Abstract
Here, we report the results of proteomic analysis of the mouse thymoma EL4 cell line exposed to bis(tri-n-butylin)oxide (TBTO), an immunotoxic organotin compound. The objective of the work was to examine whether TBTO affects the expression of proteins in this cell line and to compare the differentially expressed proteins with the corresponding mRNA expression data. The identified proteins were quantified using a label-free quantitative method based on counting the observed peptides as an index of protein abundance. The calculation of the ratio of peptides obtained from exposed and control samples allowed us to evaluate the effect of TBTO on protein expression and to compare these results to those obtained in gene expression profiling studies. Correlation of some of the differentially expressed proteins and their corresponding mRNAs was observed. The analysis of the protein ratios revealed that 12 proteins were significantly affected. These proteins included cytoskeleton proteins myosin-9, spectrin beta 2 and plectin 8. The first two proteins were down-regulated 3-fold, whereas the third was up-regulated 2-fold. Ras-related Rab1, a GTP binding protein and T-complex protein-1 subunit alpha, a chaperonin, were decreased 2- and 3.6-fold, respectively. The ribosomal S10 and eukaryotic translation factor (eIf4G1), which are involved in protein synthesis, were down-regulated 2.6- and 3.7-fold, respectively. Also, proteins involved in splicing of pre-mRNA and in transcription, splicing factor arginine/serine-rich 2 and chromodomain-helicase-DNA binding protein 4 (Chd4), were decreased 2.6- and 4.5 times, respectively. Nuclear RNA helicase II was reduced 2.8-fold. Finally, prothymosin-alpha (ProTα), an essential protein for cell proliferation, and a protein similar to ProTα, (with a molecular weight and a pI (3.54) comparable to that of ProTα) were also down-regulated 6-and 8-fold, respectively. We propose that the observed down-regulation of the expression level of ProTα in the TBTO-exposed cells could account for the previously reported anti-proliferative effect of TBTO.
Introduction
Bis (tri-n-butyltin)oxide (TBTO) is an organotin compound that has been used worldwide as a biocide in anti-fouling paints for ships and wood preservatives (van der Kerk, Citation1976; Schrantz, Citation1990). TBTO has been shown to be an endocrine-disrupting agent and to produce adverse effects in reproductive systems in aquatic organisms (Funahashi et al., Citation1980; Grote et al., Citation2004). Moreover, TBTO is known to persist in the environment with deleterious consequences to marine and aquatic ecosystems (Takashi et al., Citation1998). Human exposure to TBTO occurs mainly through the food chain by consuming fish products (Kannan et al., Citation1996). Probably, the most significant TBTO toxicity effect is immunotoxicity (Seinen and Penninks; Citation1979; Vos et al., Citation1984; Snoeij et al., Citation1987; Boyer, Citation1989). In rodents, for example, this organotin compound causes a marked thymus atrophy due to reduction in the number of cortical thymocytes and this leads to a reduced immune response (Snoeij et al., Citation1988; Bressa et al., Citation1991; De Wall et al., Citation1993, Citation1997), which is manifested through a suppressed: delayed-type hypersensitivity response; infection resistance; and, antibody response (Snoij et al., 1987; Boyer, Citation1989; Bressa et al., Citation1991; Schuurman et al., Citation1992). Further, TBTO can also affect non-specific immune functions such as macrophage (Vos et al., Citation1984) and natural killer (NK) cell activity (van Loveren et al., Citation1990; Vos et al., Citation1990; Alouch et al., Citation2006).
Although numerous studies have been performed on TBTO toxicity in different species as well as in vitro systems and the molecular mechanisms underlying its toxicity have been investigated, amongst others using DNA microarrays (Baken et al., Citation2006; Baken et al., Citation2007), details of its mechanism of toxicity still remain elusive. For instance, none of the proposed mechanisms based on indirect effects, such as metabolic disturbance, stress-induced glucocorticoid lymphocytolysis, etc., to explain the observed thymus involution has been considered satisfactory (Raffray and Cohen, Citation1993). Alternative mechanisms such as apoptosis (Raffray and Cohen, Citation1993) and the anti-proliferative effect of TBTO (Gennari et al; Citation1997; Vandebriel et al., Citation1999) have been suggested to account for the triorganotin-induced thymic involution, but, to date, the molecular events leading to these processes remain unclear (Raffray and Cohen, Citation1993; Gennari et al., Citation1997; Vandebriel et al., Citation1999; Baken et al., Citation2006).
Therefore, in order to get more insight into the mechanisms of TBTO toxicity we undertook proteomic analysis of the EL4 thymoma cell line exposed to TBTO and compared quantitatively the proteome of TBTO-exposed to non- exposed (control) cells. The EL4 cell line is known to possess an immature thymus cell phenotype (Tanaka et al., Citation1987) and has frequently been used as a model system for mouse thymocytes in mechanistic studies (Li et al., Citation2000; El-Darahali et al., Citation2005; Lee et al., Citation2008). Protein extracts obtained from both exposed and control EL4 cells were separated by one-dimensional sodium dodecyl sulfate polyacrylamide gel electrophoresis. The protein bands were excised, digested, and the obtained peptides separated by nanoflow liquid chromatography coupled to tandem mass spectrometry. The proteins were identified and quantified by using the observed peptides as an index of protein abundance. Peptide counting has been successfully applied for discovery of urinary biomarkers (Pang et al., 2000) and for monitoring protein expression changes in yeast (Gao et al., Citation2003). This peptide counting method was subsequently applied on large scale by Blondeau et al. (Citation2004). In addition, it has also been the aim of this work to compare the differentially expressed proteins with the corresponding mRNA data. The data presented in this study provide new insights into the mechanisms of TBTO toxicity.
Materials and methods
Chemicals
Sequencing-grade modified trypsin was obtained from Roche (Mannheim, Germany). DL-dithiothreitol, iodoacetamide and TBTO were purchased from Sigma. Ammonium bicarbonate was supplied from Fluka. ReproSil-Pur18-AQ3 resin was obtained from Dr. Maisch GmsH, (Ammerbuch, Germany) and C18 200A-AQ5 was from Phenomenex. The solvents ethanol absolute and acetonitrile (HPLC grade)were purchased from Bissolve. The gel for electrophoresis (10% NU-PAGE Bis-Tris-gel), Nu-PAGE SDS Running buffer (20X), Transfer buffer (20X), LDS (lithium dodecyl sulfate) sample buffer (4X) and Nu-PAGE Antioxidant and Reducing agent were obtained from Invitrogen Company. Anti-prothymosin-α rabbit polyclonal IgG (primary antibody) and goat anti-rabbit IgG-HRP were purchased from Santa Cruz Biotechnology. Hypond-P polyvinylidene difluoride (PVDF) membrane, ECL molecular weight markers and ECL detection reagents were obtained from Amersham Biosciences, UK.
