Abstract
The immunotoxicant bisphenol A (BPA) may produce toxic effects on organs and systems, in part, by altering the secretion of cytokines and chemokines. However, systematic studies of the effects of BPA, let alone of its analogs and in cases when there are interactions with other chemicals, on innate immunity and cytokine modulation are limited. The objectives of this study were to investigate the immunomodulatory effects of: (1) BPA and its analogs, BPS and BPAF; and (2) the interaction between BPA and genistein (GEN), a partial estrogen agonist or antagonist. BPA, BPS, and BPAF were incubated with PMA-differentiated-U937 cells (a widely used cell line for primary human macrophages) at concentrations of 0, 0.1, 1, 10, 100 µM for up to 96 h. BPA (0, 0.1, 1, 10 µM) and GEN (0, 1, 10 µM) were also applied at various combinations. Cell viability and 30 cytokines/chemokines were measured. The results showed that the cell viability-inhibiting effect of these three bisphenols was BPAF > BPA > BPS. At 0.1 µM, BPA and BPAF generally increased the secretion of cytokines/chemokines, while BPS had minimal effects. All three bisphenols generally suppressed the secretion of cytokines/chemokines at 1 µM, while increased their secretion at 10 µM. The most increased cytokines/chemokines were interferon (IFN)-γ, interleukin (IL)-1RA, IL-8 and MIP-1β, and the most decreased was IL-10. GEN increased cell viability at low BPA concentrations but had no effect when BPA levels were high. In general, GEN attenuated the BPA-induced secretion of cytokines/chemokines but enhanced it at low BPA concentrations. In conclusion, this study showed that BPA, BPS, and BPAF were immunotoxic to macrophages: BPS was the least toxic, while BPAF was the most toxic. Further, GEN reversed suppressive effects on macrophages that resulted from exposure to high concentrations of BPA and produced synergetic effects with BPA at low concentrations.
Introduction
Bisphenol A (BPA, 2,2-bis(4-hydroxyphenyl) propane) is used in plastics and epoxy resins to make food and beverage containers, thermal papers (used in receipts, tickets, books, and so on), toys, medical equipment, and dental products. BPA leaches from these products under normal conditions of use and gets into human bodies through the digestive system, respiration, and dermal contact. Based on the higher levels of BPA detected in food (up to 100s of ng/g), dental sealants (up to 10s of ng/ml [leaching levels]), water (up to 10,000 s of ng/L), and paper products (up to 10–100 μg/g), as opposed to in air (<18 ng/m3) and dust (0.2–17.6 μg/g) (Vandenberg et al. Citation2007), oral ingestion is considered the main route for human BPA exposure. The GI tract contains a large population of immune cells, with macrophages being the most abundant leukocytes in lamina propria (Mowat and Agace Citation2014). As such, ingestion of BPA could impact on local immunity in the gut. This would be in keeping with the fact that as an endocrine disruptor (ED), BPA exposure induces alterations in classical estrogen-target organs (e.g. brain, ovary, and uterus), as well as some non-classical targets, such as the immune system.
Both in vitro and in vivo studies showed that BPA exposure induced inflammation. Liu et al. (Citation2014) showed that 0.01 and 0.1 µM BPA exposure induced expression of T-helper (TH) cell Type-1 cytokines but suppressed TH2 cytokine expression in PMA (phorbol 12-myristate 13-acetate)-differentiated THP-1 cells, through activation of the estrogen receptor (ER)-α/β-ERK-NF-κB axis. Direct and indirect exposures to BPA were associated with inflammatory responses represented by the production of cytokines/chemokines and other inflammatory mediators in animals (O'Brien et al. Citation2014) and humans (Watkins et al. Citation2015). However, there exist inconsistencies in the literature regarding the role of BPA in inflammation. For example, studies with lipo-polysaccharide (LPS)-activated macrophages showed that up to 100 µM BPA exposure decreased the LPS-induced iNOS and tumor necrosis factor (TNF)-α expression in human and murine macrophages (Kim and Jeong Citation2003; Byun et al. Citation2005) by inhibiting the ER-NF-κB axis (Byun et al. Citation2005).
Bisphenol S (BPS; 4-hydroxyphenyl sulfone) and bisphenol AF (BPAF; [4,4′-hexa- fluoroisopropylidene] diphenol) are two BPA analogs. BPS, used as the major replacement for BPA, has been readily measured in paper products/thermal papers (Liao et al. Citation2012; Pivnenko et al. Citation2015). While in vivo and in vitro endocrine, cyto-, geno-, and reproductive toxicities of BPS have previously been reviewed (Rochester and Bolden Citation2015; Chen et al. Citation2016), information on the immunotoxicity of BPS remains relatively unknown. BPAF is found with far less frequency (than BPA or BPS) in consumer products, environmental compartments and in human fluids, and information on its safety and toxicity is limited (Chen et al. Citation2016). This knowledge gap should be filled because BPAF has the longest half-life among the three bisphenols and a high log KOW (octanol-water partition coefficient) (Chen et al. Citation2016). Accordingly, its persistence in the environment and potential to be absorbed/accumulated in tissues should be of great concern.
