Abstract
Pteridium aquilinum (bracken fern) is one of the most common plants. Epidemiological studies have revealed a higher risk of certain types of cancers (i.e., esophageal, gastric) in people who consume bracken fern directly (as crosiers or rhizomes) or indirectly through the consumption of milk from livestock that fed on the plant. In animals, evidence exists regarding the associations between chronic bracken fern intoxication, papilloma virus infection, and the development of carcinomas. While it is possible that some carcinogens in bracken fern could be responsible for these cancers in both humans and animals, it is equally plausible that the observed increases in cancers could be related to induction of an overall immunosuppression by the plant/its various constituents. Under the latter scenario, normal tumor surveillance responses against nascent (non-bracken-induced) cancers or responses against viral infections (specifically those linked to induction of cancers) might be adversely impacted by continuous dietary exposure to this plant. Therefore, the overall objective of this study was to evaluate the immunomodulatory effects of bracken fern following daily ingestion of its extract by a murine host over a period of 14 (or up to 30) days. In C57BL/6 mice administered (by gavage) the extract, histological analyses revealed a significant reduction in splenic white pulp area. Among a variety of immune response parameters/functions assessed in these hosts and isolated cells, both delayed-type hypersensitivity (DTH) analysis and evaluation of IFNγ production by NK cells during TH1 priming were also reduced. Lastly, the innate response in these hosts—assessed by analysis of NK cell cytotoxic functionality—was also diminished. The results here clearly showed the immunosuppressive effects of P. aquilinum and that many of the functions that were modulated could contribute to the increased risk of cancer formation in exposed hosts.
Abbreviations: | ||
BEH, | = | bovine enzootic hematuria |
BPV, | = | bovine papilloma virus |
CFSE, | = | 5-(and 6-)-carboxyfluorescein diacetate succinimidyl ester |
DC, | = | dendritic cells |
DTH, | = | delayed-type hypersensitivity |
DCFH-DA, | = | dichlorofluorescein diacetate |
FCA, | = | complete Freund’s adjuvant |
FBS, | = | fetal bovine serum |
FITC, | = | fluorescein isothiocyanate |
HA, | = | hemagglutination |
HE, | = | hematoxylin and eosin |
LPS, | = | lipopolysaccharides |
NK, | = | natural killer |
PBS, | = | phosphate-buffered saline |
PE, | = | R-phycoerythrin |
PE-Cy7, | = | R-phycoerythrin - cyanine dye 7 |
PerCP-Cy5.5, | = | peridinin chlorophyll protein - cyanine dye 5.5 |
PFC, | = | plaque forming cells |
PHA, | = | phytohemagglutinin A |
PI, | = | propidium iodide |
PMA, | = | phorbol myristate acetate |
RBC, | = | erythrocyte |
SAPI, | = | Staphylococcus aureus conjugated with propidium iodide |
SRBC, | = | sheep red blood cells |
TH1, | = | T-helper 1 |
TT, | = | tetanus toxoid |
UAT, | = | upper alimentary tract |
WBC, | = | leukocyte |
Introduction
Bracken fern (Pteridium aquilinum) is one of the most common plants (Taylor, Citation1990). Epidemiological studies have revealed a higher risk of certain types of cancers (i.e., esophageal, gastric) in people who consume bracken fern (or its toxins) directly, as in case of crosiers or rhizomes (Sugimura, Citation2000; Abnet, Citation2007), or indirectly through consumption of milk from livestock that have fed on the plant (Alonso-Amelot et al., Citation1996, Citation1999; Alonso-Amelot, Citation1997; Shahin et al., Citation1999; Alonso-Amelot and Avendaño, Citation2002).
Although several chemical constituents of the bracken ferm have been identified/isolated, only a few are known to possess any carcinogenic potential. For example, ptaquiloside has been shown (and the mechanism defined) to induce cancers in experimental animals (Hirono et al., Citation1987; Ojika et al., Citation1987). In contrast, effects of quercetin have been much more ambiguous: Pamukcu et al. (Citation1980) reported the constituent caused bladder\intestinal tumors in rats; Ngomuo and Jones (Citation1996) could demonstrate no genotoxic effect of this agent in mice; Harwood et al. (Citation2007)—in a review—concluded that normal dietary intake of quercetin did not produce adverse health effects; and, the American Cancer Society (2007) indicated that quercetin “has been promoted as being effective against a wide variety of diseases, including cancer”, but “as of yet there is no reliable clinical evidence that quercetin can prevent or treat cancer in humans.”; in the amounts consumed in a healthy diet, quercetin “is unlikely to cause any major problems”.
While it is possible that some carcinogens in bracken fern could be responsible for the cancers reported in both humans and animals, it is also plausible that these outcomes could be related to induction of an overall immunosuppression by the plant/its various constituents. Under this latter scenario, normal tumor surveillance responses against nascent (non-bracken-induced) cancers or immune responses against viral infections (specifically those linked to induction of cancers) might be adversely impacted by continuous dietary exposure to this plant.
There is suggestive evidence in support of this immunotoxicologically “indirect” means for increasing the risk of cancer development in hosts chronically intoxicated with bracken fern. For example, in cattle, ingestion of bracken fern causes an acute hemorrhagic syndrome associated with depression of the bone marrow (a pathology characterized by leukopenia and thrombocytopenia) (Riet-Correa, Citation2001; Do Nascimento França et al., 2002). Chronic exposure to the fern has also been related in increases in bladder carcinomas characterized by hemorrhages in the organ (termed as bovine enzootic hematuria [BEH]) (Peixoto et al., Citation2003; Carvalho et al., Citation2006). Because it has been known for more than four decades that the presence of papillomas (live bovine papilloma virus [BPV] or as formalized vaccine) also gives rise to these same outcomes (Pamukcu et al., Citation1967), the immunosuppression we hypothesize here that is induced by fern intoxication could give rise to these particular cancers and the associated BEH (specifically, BPV-2: Campo et al., Citation1992; Campo, Citation1997; Balcos et al., Citation2008) as a result of these viruses being able to flourish. A similar immunomodulation-based scenario could be adapted to explain the reported increases in BPV-4-induced squamous cell carcinomas of the upper alimentary tract (Borzacchiello et al., Citation2003; Souto et al., Citation2006) in livestock that routinely consumed bracken fern.
To the best of our knowledge, no formal dedicated demonstration of the effects of P. aquilinum on the immune system of either grazing or experimental animals has been reported in the literature. Thus, the objective of this study was to evaluate the possible immunomodulatory effects (in particular, those endpoints reflective of host general immune status and some specific to host anti-tumor resistance) of bracken fern in mice repeatedly provided (by gavage) an extract of this plant over a period of weeks.
Materials and methods
Mice
Sixty-day-old male C57Bl/6 mice (weighing 23.9 [± 1.48] g at onset of the experiment), bred in the Department of Pathology at the School of Veterinary Medicine and Animal Sciences, were used. The mice were maintained under controlled conditions of temperature (22–25°C), relative humidity (50-65%) and lighting (12 hour/12 hour light/dark cycle). Drinking water and standard diet (Nuvilab-CR1®, Nuvital Nutrientes LTDA) were provided ad libitum. All procedures using the mice were performed following “Guide for the Care and Use of Laboratory Animals” (NIH publication No. 85-23; at http://www.nap.edu/readingroom/books/labrats/) and were reviewed and approved by the Bioethics Committee of the FMVZ-USP (process #620/2005 and process #1061/2007).