Cell culture and treatment
EL4 cells were cultured in RPMI 1640 medium supplemented with 10% fetal calf serum (FCS) and containing 1% penicillin (10,000 U/ml) and streptomycin (10,000 μg/ml). A total of forty milliliters of cell suspension (1 × 106 cells /ml) was divided into two equal parts; half of the cell suspension ( 20 ml) was used for the TBTO exposure experiment and the other half for the control. Three independent replicate experiments were performed. A 1 mM TBTO stock solution was prepared in ethanol and 20 μl of this solution was added to one of the cell suspension samples so that the final concentration of TBTO was 1 μM, whereas 20 μl of ethanol without TBTO was added to the control cells. Subsequently, cells were incubated at 37°C for 6 hr. Cell viability was evaluated by trypan blue dye exclusion and ranged 85–90% in all experiments, which was in agreement with a previous report (Raffray and Cohen, Citation1991). The cells underwent apoptosis at concentrations of TBTO higher than 1 μM and incubation time longer than 6 hr. EL4 cells were less sensitive than reported for primary thymocytes to TBTO exposure (Baken et al., Citation2007).
Protein extraction
After 6 hr of incubation, the cell suspensions were centrifuged at 1200 rpm at 4°C for 10 min. The cell pellets were washed twice in 20 ml of phosphate-buffered saline (PBS) and centrifuged for 10 min. Each of the cell pellets (sample and control) was suspended in 2 ml of 100 mM Tris-HCl (pH 8) containing 5 mM EDTA and 1% SDS (sodium dodecyl sulfate). These cell suspensions were sonicated in 4 cycles of 10 sec, and each 5 sec sonication was followed by a 5 sec break. After sonication, the samples were centrifuged at 11,000 rpm for 20 min at 4°C. The supernatants containing the protein were then kept at −20°C until use. Protein concentrations were determined using the Bradford method (Bradford, Citation1976). To get appropriate amounts of protein for SDS-PAGE electrophoresis, aliquots of the samples were concentrated by SpeedVac.
SDS-PAGE of protein analysis
For the separation of the proteins by SDS-PAGE, samples were prepared using NU-PAGE LDS-containing sample buffer, reduced by adding dithiothreitol 50 mM (DTT; final concentration), and heated at 70°C for 10 min. Seventy μg of the exposed sample and the control were then loaded onto a 10% Nu-PAGE Bis-Tris-gel. After the gel had been run at a constant voltage of 160 V for 90 min, it was stained by using a Coomassie dye (SimplyBlue SafeStain, following the instructions of the Invitrogen Company). Briefly, the gel was rinsed three times with 100 ml deionized water for 5 min to remove SDS and salts. The gel was then stained with 20 ml of SimplyBlue SafeStain for 2 hr. After that, the gel was kept in deionized water for several hours (maximum; overnight). Lanes of test and control samples were cut horizontally into 15 bands each. Protein-containing slices were then excised from the gel, cut into small pieces, and each fraction transferred into 1.5 ml Eppendorf tubes.
In-gel digestion
Each fraction was destained twice with 150 μl of 50 mM NH4HCO3/50% ethanol for 25 min., followed by dehydration twice with 150 μl 100% ethanol. The fractions were reduced with 10 mM DTT and incubated at 37°C for 45 min. This was followed by alkylation with 55 mM iodoacetamide and incubation at 37°C in the dark for 45 min. Subsequently, the fractions were suspended in 100 μl 100% ethanol to dehydrate the gel pieces. After removal of the liquid, the fractions were air-dried for 15 min. The gel was then covered with 30 μl of trypsin solution (12.5 ng/μl) in 50 mM ammonium bicarbonate buffer. Thirty μl of 50 mM ammonium bicarbonate buffer was added to each fraction to ensure that the gel pieces are covered and the fractions were incubated at 37°C overnight. After 17 hr of incubation, additional 30 μl of 50 mM ammonium bicarbonate buffer was added to each fraction and incubated for two more hours. The supernatant of each fraction was collected into a clean Eppendorf tube and 25 μl of 50% ethanol containing 5% formic acid was added to each fraction, mixed and pooled it with the previous one. This latter step was repeated once. After that, the fractions were dried in a speed vac. The dried fractions were suspended in 60 μl 100% acetonitrile and dried by using a Speed Vac. Last, each fraction was dissolved in 20 μl 2% acetonitrile/10% formic acid solution. The samples were then kept at −20°C until use.
Nano-LC/MS/MS analysis
The digested fractions were analyzed by a nano-LC/MS/MS system consisting of an Agilent 1100 Series LC set up (vacuum degasser, autosampler and one high pressure-mixing binary pump without static mixer) coupled to a LCQ Deca Quadruple Ion Trap mass spectrometer (Thermo Finnigan, San Jose, CA) as described by Meiring, et al. (Citation2002). Briefly, 5 μl peptide solution was delivered to a trap column (Aqua C18 [Phenomenex]; l = 15 mm–100 μm ID, packed in-house) at 5 μl/min of 100% Solvent A (0.1 M acetic acid). After decreasing the flow to approximately 150 nl/min by a splitter, the peptides were transferred to the analytical column (Reprosil C18RP, Dr. Maisch GmbH (l = 20 cm, 50 μm ID, packed in- house) with a linear gradient from 0–100% Solvent B (0.1 M acetic acid in 80% acetonitrile) in 40 min. The 100% Solvent B was maintained for 5 min., followed by decreasing Solvent B to 0% in 0.1 min, and washing and re- equilibrating the system at 100% Solvent A for 10 min. The column eluent was sprayed directly into the ESI source of the mass spectrometer via a butt-connected nano-ESI emitter (New Objective). The LCQ operated in a positive ion mode and the peptides were fragmented in a data-dependent mode. A full MS scan was followed by tandem spectra of the three most intense precursor ions present in the MS scan. The capillary voltage was 1.9 kV and the capillary temperature was 200°C. The following settings were used: repeat count was set at 1, repeat duration was set at a 0.25, exclusion list size was set at 50 and the exclusion duration was set at 0.5 min.