Genistein (GEN) is a phytoestrogen found in leguminous food plants. GEN binds to both ER-α and -β at much higher affinities than BPA (Morito et al. Citation2001), and thus possesses greater transactivation capabilities (Katchy et al. Citation2014). Although co-exposure to BPA and GEN resulted in additive estrogenic effects in MCF7 breast cancer cells (Katchy et al. Citation2014), it was also seen that there was a decreased epithelial-mesenchymal transition and migration among BG-1 human ovarian cancer cells following BPA and GEN co-exposure (when compared to exposing to BPA alone) (Kim et al. Citation2015). Apart from these effects in cancer cells, it has also been observed that GEN can act as an immunoregulator using ER-independent modes of action (MOA). One such MOA is through interference with the binding of LPS to its receptor on immune (and other) cells, toll-like receptor (TLR)-4 (Jeong et al. Citation2014). With these studies as background, it remains to be determined if there are any interactive effects (akin to what was noted in the cancer cell studies) between BPA and GEN with respect to their individual effects on innate immunity or cytokine/chemokine modulation.
Even in the absence of systematic studies of BPA (let alone of its analogs and during interactions with other chemicals) effects on innate immunity and cytokine modulation, it would seem plausible BPA may act like many other immunotoxicants by causing alterations in the secretion of cytokines and chemokines. Accordingly, the objectives of this study were to investigate: (1) the immunomodulatory effects of BPA (and its analogs BPS and BPAF) in a human cell model system, i.e. the U937 differentiated macrophage; and, (2) the potential interactions (immunotoxicologic) between BPA and GEN. It was hypothesized here that BPA, BPS, and BPAF would impart differential modulatory effects on cytokine/chemokine production in these cells and that these effects which might be modulated (i.e. attenuated) by co-exposure to GEN.
Materials and methods
Cell culture and viability test
Human monocytic leukemia U937 cells (ATCC, Manassas, VA) were grown in complete medium, i.e. RPMI 1640 supplemented with 10% fetal bovine serum (FBS), 10 mM HEPES, and 50 μg/ml gentamycin (all Amresco, Solon, OH). The cells were cultured at 37 °C in a 5% CO2 atmosphere, and sub-cultured weekly when they reached 70% confluence. For use in the assays below, aliquots (100 μl) of U937 (stock = 5 × 105/ml) cells were pipetted into each well of flat-bottom 96-well plates and treated with 10 nM PMA for 24 h to permit differentiation.
BPA, BPS, and BPAF (Sigma, St. Louis, MO) were dissolved in ethanol to yield 20 mM stock solutions which were then further diluted in medium to final concentrations of 0.2, 2, 20, and 200 μM. For this assay, 100 μl of each solution was applied to the differentiated-U937 cells with medium containing the corresponding amount of alcohol as controls. After 48 h treatment, 100 μl of the supernatant was saved for cytokine/chemokine determination, and the rest was removed and discarded. The cells were washed with phosphate-buffered saline (PBS, pH 7.4) and stained with Alamar blue (Bio-Rad Labs, Raleigh, NC) for 4 h. Fluorescence was then measured at excitation and emission wavelengths of 530 and 590 nm, respectively, using a Synergy 4 hybrid multi-mode microplate reader (BioTek Instruments, Winooski, VT). All experiments were conducted in replicate (four wells per dose).
For the study of potential interactions between BPA and GEN, GEN (LC Laboratories, Woburn, MA) was dissolved in DMSO (Sigma) to yield a 370 mM stock solution, which in turn was diluted using 25 mM sodium carbonate (as GEN has low water solubility) to concentrations of 4 or 40 μM. BPA was similarly prepared and diluted in medium to reach concentrations of 0.4, 4, and 40 μM. Each GEN solution (dose) was combined with each BPA solution (dose) at a ratio of 1:1 (v/v). Thereafter, 100 μl of each solution was applied to the differentiated U937 cells. Both supernatant collection and viability tests were then performed as above (over a 96 h period). All treatments were repeated in replicate (five wells/treatment condition).
Cytokine/chemokine quantitative and analysis
Levels of 30 cytokines/chemokines in culture supernatants, including epidermal growth factor (EGF), eotaxin, G-CSF, GM-CSF, interferon (IFN)-α2, IFNγ, interleukin (IL)-10, -12 (p40), -12 (p70), -13, -15, -17, -1RA, IL-1α, -1β, -2, -3, -4, -5, -6, -7, and IL-8, IFNγ-induced protein (IP)-10, monocyte chemoattractant protein (MCP)-1, macrophage inflammatory protein (MIP)-1α and -1β, TNFα and -β, vascular endothelial growth factor (VEGF) and regulated on activation, normal T-cell-expressed and -secreted (RANTES), were each measured via a MILLIPLEX MAP human kit (Millipore, Billerica, MA). In brief, sample supernatant and beads were combined in kit plate wells, and the plates then incubated at 4 °C overnight on an orbital shaker. Kit-provided detection antibody was then added, followed by streptavidin-phycoerythrin (PE). After 30 min incubation at room temperature, the plates were analyzed in a BioPlex MAGPIXTM Multiplex Reader (Luminex, Austin, TX) using xPONENT 4.2 (Luminex, Austin, TX) for data acquisition and MILLIPLEX Analyst 5.1 (Millipore, Bilerica, MA) for analysis. Each biomarker concentration was calculated in terms of pg/ml. Each sample was analyzed in three (BPA analogs) or five (BPA and GEN) replicates.
