Abstract
Aim of the study: Following exposure to toxic chemicals, skin uptake is a potential route of intoxication. Therefore, efficient methods for rapid skin decontamination to mitigate systemic effects are of utmost importance. In operational guidelines, skin decontamination is recommended to be performed by dry absorption and washing with water or soapy water. In the present study, evaluation of decontamination efficacy using water or soapy water was performed for five chemicals, three toxic industrial chemicals and two simulants for chemical warfare agents.
Materials and methods: Decontamination was initiated at time points 5, 15, 45 and 120 min after exposure in order to evaluate the time window for efficient decontamination. Experiments were conducted utilizing an in vitro skin penetration model to allow exposure of toxic chemicals on human skin.
Results: For all test substances, it was clearly demonstrated that decontamination had greater efficacy when initiated at the earliest time-point while decontamination after 120 min was less efficient. Adding soap to the water showed no significant improvement for any of the tested substances.
Conclusion: These results are of reledvance for the development of efficient operational decontamination procedures.
1. Introduction
Skin decontamination is the primary intervention to immediately remove toxic chemicals from the skin following exposure. On a practical basis, the most commonly operated wet decontamination procedures are washing with soap and water or water only.
The need for skin decontamination to mitigate systemic effects is related to the ability for dermal penetration of the chemical. For assessment of skin decontamination procedures in terms of life-saving measures, it is also mandatory to consider toxicity of the compound. Examples of hazardous compounds with well-known skin penetration properties are organophosphorus pesticides, chemical warfare nerve agents and certain toxic industrial chemicals. Dermal penetration is dependent on many different factors such as physicochemical properties of the chemical, substance concentration, involvement of a vehicle or carrier, possible occlusion, exposure pattern, environment factors and skin conditionCitation1,Citation2. The epidermal membrane on top of human skin, including the corneal outer layer stratum corneum, serves as a protective barrier against foreign materialsCitation3. Skin absorption through the epidermal barrier occurs via diffusion and the stratum corneum protects the lower layers of the skin by limiting the dermal absorption rateCitation4. Due to the hydrophobic nature of the stratum corneum, lipophilic molecules generally penetrate skin more readily than hydrophilic compoundsCitation5. Another major determinant for chemical penetration is the size of the molecule; the molecular weight should be below 500 Dalton to allow for skin absorptionCitation6. Volatility of chemicals is an additional parameter influencing dermal absorption and subsequently the need for decontaminationCitation7–9. As opposed to volatile compounds, non-volatile chemicals will remain on the surface for longer periods of time and consequently are more likely to penetrate the skin.
In response to exposures to toxic chemicals, disrobing will remove the majority of contamination from the casualtiesCitation10. However, when exposed to liquid or solid compounds, decontamination is normally required to remove the toxic chemical from skin, hair and eyesCitation11,Citation12. In guidelines for immediate operational decontamination, procedures generally consist of disrobing and wet decontamination by showering, washing with soap and water or water onlyCitation12. Lately, dry decontamination has been recommended for promptly initiated response following exposures for all non-corrosive liquid contaminantsCitation10,Citation13 Dry decontamination can be performed using any available absorbing material and has been emphasized to increase decontamination efficacy and minimize the risk of enhanced dermal penetration by wash-in effects. Evaluation of wet decontamination following exposure to industrial chemical have shown that differences in dermal absorption between chemicals and the choice of detergent may have an impact on the decontamination efficacyCitation14,Citation15.