Database searches
All MS/MS data were analyzed using SEQUEST engine (Bioworks version 3.3) (Yates et al., Citation1995) against the IPI mouse v 3.18 fasta database. The searches were performed allowing for fixed modification on methionine (oxidation) and cysteine (carbamiodomethylation) residues. The peptide and fragment mass tolerance were set at 1.4 and 1 Da, respectively. Trypsin was used for protein digestion and two missed cleavages were allowed. For protein identification acceptance, the following filtering criteria were used: a Delta Cn (ΔCn) was set to 0.1 SEQUEST Xcorr vs. charge state (Xcorr = 2 for z = 1, Xcorr = 1.5 for z = 2 and 3.3 for z = 3 and a peptide probability score < 0.01. Protein identification was also based on at least two different peptides. Spectra that did not satisfy these requirements were not considered. The total of identified proteins was obtained by combining the identified proteins of both the samples and the controls.
Western blot analysis
Equal amounts of protein extracts obtained from both TBTO-exposed and control cells were subjected to 10% SDS-PAGE separation (see SDS-page protein analysis), followed by electrotransfer of the proteins Hybond-P polyvinylidene difluoride (PVDF) membrane at 25 V for 90 min. The transfer buffer contained 0.1% Nu-page Antioxidant. The membrane was blocked with a solution of 5% non-fat milk powder in PBS, (containing 0.2% Tween) and probed with rabbit anti-prothymosin-α antibody (1 μg/ml) in 5% non-fat milk powder/PBS buffer containing 0.2% Tween. Detection was performed by using goat anti-rabbit IgG conjugated with horseradish peroxidase and ECL Western blotting detection reagents (Amersham Pharmacia).
RNA isolation
After exposure of EL4 cells to TBTO for 6 hr, total RNA of both TBTO-treated and the control cells were isolated as described before (Baken et al., Citation2007) and analyzed using Agilent 44K mouse oligo arrays according to Heneweer et al. (Citation2007).
Results
Separation of proteins by 1-D-SDS-PAGE
After the separation of the proteins extracted from both the TBTO-exposed and the control EL4 cells by one- dimensional SDS-PAGE electrophoresis, the protein bands of both treated sample and control were divided into 15 fractions and digested with trypsin (data not shown). Subsequently, the peptides obtained from this proteolysis were subjected to nano-LC/MS/MS analysis (). Protein concentration was found to be 20% (mean value) less in the TBTO-exposed samples compared to that of the controls (data not reported), suggesting that TBTO inhibits protein synthesis in agreement with an earlier report (Raffray et al., 1993)
Protein identification
The combination of the results of TBTO exposed and the control samples led to the identification of 822 proteins from the EL4 cells. A complete list of the proteins identified is available in Supplementary Table (S1). As reported in , 450 of the identified proteins were found in both TBTO-exposed and the control samples. The proteins identified in at least of one the exposed triplicates but not in the controls numbered 105 (), whereas those detected in at least one of the controls (but not in any of the treated samples) were 267 (). To evaluate whether TBTO affects the protein expression level, the relative protein abundance in both the exposed and the control cells was determined, using a free-label quantitative method based on counting the observed peptide hits. It has been previously shown that there is a correlation between the number of peptides sequenced and the amount of protein present in a sample (Rappsilber et al., Citation2002).
Figure 2. Number of identified proteins common for control and TBTO-exposed sample, as well as the number proteins detected in either the control or TBTO-exposed cells.
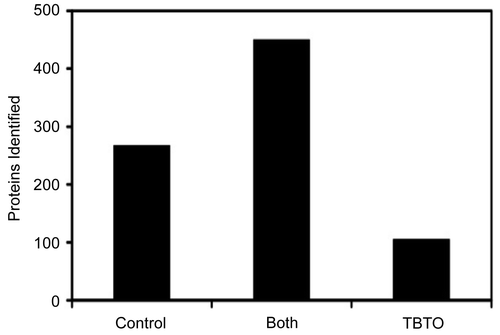
Recently, it has been shown that this correlation between protein abundance and peptide hit is logarithmic (Gao et al., Citation2006). This label-free peptide hit technique has been successfully applied for discovery of urinary biomarkers (Pang et al., Citation2002) and for monitoring protein expression changes in yeast (Gao et al., Citation2003). Thus, we calculated the ratio of the peptides of the TBTO-treated cells to the control cells, which reflect the proteins present in the cells. If a protein was absent, for example, in a control run while present in the corresponding exposed sample, a value of one peptide was assumed for that protein in the control. The same assumption was made if a given a protein was present in a control but was absent in the corresponding exposed sample.
Proteins altered by the exposure of EL4 cell line to TBTO
To attribute a specific effect of TBTO on protein expression levels certain criteria were applied. First, a change of 1.5-fold or greater in the protein expression level was considered relevant. Second, this effect on the protein had to be consistent in all three independent experiments. Thus, a protein affected in two experiments but not in the third experiment was considered unaltered. As shown in , we found that 12 proteins fulfilled these criteria and were considered affected by the exposure of EL4 cells to TBTO. Some of these proteins are involved in cell architecture and stability such as myosin-9, spectrin-beta 2; and plectin-8. The former two proteins were down regulated 3-fold (mean value), whereas the third was up regulated 2-fold. Proteins involved in protein synthesis were also affected. Both ribosomal protein S10 and isoform 1 of eukaryotic translation initiation factor 4 gamma (eIf4G1) were down regulated 2.6- and 3.7-fold, respectively. Ras-related Rab-1, a protein that belongs to a small GTP-binding family of proteins and which is involved in cell protein trafficking and endocytosis (Nuoffer et al., Citation1994; Pind et al., Citation1994; Filipeanu et al., Citation2004) was increased 2-fold.
Table 1. Proteins altered upon exposure to TBTO.
Furthermore, TBTO altered proteins involved in splicing pre-mRNA and in transcriptional machinery. The splicing factor arginine/serine-rich-2 was decreased 2.6-fold, whereas chromodomain-helicase-DNA-binding protein 4 was down-regulated 4.5-fold. The latter is a part of a protein complex known as the nucleosome remodeling and deacetylating (NRD) complex (Tong et al., Citation1998; Thompson et al., Citation2003). Nucleolar RNA helicase II, a nucleolar protein implicated in the biogenesis of ribosomal RNA (Yang et al., Citation2003), was reduced 2.8-fold. Another protein that was altered by the exposure of EL4 cells to TBTO is the chaperonin T-complex protein-1 subunit alpha A, which is localized in the centromere and which is believed to be important for microtubule growth (Yoo et al., Citation2001). This TCP-1 was down-regulated 3.6 times. Finally, prothymosin-alpha (ProTα), a protein that is thought to play an important role in the regulation of cell proliferation, was also affected by TBTO. This protein was down regulated 6-fold (). Interestingly, a small acidic protein (predicted: similar to ProTα, LOC622152), which shares 48.2% similarity in sequence and both a pI (3.54) and molecular weight that is comparable to ProTα (), was identified in this study.
Table 2. TBTO-altered protein-fold changes compared to the corresponding mRNA ratio.