Statistics
Comparisons of test bisphenol effects on macrophage viability and cytokine/chemokine production were evaluated using a one-way analysis of variance (ANOVA), followed by a Duncan post-hoc test. Post-treatment cytokine/chemokine levels were also analyzed using Principle Component Analysis (PCA) to determine any differences in effects (30 cytokines/chemokines, all included) among the three agents. Lastly, potential BPA and GEN interactions as related to cytokine/chemokine production were analyzed using a two-way ANOVA, with each effect at an individual concentration of the other being conducted by simple effect analysis. All non-PCA analyses were carried out using SPSS Statistics 24 software (IBM, Armonk, NY). PCA analyses were done in SAS Institute software (Cary, NC). In all cases, significance was accepted as p < 0.05.
Results
BPA cytotoxicity in U937 differentiated human macrophages
Cytotoxicity of BPA in the U937 differentiated macrophages was evaluated in terms of viability for up to 96 h (). BPA treatment at 0.1–10 µM imparted no significant effects on viability within the first 48 h. BPA at 0.1 µM significantly increased the viability at 72 h, and both 1 and 10 µM concentrations significantly decreased viability by 96 h. Because the subsequent experiments were designed to investigate modulatory effects of bisphenols (and GEN) on cytokine/chemokine secretion by these cells, 48 h exposures were chosen to avoid significant alterations in cell viability as a confounding factor for any observed changes.
Cytokine/chemokine modulation in U937 differentiated macrophages by BPA, BPS, and BPAF
Data showed that BPA at low and high concentrations/doses could generate differential effects, suggesting the immunoregulatory effect of BPA and its analogs might also be non-dose dependent. As such, the tested concentrations of BPA, BPS, and BPAF here ranged from 0.1–100 µM, and their cytotoxicity and capacity to modulate U937 differentiated macrophage cytokine/chemokine production were evaluated following 48 h treatments. The results indicated BPS (at concentrations up to 100 µM) did not affect cell viability (). However, relative to control cultures (i.e. those receiving only vehicle in medium), BPA and BPAF significantly decreased viability at a concentration of 100 µM, with BPAF causing a >70% reduction in cell survival. When comparing the three bisphenols at 100 µM, interestingly, there were no significant differences between BPA and BPS (though as noted, the latter did not cause a significant reduction in viability vs. control); BPAF significantly decreased cell viability relative to the other forms. Due to this dramatic decrease in viability, changes in cytokine/chemokine levels associated with the cells were not analyzed from those that were treated with 100 µM BPAF.
Figure 2. Viability of human macrophages after treating with bisphenols at different concentrations for 48 h (n = 4, mean ± SD). **p < 0.01 vs. 0 µM; abp < 0.05 among bisphenols.
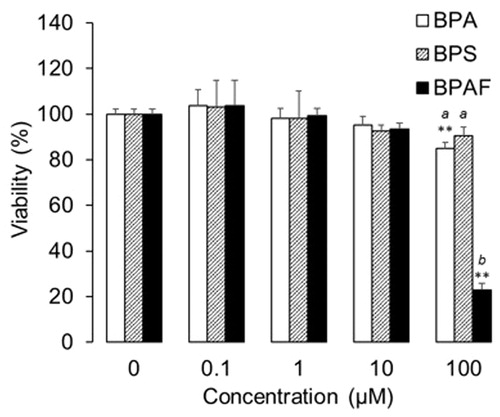
Thirty cytokine/chemokine levels were measured to determine if these three bisphenols differentially modulated cytokine/chemokine profiles (). Subsequently, the entire profile (alteration) associated with each bisphenol was analyzed by PCA (). At the lowest test concentration, i.e. 0.1 µM, both BPA and BPAF – but not BPS – caused an overall activation of cytokine/chemokine expression (reflected in PCA analysis; ). Overall, BPS over-lapped, BPA partially separated, and BPAF totally separated from the medium control. When outcomes were narrowed to reflect those cytokines/chemokines most impacted (i.e. by >20%; ), the results indicated that levels of IL-1β were dramatically increased by both BPA and BPAF, VEGF, G-CSF, IL-8, and MIP-1β were increased solely by BPA, and IFNγ was increased only by BPAF. In contrast, expressions of IL-10 and IL-15 were each decreased by BPA and BPS, respectively.
Figure 3. Heat-map of cytokine/chemokine profiles modulated by bisphenols. Numbers in the control line indicate cytokine/chemokine levels (pg/ml).
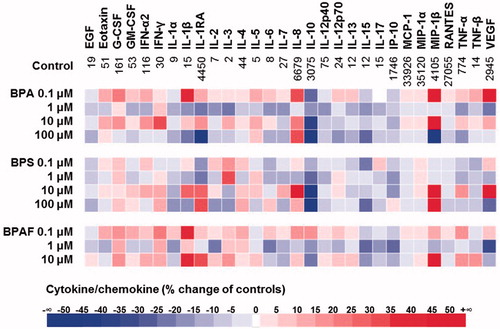
Figure 4. Analysis of cytokine/chemokine data regulated by bisphenols. (A, C, E, G) Differences among BPA, BPS, and BPAF effects on cytokine/chemokine modulation were analyzed using PCA by comparing to the control. (B, D, F, H) Cytokines/chemokines altered by >20%.