To examine the generalisability of decontamination procedures, five chemicals with skin absorption properties were included in the present study of which three have been assessed as risk chemicals for harmful skin penetration. The choice of toxic industrial chemicals was based on risk assessment for percutaneous toxicity in occupational settingsCitation16–18. Acrylonitrile is used as an intermediate in the production of acrylic fibres, styrene plastics and adhesives. The chemical is rapidly absorbed via all routes of exposure and its toxic effects are ranging from headache and nausea to cardiovascular collapseCitation19. Several chemical accidents with acrylonitrile have been reported, e.g. Belgium in 2013 and Germany in 2008Citation20,Citation21. 2-Butoxyethanol is a high-production-volume glycol ether widely used within the industry, commonly included as a solvent in surface coatings in addition to metal and household cleaners and paints. The chemical is readily absorbed following inhalation, oral and dermal exposure and causes headache, vomiting, breathing difficulties and effects on the blood circulationCitation22. Human exposure has mainly been accidental in occupational settings or through contact with household productsCitation23. Tributylamine is utilized as an intermediate in a variety of chemical processesCitation24. The chemical has also been used a stabilizer for the nerve agent sarinCitation25. The main exposure routes are via the respiratory tract and through skin and following exposure both local effects, such as membrane irritation and breathing difficulties, in addition to systemic effects, e.g. convulsions, are observedCitation24. Two simulants for highly toxic chemical warfare agents were also included in the study; ethyl lactate and methyl salicylate which previously been used as simulants for chemical warfare agents in in vivo decontamination studies on human volunteersCitation18. Ethyl lactate is a simulant for the nerve agent sarin which is highly toxic whether inhaled or via skin uptakeCitation26. Sarin was used in two terrorist attacks in Matsumoto and Tokyo, Japan, in 1994 and 1995, which led to the death of 19 people and causing thousands to seek medical careCitation27,Citation28. There was also confirmed use of sarin in the Ghouta attack in 2013Citation29. Methyl salicylate is utilized as a simulant for sulphur mustard, which is a vesicant chemical warfare agent that upon skin contact causes blistering in the skin and mucous membranesCitation30,Citation31. It was used extensively in the Iran–Iraq war during 1980–1988 and most recently in the Syria conflictCitation32.
The aim of the present study was to evaluate the efficacy of the common wet decontamination procedures following skin exposure to the selected panel of chemicals. Specifically, the importance of early initiated wet decontamination was evaluated as well as the possible beneficial effects of adding soap to the washing procedure. Washing with soap and water or water only was initiated at four time points post-exposure: 5, 15, 45 and 120 min. The time points were selected based on scenarios: (1) an exposure in an environment with very high preparedness e.g. at a laboratory or an industrial plant (5 min), (2) immediate decontamination in the field performed by first responders (15 min), (3) decontamination performed by paramedics at a field decontamination unit or at the hospital emergency department (45 min) and (4) a delayed decontamination in the field or at the hospital (120 min). Experiments were conducted utilizing an in vitro skin penetration model to allow exposure of human skin to toxic chemicals. Skin penetration of the chemicals was monitored continuously during 5 h. The decontamination efficacy was evaluated by calculating a decontamination factor (DF), i.e. the ratio of the mean total cumulative penetrated amount without decontamination and the cumulative amount with a decontamination procedure.
2. Materials and methods
2.1. Chemicals
Acrylonitrile (≥99%; CAS no. 107-13-1), methyl salicylate (≥99%; CAS no. 119-36-8), ethyl lactate (≥99%; CAS no. 687-47-8), tributylamine (≥98.5%; CAS no. 102-82-9) and 2-butoxyethanol (≥99%; CAS no. 111-76-2) were obtained from Alfa Aesar (Haverhill, MA). DAX soap was acquired from CCS Healthcare AB (Borlänge, Sweden). The soap contained sodium laureth sulphate (3–5%), sodium chloride (1–3%), propylene glycol (<1%), citric acid (<1%) and glycerol (1–3%). The receptor solution contained a mixture of ethanol and deionized water (1:3).
2.2. Skin preparation
Full-thickness human Caucasian skin of abdominal origin was obtained from plastic surgery after informed consent of each patient. The full-thickness skin was cropped from subcutaneous fat and connective tissue and stored at −80 °C. Following acclimatisation at −20 °C for 3–4 days, skin was thawed in room temperature and dermatomed to a nominal thickness of 500 µm using the Humeca D80 dermatome (Humeca BV, Borne, The Netherlands). Small discs (0.6 cm2) were punched out and the discs were hydrated for 18–24 h at +4 °C before use. The physical condition of skin samples was assayed visually to exclude any surface damage. The study was approved by the Research Ethics Committee, Faculty of Medicine and Odontology, Umeå University, Umeå, Sweden (No. 03-161).