The sequence of this protein has been predicted by computational analysis of mouse genomic sequence (Griffiths-Jones et al., Citation2006) but to the best of our knowledge no experimental evidence for its identification from mouse tissues has been reported. This protein has eight amino acids less than ProTα and has the following primary structure (Griffiths-Jones et al., Citation2006): Met-Ser-Glu-Ala-Ala-Val-Asp-Thr-Ser-Ser-Glu-Glu-Lys-Lys-Glu-Val-Val-Glu-Glu-Ala-Glu-Ser-Gly-Arg-Asp-Ala-Pro-Ala-Asn-Ala-Asn-Ala-Gln-Asn-Glu-Lys-Asn-Gly-Glu-Gln-Glu-Ala-Asp-Asn-Glu-Val-Asp-Glu-Glu-Glu-Glu-Glu-Gly-Gly-Gly-Glu-Glu-Glu-Glu-Glu-Gly-Asp-Gly-Glu-Glu-Glu-Asp-Gly-Asp-Asp-Asp-Glu-Glu-Ala-Glu-Ala-Pro-Thr-Gly-Lys-Arg-Val-Ala-Glu-Asn-Asp-Glu-Asp-Asp-Asp-Val-Asp-Thr-Lys-Lys-Gln-Lys-Thr-Glu-Glu-Asp-Ala-OH. Like ProTα (Haritos et al., 1987), this protein does not contain aromatic amino acids, but is rich (43%) in acidic amino acids (glutamic and aspartic acids). This protein was also down-regulated 8-fold.
To further substantiate the observed down-regulation of prothymosin α in the TBTO-exposed cells compared to the control cells, we did Western blot on extracts obtained from both TBTO-treated and non-treated samples (). The Western blot analysis revealed a band present in both samples. The intensity of the band in the TBTO-treated sample was lower than that in the control though the molecular weight of the observed band (circa 31 kDa) was greater than the predicted molecular weight of prothymosin α, suggesting the formation of a complex between ProTα with another protein that consequently retarded its migration (). A specific interaction of ProTα with the basic histone H1 in vitro was previously reported (Papamarcaki and Tsolas, Citation1994; Karetsou et al., Citation1998). Alternatively, the observed band may be an oligomeric form of ProTα, since previous studies showed that proTα exhibits anomalous behavior on SDS-electrophoresis, forming oligomers even under denaturing and reducing conditions (Palvimo and Linnala-Kankkunen, Citation1990; Codero et al., Citation1992).
Figure 3. Protein extracts of TBTO-treated and non-treated cells (70 μg each; obtained as described in Materials and Methods) were separated by 10% SDS-PAGE, transferred onto Hybond-P PVDF membrane, and probed with rabbit anti-prothymosin-α polyclonal IgG. Detection was performed using goat anti-rabbit IgG conjugated with horseradish peroxidase and ECL Western blotting detection reagents. The numbers on the left indicate the molecular weights of the ECL markers.
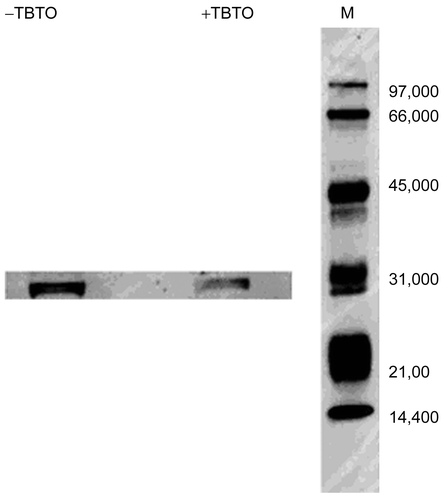
In addition, the ratios determined for the differentially-expressed proteins were compared with mRNA data obtained from DNA microarray analysis of BTO-treated EL4 cells. There was an agreement between the changes of most of the differentially expressed proteins and their corresponding mRNA levels (). For instance, of the 12 proteins altered by TBTO, the mRNA levels of only Plectin and Rab1 were inconsistent with the observed up-regulation of the corresponding proteins (). The observed down-regulation of the other altered proteins, excluding the protein which had no gene annotation but was predicted similar to ProTα, was in accord with the decrease of their corresponding mRNA levels (). Thus, it seems that the down-regulation of these proteins by TBTO reflects the down-regulation of their corresponding mRNA levels.
Discussion
To our knowledge, this communication is the first report that has addressed the proteomic analysis of the EL4 cell line exposed to TBTO, an immunotoxicant and environmental pollutant. Our results show that TBTO affected only 12 proteins and that the changes observed for these proteins were reproducible (). We cannot, however, exclude that other proteins might have been affected by TBTO which were either not detectable in our experimental conditions or have not fulfilled the required criteria. The altered proteins include cytoskeleton proteins such as myosin-9, spectrin-β, and plectin 8. The decrease in the relative abundance of both myosin-9 and spectrin β may be due to reduction in their expression level by TBTO, but can also be due to their degradation or both. It is known that TBTO causes oxidative stress in cells (Gennari et al., Citation2000) and therefore radicals could be formed which might degrade these proteins. This would be in agreement with previous studies in which both myosin (Aragno et al., Citation2006) and spectrin β (Beneke et al., Citation2005) bands were shown to decrease under oxidative stress conditions. This result is also consistent with previous observations that reported triorganotin-induced cytoskeleton modifications in rat thymocytes (Chow and Orrenius, Citation1994) and human neutrophils (Marinovich et al., Citation1990). In contrast, plectin 8, a versatile high molecular weight (>500,000) cytolinker, was up-regulated 2-fold.
Plectin plays a role not only as an anchorage protein of the structure of intermediate filaments (Ifs) (Svitkina et al., Citation1996), but it also acts as a scaffolding platform of proteins involved in cellular signaling (Osmanagic-Myers and Wiche, Citation2004). It was also demonstrated before that plectin is inducible and that it is involved in anti-proliferative pathways (Pálmer et al., Citation2003). Another protein that was affected by TBTO is T-complex protein-1 (), a chaperonin that is involved in microtubule growth (Tong et al., Citation1998). Because microtubules are important for cell cycle, the expression level of this protein could be critical for both mitosis and meiosis (Yoo et al., Citation2001). Earlier study associated the expression level of TCP-1 with the arrest of Down Syndrome (DS) neuron cells at the G2/M transition before the cells undergo apoptosis (Nagy, Citation1999). More recently, a decrease of TCP-1 level in Down syndrome parietal cortex was reported, and the Authors proposed that this reduction of the protein level may lead to arrest of cells at G2/M transition (Yoo et al., Citation2001). This would be in accord with a previous report in which organotin compounds have been shown to inhibit both spindle formation and microtubule assembly (Jensen et al., Citation1991).