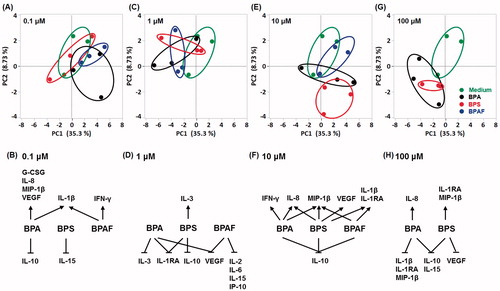
Exposure of the macrophages to 1 µM of each bisphenol (228.23 ng BPA/ml, 250.27 ng BPS/ml, and 336.23 ng BPAF/ml), levels close to BPA concentrations detected in human fluids and tissues (Vandenberg et al. Citation2007; Calafat et al. Citation2008), generally inhibited cytokine/chemokine production (). Of note, IL-1RA was decreased by both BPA and BPS, IL-10 was decreased only by BPS, VEGF was decreased by both BPA and BPAF, and IL-2, IL-6, IL-15, and IP-10 were decreased solely by BPAF. Although IL-3 was oppositely modulated by BPA and BPS, the absolute concentration was low (1.77–3.04 pg IL-3/ml). At an exposure level of 10 µM, several cytokines/chemokines were increased by BPA, BPS, and BPAF. Overall, BPS shifted the profile more than the other two agents (). The most affected cytokines/chemokines included MIP-1β which was up-regulated by BPA, BPS and BPAF, IL-8 which was up-regulated by BPA and BPS, IL-1RA and IL-1β both of which were up-regulated by BPS and BPAF, IFNγ which was uniquely up-regulated by BPA, and VEGF which only up-regulated by BPS. In contrast, IL-10 was down-regulated by all three bisphenols. Cytokine/chemokine profiles following exposure to 100 µM BPA or BPS revealed that both agents imparted inhibitory effects, with BPA being more potent (). Both IL-10 and IL-15 were dramatically suppressed by BPA and BPS, while IL-1RA and MIP-1β were oppositely regulated by BPA and BPS. In cases where only one of the two agents imparted a significant effect, IL-8 was up-regulated and IL-1β down-regulated by BPA, while VEGF was down-regulated by BPS.
BPA interacting with GEN
Potential interactions between BPA and GEN were studied using variable concentrations and treatment durations. GEN alone increased macrophage viability (relative to vehicle control) when treatment lasted up to 96 h. GEN was also able to mitigate the impact of BPA on cell viability when BPA was used at low concentrations (0.1 and 1 µM), but had no effect when the BPA was used at 10 μM (). With regard to cytokine/chemokine production following 48 h treatment, BPA alone gave rise to similar effects in two experiments using two different vehicles (ethanol vs. DMSO). In general, GEN alone suppressed cytokine/chemokine production at 1 µM whereas it led to an increased formation at a concentration of 10 µM (, top panel). The interactive effects of BPA and GEN were significant for nine cytokines/chemokines, e.g. GM-CSF, IL-1RA, IL-8, IL-10, IP-10, MIP-1α, MIP-1β, RANTES, and TNFα ().
Figure 5. Viability of human macrophages after treating with BPA and/or genistein (GEN) at different concentrations and for different durations (n = 5, mean ± SD). *p < 0.05, **p < 0.01 vs. GEN (0 µM).
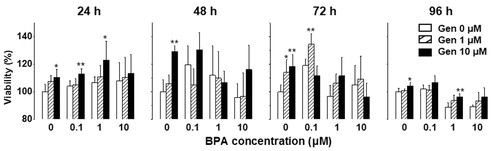
Figure 6. Heat-map of cytokine/chemokine profiles modulated by BPA and/or GEN. Numbers in the BPA (0 µM) GEN (0 µM) indicate cytokine/chemokine levels (pg/ml).
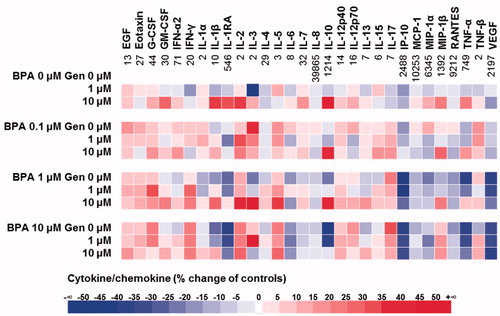
Figure 7. Effect of BPA and GEN on cytokine/chemokine expression. Significant interactions between BPA and GEN were detected on cytokines (GM-CSF, IL-1RA, IL-10, and TNFα) and chemokines (IL-8, IP-10, MIP-1α, MIP-1β, and RANTES). *p < 0.05 vs 0 µM BPA at same GEN level, +p < 0.05 vs 0 µM GEN at same BPA level.
