II: TDI control (distilled water); Group-III: dexamethasone (DXM; 2.5 mg/kg body weight [BW] per os, as reference standard); Group-IV: MO1 (100 mg/kg per os, of ethanolic extract of M. oleifera); and, Group-V: MO2 (200 mg/kg per os, of ethanolic extract of M. oleifera).
All the rats (except Group-I) were sensitized by the slight modified method of Zheng et al. (Citation2001b) by dropping 5 μ l of 10% TDI into each nostril for seven consecutive days under light ether anaesthesia. After a week of rest, all the rats (except Group-I) were re-sensitized for 7 d. One week after the second course of sensitization the rats were provoked by intranasal administration of 5 μ l of 5% TDI. All the animals of Groups II, III, IV, and V were given the respective drug treatment by oral route from 1 week prior to the initiation of sensitization until the day of final challenge with 5% TDI. The treatment was daily and continued during resting period also. The last dose of drug treatment was given 2 hr prior to provocation with 5% toluene diisocyanate.
The rats of Group-I (vehicle treated) were treated with intranasal ethyl acetate and provoked with 5% TDI in the same protocol. After provocation, all animals were observed for outward observable symptoms of airway hyperreactivity for a period of 1 hr. Specifically, it was noted that all animals in the TDI-control group coughed severely and exhibited labored breathing, had their mouths open, and were gasping for air. Prolongation of the expiratory phase was also observed. These rats also demonstrated irritability, aggression, and sneezing. None of these symptoms were found in animals from any of the other treatment groups.
The daily doses of MOEE used herein were selected based upon findings in a previous study from our laboratory (Mahajan et al., Citation2007). Specifically, in those earlier studies that screened for MOEE-induced prevention of induction of arthritic activity in rats, promising dose dependent activity was obtained at both the 100 and 200 mg/kg levels (i.e., the highest dose had little more effect than the 200 mg/kg dose). Similar findings were obtained in preliminary studies here with the TDI-treated rat model. One additional reason for selecting these doses of extract was that we have observed protection against histamine- and acetylcholine-induced broncho-spasm in guinea pigs using the same dose range (data not shown).
Blood Cell Count and Serum Preparation
After the airway symptoms have been observed, rats were administered an intraperitoneally-delivered overdose of pentobarbital (120 mg/kg), blood was removed from the cervical vein. Each sample was then divided into two portions. The first aliquot was placed in a non-heparinized tube for serum separation; the isolated serum was stored and −80°C for later quantitative determinations of levels of cytokines TNFα as well as IL-4 and IL-6. The second portion of the blood was placed in a heparinized tube and used for total and differential leukocyte counts. Total leukocytes were counted after the blood was diluted at 1:10 with 1% acetic acid to lyse red blood cells. Detailed procedure for total and differential leukocyte counts was same as mentioned in BAL fluid (see next).
Bronchoalveolar Lavage (BAL) Collection and Differential Cell Count Analyses
The lungs of each rat were lavaged 5 times with 2 ml aliquots of warm (37°C) 0.9% saline via a tracheal cannula. The recovered lavage fluid was centrifuged at 200 × g for 10 min at 20°C; the supernatant was collected and stored at −80°C until used in cytokine determinations. The cell pellets were washed in 250 μ l 0.9% saline containing 10% bovine serum albumin. Total cell counts were then performed on automated cell counter (Cell Dyne 3500, Abbott, New York, NY). For differential counts, slides were prepared and then stained with Field's stain; after drying, 300 cells/slide were counted on a compound microscope (Optima X5Z-H) oil immersion power at 400 × magnification. To perform the differentials, cells were identified as eosinophils, lymphocytes, macrophages, or neutrophils using standard morphologic determinants.
Measurement of Inflammatory Mediators in Serum and BAL Fluid
The levels of TNFα, IL-4, and IL-6 in recovered serum and BAL samples were measured using enzyme-linked immunosorbent assay kits. After following the manufacturer's instructions, all plates were analyzed on an automated plate reader (Lab System Multiscan Model-51118220, Thermo Bioanalysis Co., Helsinki, Finland). The detection limit of the kits for TNFα, IL-4, and IL-6 were < 4 pg/ml, < 2 pg/ml and < 8 pg/ml, respectively.
Assessment of Pro-Oxidant and Antioxidant Status
All the animals were sacrificed by cervical dislocation and their lungs were isolated. Each lung was homogenized in Tris HCl buffer (0.01 M, pH. 7.4) using a REMI homogeniser (REMI Motor, Bombay, India) to generate a 10% homogenate (Hogberg et al., Citation1974). The method of Devasagayam (Citation1986) was used to measure the presence of lipid peroxides in the homogenate and is based upon the release of malondialdehyde. For the estimation of antioxidant enzyme activities in the samples, superoxide dismutase (SOD) was assayed according to the method of Misra and Fridovich (Citation1972), with the degree of inhibition of the auto-oxidation of epinephrine by SOD being used as the index of activity. Catalase activity was assayed by the method of Sinha (Citation1972). The method of Moron et al. (Citation1979) was used for estimation of total reduced glutathione (GSH). Protein was estimated according to the method of Lowry et al. (Citation1951). All the parameters were estimated spectrophotometrically (UV 1601, Shimadzu (Asia Pacific) Pte Ltd., Sydney, Australia) at their respective specified wavelengths.
Histopathology
Histopathologic analyses of lung tissues obtained from rats were also carried out to study the effects of the extract on the immune-mediated responses elicited by TDI. Dissected lungs were washed with normal saline and then placed in Bouin's fixative for 18–24 hr. The tissues were then washed twice with distilled water and placed in 70% alcohol. A pinch of lithium carbonate was added to remove excessive Bouin's stain. The tissues were then sequentially washed in 70% and 90% alcohol, and then placed in 90% alcohol overnight. Finally, the tissues were transferred into 100% alcohol for 3 hr and then placed in xylene until they become transparent. These tissues were then embedded in melted paraffin and sectioning performed. Several sections (3 μ m thickness) were taken from each tissue and sections with uniform shape and size were selected for histology. These sections were fixed on clear glass slides and stained using Heamotoxyllin and Eosin (H & E).
Statistical Analyses
Results were reported as mean ± SEM. Statistical analysis was performed using one-way analysis of variance (ANOVA). If the overall F-value was found statistically significant (p < 0.05), further comparisons among groups were made according to post-hoc Tukey's test. All statistical analyses were performed using Graph Pad software (San Diego, CA). Though data was considered statistical significant at p < 0.05, when data was found to be very (i.e., p < 0.01) or highly (i.e., p < 0.001) significant, this is indicated in the Results.