Chemicals and reagents
Complete Freund’s adjuvant (FCA), lipopolysaccharide (LPS 0127:B8), phorbol myristate acetate (PMA), dichlorofluorescein diacetate (DCFH-DA), propidium iodide (PI), diethylaminoethyl-dextran (DEAE-Dextran), and quercetin were obtained from Sigma Chemical Co. (St. Louis, MO). Phytohemagglutinin A (PHA; M form), RPMI-1640 medium, and fetal bovine serum (FBS) were from Gibco (Carlsbad, CA). Thiamine (B1 vitamin) was from Labsynth (Sao Paulo, Brazil), 5-(and 6)-carboxyfluorescein diacetate succinimidyl ester (CFSE) was from Molecular Probes (Carlsbad, CA), and EDTA from Merck (Darmstadt, Germany). Transfusion pack Biopack® was from Halex Istar Indústria Farmacêutica Ltda (Goiania, Brazil) and Zoletil 50® from Virbac Saúde Animal (Sao Paulo, Brazil). Glass slides and coverslips were from GlassTécnica (Sao Paulo, Brazil). The Limulus Amebocyte Lysate (LAL) test (Charles River Endosafe®, U.S. License No. 1197) was obtained from Charles River Laboratories, Inc. (Charleston, SC).
Tetanus toxoid (TT) without adjuvant (titer 3200 LF/ml) lot n°: IB – AnT/PAG 04/03 was produced by the Butantan Institute (Sao Paulo, Brazil). Phycoerythrin (PE)-labeled anti-CD19, PE-anti-CD4, PE-anti-CD8a, PE-cyanine 7 (PE-Cy7)-labeled anti-CD11c, PE-labeled anti-CD83, peridinin chlorophyll-cyanine dye 5.5 (PerCP-Cy5.5)-labeled anti-NK1.1, PE-anti-NKG2D, and fluorescein isothiocyanate (FITC)-labeled anti-interferon (IFN)-γ antibodies were from BD Pharmingen (BD Biosciences, San Jose, CA); FITC- anti-rat F(ab’)2 and anti-interleukin (IL)-12 were from Invitrogen. Skimmed milk (Difco™ Skim Milk) and kit BD Cytofix/Cytoperm™ Plus with BD GolgiPlug™ Protein Transport Inhibitor (containing Brefeldin A) were purchased from BD Biosciences (San Jose, CA).
Pteridium aquilinum
A voucher specimen (N° MH 513) was deposited at the Laboratory of Chemistry of Natural Products, Centre of Animal Health, Biological Institute of Sao Paulo. Pteridium aquilinum var. arachnoideum was identified by Dr. Jefferson Prado, a specialist in Pteridophyta identification from the Botanical Institute of Sao Paulo.
Pterdium aquilinum buds were collected in Pirassununga, Sao Paulo, Brazil, in February, 2005. The buds were maintained frozen at -80°C until extract preparation. In addition, a sample was dried and submitted to quercetin HPLC analysis (Shimadzu Model LC-10AT, Kyoto, Japan), which detected 0.08% quercetin concentration in dry matter of P. aquilinum buds. For extract preparation, the frozen buds (1 kg) were ground up and submitted to pressure to yield a viscous residue (60 g; yield 6%). The extract was maintained frozen at -80°C and, at the moment of administration, was suspended in distilled water and used in doses equivalent to 1, 10 and 30 g of plant/kg body weight (BW) (i.e., 60, 600, and 1800 mg of extract residue/kg BW); these doses were equivalent to 0.2, 2, and 6 mg of quercetin/kg BW, respectively. Doses of 10 and 30 g of P. aquilinum, respectively, are responsible for chronic and acute natural intoxication of bovine in Brazil (Tokarnia et al., Citation2000). Any potential contamination by endotoxin was assessed by the Limulus Amebocyte Lysate (LAL) test; levels of endotoxin in the samples were determined as < 0.125 EU/ml in the extract, a value that corresponds to the detection limit of the assay.
Experimental design
In general, experimental mice were treated by daily gavage either for 14 days with 1 (P1), 10 (P10) or 30 g of plant/kg BW (P30), or for 30 days with 30 g of plant/kg BW (P30’), with the same lot of prepared P. aquilinum extract. Control (Co) mice received only water and were treated at the same time as the experimental mice. In addition, all mice were supplemented with Vitamin B1 in water ( 10 mg/ml), as proposed by Schacham et al. (Citation1970), throughout the treatment period to avoid the effects of thiaminase 1, a component of P. aquilinum (Fenwick, Citation1988). Subsequent to the 14- or 30-day treatments, mice were then randomly designated into one of four regimens (see describing Regimens 1A-1D) for analyses of the various endpoints outlined herein. The utility of the assays for detection of any immunomodulatory effects from the exposures to the P. aquilinum extract has been verified previously in our laboratory in pilot/other studies that employed methylprednisolone treatments of mice as a positive control (data not shown here; Readers are directed to Caniceiro et al. [Citation2008] to examine some of these particular assays results).
Throughout the various exposures, body weight of each mouse was measured every 3 days for dose adjustment. Food and water consumption were also measured every three days throughout this period.
Figure 1. Treatment schedules: mice were treated with 1 (P1), 10 (P10) or 30 (P30) g/kg BW of P. aquilinum and supplemented with B1 vitamin in water ( 10 mg/ml) for 14 (or up to 30) days. A. Mice were not immunized after P. aquilinum treatment. B. One day after P. aquilinum treatment, mice were immunized with SRBC. C. One day after P. aquilinum treatment, P309 and control mice were sensitized with SRBC and 7 days later were challenged with SRBC. D. One day after P. aquilinum treatment, mice were immunized with TT and 7 days later, splenocytes were challenged with TT in vitro.
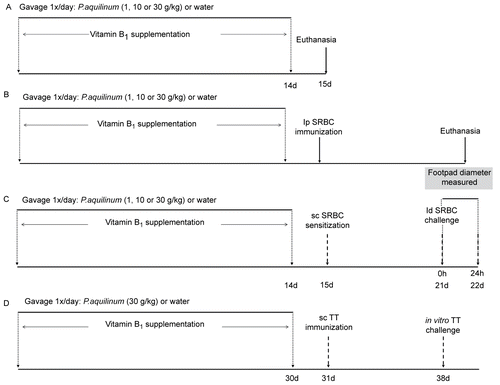
Preparation of sheep red blood cells (SRBC)
A healthy adult sheep from the Veterinary Hospital of the Faculty of Veterinary Medicine and Animal Sciences, Sao Paulo, Brazil, was used. Blood ( 200 ml) was collected from the jugular vein with a transfusion pack Biopack® and kept at 4°C in sterile conditions until use. For SRBC preparation used for immunization, blood was centrifuged at 2000 rpm for 8 minutes, washed three times with phosphate-buffered saline (PBS, pH 7.4) and diluted to provide the desired concentration by a counter (CC-530 Celm®, Sao Paulo, Brazil).