2.3. Skin penetration experiments
In vitro skin penetration experiments were performed utilizing a diffusion cell containing a flow-through receptor solution compartment as previously describedCitation33. The diffusion chamber was maintained at 32 °C throughout the experiments (AccuBlock Digital Dry Bath; Labnet Int. Inc, Edison, NJ) and the receptor solution was continuously pumped at a fixed flow rate of 20 μl min−1 using a syringe pump (CMA 400; CMA Microdialysis AB, Kista, Sweden). In prior to the experiment, the complete system was allowed to reach equilibrium of temperature and water balance. The dermatomed disc was mounted in the diffusion cell and a teflon seal was positioned upon to achieve a closed system. Prior to adding donor solution, a zero sample of receptor solution was collected. Following exposure of neat agent (10 µl) for 5, 15, 45 or 120 min, decontamination was initiated and the separate protocols are described below. Infinite dosing of agents was utilized throughout the study. Due to the high volatility of acrylonitrile, a total volume of 45 µl was used in control experiments. The nominal skin diffusion area was 0.13 cm2. The experimental time was 5 h and samples were collected every 10 min and kept at 10 °C in a fraction collector (CMA 470 Refrigerated Fraction Collector; CMA Microdialysis AB, Kista, Sweden). All vials were sealed immediately after the experiment was completed and stored at −20 °C until analysis. Each experimental set-up was repeated six times.
2.3.1. Soapy water decontamination protocol
The soapy water was an aqueous solution containing 2% soap in water, prepared immediately in prior of decontamination. Decontamination was performed in the diffusion cell by washing the agent off using 50 µl soapy water and instantly removed by careful vacuum suctioning. The procedure was repeated ten times.
2.3.2. Water decontamination protocol
In experiments including washing with water, 50 µl of water was added on skin in the diffusion cell and instantly removed by careful vacuum suctioning. The procedure was repeated ten times.
2.4. Sample analysis
The samples were thawed prior chromatographic analysis. A unique calibration curve for each replicate sample set was obtained by analysing standards in conjunction with the samples.
Acrylonitrile was analyzed by liquid chromatography with ultraviolet/visible spectroscopy detection (LC-UV/VIS), 2-butoxyethanol and tributylamine by liquid chromatography with triple quadrupole mass spectrometry detection (LC-MS/MS). Ethyl lactate and methyl salicylate were analyzed by gas chromatography with flame ionization detection (GC–FID). The limit of quantification (LOQ) was determined to be the lowest calibrated level, i.e. 1 ppm, for all substances ().
Table 1. Properties for chemicals included in the study.
The LC-UV/VIS analysis of acrylonitrile was performed on an Agilent 1100 series HPLC system, equipped with a multiple wavelength detector and the wavelength was set at 195 nm. The analyte was separated on a GromSil C-18, 100 × 2 mm (4 μm) column (GROM Analytik, Dr Maisch HPLC GmbH, Ammerbuch-Entringen, Germany) and eluted with an isocratic gradient. The mobile phase was milli-Q 18.2 MΩ, the flowrate was 0.2 ml min−1 and the column temperature was kept at ambient temperature (18−23 °C). The LC-MS/MS analysis of 2-butoxyethanol and tributylamine were carried out on an Acquity UPLC coupled to a Xevo TQ MS, triple quadrupole mass spectrometer (Waters Corp, Etten-Leur, The Netherlands). The MS was equipped with an electrospray ionization source (ESI) and operated in positive ion mode. The capillary voltage was set to 3.2 kV and argon was used as collision gas. The compounds were analyzed in multiple reaction monitoring (MRM) mode, each with two transitions, compound-specific settings are listed in .
Table 2. Compound-specific settings for analysis of tributylamine and 2-butoxyethanol.
The analytes were separated on an Acquity UPLC BEH C18, 2.1 × 50 mm (1.7 µm) column (Waters Corp, Etten-Leur, The Netherlands). Mobile phase A comprised 0.1% formic acid in water (v/v) and mobile phase B 0.1% formic acid in acetonitrile (v/v). Chromatographic separation was obtained by using a gradient starting at 5% B for 0.2 min, increasing to 98% B in 0.8 min, which was held for 0.5 min, decreased to 5% B in 0.1 min and held for 0.4 min. The flowrate was 0.6 ml min−1 and the column temperature was 60 °C.