RESULTS
Phytochemical Study
The preliminary phytochemical screening of the ethanolic extract of M. oleifera showed the presence of alkaloids, flavanoids, glycosides, tannins and terpenoids ( and ).
TABLE 2 List of major phytochemical constituents of the seeds of M. oleifera
Effect of MOEE on Airway Hyperreactivity Symptoms
Within 2–10 min after provocation with 5% TDI, all the rats in the TDI-control group exhibited irritability, sneezing, and hyperrhinorrhea. Exertional breathing that was similar to that seen during asthma was observed to last 8–10 minutes in TDI-control rats. The control, DEXA-, and MOEE-treated rats did not show any signs of airway abnormality.
Effect of MOEE on Circulating Leukocyte Count
The number of total leucocytes, monocytes, and lymphocytes did not significantly differ among all of the different treatment groups. In contrast, the numbers of circulating eosinophils and neutrophils in the blood were significantly decreased in MOEE- and DEXA-treated rats as compared to those levels in TDI- control rats ().
TABLE 3 Total cells and differential leukocyte counts in blood (×103 cells/ml)Footnotea
Effect of MOEE on BAL Cellular Content and Differentials
The total cells and each differential leukocyte count in BAL fluid in TDI-sensitized rats were significantly elevated many fold compared to those in unsensitized rats. However, treatment with DEXA or MOEE induced significant reductions in these counts ().
TABLE 4 Total cells and differential leukocyte count in BAL fluid (×105 cells/ml)
Effect of MOEE on Select Cytokine Levels in Serum
TNFα, IL-4, and IL-6 concentrations in the serum of TDI control rats were all highly significantly increased compared to those in unsensitized controls. Treatment with DEXA and MO2 caused significant reductions in the levels of IL-4 and IL-6. In contrast, these treatments had no effect on TNFα levels. In addition, there were no effects whatsoever from the MO1 treatment on any of the serum cytokine levels (, , and ).
FIG. 1 Serum TNFα levels in rats. *Value significantly different from non-arthritic control (p < 0.001); value different from TDI-controls ($ p < 0.05). Values shown are the mean ± SEM from non-sensitized controls, sensitized controls (DC), and treatment regimen rats (DEXA = dexamethasone; MO1 = MOEE 100 mg/kg; MO2 = MOEE 200 mg/kg; n = 8 rats/group).
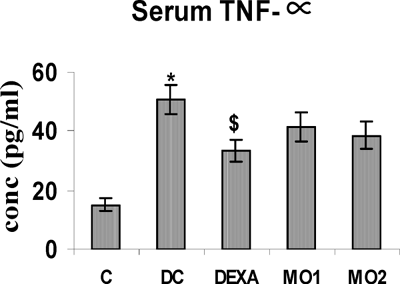
FIG. 2 Serum IL-4 levels in rats. *Value is significantly different from nonsensitized control (p < 0.001); value significantly different from TDI-controls (@ p < 0.001, # p < .01). Values shown are the mean ± SEM from non-sensitized controls, sensitized controls (DC), and treatment regimen rats (DEXA = dexamethasone; MO1 = MOEE 100 mg/kg; MO2 = MOEE 200 mg/kg; n = 8 rats/group).
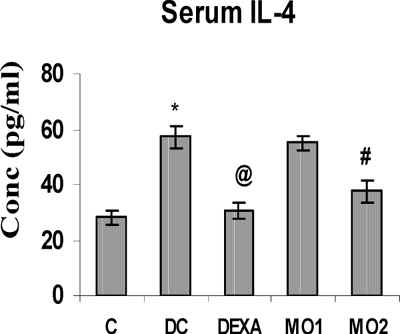
FIG. 3 Serum IL-6 level in rats. *Value is significantly different from non-sensitized control (p < 0.001); value significantly different from TDI-controls (# p < 0.01, $ p < 0.05). Values shown are the mean ±SEM from non-sensitized controls, sensitized controls (DC), and treatment regimen rats (DEXA = dexamethasone; MO1 = MOEE 100 mg/kg; MO2 = MOEE 200 mg/kg; n = 8 rats/group).
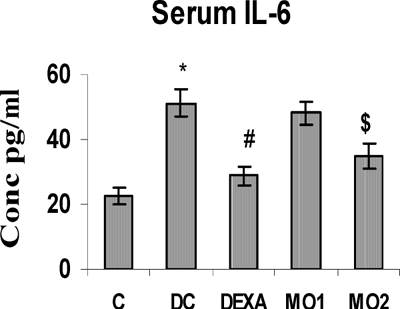
Effect of MOEE on Select Cytokine Levels in BAL Fluid
The concentrations of BAL TNFα, IL-4, and IL-6 in TDI-sensitized rats were highly significantly greater than those in unsensitized controls. The MO2 treatment regimen significantly reduced these levels to ones comparable to that obtained with DEXA. Interestingly, there was no effect from the MO1 regimen on TNFα and IL-4 levels (, , and ), although this protocol did cause reductions in IL-6 levels compared to the TDI-sensitized controls.
FIG. 4 BAL fluid TNFα levels in rats. *Value is significantly different from nonsensitized control (p < 0.001); value significantly different from TDI-controls (# p < 0.01, $ p < 0.05). Values shown are the mean ± SEM from non-sensitized controls, sensitized controls (DC), and treatment regimen rats (DEXA = dexamethasone; MO1 = MOEE 100 mg/kg; MO2 = MOEE 200 mg/kg; n = 8 rats/group).
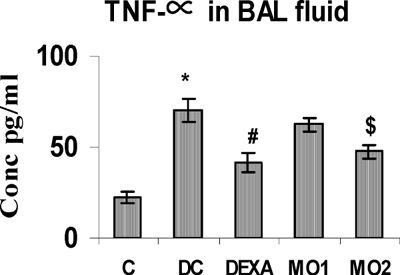
FIG. 5 BAL fluid IL-4 levels in rats. *Value is significantly different from control (p < 0.001); value significantly different from TDI-controls (@ p < 0.001). Values shown are the mean ± SEM from non-sensitized controls, sensitized controls (DC), and treatment regimen rats (DEXA = dexamethasone; MO1 = MOEE 100 mg/kg; MO2 = MOEE 200 mg/kg; n = 8 rats/group).
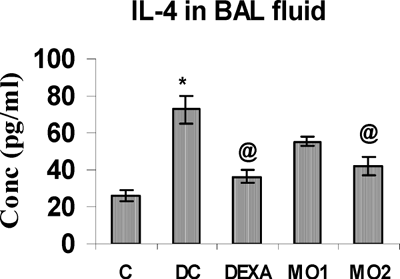
FIG. 6 BAL fluid IL-6 levels in rats. *Value is significantly different from control (p < 0.001); value significantly different from TDI-controls (@ p < 0.001, # p < 0.01, $ p < 0.05). Values shown are the mean ± SEM from non-sensitized controls, sensitized controls (DC), and treatment regimen rats (DEXA = dexamethasone; MO1 = MOEE 100 mg/kg; MO2 = MOEE 200 mg/kg; n = 8 rats/group).