Guinea pig complement
Four healthy adult guinea pigs (from Biological Institute of Sao Paulo, Brazil) were used. The guinea pigs were subjected to intramuscular anesthesia with Zoletil 50® ( 20 mg/kg) prior to blood collection by cardiac puncture. Recovered blood samples were allowed to stand for 45 minutes at room temperature before being centrifuged at 4000 rpm for 10 minutes. The serum from each sample was recovered, pooled, and then aliquoted into smaller volume samples and placed at - 20°C until required.
Lymphoid organ and histological evaluation
On Day 15 of the experiment, a subset of control and treated mice (from Regimen 1A) was euthanized by decapitation. After blood samples had been collected from each mouse (see below), their respective liver, spleen, and thymus organs were harvested and weighed to calculate their relative weight. Cell suspensions of a representative portion of the spleen, as well as bone marrow from one femur of each mouse, were prepared to verify (using a hemocytometer) the cellularity of these immunologically critical sites.
Histopathologic analyses were performed on sections (5 μm thick) of each thymus, spleen, and liver, as well as isolated mesenteric lymph nodes, Peyer’s patches, sternum bone marrow, and kidney from each mouse, that had been stained with hematoxylin and eosin (HE).
Morphometric analysis
Representative sections of the mouse spleens isolated above were submitted to morphometric analysis in an Image-Pro Plus system, Version 4.5 (Media Cybernetics Inc, Bethesda, MD). White pulps were defined with an electronic pen and the software calculated each area automatically.
Blood samples
Blood from these mice was collected in a 1.5 ml tube containing 10 μl EDTA. Erythrocyte (RBC) and leukocyte (WBC) counts were subsequently performed using a CC-530 Celm® counter.
Lymphocyte phenotyping of spleen
A second subset of Regimen 1A treated and control mice was also sacrificed on Day 15 to prepare splenic cell suspensions. Briefly, for each mouse, the isolated spleen was gently fragmented by squeezing between the ends of two sterile microscope slides in cold RPMI medium. The erythrocytes present in the suspension were then lysed with 0.4% ammonium chloride sterile solution. Cell viability of the non-RBC cells was then determined via trypan blue exclusion. Triplicate samples of 1 × 106 cells were then each incubated for 1 hour (in the dark) with phycoerythrin (PE)-conjugated anti-CD4, anti-CD8a, or anti-CD19. The cells were then washed free of unbound antibody and resuspended in PBS for flow cytometric acquisition in a FACS Calibur flow cytometer equipped with Cell Quest Pro® software (Becton Dickinson [BD] Immunocytometry System). Data analyses were realized with FlowJo 7.2.2® software (Tree Star Inc, Ashland, KY).
Splenocyte proliferation assay
A third subset of Regimen 1A treated and control mice was also sacrificed on Day 15 to prepare splenic cell suspensions. Cell suspensions of the splenic tissue from each mouse were prepared as outlined above except that in this study, the cells were ultimately re-suspended in RPMI complete medium (RPMI supplemented with 10% FBS). The samples were incubated on 6-well plates (≅ 1 × 108 cells/well) for 2 hours at 37°C in a humidified atmosphere containing 5% CO2 to permit macrophage adhesion. The non-adherent cells from each well were then collected, then numbers adjusted to 1 × 107 cells/ml, and these cells were then incubated with 1 μl CFSE for 20 minutes at 37°C in the dark. After staining, the cells were washed, adjusted to 2 × 106 cells/ml in complete RPMI medium, and transferred (in 100 μl aliquots) to the wells of a 96-well microtiter plate. Triplicate wells were then received vehicle (untreated control group), Phytohemagglutinin A (PHA; 10 μl/well), or LPS (30 μg/well), and the cells were then incubated for 96 hours at 37°C in a humidified atmosphere with 5% CO2. Cells were then acquired by flow cytometry (FACS Calibur System), with logarithmic detection of green fluorescence, and analyzed using FlowJo 7.2.2® software. The percentage of proliferation was calculated as described by Lyons (Citation2000).
Natural killer (NK) cell activity assay
A fourth subset of Regimen 1A treated and control mice was also sacrificed on Day 15 to prepare splenic cell suspensions. Cell suspensions of the splenic tissue from each mouse were prepared as outlined above, and NK (effector cell) activity in the splenic preparation was then measured against the YAC-1 mouse lymphoma cell line target, as described by Marcusson-Stahl and Cederbrant (Citation2003). Briefly, cell cultures containing 5 × 105 effector and 5 × 103 YAC-1 cells (stained with CFSE [E:T ratio = 100:1]) were incubated 20 hours at 37°C in a humidified atmosphere containing 5% CO2. Spontaneous death was determined by incubating YAC-1 tumor cells only in complete medium. PI was then added and the samples measured by flow cytometry. Two thousand target cells were collected by flow cytometry (FACS Calibur) and analyzed using FlowJo 7.2.2® software. The level of NK cell cytotoxicity was expressed as:
Peritoneal macrophage oxidative burst and phagocytosis analysis
On Day 10 of the experiment, a dedicated fifth subset of Regimen 1A control and treated mice were subjected to IP injection with 1 ml of 10% thioglycollate. On Day 15, these mice were euthanized and their peritoneal macrophages collected in cold PBS. For analysis of the oxidative burst, cells obtained from each mouse (1 × 106 cells/tube) were incubated for 30 minutes at 37°C with either dichlorofluorescein diacetate (DCFHDA) or DCFH-DA + phorbol myristate acetate (PMA) or DCFH-DA + Staphylococcus aureus conjugated with propidium iodide (SAPI). For the analysis of phagocytic activity, cells obtained from each mouse (1 × 106 cells/tube) were incubated for 30 minutes at 37°C with either SAPI or DCFH-DA and SAPI. Ten thousand macrophages were then acquired by flow cytometry (FACS Calibur) and the data were analyzed using FlowJo 7.2.2® software.
Assay of plaque forming cells (PFC) and hemagglutination (HA) titer
A set of treated and control mice (from Regimen 1B) was subjected to intraperitoneal (IP) immunization with 200 μl of SRBC suspension (5 × 108 cells/ml) on Day 15 to determine the PFC assay and HA titers. Seven days after immunization, the mice were euthanized, their blood was collected to separate serum for primary antibody titers, and their spleens removed to prepare cell suspensions. For the PFC assay, 35 μl of SRBC (1:2 in PBS) combined with 25 μl of guinea pig complement and 340 μl Bacto agar (0.5 % in RPMI and 1. 6 ml of 0.05% DEAE-Dextran solution/ 100 ml agarose) at 47°C was added to 100 μl of spleen cell suspension (2 × 106 cells/ml). Cunningham chambers were prepared using a glass slide (26 × 76 mm) and cover slips (22 × 22 mm). The chambers were loaded with 50 μl of assay mixture, sealed, and incubated for 3 hours at 37°C in a humidified atmosphere at 5% CO2. The plaques were then counted under a light microscope and expressed as PFC per 105 spleen cells and PFC per spleen (total cellularity).