Furthermore, TBTO down-regulated ribosomal S10 and Isoform 1 eukaryotic translation factor 4 gamma-1 (eIf4G1). Both proteins play an important role in protein translation. The ribosomal S10 has been shown to interact with initiation factor 3 at the beginning of the translation (Pei, Citation1999), whereas eIf4G is known to form a crucial link between ribosome and mRNA thereby controlling the translational process (Hentze, Citation1997). The decrease of these proteins in the exposed cells suggests that TBTO could affect the rate of protein synthesis at the translation level. This is in accord with the observed decrease of protein yield in the TBTO-exposed cells. TBTO also down-regulated RNA helicase II, a nucleolar protein that has been implicated in the biogenesis of ribosomal RNA (rRNA) (Yang et al., Citation2003). Evidence has been earlier presented for the depletion of 18 and 28 S rRNAs when nucleolar RNA helicase II in Xenopus oocyte was down-regulated (Yang et al., Citation2003). Moreover, the observed down- regulation of both the splicing factor arginine/serine- rich 2 and the chromodomain-helicase-DNA binding protein 4 (Chd4) () that which are involved in the splicing of pre-mRNA and the deacetylation of histones in the chromatin structure, respectively, indicates that TBTO may affect both the mRNA splicing and the transcription processes. It is known that changes in the chromatin structure, for example acetylation and deacetylation of nucleosomes, could modify the accessibility of the DNA to transcriptional machinery (Thompson et al., Citation2003). While the acetylation of lysine residues on the histones decreases the affinity between DNA and histones allowing transcription to occur, the deacetylation reaction catalyzed by proteins like chromodomain-helicase-DNA binding protein 4 increases the affinity between the histones and DNA, inhibiting the transcription process (Thompson et al., Citation2003).
Prothymosin-alpha (ProTα), an essential protein associated with cell proliferation (Sburlati et al., Citation1991; Evstafieva et al., Citation2000), was also significantly down-regulated () in the cell line exposed to TBTO. A down-regulation of the ProTα gene by TBTO was also observed in rat primary thymocytes (Baken, personal communication). The Western blot analysis result lends support to the observed down-regulation of ProTα in the TBTO-exposed cells () though further study is required to shed light on whether ProTα selectively binds with another protein, or forms an oligomer on SDS-PAGE electrophoresis as previously reported (Palvimo and Linnala-Kankkunen, Citation1990; Codero et al., Citation1992) This small nuclear protein (Mr = 12122 Da) is found in all mammalian tissues (Evstafieva et al., Citation2000).
Several lines of evidence (Sburlati et al., Citation1991; Evstafieva et al., Citation2000) support that ProTα is involved in cell proliferation: First, ProTα is abundant in proliferating tissues, especially in cancer cells. Second, over-expression of the protein in HL-60 cells stimulated the cells to undergo cell division. Third, treatment of human myeloma cells with ProTα anti-sense oligonucleotides inhibited cell division. The down-regulation of ProTα reported in this study suggests a possible mechanism for the anti-proliferative effect of TBTO. This down-regulation of proTα may also play a role in the oxidative stress induced by TBTO since this protein has been shown to bind to Keap1, displacing Nrf2 from keap1 and therefore up-regulating Nrf2-mediated antioxidant gene expression (Karapetian et al., Citation2005).
While the over-expression of ProTα stimulates cell proliferation, this protein is also a negative regulator of apoptosis since it inhibits the formation of apoptosome complex and consequently blocks the caspase activation pathway (Barbini et al., Citation2006). The TBTO-induced down-regulation of ProTα presented here may explain the intriguing question regarding the mechanism of organotin-induced caspase activation reported in the literature (Stridh et al., Citation1999). Recent findings suggest that both the expression level of ProTα and its localization in the subcellular compartments may be important in determining its role in cell activities, i.e., proliferation versus apoptosis (Barbini et al., Citation2006). Further, we identified a small acidic protein (Predicted: ProTα) with comparable molecular weight to ProTα and, which like ProTα, was also significantly down-regulated (). Although the function of this latter protein remains yet to be defined, due to similarity, in molecular weight, sequence and susceptibility to TBTO, with ProTα, this protein may also be involved in cell proliferation.
However, there is strong evidence that, in addition to cell proliferation, ProTα and other thymic peptides, including thymosin-α1, a proteolytic degradation product of ProTα (Ajit and Saki, 2002), exert immunomodulating functions. For instance, in vitro studies have shown that ProTα exerts immunostimulating effects on various cell types, including NK cells, T-lymphocytes, granulocytes, and monocytes (Cordero et al., Citation1990, Citation1992; Garbin et al., Citation1994; Wykretowicz et al., Citation1994; Gast et al., Citation1995). It was also shown that ProTα increased NK and LAK-cell cytotoxicity against various tumor cells and enhanced cytokine production (Eckert et al., Citation1995, Citation1997; Garbin et al., Citation1997; Grünberg et al., Citation1997). These conclusions are in line with the mechanism proposed in the present study in which TBTO, by down-regulating the expression level of ProTα, suppresses the immune response rather than stimulating it.
Finally, a correlation was found between the expression levels of most of the TBTO-affected proteins and their corresponding mRNA expression ratios (). The observed down-regulation for most of the proteins affected by TBTO is paralleled by a decrease in the level of their corresponding mRNA levels (). Discordance between protein and mRNA ratios was observed only for two of the affected proteins (Plectin 8 and Rab1a). Inconsistencies between protein and mRNA ratios have previously been reported in several studies (Li et al., Citation2003). Possible explanations for these inconsistencies have been suggested (Li et al., Citation2003 and the references therein].
In conclusion, we have shown that the exposure of EL4 cells to TBTO resulted in the down-regulation of proteins involved in cell stability, transcription, splicing of pre-mRNA, formation of ribosomal RNA, and protein translation. TBTO also down-regulated proteins associated with proliferation of cells and cell cycle. Together, our results show that TBTO could affect both the rates of transcription and protein translation. The down-regulation of ProTα observed in this study suggests a plausible molecular mechanism underlying the well documented anti-proliferative and apoptosis effects of TBTO.
Supplementary Material
Download MS Excel (201.5 KB)Acknowledgments
This work has been funded by the Netherlands Genomics Initiative Organization for Scientific Research (NWO) under OWB/FB/2001/34440. The authors thank Paul Schwillens for technical assistance.
Declaration of interest: The authors report no conflicts of interest. The authors alone are responsible for the content and writing of the paper.