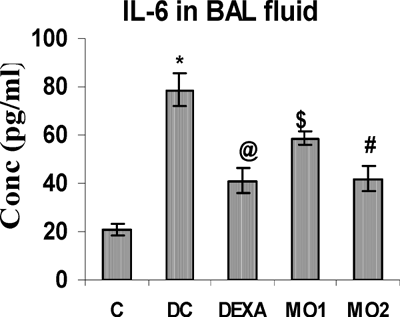
Effect of MOEE on Lipid Peroxidation as Reflected by Malondialdehyde (MDA) Levels
Tissue MDA levels were found to be significantly increased in the lungs of TDI-sensitized rats as compared to those in the lungs of normal control rats. Treatment with the higher dosage of MOEE produced very significant decreases in lung MDA levels. Furthermore, these reductions led to MDA levels comparable to those obtained when rats received the DEXA treatment ().
TABLE 5 Effect of MOEE on oxidative stress in TDI sensitized rats
Effect of MOEE on Antioxidant Enzymes (Levels of SOD, CAT and GSH)
Activities of superoxide dismutase and catalase, and the levels of reduced glutathione were all highly significantly decreased in TDI-sensitized animals as compared to those values seen in unsensitized controls. The treatment with MOEE increased the levels of each endpoint in a highly significant fashion as compared to sensitized rats that received only vehicle. In fact, the higher dosage treatment brought about values of SOD and GSH to nearly those seen in the unsensitized controls (). In general, among these three endpoints, effects on catalase appeared the most resistant to large changes due to the MOEE regimens.
Histolopathology Results
In TDI-control rats, a prominent infiltration of eosinophils, total leukocyte infiltration and reduced lumen size was observed in their lung tissues. Each treatment regimen showed a protective effect as evidenced by the presence of much less or only mild pathological changes ().
FIG. 7 Histopathological effects from the various treatments. Lung tissue sections of treated and untreated TDI sensitized animals stained with hematoxylin-eosin. (A) Shows normal view of lung pathology. (B) Shows typical damaged lung tissue from TDI-control group with total and differential leukocyte infiltration (eosinophils), reduced lumen size. (C) Shows less leukocyte infiltration lumen size by dexamethasone treatment. (D) MO1 shows less leukocyte infiltration but does not protected animal from other pathological features. (E) MO2 shows significant protection against leukocyte infiltration, and constriction of lumen size.
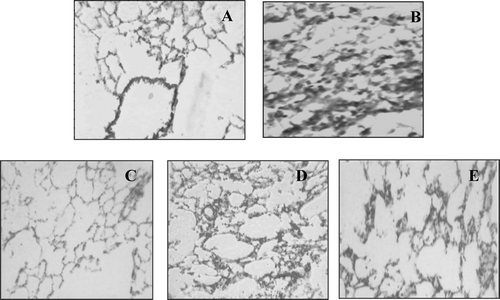
DISCUSSION
The objective of this study was to evaluate the effect of ethanolic extract of seeds of M. oleifera on TDI-induced immune-mediated airway inflammation in Wistar rats. To evaluate this, an established animal model was used in which rats were sensitized and provoked with TDI. This treatment with TDI induces the onset of asthma-like symptoms, e.g., airway inflammation, differential cell infiltration into local tissues of lungs and airways, eosinophilia, and release of chemical mediators like tumor necrosis factor (TNF)-α and interleukins (IL)-4 and -6.
Ayurveda has recommended a number of drugs from indigenous plants sources for the treatment of bronchial asthma and other allergic disorders and has been successful in controlling the disease as well (Zimmet and Tashkin, Citation2000). Large numbers of medicinal plant preparations have been reported to possess anti-asthmatic effects; these include Albizzia lebbeck (Tripathi and Das, Citation1977), Tinospora cordifolia (Nayampalli et al., Citation1986), Solanum xanthocarpum (Chitravanshi et al., Citation1990), Vitex negundo (Nair and Saraf, Citation1995), Tephrosia purpurea (Gokhale et al., Citation2000), and Ganoderma tsugae (Lin et al., Citation2006). The majority of herbal products found to be useful in the management of asthma in the experimental studies have yet to undergo clinical trials. In the light of above facts, in-depth studies are required to establish and make the best use of plant resources for the treatment of bronchial asthma. In this connection, we have selected the plant Moringa oleifera to reveal its potential usefulness in the prevention/amelioration of symptoms of asthma. This report here is the first to examine the effects of this herbal plant in TDI-induced immune-mediated responses in rats.
Chronic exposure to toluene diisocyanate (TDI), a low molecular weight compound widely used as an industrial chemical, has been associated with asthma (Berstein, Citation1982). Although the pathogenesis of diisocyanate-induced asthma is still largely unknown (Baur et al., Citation1994; Bolognesi et al., Citation2001), increasing evidence from human and laboratory animal models has indicated that the OA induced by TDI shares several features with allergic asthma (Wisnewski and Redlich, Citation2001). Indeed intranasal instillation of TDI resulted in systemic TH2-dominated immune responses characterized by lower TH1 cytokines (IFNγ) associated with a rise in TH2 cytokines (IL-4, IL-5, IL-6, and TNFα). It also causes an allergic inflammation throughout the conducting airways characterized by a proliferation of goblet cells and an influx of eosinophils (Ban et al., Citation2006).
In the present study, the airway inflammation observed was in agreement with respiratory hyperreactivity symptoms that were similar to asthma noted by Abe et al. (Citation1993). Moreover, a variety of inflammatory mediators, released through the activation of many inflammatory and structural cells, result in the typical pathophysiologic changes of asthma (Barnes, Citation1996; Barnes et al., Citation1998). Activated eosinophils can release a variety of cationic including major basic protein (MBP), eosinophil peroxidase (EPO), eosinophil cationic protein (ECP) and eosinophil derived neurotoxin (EDN) there is evidence from a number of studies that these proteins can damage the respiratory epithelium (Frigas et al., Citation1980; Motojima et al., Citation1989).