For quantification of HA titers, antibody levels were assessed by the modified hemagglutination technique described by Liu et al. (Citation2005). Briefly, serial dilutions of 25 μl of each serum were realized in an equal volume of saline in a microtitration plate, to which 25 μl of SRBC suspension in saline (9 × 108 cells/ml) was added. After mixing, the plates were incubated for 1 hour at 37°C in a humidified atmosphere containing 5% CO2. The reciprocal of the highest dilution of test serum that presented agglutination was determined as the antibody titer (expressed as log2).
Measurement of delayed-type hypersensitivity (DTH)
On Day 15 of the experiment, a set of treated and control mice (from Regimen 1C) was subjected to subcutaneous (SC) sensitization with 50 μl of 1 × 108 SRBC/ml (in a 1:1 PBS:FCA solution). Six days later, the diameter of the left hind footpad was measured (0 hour value). The mice were then challenged by SC injection of 20 μl of 1 × 108 SRBC/ml (in PBS only) into the left hind footpad. The footpad diameter was measured again 24 hours later; the swelling was calculated as the difference in diameter at 0 vs. 24 hours, and expressed in millimeters.
Evaluation of dendritic cells (DC) and NK cells during TH1 priming
On Day 31 of the experiment, a set of mice that had received the vehicle or the P. aqulinum extract (i.e., Regimen 1D) was subjected to SC immunization with 100 μl of Tetanus Toxoid (TT) [50 LF {limes flocculans}/ml] in 1:1 of PBS and FCA. Seven days after immunization, the hosts were sacrificed and their spleens collected to prepare cell suspensions. Splenocytes were centrifuged at 1200 rpm (4°C, 8 minutes) and the pelleted cells were resuspended in RPMI complete medium supplemented with 10% FBS. The samples were incubated on 6-well plates for 2 hours at 37°C in a humidified atmosphere containing 5% CO2 to separate adherent from non-adherent cells. The separated non-adherent cells were adjusted to 38.7 × 105 cells/ml; adherent cells were recovered from the plates using complete RPMI medium at 4°C, and their numbers then adjusted to 1.3 × 105 cells/ml. The cell populations were then co-incubated at a non-adherent:adherent cell ratio of 30:1 (in 100 μl aliquots) in the wells of a 96-well microtiter plate.
Triplicate cultures for each challenge then received vehicle (untreated) or TT (20 LF/well) and were incubated 3 hours at 37°C in a humidified atmosphere with 5% CO2. After stimulation, GolgiPlug™ (Brefeldin A) was added (following manufacturer’s protocol) and the cells were cultured an additional 2 hours. Cells were then blocked with 5% skimmed milk for 1 hour at 4°C and then stained (simultaneously) for surface antigens for 30 minutes at 4°C in the dark. The cells were then washed in 2 ml PBS, fixed and permeabilized with a Cytofix/Cytoperm Plus Kit (following manufacturer’s protocol). At the permeabilization step, the cells were stained intracellularly for 30 minutes at 4°C in the dark, washed, and then analyzed in flow cytometry. Twenty thousand target cells were collected by flow cytometry (FACS Calibur) and the results were expressed as a percentage. The data were analyzed using FlowJo 7.2.2® software.
Antigen-specific T-lymphocyte expansion
Antigen specific T-lymphocyte expansion was performed on the same samples collected from mice used for DC and NK cell evaluation during TH1 priming. The protocol used is the same as immediately-outlined above, except that after the 2-hour incubation to separate adherent from non-adherent cells, the supernatants bearing the non-adherent cells were adjusted to 1 × 107 cells/ml and incubated with 1 μl CFSE for 20 minutes at 37°C in the dark. After staining, the cells were washed, adjusted to 38.7 × 105 cells/ml ml; adherent cells were recovered from the plates using complete RPMI medium at 4°C, and their numbers then adjusted to 1.3 × 105 cells/ml. The cell populations were then co-incubated at a non-adherent:adherent cell ratio of 30:1 (in 100 μl aliquots) in a 96-well microtiter plate. Triplicate cultures for each challenge then received vehicle (untreated) or TT (20 LF/well) and were incubated 72 hours at 37°C in a humidified atmosphere with 5% CO2. Twenty thousand target cells were then collected by flow cytometry (FACS Calibur) and analyzed using FlowJo 7.2.2® software. Antigen-specific T-lymphocyte expansion was expressed as
Statistical analysis
The data were analyzed using GraphPad Prism 4.00® software (GraphPad Software, Inc., San Diego, CA) by one-way analysis of variance (ANOVA) followed by Dunnett’s test for multiple comparisons. Percentage data from three or more groups were compared by the Kruskal–Wallis test followed by Dunn’s test and percentage data from two groups were compared by the Mann–Whitney test. All data were expressed as the mean ± SD and differences were considered to be statistically significant at p < 0.05.
Results
Effects of P. aquilinum treatment on food consumption, body and lymphoid organ weight, and on lymphoid (and non-lymphoid) organ cellularity and histology
Among those mice that were subjected to Regimen 1A, food consumption, body weight, body weight gain, relative thymic and splenic weights, and spleen cellularity were all unaffected by the 14-day treatment (data not shown). Furthermore, histological evaluations of samples of bone marrow, thymus, mesenteric lymph nodes, Peyer’s patches, liver and kidney recovered from each P. aquilinum-treated mouse also revealed no differences from the samples obtained from control mice (data not shown).
Effects of P. aquilinum treatment on lymphoid organs
The effect of P. aquilinum treatment on bone marrow cellularity among those mice that were subjected to Regimen 1A is shown in . Bone marrow cellularity was diminished in all groups treated with P. aquilinum (p < 0.0001, one-way ANOVA). Histological analysis of the spleen is shown in . The P30 group-mice displayed a significant reduction in white pulp area as determined by morphometric analysis (p = 0.004, Mann-Whitney test) ().
Figure 2. Reduction in the bone marrow cellularity of mice treated with 1 (P1), 10 (P10) and 30 (P30) g/kg BW of P. aquilinum and supplemented with B1 vitamin in water ( 10 mg/ml) for 14 days (p < 0.0001, vs. control values; one-way ANOVA followed by Dunnett’s test). Data are expressed as the mean ± SD (n = 9).
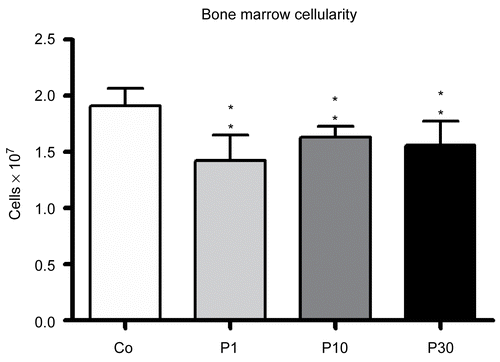
Figure 3. Effects in the spleens of mice treated with 30 (P30) g/kg BW of P. aquilinum and supplemented with B1 vitamin in water ( 10 mg/ml) for 14 days. A. Representative spleen sections from the control group and from the P30 group. Observe smaller white pulp area in spleens from the P30 group. Bar = 100 µm. B. Morphometric analyses showed a significant reduction in white pulp area in spleens from the P30 group (p = 0.004, Mann–Whitney test). Data are expressed as the mean ± SD (n = 9).