The GC system (Agilent 7890A, Agilent Technologies Inc., Santa Clara, CA) was equipped with a split-splitless injector. The injection was a pulsed split injection with ratio 1:1 (pulse pressure 300 kPa until 0.75 min), the flow rate of the carrier gas was adjusted to ∼3 ml min−1 and the injector temperature was 180 °C. The temperature of the FID was adjusted to 280 °C, the H2 flow 30 ml min−1, the air flow 400 ml min−1 and the makeup flow 25 ml min−1. The GC oven programme for the ethyl lactate analysis was 120 °C held for 2 min, ramped 10 °C min−1 to 160 °C, ramped 40 °C min−1 to 250 °C that was held for 0.5 min. The separation was achieved on a Stabilwax® with Integra-Guard® column, 30 m × 320 μm × 1 μm (40–240 °C (250 °C)) (Restek Corp, Pittsburgh, PA). The GC oven programme for the methyl salicylate analysis was 100 °C, ramped 20 °C min−1 to 125 °C, ramped 40 °C min−1 to 225 °C, ramped 20 °C min−1 to 255 °C, ramped 30 °C min−1 to 270 °C that was held for 1 min. The separation was achieved on a DB-5MS column, 30 m × 320 μm × 1 μm (−60 °C to 300 °C (320 °C)) (Agilent Technologies Inc., Santa Clara, CA).
2.5. Data and statistical analysis
All results are presented as the mean ± the standard error of mean (SEM). The cumulative penetration following completed experiment, defined as cumulative amount, was calculated as µg cm−2 and the penetration rate as µg cm−2 h −1 in all experiments. For comparison of decontamination efficacy between products, the decontamination factor (DF) was defined as the ratio of the total cumulative amount of the control experiments (without decontamination) and the total cumulative amount of the experiments including decontamination.
The graphs were prepared and statistical analyses were performed using the GraphPad Prism programme (version 6.0 GraphPad software Inc., San Diego, CA). Statistical comparisons of the total cumulative amount were performed by using one-way analysis of variance (ANOVA) followed by Dunnett’s multiple comparison test. Statistical significance was assessed by using two-way ANOVA followed by Bonferroni multiple comparison test to determine differences in penetration rates. The significance was set at p < 0.05.
3. Results
The skin penetration ability of five chemicals was determined following exposure to neat agent. Observable penetration through dermatomed human skin was obtained for all chemicals evaluated during the 5 h experimental time (). Infinite dosing was utilized for all chemicals except for acrylonitrile due to vaporization during skin exposure. Acrylonitrile demonstrated the shortest lag time of all chemicals with a significantly increased penetration rate during the first 30 min post-exposure and the greatest cumulative amount penetrated during the 5 h experimental time. Tributylamine demonstrated the longest lag time and the lowest cumulative amount penetrated through skin after 5 h. Ethyl lactate and methyl salicylate displayed similar penetration patterns throughout the entire experimental time.
Figure 1. Cumulative penetration following skin exposure to neat agent concentration during 5 h experimental time. Acrylonitrile (top line), 2-butoxyethanol (2nd line), ethyl lactate (3rd line), methyl salicylate (4th line) and tributylamine (5th line). The results are presented as mean ± SEM (n = 6).
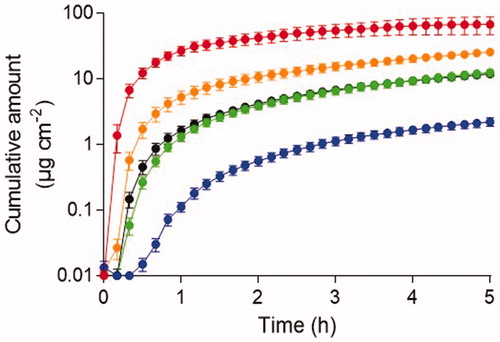
The decontamination efficacy of 2% soapy water and only washing the chemicals off by water was compared following exposure to neat acrylonitrile, methyl salicylate, ethyl lactate, tributylamine, and 2-butoxyethanol. Decontamination was initiated 5, 15, 45, or 120 min post-exposure by repeated washing 10 times with soapy water or only water. Due to the high volatility of acrylonitrile, only the 5 and 15 min decontamination time points were evaluated. To support comparisons of the decontamination efficacy between protocols, a decontamination factor (DF) was calculated for each experimental set-up.
Washing with water only resulted in significantly decreased cumulative penetration of all chemicals through skin when decontamination was initiated 5, 15, and 45 min post-exposure (). At the 120 min decontamination time-point, a significantly decreased agent skin penetration was demonstrated for acrylonitrile, ethyl lactate, methyl salicylate, and tributylamine. No decontamination efficacy was detected following skin exposure to 2-butoxyetanol at the 120 min decontamination time-point.
Table 3. The cumulative amount of chemicals penetrated through human dermatomed skin with or without water decontamination.