Recent evidence has indicated a possible correlation between activation status of eosinophils and immune mediated responses (Filipovic and Cekic, Citation2001). Seltzer and colleagues reported that nonasthmatic subjects who had been exposed acutely to ozone-developed airway hyperresponsiveness in association with airway neutrophilia (Seltzer et al., Citation1986; Wenzel et al., Citation1997). Other clinical studies have since found that, measures of chronic asthma severity, such as FEV1, correlate with the degree of neutrophilia in sputum or bronchial biopsy specimens (Jatakanon et al., Citation1999; Louis et al., Citation2000). Neutrophilic inflammation in the airway is also increasingly recognized in acute exacerbations of asthma and in status asthmaticus (Sur et al., Citation1993; Ying et al., Citation1997; Ordonez et al., Citation2000).
In our study we have found increase in levels of these cells in blood of TDI-control rats, which were found to be decreased significantly by drug treatment. On the other hand, Kouadio et al. (Citation2005) found that eosinophils and neutrophils were increased 17.2 and 4.6 times, respectively, in the BAL fluids of rats exposed to 0.38 ppm TDI. In our study, animals in the TDI-control group showed significant increases in the number of total and differential leukocyte counts. This finding is consistent with data reported by other researchers who previously described the induction of asthma by TDI in the guinea pig model (Tanaka et al., Citation1988). The findings of these current studies indicate that MOEE alleviated bronchoalveolar inflammation by inducing decreases in the infiltration of inflammatory cells and in the secretion of inflammatory mediators into the airways.
With regard to the role of cytokines in the observed TDI responses, two recent animal studies have demonstrated that TDI exposure leads to the release of TH2 cytokines in the lungs. Plitnick et al. (Citation2005), using molecular immunology, compared cytokine mRNA responses in the auricular lymph nodes of BALB/c mice following treatment with isocyanates. Kouadio et al. (Citation2005) exposed Wistar rats to TDI vapor (4 hr/d for 5 consecutive days) and then quantified IL-2, -4, and -6 levels in their BAL fluid.
In these two studies, a significant increase in IL-4 levels was noted in the airways of the TDI-exposed hosts as compared to controls. The findings of these studies also indicated that a short period of repeated exposure to TDI caused respiratory hypersensitivity in which airway inflammatory and immunological events, represented in part by eosinophil infiltration and increases in the levels of select TH2 cytokines, may have played an important role. These latter findings build upon those from a study by Ban et al. (Citation1997) in which it was noted that the levels of TNFα and IL-6 were increased in the BAL fluid of pigs after exposure to TDI.
TNFα may have an important amplifying effect in immune-mediated inflammation (Kips et al., Citation1992). There is evidence for increased TNFα expression in asthmatic airways (Bradding et al., Citation1994), and IgE triggering in sensitized lungs leads to increased TNFα expression in epithelial cells in both the rat and human lung (Ohno et al., Citation1990; Ohkawara et al., Citation1992). TNFα is also present in bronchoalveolar lavage fluid obtained from asthmatic patients (Broide et al., Citation1992). Infusion of TNFα causes increased airway responsiveness in Brown-Norway rats (Kips et al., Citation1992). Our results indicated that while TNFα was predominantly secreted into the serum as well as the BAL fluid of TDI-control rats, MOEE supplementation inhibited its secretion into the lungs only.
IL-4 was reported to be produced by CD41 and CD81 T-lymphocytes, eosinophils, and mast cells in both atopic and nonatopic asthma (Bradding et al., Citation1994; Ying et al., Citation1997). IL-4 is well known to be required for the priming of TH2 cells; it promotes the production of IgE antibodies that play a key role in the elicitation of immediate hypersensitivity reactions associated with respiratory allergy. IL-4 receptor blockade prevents the development of antigen-induced airway hyperreactivity, goblet cell metaplasia, and pulmonary eosinophilia in a mouse model (Gavett et al., Citation1995). While the significant increase of IL-4 production in the serum and airways of TDI-exposed rats in this study is consistent with the findings of previous studies on TDI-induced occupational asthma (Dearman et al., Citation1996; Maestrelli et al., Citation1997), our findings indicate that treatment with the MOEE resulted in significant decrease in this expected production of IL-4.
IL-6 has been reported to have activities on a wide range of cellular processes, including T-lymphocyte activation and immunoglobulin production by B-lymphocytes; as such, it can also enhance IL-4-dependent IgE synthesis. There is evidence for increased release of IL-6 from alveolar macrophages from asthmatic patients after allergen challenge and increased basal release, compared with nonasthmatic subjects (Gosset et al., Citation1991). IgE-dependent triggering stimulates the secretion of IL-6 from both blood monocytes and alveolar macrophages in vitro (Broide et al., Citation1991). Increased levels of IL-6 were also detected in the nasal washings from children after rhinovirus infection (Zhu et al., Citation1996). In TDI-exposed rats, the level of IL-6 was found to be increased in the BAL itself and in isolated cells cultured in vitro (Zheng et al., Citation2001a). In the current studies, the increases in expected IL-6 levels were ameliorated significantly by treatments with the MOEE extract.
Oxidative stress plays an important role in the pathogenesis of airway diseases, particularly when inflammation is prominent (Paredi et al., Citation2002). Inflammatory and immune cells in the airways, such as eosinophils, monocytes/macrophages, neutrophils, and release increased amounts of reactive oxygen species (ROS) in asthmatic patients (Sedgwick et al., Citation1990; Kanazawa et al., Citation1991). It is reported that the generation of ROS in the lungs is enhanced after exposure to exogenous chemical agents including TDI (Janssen et al., Citation1993). In our study, an increased level of tissue malondialdehyde (MDA) and decreased activities of both select antioxidant enzymes and in levels of reduced glutathione (GSH) were observed in TDI-sensitized rats. This increased degree of lipid peroxidation is expected, and is known to, correlates with changes in the degree of airway obstruction (Montuschi et al., Citation2000).
Decreased levels of superoxide dismutase (SOD; De Raeve et al., Citation1997; Tekin et al., Citation2000; Shanmugasunaram et al., Citation2001), catalase (Navak et al., Citation1991) and GSH (Li et al., Citation1994) in the lungs of asthmatics have been reported. Studies by Varshavski et al. (Citation2003) had revealed suppressed activity of SOD and catalase, as well as reductions in the levels of reduced glutathione in patients with bronchial asthma. Both SOD and catalase play important roles in the detoxification of superoxide anion and H2O2, respectively, thereby protecting cells against free radical-induced damage (Wood et al., Citation2003). Reduced glutathione (GSH), in conjunction with glutathione peroxidase (GPx) and glutathione-S-transferase (GST) play a central role in the defense against free radicals, peroxides, and a wide range of xenobiotics (Rahman et al., Citation2006).