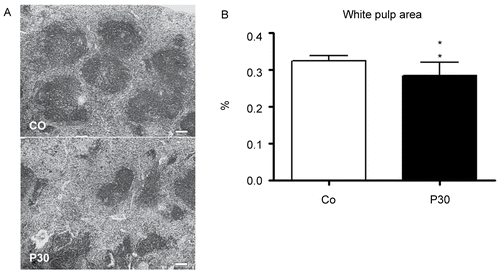
Effects of P. aquilinum treatment on spleen lymphocyte phenotypes
Spleen lymphocyte phenotyping was performed to determine the proportion of CD4+ T-lymphocytes, CD8+ T-lymphocytes and B-lymphocytes in the organs of mice that were subjected to Regimen 1A. The percentages of these cells remained unaltered in P. aquilinum-treated mice compared with control mice (data not shown).
Effects of P. aquilinum treatment on primary antibody response to SRBC
Primary antibody response to SRBC were assessed (by PFC and HA titer techniques) among mice that were subjected to Regimen 1B. The PFC per 105 splenocytes and per spleen and HA titers all remained unaltered in P. aquilinum-treated mice compared with control mice ().
Figure 4. Effects on primary antibody response to SRBC of mice treated with 1 (P1), 10 (P10) and 30 (P30) g/kg BW of P. aquilinum and supplemented with B1 vitamin in water ( 10 mg/ml) for 14 days. The PFC per 105 splenocytes and per spleen and HA titers all remained unaltered in P. aquilinum-treated mice compared with control mice. Data are expressed as the mean ± SD (n = 9).
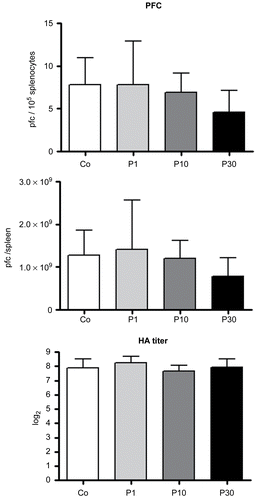
Effects of P. aquilinum treatment on cellular immune response
The effects of P. aquilinum treatment on cellular immune responses among mice that were subjected to Regimen 1C are shown in . In order to verify this response the DTH technique was used. The swelling measured was smaller in the P10 and P30 groups compared with the control group (p = 0.021, one-way ANOVA followed by Dunnett’s test).
Figure 5. Effects of P. aquilinum treatment on cellular immune response. Measurement of footpad swelling induced by DTH response in mice immunized and challenged with SRBC after treatment with 1 (P1), 10 (P10) or 30 (P30) g/kg BW of P. aquilinum and supplementation with B1 vitamin in water ( 10 mg/ml) for 14 days. P. aquilinum treatment reduced DTH response in the P10 and P30 groups compared with the control group (p = 0.021, one-way ANOVA followed by Dunnett’s test). Data are expressed as the mean ± SD (n = 9).
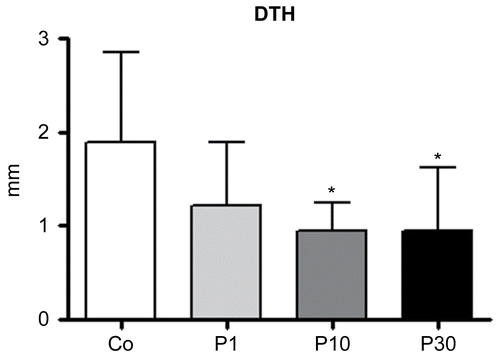
Effects of P. aquilinum treatment on splenocyte proliferation
Splenocyte proliferation was induced with PHA and LPS, causing T- and B-lymphocyte proliferation, respectively (Suni et al., Citation2005; Janeway et al., Citation2007). The proliferation percentages for these cells remained unaltered in P. aquilinum-treated mice compared with control mice (data not shown). The treatment schedule used in this experiment is shown in .
Effects of P. aquilinum treatment on peritoneal macrophage oxidative burst and phagocytosis
Peritoneal macrophage activity (assessed by oxidative burst and phagocytosis analyses) by cells obtained from those mice that were subjected to Regimen 1A. Peritoneal macrophage activity was unaltered in P. aquilinum-treated mice compared with control mice (data not shown).
Effects of P. aquilinum treatment on NK cell cytotoxicity
The effect of P. aquilinum treatment on NK cell cytotoxic activity using those cells isolated from mice that were subjected to Regimen 1A is shown in . In the present study, NK cell cytotoxicity was measured using effector cells isolated from the spleen and YAC-1 cells as a target. The results showed that NK cell cytotoxicity was diminished in P. aquilinum-treated mice (P30) compared with control mice (p = 0.026, Kruskal–Wallis test followed by Dunn’s test).
Figure 6. Effects of P. aquilinum treatment on NK cells cytotoxicity. YAC-1 cells were used as a target and effector cells (NK cells) were isolated from the spleens of mice treated with 1 (P1), 10 (P10) or 30 (P30) g/kg BW of P. aquilinum and supplemented with B1 vitamin in water ( 10 mg/ml) for 14 days. P. aquilinum-treatment reduced NK cells cytotoxicity in the P30 group compared with the control group (p = 0.026, Kruskal-Wallis test followed by Dunn’s test). Data are expressed as the mean ± SD (n = 5).
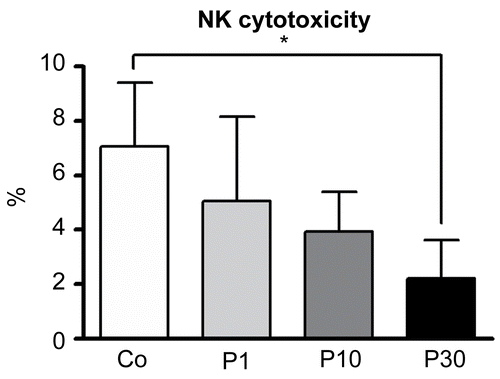
Effects of P. aquilinum treatment on DC cells and NK cells during TH1 priming
The effects of P. aquilinum treatment on DC cell maturation and IL-12 production by cells recovered from mice that were subjected to Regimen 1D are shown in and , respectively. The effects of P. aquilinum treatment on NK cell activation and IFNγ production are shown in and , respectively. In the present study, DC and NK cells were analyzed during TH1 priming using cells isolated from the spleen and Tetanus Toxoid as the challenge. The results showed that DC cell maturation (CD11c+ CD83+) and IL-12 production were unaltered in the P. aquilinum-treated mice (P309) compared with control mice. However, the results for NK cells showed that NK cell numbers (NK1.1+ NKG2D+) (p = 0.015, two-tailed Mann–Whitney test) and IFNγ production were diminished (p = 0.023, two-tailed Mann–Whitney test) in the P. aquilinum-treated mice (P30) compared with control mice.