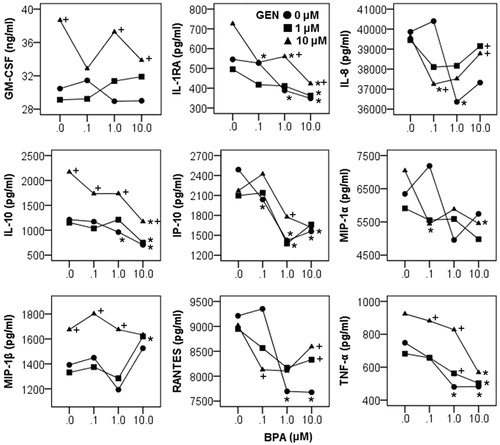
Interactions between BPA and GEN were in general characterized by non-parallel lines and crossing of lines (). When the interaction was detected, the impact of one chemical on cytokines/chemokines was analyzed at each level of the other (and this was marked in the graphs as well). Four interaction patterns can be summarized. (1) GM-CSF expression was- decreased by BPA at increasing concentrations when there was no GEN, increased by BPA at increasing concentrations when present with 1 µM GEN, and showed bimodal effect when present with 10 µM GEN. (2) Levels of IL-1RA, IL-10, and TNFα were decreased with increasing BPA concentrations in spite of the applied concentration of GEN. Low concentration GEN (1 µM) did not alter the quantity of these three cytokines, whereas high concentration (10 µM) significantly increased the cytokine production. (3) GEN alone showed no effects but could attenuate inhibitory effects of BPA on IL-8, RANTES, IP-10, and MIP-1α production. (4) MIP-1β was increased by BPA at 10 µM and GEN at 10 µM.
Discussion
In the current study, BPA at multiple concentrations i.e. 0.1, 1, 10, or 100 μM - levels calculated to be equivalent to 22.83, 228.29, 2282.90, and 22829.00 ng/ml – were tested, as BPA over a wide range of doses is known to impart non-monotonic effects (Vandenberg et al. Citation2012). These concentrations also reflected BPA levels calculated as being in human fluids and organs after normal and high BPA exposures. Analysis of human fluids and tissues showed that urinary BPA levels ranged from 0.4–149 ng/ml (Calafat et al. Citation2008), unconjugated BPA in sera ranged from 0.2–20 ng/ml, and placental tissue averaged at 11.2 ng/g with an upper range of 104.9 ng/g (Vandenberg et al. Citation2007). The United States EPA selected 50 μg/kg/day as a reference dose of BPA (Lorber et al. Citation2015). In vitro studies employed a broader range (i.e. 10−2 – 102 µΜ). Yoshitake et al. (Citation2008) applied up to 100 µM BPA to murine macrophages for 12 h and found no effect on cell viability, an outcome consistent with the 48 h no-effect observation in the current study. Literature dealing with longer exposures is rare. The current study found that exposure at 0.1 µM BPA (within range of levels in human fluids) increased macrophage viability at 72 h, but higher concentrations (1 and 10 µM) significantly decreased viability by 96 h.
Cytokine/chemokine modulation by BPA had been studied with different vehicles, and showed high consistency in this work - overall, BPA increased cytokine/chemokine expression at a level of just 0.1 µM, inhibited it at 1 µM, and differentially affected it at 10 µM (e.g. increased pro-inflammatory cytokines while inhibited anti-inflammatory IL-10), and generally inhibited that at 100 µM. These observations suggested that BPA exhibited its effect in an inverted U-type when applied at a broad range of doses, as is typical for ED agents (Takano et al. Citation2006). Interestingly, during modulation of the cytokines/chemokines, a break in the curve (at 1 µM) was noted for BPA.
The overall changes in cytokines/chemokines suggested that low-dose BPA (i.e. 0.1 μM) had a potential to non-specifically activate immune cells and/or lead to overactive immune responses. Similar activation and differentiation with BPA have been observed using bone marrow dendritic cell models (Pisapia et al. Citation2012). Both macrophages and dendritic cells are important antigen-presenting cells, cytokine/chemokine secretors, and bridge naïve and adaptive immunities. In animal models (mice), prenatal BPA exposure has been shown to augment formation of IL-4, IgG1, IgG2a, and IFNα; however, the increases in endpoints associated with TH1 responses (re: IgG2a and IFNα) were more than those with TH2 responses (re: IL-4 and IgG1) (Yoshino et al. Citation2004). A robust activation of the immune system and a shift in the TH1/TH2 balance after BPA exposure could be one reason for exacerbation of autoimmune diseases known to occur with exposure to BPA in experimental animals (Bodin et al. Citation2013).
Suppression of cytokines/chemokines by BPA at 1 and 100 µM might help to explain how host defense in exposed individuals is compromised during bacterial and/or virus infection (Sugita-Konishi et al. Citation2003). Specifically, subcutaneous BPA exposure (5 mg/kg) diminished clearance of Escherichia coli K-12 from the peritoneal cavity of BALB/c mice within 24 h. These effects were accompanied by reductions in neutrophil phagocytic activity and by reductions in expected levels of macrophages and lymphocytes at infection foci. Another study noted that BPA exposure (perinatal, 5 μg/kg/day) of Wistar rats resulted in decreased numbers of T-cells and dendritic cells in the spleen and mesenteric lymph nodes, outcomes that contributed to an increased residence of Nippostrongylus brasiliensis following infection (Ménard et al. Citation2014).