Furthermore, increases in dietary antioxidant status particularly that of α -tocopherol, correlated with lower levels of lipid peroxidation and improved lung function (Britton et al., Citation1995). A beneficial link between polyphenol intake and lower disease risk is likely, with many of the clinical benefits being attributed to both their antioxidant and anti-inflammatory properties (Arts and Hollman, Citation2005). MOEE have been purported to be a rich source of antioxidants, including flavanoids and polyphenolic compounds (Sanchez et al., Citation2006) that may inhibit inflammatory cytokine release. The results obtained here were consistent with the work of Culpitt et al. (Citation2003). Specifically, treatment with the higher dosage of MOEE significantly reduced MDA levels, and significantly improved antioxidant enzymes levels as compared to those levels seen in TDI- control rats, and more toward those seen with the unsensitized controls.
The results of this study were consistent with the work of several other investigators in determining the effectiveness of select natural products against inflammation-related pathologies in the lungs. For example, Singh et al. (Citation2000) demonstrated that Elaeocarpus sphaericus fruits possessed both mast-cell stabilizing activity and an efficacy against bronchial asthma. Lin et al. (Citation2006) have shown that Ganoderma tsugae alleviated bronchoalveolar inflammation in an airway sensitization and challenge mouse model. Ishimitsu et al. (Citation2001) reported effects of a traditional Japanese medicine, Bu-zhong-yi-qi-tang, against allergic asthma in mice. Benzylisothiocyanate has been noted for its antiinflammatory activity (Das et al., Citation1958). Our findings suggest that MOEE (specifically at a dose of 200 mg/kg BW) suppresses airway hyperreactivity symptoms. It also alleviates bronchoalveolar inflammation by decreasing the infiltration of both total and differential inflammatory cells (particularly eosinophils and neutrophils) and the release of inflammatory mediators into the local tissues of lungs and airways.
It is important to also note that, in the present study, a crude ethanolic extract of seeds of Moringa oleifera, and not any one purified compound, was used. Qualitative phytochemical investigation of MOEE here and by other investigators has shown the presence of various active components (see and ). We have also succeeded in isolating benzylisothiocynate from this extract. The active principle(s) listed in the tables might be responsible for one or more mechanisms for the anti-inflammatory activities observed here. Clearly, further investigations are required (and ongoing) to verify this and to further characterize the composition of the extract by activity-guided fractionation. These results, using an antecedent approach, provide evidence that the consumption of certain common plants may have the potential to inhibit the chemically stimulated release of cytokines and justify the rationale behind the use of Moringa oleifera in the treatment of respiratory tract infection, rhinitis, and bronchial relaxation.
REFERENCES
- Abe Y., Ogino S., Irifune M., Imamura I., Liu Y. Q., Fukui H., Matsunaga T. Histamine content, synthesis and degradation in nasal mucosa and lung of guinea pigs treated with toluene diisocyanate (TDI). Clin. Exp. Allergy 1993; 23: 512–517
- Arts I. C., Hollman P. C. Polyphenols and disease risk in epidemiologic studies. Am. J. Clin Nutr. 2005; 81: 317S–325S
- Ban M., Morel G., Langonne I., Huguet N., Pepsin E., Binet S. TDI can induce repiratory allergy with TH2-dominated response in mice. Toxicology 2006; 218: 39–47
- Ban M., Hettich D., Goutet M., Bonnet P. TDI inhalation in guinea-pigs involves migration of dendritic cells. Toxicol. Lett. 1997; 93: 185–194
- Barnes P. J. Pathophysiology of asthma. Br. J. Clin. Pharmacol. 1996; 42: 3–10
- Barnes P. J., Chung K. F., Page C. P. Inflammatory mediators of asthma. Pharmacol. Rev. 1998; 50: 515–596
- Baur X., Mareck W., Ammon J., Czuppon A. B., Marczynski B., Raulf-Heimsoth M., Roemmelt H., Fruhman G. Respiratory and other hazards of isocyanates. Int. Arch. Occup. Environ. Health. 1994; 66: 141–152
- Becklake M. R. Prevalence and determinants. Asthma in the workplace, 1st Edition, I. L. Bernstein, M. Chan-Yeung, J. L. Malo, D. I. Bernstein. Marcel Dekker, New York 1993; 29–59
- Bernstein J. A. Occupational asthma. “My job is making me sick”. Postgrad Med. 1992; 92: 109–118
- Bernstein L. Isocyanate-induced pulmonary diseases: A current perspective. J. Allergy Clin. Immunol. 1982; 70: 24–35
- Bolognesi C., Baur X., Marczynski B., Norppa H., Sepai O., Sabbioni G. Carcinogenic risk of toluene diisocyanate and 4,4′-methylenediphenyl diisocyanate: Epidemiological and experimental evedence. CRC Crit. Rev. Toxicol. 2001; 131: 737–772
- Bousquet J., Jeffery P. K., Busse W. W., Johnson M., Vignola A. M. Asthma: From bronchoconstriction to airways inflammation and remodeling. Am. J. Respir. Crit. Care. Med. 2000; 161: 1720–1745
- Bradding P., Roberts J. A., Britten K. M., Montefort S., Djukanovic R., Mueller R., Heusser C. H., Howarth P. H., Holgate S. T. Interleukins-4, -5 and -6, and tumor necrosis factor-α in normal and asthmatic airways: Evidence for the human mast cell as a source of these cytokines. Am. J. Respir. Cell Mol. Biol. 1994; 10: 471–480
- Britton J. R., Pavord I. D., Richards K. A., Knox A. J., Wisniewski A. F., Lewis S. A. Dietary antioxidant vitamin intake and lung function in the general population. Am. J. Respir. Crit. Care Med. 1995; 151: 1383–1387