Figure 7. Effects of P. aquilinum treatment on NK cells during TH1 priming. Evaluation of NK cells isolated from the spleens of mice immunized and challenged with TT after treatment with 30 (P30′) g/kg BW of P. aquilinum and supplementation with B1 vitamin in water ( 10 mg/ml) for 30 days. The percentage of total events acquired (20000) are indicated in the graphs. A. P. aquilinum-treatment reduced NK cell numbers (p = 0.015, two-tailed Mann–Whitney test). B. P. aquilinum-treatment diminished IFNγ production by NK cells (p = 0.023, two-tailed Mann–Whitney test). Data are expressed as the mean ± SD (n = 10).
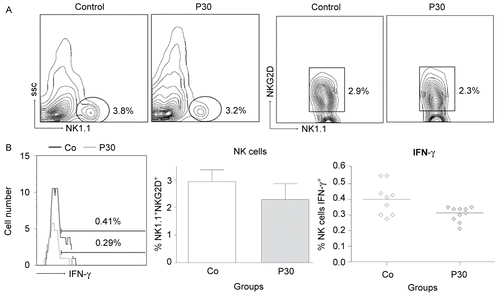
Figure 8. Effects of P. aquilinum treatment on DC cells during TH1 priming. Evaluation of DC cells isolated from the spleens of mice immunized and challenged with TT after treatment with 30 (P30′) g/kg BW of P. aquilinum and supplementation with B1 vitamin in water (10 mg/ml) for 30 days. The percentage of total events acquired (20,000) are indicated in the graphs. A. P. aquilinum-treatment did not alter DC cell maturation. B. P. aquilinum-treatment did not affect IL-12 production by DC cell. Data are expressed as the mean ± SD (n = 10).
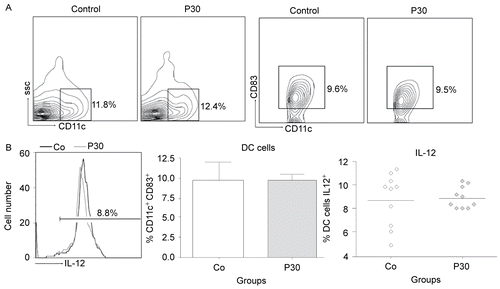
Effects of P. aquilinum treatment on antigen-specific T-lymphocyte expansion
The effect of P. aquilinum treatment on antigen-specific T-lymphocyte expansion by cells recovered from mice that were subjected to Regimen 1D is shown in . The results showed that antigen-specific T-lymphocyte expansion was decreased (p = 0.004, two-tailed Mann–Whitney test) in the P. aquilinum-treated mice (P30’) compared with control mice.
Figure 9. Effects of P. aquilinum treatment on antigen-specific T-lymphocyte expansion. Evaluation of lymphocytes isolated from the spleens of mice immunized and challenged with TT after treatment with 30 (P30′) g/kg BW of P. aquilinum and supplementation with B1 vitamin in water ( 10 mg/ml) for 30 days. P. aquilinum-treatment diminished antigen-specific T-lymphocyte expansion (p = 0.004, two-tailed Mann Whitney test). Data are expressed as the mean ± SD (n = 10).
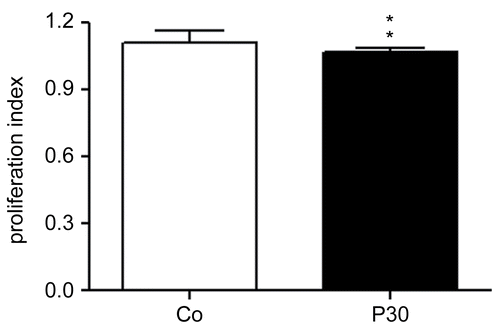
Discussion
Studies have consistently shown that the association of chronic exposure to bracken fern and bovine papilloma virus (BPV) infection induces the development of carcinomas in cattle. In fact, Souto et al. (Citation2006) necropsied bovines presenting upper alimentary tract (UAT) neoplasias linked to the spontaneous ingestion of bracken fern and observed that 29 out of 30 bovine evaluated presented papillomas in the UAT. In addition, in 16 of these bovine, malignant transformation of the papillomas into squamous-cell carcinomas had occurred, leading the authors to suggest that these alterations were not only dependent on interrelations between the virus and mutagenicity produced by Pteridium aquilinum, but also due to a possible immunosuppressor effect on the immune response against viral infection.
In this context, the P. aquilinum immunotoxic effects could affect not only immune response against viral infection but cancer immune surveillance. Cancer immune surveillance is considered to be an important host protection process for inhibiting carcinogenesis and maintaining cellular homeostasis. In the interaction of host and tumor cells, three essential phases have been proposed: elimination, equilibrium and escape, which are termed as cancer immunoediting (Kim et al., Citation2007). Thus, an immunosuppressive environment induced by P. aquilinum could contribute to the escape phase of tumor cells. Consequently, studies that investigate the immunomodulatory effects of P. aquilinum are important for providing a clearer understanding of the relationship between its carcinogenic effects and its immunotoxic effects.
For immunotoxicity studies, a variety of assays have been described in the literature that can be used as tools, such as histological evaluation of lymphoid organs and immune function tests on innate and acquired immune responses (Descotes, Citation2006; De Jong and Van Loveren, Citation2007). The assays used in the present study are described in Tier I and II for detecting immune alterations and were validated for sensitivity and reproducibility for immunotoxicity evaluation in mice (Luster et al., Citation1988). The present study is the first report that sought to investigate the immunotoxicity of P. aquilinum.
In a histopathological study of bovines with acute hemorrhagic syndrome caused by natural intoxication with P. aquilinum, a reduction in the white pulp area of the spleen was described (Tokarnia et al. Citation1967). In the present study, the same pattern of spleen alterations was verified in mice treated with the highest dose of P. aquilinum (see ). This result was consistent with the idea that bracken fern presents immunosuppressive effects, but at the time it was not possible to confirm our suppositions regarding a reduction in lymphocyte population. Thus, spleen lymphocyte phenotyping of P. aquilinum-treated mice was performed in order to more clearly characterize a reduction in the white pulp area of the spleen. Nevertheless, the results showed no alteration in the proportion of T-helper (CD4+), T-cytotoxic (CD8+) and B-lymphocytes (data not shown). In brief, the results revealed an equivalent reduction in all the spleen lymphocyte populations assessed.
In relation to humoral immunity, it is well known that it is mediated by B-lymphocytes, which synthesize and secrete antibodies into the bloodstream that show specificity against the foreign substance (Abbas et al., Citation2007). In the present work, this response was assessed by the plaque forming cell (PFC) assay, which reveals the capacity of B-lymphocytes to synthesize antibodies against SRBC, and hemagglutination titers, to measure the level of serum antibody specific for SRBC. However, no changes were observed in either analysis, indicating that P. aquilinum exposure does not act on humoral response (see ).