It should be noted that BPA specifically induced responses in the cells that could be deemed pro-inflammatory at certain concentrations, with the most altered signature cytokines being IL-10 (decreased) and IFNα (increased). The modulatory effect of BPA at low doses on cytokine/chemokine production was previously studied in differentiated human THP-1 macrophages (Liu et al. Citation2014). In that model, BPA induced a pro-inflammatory “profile” with the cells, with up-regulation of IL-6 and TNFα expression and down-regulation of IL-10 and TGFβ. This was an important observation, as inflammation as a contributing pathology has been implicated in various diseases, including obesity and diabetes (Shankar and Teppala Citation2011; Eng et al. Citation2013). Mechanistic studies have also shown that BPA could impair insulin-stimulated glucose utilization in human adipocytes; these effects occurred in tandem with induction of IL-6 and IFNγ production – events that evolved, in part, through activation of the JNK-STAT3-NF-κB axis in the cells (Valentino et al. Citation2013).
When the three test bisphenols, e.g. BPA, BPS, and BPAF, were compared for potential cytotoxicity in the U937 differentiated macrophage model, potencies were seen to be BPAF > BPA > BPS, a pattern consistent with previous studies using human hepatoma HepG2 cells (Fic et al. Citation2013) and normal human monocytes (Michalowicz et al. Citation2015). In those cases, it was believed that oxidative stress instead of genotoxicity was the primary mechanism underlying the cytotoxicities. These bisphenols also modulated cytokine/chemokine levels formed/released by the U937 differentiated macrophages. Each evinced similar types of modulation, i.e. all three imparted inhibitory effects at 1 µM in addition to an inverted U-type regulatory mode (BPAF at 100 µM was expected to cause overall inhibition of cytokines/chemokines due to cell death). Mechanisms for the cytokine/chemokine inhibitory effect at 1 µM needs to be studied further. Although BPS seemed to be less toxic than BPA and BPAF at the lowest test concentration here (i.e. 0.1 µM), concentration-related modulation of several signature cytokine/chemokines associated with inflammation (i.e. increases in IFNγ, IL-1RA, IL-8, and MIP-1β; decrease in IL-10) raise some concern.
Some mechanisms that underpin bisphenol-induced modulation of cytokines/chemokine formation/release have been proposed. One school of thought is that the effects are related to impact of the agents on ER in immune cells: BPA and BPAF each have estrogenic activities for both ER-α and -β; BPS selectively activates ER-α (Li et al. Citation2018). Moreover, BPA, BPS, and BPAF differentially recruit co-regulators to the bisphenol-ER-α complex; this could be one possible reason for differential biological actions induced by these agents. Qiu et al. (Citation2018) showed that BPA and BPS up-regulated IL-1β, INFγ, TNFα, IL-6, -8, -10, -11, and -12(p35) mRNA expression in association with increased expression of both ER-α and -β in fish primary macrophages (isolated from head kidney of Cyprinus carpio). BPA-induced ER-α signaling can lead to increased activation of ERK/MAPK and PI3K/AKT pathways (e.g. increased cell proliferation), while BPA-induced ER-β signaling involves p38/MAPK pathways (e.g. decreased call apoptosis); these changes could lead to (epi)genetic modifications (e.g. hormone receptors) (Acconcia et al. Citation2015). In addition to ER, BPA has been reported to be able to bind to androgen, estrogen-related, thyroid hormone, pregnane X, peroxisome proliferator-activated, and aryl hydrocarbon receptors (Acconcia et al. Citation2015).
Compared to BPA, the MOA of its analogs are less understood. It was reported that BPS exposure at low concentrations induced up-regulation of inflammatory TNFα, IL-1β and -6, and down-regulation of TGFβ and IL-10 mRNA (Zhao et al. Citation2017). Those authors believed these effects were related to alterations in the regulation of the formation/removal of inflammation-associated metabolites and in lipid signaling pathways (i.e. glycolysis, GSH biosynthesis, sphingomyelin-ceramide signaling/biosynthesis, as well as degradation of glycerophospholipids and glycerolipids).
GEN was shown to be able to modulate the cytotoxicity of BPA, i.e. led to increases in cell viability by itself or in the presence of low concentrations of BPA. The effect of GEN on cytokines/chemokine production was generally opposite to that of BPA, and the effective GEN concentration was specific for individual cytokine/chemokine. For example, 1 µM GEN was able to abrogate any BPA (0.1–10 µM)-induced increases in IL-8, RANTES, and MIP-1α; effects on GM-CSF, IL-1RA, IL-10, TNFα, and IP-10 production required a higher GEN concentration. This was likely due to known anti-inflammatory properties of GEN that are mediated through ER. Specifically, a previous study showed that GEN differentially regulated ERα and -β to cause a down-regulation in the formation/release of inflammatory cytokines in a murine model of peritoneal endometriosis (Sutrisno et al. Citation2017). When rats were pre-pubertally exposed to GEN and BPA, opposite cell proliferation and apoptosis were observed at postpartum Day 21 and 50, with differential expression of ER-β, caspases, PARP, Bad, p21, Akts, PTEN, and SRC1–3 (Wang et al. Citation2014). ER-independent mechanisms have also been proposed to explain how GEN can act to down-regulate LPS-induced inflammation (Jeong et al. Citation2014). At this point, further study is needed to determine if ER-independent mechanisms might be involved in GEN-related changes (enhancing or mitigating, as related to BPA dose) in BPA-induced responses.