- Broide D. H., Firestein G. S. Endobronchial allergen challenge: Demonstration of cellular source of granulocyte macrophage colony-stimulating factor by in situ hybridization. J. Clin. Invest. 1991; 88: 1048–1053
- Broide D. H., Lotz M., Cuomo A. J., Coburn D. A., Federman E. C., Wasserman S. I. Cytokines in symptomatic asthma airways. J. Allergy Clin. Immunol. 1992; 89: 958–967
- Caceres A., Cebreva O., Morales O., Miollined P., Mendia P. Pharmacological properties of M. oleifera 1. Preliminary screening for antimicrobial activity. J. Ethnopharmacol. 1991; 33: 213–216
- Caceres A., Saravia A., Rizzo S., Zabala L., De-Leon E., Nave F. Pharmacological properties of M. oleifera 2. Screening for antispasmodic, anti-inflammatory and diuretic activity. J. Ethnopharmacol. 1992; 36: 233–237
- Chan-Yeung M., Malo J. L. Natural history of occupational asthma. Asthma in the Workplace, 1st Edition, I. L. Bernstein, M. Chan-Yeung, J. L. Malo, D. I. Bernstein. Marcel Dekker, New York 1993; 299–322
- Chaudhri R. D. Herbal Drug Industry: A Practical Approach to Industrial Pharmacognosy, R. D. Chaudhri. Eastern Publisher, New Delhi 1996; 58–62
- Chitravanshi V. C., Gupta P. P., Kulshrestha D. K., Kar K., Dhawan B. N. Anti-allergic activity of Solanum xanthocarpum. Ind. J. Pharmacol. 1990; 22: 23–24
- Chopra's Indigenous Drugs of India, R. N. Chopra, I. C. Chopra, K. Handa, D. Kapur. U.N. Dhur & Sons, Calcutta 1938
- Coker R. K., Laurent G. J. Pulmonary fibrosis: Cytokines in the balance. Eur. Respir. J. 1998; 11: 1218–1221
- Culpitt S. V., Rogers D. F., Fenwick P. S., Shah P., De Matos C., Russell R. E. Inhibition by red wine extract, resverotrol, of cytokine release by alveolar macrophages in COPD. Thorax 2003; 58: 942–946
- Dahot M. U., Memon A. R. Nutritive significance of oil extracted from Moringa oleifera seeds. J. Pharm. Univ. Karachi 1985; 3: 75–80
- Das B. R., Kurup P. A., Narasimha Rao P. L. Antibiotic principle from Moringa Pterosperma Part IX. Inhibition of transaminase by isocyanates. Ind. J. Med. Res. 1958; 46: 75–77
- Dayrit F. M., Alcantara A. D., Villasenor I. M. The antibiotic compound and its deactivation in aqueous solution. Phil. J. Sci. 1990; 119: 23–26
- Dearman R. J., Basketter D. A., Kimber I. Characterization of chemical allergens as a function of divergent cytokine secretion profiles induced in mice. Toxicol. Appl. Pharmacol. 1996; 138: 308–316
- De Raeve H. R., Thunnissen F. B., Kaneko F. T., Guo F. H., Lewis M., Kavuru M. S., Secic M., Thomassen M. J., Erzurum S. C. Decreased Cu, Zn-SOD activity in asthmatic airway epithelium: Correction by inhaled corticosteroid in vivo. Am. J. Physiol. 1997; 272: L148–L154
- Devasagayam T. P. Lipid peroxidation in rat uterus. Biochim. Biophys. Acta 1986; 876: 507–514
- Dworski R. Oxidant stress in asthma. Thorax 2000; 55(S2)S51–S53
- Elias J. A., Wu Y., Zheng T., Panettieri R. Cytokine and virus-stimulated airway smooth muscle cells produce IL-11 and other IL-6-type cytokines. Am. J. Physiol. 1997; 273: L648–L655
- Faizi S., Siddiqui B. S., Saleem R., Siddiqui S., Aftab K., Gilani A. H. Fully acetylated carbamate and hypotensive thiocarbamate glycosides from Moringa oleifera. Phytochemistry (Oxford) 1995; 38: 957–963
- Filipovic M., Snezana C. The role of eosinophils in asthma. Med. Biol. 2001; 8: 6–10
- Frigas E., Loegering D. A., Gleich G. J. Cytotoxic properties of the eosinophil major basic protein on tracheal epithelium. Lab Invest. 1980; 42: 35–43
- Gavett S. H., O'Hearn D. J., Li X., Huang S. K., Finkelman F. D., Wills K. M. Interleukin-12 inhibits antigen-induced airway hyperresponsiveness, inflammation, and TH2 cytokine expression in mice. J. Exp. Med. 1995; 182: 1527–1536
- Gharaee-Kermani M., Nozaki Y., Hatano K., Phan S. H. Lung interleukin-4 gene expression in a murine model of bleomycin-induced pulmonary fibrosis. Cytokine 2001; 15: 138–147
- Gokhale A. B., Dikshit V. J., Damre A. S., Kulkarni K. R., Saraf M. N. Influence of ethanolic extract of Tephrosia purpurea Linn. on mast cells and erythrocytes membrane integrity. Ind. J. Exp. Biol. 2000; 38: 837–840
- Gosset P., Tsicopoulos A., Wallaert B., Vannimenus C., Joseph M., Tonnel A. B., Capron A. Increased secretion by tumor necrosis factor-α and interleukin-6 by alveolar macrophages consecutive to the development of the late asthmatic reaction. J. Allergy Clin. Immunol. 1991; 88: 561–571
- Graham D. M., Blaiss M. S. Complementary/alternative medicine in the treatment of asthma. Ann. Allergy Asthma Immunol. 2000; 85: 438–447
- Guevara A. P., Vargas C., Sakurai H., Fujiwara Y., Hashimoto K., Maoka T., Kozuka M., Ito Y., Tokuda H., Nishino H. An antitumor promoter from Moringa oleifera Lam. Mutat. Res. 1999; 440: 181–188
- Guyton K. Z., Liu Y., Gorospe M., Xu Q., Holbrook N. J. Activation of mitogen-activated protein kinase by H2O2. Role in cell survival following oxidant injury. J. Biol. Chem. 1996; 271: 4138–4142
- Hatch G. E. Asthma, inhaled oxidants, and dietary antioxidants. Am. J. Clin. Nutr. 1995; 61: 625S–630S
- Hogberg J., Larson R. E., Kristoferon A., Orrenius S. NADPH-dependent reductase solubilised from microsomes of peroxidation and its activity. Biochem. Biophys. Res. Commun. 1974; 56: 836–842
- Ishimitsu R., Nishimura H., Kawauchi H., Kawakita K., Yoshikai Y. Dichotomous effect of a traditional Japanese medicine, Bu-zhong-yi-qi-tang, on allergic asthma in mice. Int. Immunopharmacol. 2001; 1: 857–865
- Janssen Y. M., Houten B., Borm P. J., Mossman B. T. Cell and tissue responses to oxidative damage. Lab. Invest. 1993; 69: 261–274
- Jatakanon A., Uasuf C., Maziak W. Neutrophilic inflammation in severe persistent asthma. Am. J. Respir. Crit. Care Med. 1999; 160: 1532–1539