Cellular immunity is mediated by T-lymphocytes and is the principal pattern of immunological response to a variety of intracellular microbiological agents, such as viruses, fungi, protozoa, and parasites (Coico et al., Citation2003). To verify this response in mice submitted to P. aquilinum treatment, a delayed-type hypersensitivity (DTH) response was used. The major events leading to this response involved three steps: (1) the activation of antigen-specific inflammatory T-helper 1 (TH1) cells through interaction with dendritic cells (DC) in a previously sensitized individual; (2) the elaboration of pro-inflammatory cytokines by antigen-specific TH1 cells; and (3) the recruitment and activation of antigen-nonspecific inflammatory leukocytes, mainly macrophages (Kobayashi et al., Citation2001).
In this study, the two groups of mice treated with the highest doses of P. aquilinum showed a reduction in DTH response (see ), demonstrating for the first time that P. aquilinum diminishes cellular immune response. Considering that the cellular immune response is the principal mechanism for controlling intracellular microbiological agents, like viruses (Coico et al., Citation2003), it is feasible to suppose that bracken fern could contribute to the malignant transformation of papillomas. In addition, the fact that P. aquilinum promoted a reduction in cellular immune response supports the possibility that humans and animals exposed to P. aquilinum are more susceptible to infections.
In order to verify whether a reduction in DTH response was associated with the direct action of P. aquilinum on cells that mediate acquired immune responses, lymphocyte proliferation was induced with PHA and LPS, which are known to cause T- and B-lymphocyte proliferation, respectively (Janeway et al., Citation2007; Suni et al., Citation2005); however no differences were observed between control and P. aquilinum-treated mice. Therefore, it was hypothesized that the effect of P. aquilinum targets innate response cells that also participate in a DTH response. For instance, NK cells that contribute to the activation of antigen-specific inflammatory TH1 cells through interaction with DC and macrophages as effector cells that are recruited and activated (Kobayashi et al., Citation2001). In order to clarify this supposition, macrophages and NK cell activities were assessed.
Macrophages are phagocytes and their major function is the removal of bacteria and parasites from the body (Abbas et al., Citation2007). In the present study, macrophage activity was assessed by oxidative burst and phagocytosis activity. No differences between mice from the control and P. aquilinum groups were noted in either analysis, suggesting that the decrease in DTH response observed in P. aquilinum treated-mice was not due to a reduction in macrophage activity.
NK cells are large granular lymphocytes that are able to lyse certain virus-infected cells and tumor cells prior to stimulation (Abbas et al., Citation2007). Additionally, NK cells secrete IFNγ that acts on DC maturation and contributes to DC-mediated TH1 cell polarization (Kapsenberg, Citation2003; Corthay, Citation2006), as well as acting directly on T-lymphocytes, which also assists TH1 priming (Martin-Fontecha et al., Citation2004; Vivier et al., Citation2008). In this study, NK cell (effector cell) cytotoxicity was measured using the mouse lymphoma cell line YAC-1 as a target, while the effector cells used were those isolated from the spleen. The data demonstrated a decrease in NK cell cytotoxicity in P. aquilinum-treated mice (see ). We hypothesized that this effect on NK cell activity could be linked to the diminished DTH response in P. aquilinum-treated mice and that this occurred due to its action on DC maturation and on T-lymphocytes and, consequently, to its effect on DC-mediated TH1 cell polarization. Therefore, DC and NK cells isolated from the spleen were evaluated in order to clarify the effects of P. aquilinum on these cells during activation of antigen-specific TH1 cells; moreover, antigen-specific T-ymphocyte expansion was evaluated. In this study, tetanus toxoid was used to challenge splenocytes in vitro, the same antigen injected in mice for immunization. The results demonstrated a reduction in NK cell numbers and a decrease in IFNγ production by NK cells during TH1 priming in P. aquilinum-treated mice (see ). In addition, a reduction in antigen-specific T-lymphocyte expansion was observed (see ). Thus, we were able to conclude that the reduction in DTH response in P. aquilinum-treated mice is caused by these effects on NK cell activity, which disrupt its action on T-lymphocytes, while DC maturation and IL-12 production remain unaffected. We also theorized that this reduction in activities (cytotoxicity and IFNγ production), in NK cells the only cell able to lyse tumor cells prior to stimulation-could be directly involved in the development of cancer in humans and animals that feed on bracken fern.
In summary, P. aquilinum presents effects on cellular immune response and NK cell cytotoxicity, which are components of acquired and innate immune responses, respectively; however, not all types of acquired and innate responses were modified by the action of bracken fern, as demonstrated by analyses of both humoral response and macrophage activity. Taken together, these results showed, for the first time, the immunosuppressive effect of bracken fern in mice.
Acknowledgments
The authors would like to thank Ms. Beatriz D. Caniceiro, Ms. Domenica P. Mariano-Souza, Mr. Alison Ribeiro and Mr. Ricardo M. Akinaga for their collaboration, Dr. Cristiane M. Gaitani and Dr. Marcos J. Salvador for the quercetin HPLC analysis and Dr. Paulo C. Maiorka for his assistance with the histopathological analyses. Andreia O. Latorre was supported by a fellowship from CAPES and FAPESP (Proc. 07/50313-4), Brazil.
Declaration of interest: The authors report no conflicts of interest. The authors alone are responsible for the content and writing of the paper.
References
- Abbas, A. K., Litchman, A. H., and Pober, J. S. (Eds.). 2007. Cellular and Molecular Immunology, Sixth Edition. London: W. B. Saunders Company.
- Abnet, C. C. 2007. Carcinogenic food contaminants. Cancer Invest. 25:189–196.
- Alonso-Amelot, M. E., Castillo, U., Smith, B. L., and Lauren, D. R. 1996. Bracken ptaquiloside in milk. Nature 382:587.
- Alonso-Amelot, M. E., 1997. The link between bracken fern and stomach cancer: Milk. Nutrition 13:694–696.
- Alonso-Amelot, M. E., 1999. Bracken fern, animal health and human health. Rev. Faculd. de Agron. (LUZ) 16:528–547.
- Alonso-Amelot, M. E., Avendaño, M. 2002. Human carcinogenesis and bracken fern: A review of the evidence. Curr. Med. Chem. 9:675–686.
- ACS (American Cancer Society). 2007. Quercitin (3,3’,4’,5,7-pentahydroxyflavone). Information located at http://www.cancer.org/docroot/ETO/content/ETO_5_3x_Quercetin.asp.
- Balcos, L. G., Borzacchiello, G., Russo, V., Popescu, O., Roperto, S., and Roperto, F. 2008. Association of bovine papilloma virus Type-2 and urinary bladder tumors in cattle from Roman. Res. Vet. Sci. (in press).
- Borzacchiello, G., Ambrosio, V., Roperto, S., Poggiali, F., Tsirimonakis, E., Venuti, A., Campo, M. S., and Roperto, F. 2003. Bovine papilloma virus Type 4 in esophageal papillomas of cattle from the south of Italy. J. Comp. Pathol. 128:203–206.
- Campo, M. S., 1997. Bovine papilloma virus and cancer. Vet. J. 154:175–188.
- Campo, M. S., Jarrett, W. F., Barron, R., O’Neil, B. W., and Smith, K. T. 1992. Association of bovine papilloma virus Type 2 and bracken fern with bladder cancer in cattle. Cancer Res. 52:6898–6904.