Conclusions
Several conclusions can be made based on the results of the current studies. (1) BPS was the least toxic among the three bisphenols tested in the U937 differentiated human macrophage model system, and BPAF was more toxic than BPA. (2) All three bisphenols generally suppressed secretion of the measured cytokines/chemokines at a level of just 1 µM, while BPA and BPAF increased production of several at dosed of both 0.1 and 10 µM. (3) Bisphenols differentially altered the production of several cytokines/chemokines at high concentration (e.g. increases in IFN-γ, IL-1RA, IL-8, MIP-1β vs. decreases in IL-10). (4) GEN increased the cell viability when in the presence of low BPA concentrations but had no effect when higher concentrations were provided. Overall, GEN attenuated BPA-induced production of some of the assayed cytokines/chemokines, but also seemed to enhance it when the BPA was present at a low concentration. Because macrophages play such a critical role in the immune system and are integral players in various pathologies, the present findings may shed light on potential detrimental effects of these endocrine disruptors.
Acknowledgments
The authors would like to thank Deborah Keys for the assistance in performing the statistical analyses. BPAF was a kind gift provided by Dr. Xiaozhong Yu (Department of Environmental Health Science, University of Georgia).
Disclosure statement
The authors declare no conflicts of interest. The authors alone are responsible for the content of this manuscript.
Additional information
Funding
References
- Acconcia F, Pallottini V, Marino M. 2015. Molecular mechanisms of action of BPA. Dose Response. 13:1559325815610582.
- Bodin J, Bølling A, Samuelsen M, Becher R, Lovik M, Nygaard U. 2013. Long-term bisphenol: A exposure accelerates insulitis development in diabetes-prone NOD mice. Immunopharmacol Immunotoxicol. 35:349–358.
- Byun J, Heo Y, Kim Y, Pyo M. 2005. Bisphenol A-induced down-regulation of murine macrophage activities in vitro and ex vivo. Environ Toxicol Pharmacol. 19:19–24.
- Calafat A, Ye X, Wong L, Reidy J, Needham L. 2008. Exposure of the U.S. population to bisphenol A and 4-tertiary-octylphenol: 2003–2004. Environ Health Perspect. 116:39–44.
- Chen D, Kannan K, Tan H, Zheng Z, Feng YL, Wu Y, Widelka M. 2016. Bisphenol analogues other than BPA: Environmental occurrence, human exposure, and toxicity-a review. Environ Sci Technol. 50:5438–5453.
- Eng D, Lee J, Gebremariam A, Meeker J, Peterson K, Padmanabhan V. 2013. Bisphenol A and chronic disease risk factors in U.S. children. Pediatrics. 132:e637–e645.
- Fic A, Žegura B, Sollner Dolenc M, Filipič M, Peterlin Mašič L. 2013. Mutagenicity and DNA damage of bisphenol A and its structural analogues in HepG2 cells. Arh Hig Rada Toksikol. 64:189–200.
- Jeong J, Lee H, Han M, Kim G, Kim W, Choi Y. 2014. Anti-inflammatory effects of genistein via suppression of the toll-like receptor 4-mediated signaling pathway in lipopolysaccharide-stimulated BV2 microglia. Chem Biol Interact. 212:30–39.
- Katchy A, Pinto C, Jonson P, Nguyen-Vu T, Pandelova M, Riu A, Schramm K, Samarov D, Gustafsson J, Bondesson M, et al. 2014. Co-exposure to phytoestrogens and bisphenol A mimics estrogenic effects in an additive manner. Toxicol Sci. 138:21–35.
- Kim Y, Choi K, Hwang K. 2015. Genistein suppressed epithelial-mesenchymal transition and migration efficacies of BG-1 ovarian cancer cells activated by estrogenic chemicals via estrogen receptor pathway and downregulation of TGF-β signaling pathway. Phytomedicine. 22:993–999.
- Kim J, Jeong H. 2003. Down-regulation of inducible nitric oxide synthase and TNF expression by bisphenol A via NF-B inactivation in macrophages. Cancer Lett. 196:69–76.
- Li Y, Perera L, Coons L, Burns K, Tyler Ramsey J, Pelch K, Houtman R, van Beuningen R, Teng C, Korach K. 2018. Differential in vitro biological action, co-regulator interactions, and molecular dynamic analysis of bisphenol A (BPA), BPAF, and BPS ligand-ER complexes. Environ Health Perspect. 126:017012.
- Liao C, Liu F, Kannan K. 2012. Bisphenol S, a new bisphenol analogue, in paper products and currency bills and its association with bisphenol a residues. Environ Sci Technol. 46:6515–6522.
- Liu Y, Mei C, Liu H, Wang H, Zeng G, Lin J, Xu M. 2014. Modulation of cytokine expression in human macrophages by endocrine-disrupting chemical Bisphenol-A. Biochem Biophys Res Commun. 451:592–598.
- Lorber M, Schecter A, Paepke O, Shropshire W, Christensen K, Birnbaum L. 2015. Exposure assessment of adult intake of bisphenol A (BPA) with emphasis on canned food dietary exposures. Environ Int. 77:55–62.
- Ménard S, Guzylack-Piriou L, Lencina C, Leveque M, Naturel M, Sekkal S, Harkat C, Gaultier E, Olier M, Garcia-Villar R, et al. 2014. Parinatal exposure to a low dose of bisphenol A impaired systemic cellular immune response and predisposes young rats to intestinal parasitic infection. Plos One. 9:e112752.