References
- Ajit, S., and Paul, S. 2002. Involvement of mitogen-activated protein kinases in the signal transduction pathway of bone marrow-derived macrophage activation in response to in vitro treatment with thymosin-α1. Int. Immunopharmacol. 2:47–58.
- Aluoch, A. O., Odman-Ghazi, S. O., and Whalen, M. M. 2006. Alteration of an essential NK cell signaling pathway by low doses of tributyltin in human natural killer cells. Toxicology 224:229–237.
- Aragno, M., Mastrocola, R., Medana, C., Catalano, M.G., Vercellinatto, I., Danni, O., and Bocuzzi, G. 2006. Oxidative stress-dependent impairment of cardiac specific transcription factors in experimental diabetes. Endocrinology 147:5967–5974.
- Baken, K.A., Pennings, J.L.A., de Vries, A., Breit, T.M., van Steeg, H., and van Loveren, H. 2006. Gene expression profiling of bis(tri-n-butyltin)oxide (TBTO)-induced immunotoxicity in mice and rats. J. Immunotoxicol. 3:227–244.
- Baken, K. A., Arkusz, J., Pennings, J.L.A., Vandebriel, R.J., and van Loveren, H. 2007. In vitro immunotoxicity of bis(tri-n-butyltin)oxide (TBTO) studied by toxigenomics. Toxicology 237:35–48.
- Barbini, L., Gonzalez, R., Dominguez, F., and Vega, F. 2006. Apoptic and proliferating hepatocytes differ in prothymosin-α expression and cell localization. Mol. Cell. Biochem. 291:83–91.
- Beneke, R., Bihn, D., Hütler, M., and Leithäuser, R. M. 2005. Hemolysis caused by alterations of α- and β-spectrin after 10 to 35 min of severe exercise. Eur. J. Appl. Physiol. 95:307–312.
- Bressa, G., Hinton, R. H., Price, S. C., Isbir, M., Ahmed, R. S., and Grasso, P. 1991. Immunotoxicity of tri-n.butyltin oxide TBTO) and tri-n-butyltin chloride (TBTC) in the rat. J. Appl. Toxicol. 11:397–402.
- Blondeau, F., Ritter, B., Allaire, P., Wasiak, S., Girad, M., Hussain, N. K., Angers, A., Legendre-Guillemin, V., Roy, L., Boismenu, D., Kearney, R. E., Bell, A. W., Bergeron, J. M., and McPherson, P. S. 2004. Tandem MS analysis of brain clathrin-coated vesicles reveals their critical involvement in synaptic vesicle recycling. Proc. Nat. Acad. Sci. USA 101:3833–3838.
- Boyer I. J. 1989. Toxicity of dibutyl, tributyl and other organotins to humans and to experimental animals. Toxicology 55:253–298.
- Bradford, M. M. 1976. A rapid and sensitive method for the quantitation of microgram quantities of protein utilizing the principles of protein dye binding. Anal. Biochem. 72:248–254.
- Chow, S. C., and Orrenius, S. 1994. Rapid cytoskeleton modification in thymocytes induced by the immunotoxicant tributyltin. Toxicol. Appl. Pharmacol. 127:19–26.
- Cordero, O. J., Sarandeses, C. S., Lopez, J. L., and Nogueira, M. 1990. Phytohemagglutin-stimulated human T-cell: Prothymosin-α as an accessory signal. J. Biol Regul. Homeostatic Agents 2:7–12.
- Cordero, O. J., Sarandeses, C. S., Lopez, J. L., and Nogueira, M. 1992. Prothymosin-α enhances human natural killer cell cytotoxicity: Role in mediating signals for NK activity. Lymphokine Cytokine Res. 11:277–285.
- Cordero, O. J., Sarandeses, C. S., Lopez, J. L., and Nogueira, M. 1992. On the anomalous behavior on gel-filtration and SDS-electrophoresis of prothymosin-alpha. Biochem. Int. 28:1117–1124.
- De Waal, E.J., Schuurman, H. J., van Loveren, H., and Vos, J. G. 1997. Differential effects of 2,3,7,8-tetrachlorodibenzo-p-dioxin, bis(tri-n-butyltin)oxide, and cyclosporine on thymus histophysiology. Crit. Rev. Toxicol. 27:381–430.
- De Waal, E. J., Schuurman, H. J., van Loveren, H., and Vos, J. G. 1993. The cortical epithelium of the rat thymus after in vivo exposure to bis(tri-n-butyltin)oxide (TBTO). An (immuno) histological and ultrastructural study. Arch. Toxicol. 67:186–192.
- Eckert, K., Garbin, F., Maurer, H. R., Büttner, P., Garbe, C., and Czarnecki, J. 1995. Prothymosin-α modulates lymphokine-activated killer cell activity and IL-2 production by peripheral blood lymphocytes from melanoma patients in vitro. Int. J. Immunopharmacol. 17:555–561.
- Eckert, K., Grünberg, E., Immenschuh, P., Garbin, F., Kreuser, E. D., and Maurer, H. R. 1997. Interleukin-2-activated killer cell activity in colorectal tumor patients: Evaluation of in vitro effects by prothymosin Eckert, K α 1. Cancer Res. Clin. Oncol. 123:420–428.
- El-Darahali, A., Fawcett, H., Mader, J. S., Conrad, D. M., and Hoskin, D. W. 2005. Adenosine-induced apoptosis in EL-4 thymoma cells is caspase-independent and mediated through a non-classical adenosine receptor. Exp. Mol. Pathol. 79:249–258.
- Evstafieva, A. G., Belov, G. A., Kalkum, M., Chichkova, N. V., Bogdanov, A. A., Agol, V. I., and Vartapetian, A. B. 2000. Prothymosin-α fragmentation in apoptosis. FEBS Lett. 467:150–154.
- Filipeanu, C. M., Zhou, F., Claycomb, W. C., and Wu, G. 2004. Regulation of the cell surface expression and function of angiotensin II type 1 surface receptor by rab1a-mediated endoplasmic reticulum-to-Golgi transport in cardiac myocytes. J. Biol. Chem. 279:41077–41084.
- Funahashi, N., Iwasaki, I., and Ide, G. 1980. Effects of bis-(tri-n-butyltin)oxide on endocrine and lymphoid organs of male rats. Acta Pathol. Japan 30:955–966.
- Gao, J. I., Opiteck, G. J., Friedrichs, M. S., Dongre, A. R., and Hefta, S. A. 2003. Changes in the protein expression of yeast as a function of carbon source. J. Proteome Res. 2:643–649.
- Gao, J. I., Friedrichs, M. S., and Dongre, A. R. 2006. Guidelines for the routine application of the peptide of the peptide hits technique. J. Am. Soc. Mass Spec. 16:1231–1238.