- Kanazawa H., Kurihara N., Hirata K., Takeda T. The role of free radicals in airway obstruction in asthmatic patients. Chest 1991; 100: 1319–1322
- Karadi R. V., Gadge N. B., Alagawadi K. R., Savadi R. V., Mannur V. S., Kotti B. C. Antiarthritic activity of extracts of Moringa oleifera Lam. roots. Indian Drugs 2006; 43: 543–547
- Kinnula V. L., Crapo J. D., Raivio K. O. Biology of disease: Generation and disposal of reactive oxygen metabolites in the lung. Lab. Invest. 1995; 73: 3–19
- Kips J. C., Tavernier J., Pauwels R. A. Tumor necrosis factor causes bronchial hyperresponsiveness in rats. Am. Rev. Respir. Dis. 1992; 145: 332–336
- Kirtikar K. R., Basu B. D. Indian Medicinal Plants, 2nd Edition, D. Dun, B. Singh, M. P. Singh. Bishen Singh Mahendra Pal Singh, New Cannaught Place-Dehradun 1975; Vol. 1: 676–683
- Kouadio K., Zheng K. C., Tuekpe M. K., Todoriki H., Ariizumi M. Airway inflammatory and immunological events in a rat model exposed to toluene diisocyanate. Food Chem. Toxicol. 2005; 43: 1281–1288
- Laandrault N., Pouchert P., Ravel P., Gase F., Cros G., Teissedro P. L. Antioxidant activities phenolic level of French wines from different varieties and vintages. J. Agric. Food Chem. 2001; 49: 3341–3343
- Lane D. J., Lane T. V. Alternative and complementary medicine for asthma. Thorax 1991; 46: 787–797
- Li X. Y., Donaldson K., Rahman I., MacNee W. An investigation of the role of glutathione in increased epithelial permeability induced by cigarette smoke in vivo in vitro. Am. J. Respir. Crit. Care Med. 1994; 149: 1518–1525
- Lin J. Y., Chen M. L., Chiang B. L., Lin B. F. Ganoderma tsugae supplementation alleviates bronchoalveolar inflammation in an airway sensitization and challenge mouse model. Int. Immunopharmacol. 2006; 6: 241–251
- Louis R., Lau L. C., Bron A. O., Roldaan A. C., Radermecker M., Djukanovic R. The relationship between airway inflammation and asthma severity. Am. J. Respir. Crit. Care Med. 2000; 161: 9–16
- Lowry O. H., Rosenbrought N. J., Farr A. L., Randall R. J. Protein measurement with the Folin-phenol reagent. J. Biol. Chem. 1951; 193: 265–275
- Mahajan S. G., Mali R. G., Mehta A. A. Protective effect of ethanolic extract of seeds of Moringa oleifera Lam. against inflammation associated with development of arthritis in rats. J Immunotoxicol. 2007; 4: 39–47
- Mahajan S. G., Mali R. G., Mehta A. A. Anti-anaphylactic and anti-inflammatory activity of hydroalcoholic extract of leaves of Moringa oleifera. Planta Indica 2006; 2: 9–13
- Maestrelli P., Occari P., Turato G., Papiris S. A., Di Stefano A., Mapp C. E., Milani G. F., Fabbri L. M., Saetta M. Expression of IL-4 and IL-5 proteins in asthma induced by toluene diisocyanate (TDI). Clin. Exp. Allergy 1997; 27: 1292–1298
- Memon G. M., Khatri L. M. Isolation and spectroscopic studies of mono-palmitic, di-oleic triglyceride from seeds of Moringa oleifera Lam. Pak. J. Sci. Ind. Res. 1987; 30: 393–395
- Michael L., Horst K. Synthesis of active principles from the leaves of Moringa oleifera using S-pent-4-enyl thioglycosides. Carbohydr. Res. 1998; 312: 33–44
- Misra H. P., Fridovich I. The role of superoxide anion in the auto-oxidation of epinephrine and a simple assay for superoxide dismutase. Int. Biol. Chem. 1972; 247: 3170–3175
- Montuschi P., Collins J. V., Ciabattoni G., Lazzeri N., Corradi M., Kharitonov S. A. Exhaled 8-isoprostane as an in vivo biomarker of lung oxidative stress in patients with COPD and healthy smokers. Am. J. Respir. Crit. Care Med. 2000; 162: 1175–1177
- Moron M. S., Defierre J. W., Mannervik B. Levels of glutathione, glutathione reductase and glutathione-S-transferase activities in rat lung and liver. Biochim. Biophys. Acta 1979; 582: 67–78
- Motojima S., Frigas E., Loegering D. A., Gleich G. J. Toxicity of eosinophil cationic proteins for guinea-pig tracheal epithelium in vitro. Am. Rev. Respir. Dis. 1989; 139: 801–805
- Indian Materia Medica, K. M. Nadkarni, A. K. Nadkarni. Popular Prakashan, Mumbai 1976; Vol. 1: 811–816
- Nair A. M., Saraf M. N. Inhibition of antigen and compound 48/80 induced contractions of guinea pig trachea by the ethanolic extract of the leaves of Vitex negundo Linn. Ind. J. Pharmacol. 1995; 27: 230–233
- Nayampalli S., Desai N. K., Ainapure S. S. Anti-allergic properties of Tinospora cordifolia in animal models. Ind. J. Pharmacol. 1986; 18: 250–252
- Njoku O. U., Adikwu M. U. Investigation on some physicochemical antioxidant and toxicological properties of Moringa oleifera seed oil. Acta. Pharma. Zagr. 1997; 47: 287–290
- Novak Z., Nemeth I., Gyurkovits K., Varga S. I., Matkovics B. Examination of the role of oxygen free radicals in bronchial asthma in childhood. Clin. Chim. Acta 1991; 201: 247–252
- Ohkawara Y., Yamauchi K., Tanno Y., Tamura H., Outani H., Nagura H., Ohkuda K., Takishima T. Human lung mast cells and pulmonary macrophages produce tumor necrosis factor-α in sensitized lung tissue after IgE receptor triggering. Am. J. Respir. Cell Mol. Biol. 1992; 7: 385–392
- Ohno I., Ohkawara Y., Yamauchi K., Tanno Y., Takishima T. Production of tumour necrosis factor with IgE receptor triggering from sensitized lung tissue. Am. J. Respir. Cell Mol. Biol. 1990; 3: 285–289
- Ordonez C. L., Shaughnessy T. E., Matthay M. A., Fahy J. V. Increased neutrophil numbers and IL-8 levels in airway secretions in acute severe asthma: Clinical and biologic significance. Am. J. Respir. Crit. Care. Med. 2000; 161: 1185–1190
- Modern Methods of Plant Analysis, D. Paech, M. V. Tracey. Springer-Verlag, Berlin 1955; Vol. IV: 373–374
- Paredi P., Kharitonov S. A., Barnes P. J. Analysis of expired air for oxidation products. Am. J. Respir. Crit. Care Med. 2002; 166: 31–37