- Michalowicz J, Mokra K, Bak A. 2015. Bisphenol A and its analogs induce morphological and biochemical alterations in human peripheral blood mononuclear cells (in vitro study). Toxicol In Vitro. 29:1464–1472.
- Morito K, Hirose T, Kinjo J, Hirakawa T, Okawa M, Nohara T, Ogawa S, Inoue S, Muramatsu M, Masamune Y. 2001. Interaction of phytoestrogens with estrogen receptors-α and -β. Biol Pharm Bull. 24:351–356.
- Mowat A, Agace W. 2014. Regional specialization within the intestinal immune system. Nat Rev Immunol. 14:667–685.
- O'Brien E, Dolinoy D, Mancuso P. 2014. Perinatal bisphenol A exposures increase production of pro-inflammatory mediators in bone marrow-derived mast cells of adult mice. J. Immunotoxicol. 11:205–212.
- Pisapia L, del Pozzo G, Barba P, Caputo L, Mita L, Viggiano E, Russo G, Nicolucci C, Rossi S, Bencivenga U, et al. 2012. Effects of some endocrine disruptors on cell cycle progression and murine dendritic cell differentiation. Gen Comp Endocrinol. 178:54–63.
- Pivnenko K, Pedersen G, Eriksson E, Astrup T. 2015. Bisphenol A and its structural analogues in household waste paper. Waste Manag. 44:39–47.
- Qiu W, Yang M, Liu S, Lei P, Hu L, Chen B, Wu M, Wang K. 2018. Toxic effects of bisphenol S showing immunomodulation in fish macrophages. Environ Sci Technol. 52:831–838.
- Rochester J, Bolden A. 2015. Bisphenol S and F: A systematic review and comparison of the hormonal activity of bisphenol A substitutes. Environ Health Perspect. 123:643–650.
- Shankar A, Teppala S. 2011. Relationship between urinary bisphenol A levels and diabetes mellitus. J Clin Endocrinol Metab. 96:3822–3826.
- Sugita-Konishi Y, Shimura S, Nishikawa T, Sunaga F, Naito H, Suzuki Y. 2003. Effect of bisphenol A on non-specific immune defenses against non-pathogenic Escherichia coli. Toxicol Lett. 136:217–227.
- Sutrisno S, Aprina H, Simanungkalit H, Andriyani A, Barlianto W, Sujuti H, Santoso S, Dwijayasa P, Wahyuni E, Mustofa E. 2017. Genistein modulates the estrogen receptor and suppresses angiogenesis and inflammation in the murine model of peritoneal endometriosis. J Tradit Complement Med. 8:278–281.
- Takano H, Yanagisawa R, Inoue K, Ichinose T, Sadakane K, Yoshikawa T. 2006. Di-(2-ethyl-hexyl) phthalate enhances atopic dermatitis-like skin lesions in mice. Environ Health Perspect. 114:1266–1269.
- Valentino R, D'Esposito V, Passaretti F, Liotti A, Cabaro S, Longo M, Perruolo G, Oriente F, Beguinot F, Formisano P. 2013. Bisphenol-A impairs insulin action and up-regulates inflammatory pathways in human subcutaneous adipocytes and 3T3-L1 cells. PLoS One. 8:e82099.
- Vandenberg L, Colborn T, Hayes T, Heindel J, Jacobs D, Lee D, Shioda T, Soto A, vom Saal F, Welshons W, et al. 2012. Hormones and endocrine-disrupting chemicals: Low-dose effects and nonmonotonic dose responses. Endocr Rev. 33:378–455.
- Vandenberg L, Hauser R, Marcus M, Olea N, Welshons W. 2007. Human exposure to bisphenol A (BPA). Reprod Toxicol. 24:139–177.
- Wang J, Jenkins S, Lamartiniere C. 2014. Cell proliferation and apoptosis in rat mammary glands following combinational exposure to bisphenol A and genistein. BMC Cancer. 14:379
- Watkins D, Ferguson K, Anzalota del Toro L, Alshawabkeh A, Cordero J, Meeker J. 2015. Associations between urinary phenol and paraben concentrations and markers of oxidative stress and inflammation among pregnant women in Puerto Rico. Intl J Hyg Environ Health. 218:212–219.
- Yoshino S, Yamaki K, Li X, Sai T, Yanagisawa R, Takano H, Taneda S, Hayashi H, Mori Y. 2004. Prenatal exposure to bisphenol A up-regulates immune responses, including T-helper-1 and T-helper-2 responses, in mice. Immunology. 112:489–495.
- Yoshitake J, Kato K, Yoshioka D, Sueishi Y, Sawa T, Akaike T, Yoshimura T. 2008. Suppression of NO production and 8-nitroguanosine formation by phenol-containing endocrine-disrupting chemicals in LPS-stimulated macrophages: Involvement of estrogen receptor-dependent or –independent pathways. Nitric Oxide. 18:223–228.
- Zhao C, Tang Z, Yan J, Fang J, Wang H, Cai Z. 2017. Bisphenol S exposure modulate macrophage phenotype as defined by cytokines profiling, global metabolomics and lipidomics analysis. Sci Total Environ. 592:357–365.