- Garbin, F., Eckert, K., Büttner, P., Garbe, C., and Maurer, H. R. 1994. Prothymosin-α augments deficient anti-tumor activity of monocytes from melanoma patients in vitro. Anticancer Res. 14:2405–2412.
- Garbin, F., Eckert, K., Immenschuh, P., Kreuser, E. D., and Maurer, H. R. 1997. Prothymosin-α1 effects in vitro on the anti-tumor activity and cytokine production of blood monocytes from colorectal tumor patients. Int. J. Immunopharmacol. 19:323–332.
- Gast, K., Damaschun, H., Eckert, K., Schulze-Forster, K., Maurer, H. R., Müller-Frohne, M., Zirwer, D., Czarneccki, J., and Damaschun, G. 1995. Prothymosin-α: A biologically-active protein with random coil conformation. Biochemistry. 34:13211–13218.
- Griffiths-Jones, S., Grocock, R. J., van Dongen, S., Bateman, A., and Enright, A. J. 2006. miRBAse: MicroRNA sequences, targets, and gene nomenclature. Nucl. Acids Res. 34 (DATABASE ISSUE):D140–D144.
- Gennari, A., Potters, M., Seinen, W., and Pieters, R. 1997. Organotin-induced apoptosis as observed in vitro is not relevant for induction of thymus atrophy at anti-proliferative doses. Toxicol. Appl. Pharmacol. 147:259–266.
- Gennari, A., Viviani, B., Galli, C.L., Marinovich, M., Pieters, R., and Corsini, E. 2000. Organotins induce apoptosis by disturbing [Ca+2] and mitochondrial activity, causing oxidative stress and activation of caspases in rat thymocytes. Toxicol. Appl. Pharmacol. 169:185–190.
- Grote, K., Stahlschmidt, B., Talsness, C. E., Gericke, C., Appel, K. E., and Chahoud, I. 2004. Effects of organotin compounds on pubertal male rats. Toxicology 202:145–158.
- Grünberg, E., Eckert, K., and Maurer, H. R. 1997. Prothymosin-α1 enhances the interleukin-2 activated killer cell adhesion to and immunotoxicity against docetaxel-treated HT-29 colon carcinoma cells in vitro. Int. J. Thymol. 5:415–423.
- Haritos, A. A., Blacher, R., Stein, S., Caldarella, J., and Horecker, B. L. 1985. Primary structure of rat thymus prothymosin-α. Proc. Natl. Acad. Sci. USA 82:343–346.
- Heneweer, M., Houtman, R., Poortman, J., Groot, M., Maliepaard, C., and Peinenburg, A. 2007. Estrogenic effects in the immature rat uterus after dietary exposure to ethinylestradiol and zearalenone using a systems biology approach. Toxicol. Sci. 99:303–314.
- Hentze, M. W. 1997. eIF4G: A multipurpose ribosome adaptor. Science 275:500–501.
- Jensen, K. G., önfelt, A., Wallin, M., Lidums, V., and Andersen, O. 1991. Effects of organotin compounds on mitosis, spindle structure, toxicity and in vitro microtubule assembly. Mutagenesis 6:409–416.
- Kannan, K., Corsolini, S., Focardi, S., Tanabe, S., and Tatsukawa, R. 1996. Accumulation pattern of butyltin compounds in dolphin, tuna, and shark collected from Italian coastal waters. Arch. Environ. Contam. Toxicol. 35:64–69.
- Karesuo, Z., Sandaltzopoulos, R., Frangou-Lazaridis, M., Lai, C. Y., Tsolas, O., Becker, P. B., and Papamarcaki, T. 1998. Prothymosin-α modulates the interaction of histone 1 with chromatin. Nucl. Acid Res. 26:3111–3118.
- Karapetian, R. N., Evstafieva, A. G., Abaeva, I. S., Chichkova, N. V., Filonov, G. S., Rubtsov, Y. P., Sukhacheva, E. A., Melnikov, S. V., Schneider, U., Wanker, E. E., and Vartapetian, A. B. 2005. Nuclear oncoprotein prothymosin-α is a partner of keap1: Implications for expression of oxidative-stress protecting genes. Mol. Cell. Biol. 25:1089–1099.
- Lee, C. Y., Wey, S. P., Liao, M. H., Hsu, W. L., Wu, H. Y., and Jan, T. R. 2008. A comparative study on cannabidiol-induced apoptosis in murine thymocytes and EL-4 thymoma cells. Int. Immunopharmacol. 8:732–740.
- Li, J., Steen, H., and Gygi, S. P. 2003. Protein profiling with cleavable isotope coded affinity Tag (cICAT) reagents: The yeast salinity stress response. Mol. Cell. Proteom. 2:1198–1204.
- Li S., Ouyang, Y., Yang, G. H., and Pestka, J. J. 2000. Modulation of transcription factor AP-1 activity in murine EL-4 thymoma cells by vomitoxin (deoxynivalenol). Toxicol. Appl. Pharmacol. 163:17–25.
- Marinovich, M., Sanghvi, A., Colli, S., Tremoli, E., and Galli, C. L. 1990. Cytoskeleton modifications induced by organotin compounds in human neutrophils. Toxicol. In Vitro 4:109–113.
- Meiring, H. D., Van Der Heeft, E., Ten Hove, G. J., and De Jong, A. P. 2002. Nanoscale LC_MS(n): Technical design and applications to peptide and protein analysis. J. Sep. Sci. 25:557–568.
- Nagy, Z. S. 1999. Neuronal death in Down’s syndrome. J. Neur. Trans. 57:233–245.
- Nuoffer, C., Davidson, H. W., Matteson, J., Meinkoth, J., and Balch, W. E. 1994. A GDP-bound form of rab1a inhibits protein export from the endoplasmic reticulum and transport between Golgi compartments. J. Cell Biol. 125:225–237.
- Osmanagic-Myers, S., and Wiche, G. 2004. Plectin-Rack1 (receptor for activated kinase 1) scaffolding. A novel mechanism to regulate protein kinase activity. J. Biol. Chem. 279:18701–18710.
- Pálmer, H. G., Carbayo, M. S., Morán, P. O., and Larriba, M. J. 2003. Genetic signatures of differentiation induced by 1α, 2,5-dihyroxyvitamin D3 in human colon cancer cells. Cancer Res. 63:7799–7806.
- Palvimo, J., and Linnala-Kankkunen, A. 1990. Identification of a low-Mr acidic nuclear protein as prothymosin α. FEBS Lett. 277:257–260.
- Pang, J. X., Ginanni, N., Dongre, A. R., Hefta, S. A., Opiteck, G. J. 2002. Biomarker discovery in urine by proteomics. J. Proteome Res. 1:161–169.