- Plitnick L. M., Loveless S. E., Ladics G. S., Holsapple M. P., Smialowicz R. J., Woolhiser M. R., Anderson P. K., Smith C., Selgrade M. J. Cytokine mRNA profiles for isocyanates with known and unknown potential to induce respiratory sensitization. Toxicology 2005; 207: 487–499
- Rahman I., MacNee W. Role of oxidants/antioxidants in smoking induced lung diseases. Free Rad. Biol. Med. 1996; 21: 669–681
- Rahman I., MacNee W. Role of transcription factors in inflammatory lung diseases. Thorax 1998; 53: 601–612
- Rahman I., MacNee W. Lung glutathione and oxidative stress: Implications in cigarette smoke-induced airway disease. Am. J. Physiol. 1999; 277: L1067–L1088
- Rahman I., MacNee W. Oxidative stress and regulation of glutathione in lung inflammation. Eur. Respir. J. 2000; 16: 534–554
- Rahman I., Biswas S. K., Kode A. Oxidant and antioxidant balance in the airways and airway diseases. Eur. J. Pharmacol. 2006; 533: 222–239
- Rajan B. K. Apiculture and farm forestry in semi-acid tracts of Karnataka. My Forest. 1986; 22: 41–49
- Robinson D. S., Hamid Q., Sun Y., Tsicopoulos A., Barkans J., Bentley A. M., Corrigan C., Durham S. R., Kay A. B. Predominant TH2-1ike bronchoalveolar T-lymphocyte population in atopic asthma. New Engl. J. Med. 1992; 326: 298–304
- Sanchez M. D., Lopez C. J., Rios V. High-performance liquid chromatography method to measure α- and γ -tocopherol in leaves and fresh beans from Moringa oleifera. J. Chromatogr. A 2006; 1105: 111–114
- Sedwick J. B., Geiger K. M., Busse W. W. Superoxide generation by hypodens eosinophils from patients with asthma. Am. Rev. Respir. Dis. 1990; 142: 120–125
- Seltzer J., Bigby B. G., Stulbarg M., Holtzman M. J., Nadel J. A., Ueki I. F., Leikauf G. D., Goetzl E. J., Boushey H. A. Ozone-induced change in bronchial reactivity to methacholine and airway inflammation in humans. J. Appl. Physiol. 1986; 60: 1321–1326
- Sengupta A., Gupta M. P. Studies on seed fat composition of Moringacea family. Fette Seifen Anstrich. 1970; 72: 6–10
- Sergio R. The role of lymphocytes in allergic diseases. J. Allergy Clin. Immunol. 2000; 105: 399–408
- Shanmugasundaram K. R., Kumar S. S., Rajajee S. Excessive free radical generation in the blood of children suffer from asthma. Clin. Chim. Acta 2001; 305: 107–114
- Singh R. K., Bhattacharya S. K., Acharya S. B. Studies on extracts of Elaeocarpus sphaericus fruits on in vitro rat mast cells. Phytomedicine 2000; 7: 205–207
- Sinha A. K. Colorimetric assay of catalase. Anal. Biochem. 1972; 47: 389–394
- Sur S., Crotty T. B., Kephart G. M. Sudden onset fatal asthma: A distinct clinical entity with few eosinophils and relatively more neutrophils in the airway submucosa?. Am. Rev. Respir. Dis. 1993; 148: 713–719
- Tanaka K., Okamoto Y., Nagaya Y. A nasal allergy model developed in the guinea pig by intranasal application of 2,4-toluene diisocyanate. Int. Arch. Allergy Appl. Immunol. 1988; 85: 392–397
- Tekin D., Ayse S. B., Mungan D., Misirligil Z., Yavuzer S. The antioxidant defense in asthma. J. Asthma 2000; 37: 59–63
- Tripathi R. M., Das P. K. Studies on anti-asthmatic and anti-anaphylactic activity of Albizzia lebbeck. Ind. J. Pharmacol. 1977; 9: 189–194
- Varier V. P. Indian Medicinal Plants Compendium of 500 Species, P. K. Warrier, V. P. Nambiar, C. Ramankutty. Orient Longman Ltd. 1997; Vol. 4: 58–62
- Varshavskii B. I., Trubnikov G. V., Galaktipmpva L. P., Koreniak N. A., Koledeznaia I. L., Oberemok A. N. Oxidant-antioxidant status of patients with bronchial asthma during inhalation and system glucocorticoid therapy. Ter. Arh. 2003; 75: 21–24
- Villasenor I. M. Bioactive metabolites from Moringa oleifera Lam. Kimika 1994; 110: 47–52
- Villasenor I. M., Dayrit F., Lim-Sylianco C. Y. Studies on M. oleifera seeds, Part II. Thermal degradation of roasted seeds. Phil. J. Sci. 1990; 119: 33–39
- Villasenor I. M., Lim-Sylianco C. Y., Dayrit F. Mutagens from roasted seeds of Moringa oleifera. Mutat. Res. 1989; 224: 209–212
- Wardlaw A. J., Dunnette S., Gleich G. J., Collins J. V., Kay A. B. Eosinophils and mast cells in bronchoalveolar lavage in subjects with mild asthma: Relationship to bronchial hyperreactivity. Am. Rev. Respir. Dis. 1988; 137: 62–69
- Wenzel S. E., Szefler S. J., Leung D. Y. Bronchoscopic evaluation of severe asthma. Am. J. Respir Crit. Care Med. 1997; 156: 737–743
- Wisnewski A. V., Redlich C. A. Recent developments in diisocyanate asthma. Curr. Opin. Allergy Clin. Immunol. 2001; 1: 169–175
- Wood L. G., Gibson P. G., Garg M. L. Biomarkers of lipid peroxidation, airway inflammation, and asthma. Eur. Respir. J. 2003; 21: 177–186
- Ying S., Humbert M., Barkans J., Corrigan C. J., Pfister R., Menz G., Larche M., Robinson D. S., Durham S. R., Kay A. B. Expression of IL-4 and IL-5 mRNA and protein product by CD41 and CD81 T-cells, eosinophils, and mast cells in bronchial biopsies obtained from atopic and nonatopic (intrinsic) asthmatics. J. Immunol. 1997; 158: 3539–3544
- Zheng K. C., Ariizumi M., Todoriki H., Nong D. X. Cytokine profiles in airways of rats exposed to toluene diisocyanate. J. Occup. Health 2001a; 43: 39–45
- Zheng K. C., Nong D. X., Morioka T., Todoriki H., Arizumi M. Elevated IL-4 and IL-6 in rats sensitized with toluene diisocyanate. Ind. Health 2001b; 39: 334–339
- Zhimmet I., Tashkin D. P. Alternative medicines for allergy and asthma. J. Allergy Clin. Immunol. 2000; 106: 603–614
- Zhu Z., Tang W., Ray A., Wu Y., Einarsson O., Landry M. L., Gwaltney J., Elias J. A. Rhinovirus stimulation of IL-6 in vivo in vitro: Evidence for NFκ B-dependent transcriptional activation. J. Clin. Invest. 1996; 97: 421–430