- Caniceiro, B. D., Latorre, A. O., and Górniak, S. L. 2008. Effects of chronic administration of methylprednisolone in murine lymphocytes. In: Proceedings of the 16th SIICUSP International Symposium of Scientific Initiation, Ribeirão Preto, Brazil, November 2008.
- Carvalho, T., Pinto, C., and Peleteiro, M. C. 2006. Urinary bladder lesions in bovine enzootic haematuria. J. Comp. Pathol. 134:336–346.
- Coico, R., Sunshine, G., and Benjamini, E. (Eds.). 2003. Immunology: A Short Course. New York: Wiley & Sons.
- Corthay, A., 2006. A three-cell model for activation of naïve T helper cells. Scand. J. Immunol. 64:93–96.
- De Jong, W. H., and van Loveren, H., 2007. Screening of xenobiotics for direct immunotoxicity in an animal study. Methods 41:3–8.
- Descotes, J., 2006. Methods of evaluating immunotoxicity. Exp. Opin. Drug Metab. Toxicol. 2:249–259.
- Fenwick, G. R., 1988. Bracken (Pteridium aquilinum)—toxic effects and toxic constituents. J. Sci. Food Agric. 46:147–173.
- Harwood, M., Danielewska-Nikiel, B., Borzelleca, J. F., Flamm, G. W., Williams, G. M., and Lines, T. C. 2007. A critical review of the data related to the safety of quercetin and lack of evidence of in vivo toxicity, including lack of genotoxic/carcinogenic properties. Food Chem. Toxicol. 45:2179–2205.
- Hirono, I., Ogino, H., Fujimoto, M., Yamada, K., Yoshida, Y., Ikagawa, M., and Okumura, M. 1987. Induction of tumors in ACI rats given a diet containing ptaquiloside, a bracken carcinogen. J. Natl. Cancer Inst. 79:1143–1149.
- Kapsenberg, M. L., 2003. Dendritic cell control of pathogen-driven T-cell polarization. Nat. Rev. Immunol. 3:984–993.
- Kim, R., Emi, M., and Tanabe, K. 2007. Cancer immunoediting from immune surveillance to immune escape. Immunology 121:1–14.
- Kobayashi, K., Kaneda, K., and Kasama, T. 2001. Immunopathogenesis of delayed-type hypersensitivity. Microscopy Res. Tech. 53:241–245.
- Janeway, C. A., Travers, P., Walport, M., and Capra, J. D. (Eds.) 2007. Immunobiology. The Immune System in Health and Disease, 7th Edition. New York: Garland Publishing.
- Liu, H., and Wang, Z. 2005. Effects of social isolation stress on immune response and survival time of mouse with liver cancer. World J. Gastroenterol. 11:5902–5904.
- Luster, M. I., Munson, A. E., Thomas, P. T., Holsapple, M. P., Fenters, J. D., White, K. L., Jr., Lauer, L. D., Germolec, D. R., Rosenthal, G. J., and Dean, J. H. 1988. Methods evaluation. Development of a testing battery to assess chemical-induced immunotoxicity: National Toxicology Program’s Guidelines for immunotoxicity evaluation in mice. Fundam. Appl. Toxicol. 10:2–19.
- Lyons, B. A., 2000. Analyzing cell division in vivo and in vitro using flow cytometric measurement of CFSE dye dilution. J. Immunol. Meth. 243:147–154.
- Marcusson-Stahl, M., and Cederbrant, K. 2003. A flow cytometric NK-cytotoxicity assay adapted for use in rat repeated dose toxicity studies. Toxicology 193:269–279.
- Martin-Fontecha, A., Thomsen, L. L., Brett, S., Gerard, C., Lipp, M., Lanzavecchia, A., and Sallusto, F. 2004. Induced recruitment of NK cells to lymph nodes provides IFN-γ for TH1 priming. Nat. Immunol. 12:1260–1265.
- Ngomuo, A. J., and Jones, R. S. 1996. Genotoxicity studies of quercetin and shikimate in vivo in the bone marrow of mice and gastric mucosal cells of rats. Vet. Hum. Toxicol. 38:176–180.
- Ojika, M., Wakamatsu, K., Niwa, H., and Yamada, K. 1987. Ptaquiloside, a potent carcinogen isolated from bracken fern Pteridium aquilinum var. Latiusculum: Structure elucidation based on chemical and spectral evidence, and reactions with amino acids, nucleosides, and nucleotides. Tetrahedron 43:5261–5274.
- Pamukcu, A. M., Olson, C., and Goksoy, S. K. 1967. Influence of papilloma vaccine on chronic bovine enzootic hematuria. Cancer Res. 27:2197–2200.
- Pamukcu, A. M., Yalciner, S., Hatcher, J. F., and Bryan, G. T. 1980. Quercetin, a rat intestinal and bladder carcinogen present in bracken fern (Pteridium aquilinum). Cancer Res. 40:3468–3472.
- Peixoto, P. V., Do Nascimento França, T., Barros, C. S., and Tokarnia, C. H. 2003. Histopathological aspects of bovine enzootic hematuria in Brazil. Pesq. Vet. Br. 23:65–81.
- Riet-Correa, F., Schild, A. L., Méndez, M. D., and Lemos, R. A. 2001. Diseases of Ruminants and Horses 2. Sao Paulo: Varela Publisher and Bookstore LTDA.
- Schacham, P., Philip, R. B., and Gowdey, C. W. 1970. Anti-hematopoietic and carcinogenic effects of bracken fern (Pteridium aquilinum) in rats. Am. J. Vet. Res. 31:191–197.
- Shahin, M., Smith, B. L., and Prakash, A. S. 1999. Bracken carcinogens in the human diet. Mutat. Res. 443:69–79.
- Souto, M. A., Kommers, G. D., Barros, C. S., Piazer, J. V., Rech, R. R., Riet-Correa, F., and Shild, A. L. 2006. Neoplasms of the bovine upper alimentary tract associated with spontaneous consumption of bracken fern (Pteridium aquilinum). Pesq. Vet. Br. 26:112–122.
- Sugimura, T. 2000. Nutrition and dietary carcinogens. Carcinogenesis 21:387–395.
- Suni, M. A., Maino, V. C., and Maecker, H. T. 2005. Ex vivo analysis of T-cell function. Curr. Opin. Immunol. 17:434–440.
- Taylor, J. A., 1990. The bracken problem: A global perspective. AIAS Occas. Publ. 40:3–19.
- Tokarnia, C. H., Döbereiner, J., and Canella, C. F. 1967. The acute poisoning occurrence by the bracken fern (Pteridium aquilinum [L.] Kuhn) in cattle in Brazil. Pesq. Agropec. Bras. 2:329–336.
- Tokarnia, C. H., Döbereiner, J., and Peixoto, P. V. (Eds.). 2000. Plants of radiomimetic action. In: Toxic Plants of Brazil. Rio de Janeiro: Editora Helianthus.
- Vivier, E., Tomasello, E., Baratin, M., Walzer, T., and Ugolini, S. 2008. Functions of natural killer cells. Nat. Immunol. 9:503–510.