- Papamarcaki, T., and Tsolas, O. 1994. Prothymosin α binds to Histone H1 in vitro. FEBS Lett. 345:71–75.
- Pei, L. 1999. Pituitary tumor-transforming gene protein associates with ribosomal protein 10S and a novel human homologue of DnaJ in testicular cells. J. Biol. Chem. 274:3151–3158.
- Pind, S. N., Nuoffer, C., McCaffery, J. M., Plutner, H., Davidson, H. W., Farquhar, M. G., and Balch, W.E. 1994. Rab1a and Ca2+ are required for the fusion of carrier vesicles mediating endoplamic reticulum to Golgi transport. J. Cell Biol. 125:239–252.
- Raffray, M., and Cohen, G. M. 1991. Bis(tri-n-butyltin)oxide induces cell death apoptosis in immature rat thymocytes. Arch. Toxicol. 65:135–139.
- Raffray, M., and Cohen, G. M. 1993. Thymocyte apoptosis as a mechanism for tributyltin-induced thymic atrophy in vivo. Arch. Toxicol. 67:231–236.
- Rappsilber, J., Ryder, U., Lamond, A. I., and Mann, M. 2002. Large-scale proteomic analysis of the human spliceosome. Genome Res. 12:1231–1245.
- Sburlati, A. R., Manrow, R. E., and Berger, S. L. 1991. Prothymosin-α antisense oligomers inhibit myeloma cell division. Proc. Natl. Acad. Sci. USA 88:253–257.
- Schrantz, J. 1990. TBTO marine paint under EPA scrutiny. Ind. Finishing 66: 58–61.
- Schuurman, H. J., van Loveren, H., Rozing, J., and Vos, J. G. 1992. Chemicals trophic for the thymus: Risk for immunodeficiency and autoimmunity. Int. J. Immunopharmacol. 14:369–375.
- Seinen, W., and Penninks, A. H. 1979. Immune depression as a consequence of a selective cytotoxic activity of certain organometallic compounds on thymus-dependent lymphocytes. Ann. NY. Acad. Sci. 320:499–517.
- Snoeij, N. J., Penninks, A. H., and Seinen, W. 1987. Biological activity of organotin compounds -an overview. Environ. Res. 44:335–353.
- Snoeij, N. J., Penninks, A. H., and Seinen, W. 1988. Dibutyltin and tributyltin compounds induce thymus atrophy in rats due to a selective action on thymic lymphoblasts. Int. J. Immunopharmacol. 10:891–899.
- Stridh, H., Orrenius, S., and Hampton, M. B. 1999. Caspase involvement in the induction by the environmental toxicants tributyltin and triphenyltin. Toxicol. Appl. Pharmacol. 156:141–146.
- Svitkina, T. M., Verkhovsky, A. B., and Borisy, G. G. 1996. Plectin sidearms mediate interaction of intermediate filaments with microtubules and other components of the cytoskeleton. J. Cell Biol. 135:991–1007.
- Takashi, S., Isao, Y., Hisashi, Y., Nahoko, K., Kazunari, K., and Mitsunori, M. 1998. Accumulation, metabolism, and depuration of organotin compounds in the marine mussels Mytilus graynus and Mytilus edulis under natural conditions. J. Agric. Food Chem. 46:304–313.
- Tanaka, K., Koga, Y., Taniguchi, K., and Nomoto, K. 1987. T-cell recruitment from the thymus to the spleen in tumor bearing mice: Phenotypical alteration and recruitment of thymocytes raised in a tumor bearing state. Cancer Res. 47:2136–2141.
- Thompson, P. M., Gotoh, T., Kok, M., White, P. S., and Brodeur, G. M. 2003. CHD5, a new member of the chromodomain gene family, is preferentially expressed in the nervous system. Oncogene 22:1002–1011.
- Tong, J. K., Hassig, C. A., Schnitzler, G. R., Kingston, R. E., and Schreiber, S. L. 1998. Chromatin deacetylation by an ATP-dependent nucleosome remodelling complex. Nature 395:917–921.
- Van der Kerk, G. J. 1976. Organotin compounds: past, present and future. In: Organotin Compounds: New Chemistry and Applications (Zuckermann, J. J., Ed.), Washington, DC: American Chemical Society, pp. 1–25.
- Van Loveren, H., Krajnc, E. I., Rombout, P. J., Blommaert, F. A., and Vos, J. G. 1990. Effects of ozone, hexachlorobenzene, and bis(tri-n-butyltin)oxide on natural killer activity in the rat lung. Toxicol. Appl. Pharmacol. 102:21–33.
- Vandebriel, R.J., Spiekstra, S.W., Hudspith, C.M., and van Loveren, H. 1999. In vitro exposure effects of cyclosporine A and bis(tri-n-butyltin)oxide on lymphocyte proliferation, cytokine (receptor) mRNA expression, and cell surface marker expression in rat thymocytes and splenocytes. Toxicology 135:49–66.
- Vos, J. G., De Klerk, A., Krajnc, E. I., Kruzinga, W., van Ommen, B., and Rozing, J. 1984. Toxicity of bis(tri-n-butyltin) oxide in the rat II. Suppression of thymus-dependent immune responses and of parameters of non-specific resistance after short-term exposure. Toxicol. Appl. Pharmacol. 75:387–407.
- Vos, J. G., De Klerk, A., Krajnc, E. I., van Loveren, H., and Rozing, J. 1990. Immunotoxicity of bis(tri-n-butyltin)oxide in the rat: effects on thymus dependent immunity and on non-specific resistance following long-term exposure in young versus aged rats. Toxicol. Appl. Pharmacol. 105:144–155.
- Wykretowicz, A., Munzig, E., Garbin, F., Schulze-Forster, K., Eckert, K., and Maurer, H. R. 1994. Prothymosin-α1 stimulates selected functions of human polymorphonuclear neutrophils. Int. J. Thymol. 2:196–201.
- Yang, H., Zhuo, J., Ochs, R. L., Henning, D., Jin, R., and Valdez, B. C. 2003. Down-regulation of RNA Helicase II/Gu results in the depletion of 18 and 28S rNAs in Xenopus oocyte. J. Biol. Chem. 278:38847–38859.
- Yates, J. R., Eng, J. K., McCormack, A. L., and Schieltz, D. 1995. Method to correlate tandem mass spectra of modified peptides to amino acid sequences in the protein database. Anal. Chem. 67:1426–1436.
- Yoo, B. C., Vikolinsky, R., Engidawork, E., Cairns, N., Fountoulakis, M., and Lubec, G. 2001. Differential expression of molecular chaperones in brain of patients with Down Syndrome. Electrophoresis 22:1233–1241.