Following skin decontamination using water wash only, the agent penetration rate through skin decreased at all time-points for all chemicals exposed compared to the control (). The penetration rate of acrylonitrile dramatically decreased immediately following decontamination and skin penetration was completely eliminated 2 h post-exposure for both decontamination time-points evaluated. For 2-butoxyethanol, methyl salicylate, and tributylamine, agent penetration was detectable at the end of the experiment for all decontamination time-point evaluated. Water decontamination initiated 5–45 min post-exposure to ethyl lactate resulted in completely eliminated agent skin penetration during the experimental time. Following decontamination initiated 120 min post-exposure to methyl salicylate, an increased penetration rate was observed preceding the declining penetration rate to the end of the experimental time. The deliberate decrease in agent penetration rate throughout the experimental time and wide standard error of mean for the acrylonitrile control the last 2 h indicates agent evaporation from skin.
Figure 2. The penetration rate patterns for the neat agent concentration of (A) acrylonitrile, (B) 2-butoxyethanol, (C) ethyl lactate, (D) methyl salicylate, and (E) tributylamine following water decontamination. Decontamination was initiated after 5 min (open green), 15 min (closed green), 45 min (open blue), 2 h (closed blue) and without decontamination (black). Control line is the top line for all chemicals and the time points follow the order by which decontamination was initiated. Values are presented as the mean ± the SEM (n = 6). *p < 0.05 indicates significantly decreased penetration rate from the indicated time-point to the end of the experiment compared to experiment without decontamination.
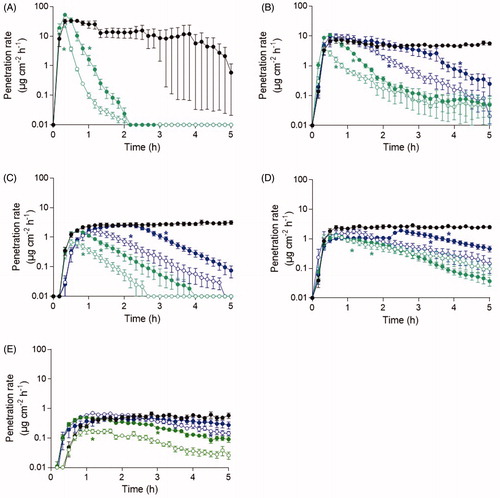
Washing with soapy water resulted in significantly decreased cumulative penetration when decontamination was initiated 5, 15, and 45 min post-exposure to all chemicals (). At the 120 min decontamination time-point, significantly decreased penetration was demonstrated for acrylonitrile, ethyl lactate, methyl salicylate and tributylamine. No decontamination efficacy was detected following skin exposure to 2-butoxyetanol at the 120 min decontamination time-point.
Table 4. The cumulative amount of chemicals penetrated through human dermatomed skin with or without decontamination with 2% soapy water.
Following skin decontamination using soapy water, the agent penetration rate through skin decreased at all time-points for acrylonitrile, 2-butoxyethanol, ethyl lactate, methyl salicylate, and tributylamine (). The penetration rate of acrylonitrile dramatically decreased immediately following decontamination and skin penetration was completely eliminated 1–2 h post-exposure for both decontamination time-points evaluated. For 2-butoxyethanol, decontamination initiated 5 and 15 min post-exposure resulted in completely eliminated skin penetration at the end of the experiment. A clear decrease in ethyl lactate penetration rate was established after decontamination at all time-points and, for the 5–45 min decontamination time-points, the skin penetration was completely eliminated. For methyl salicylate and tributylamine, agent penetration was detectable at the end of the experiment for all decontamination time-points evaluated. Following decontamination initiated 120 min post-exposure to 2-butoxyethanol and methyl salicylate, an increased penetration rate was observed preceding a declining penetration rate to the end of the experimental time.
Figure 3. The penetration rate patterns for the neat agent concentration of (A) acrylonitrile, (B) 2-butoxyethanol, (C) ethyl lactate, (D) methyl salicylate, and (E) tributylamine following soapy water decontamination. Decontamination was initiated after 5 min (open orange), 15 min (closed orange), 45 min (open red), 2 h (closed red) and without decontamination (black). Control line is the top line for all chemicals and the time points follow the order by which decontamination was initiated. Values are presented as the mean ± the SEM (n = 6). *p < 0.05 indicates significantly decreased penetration rate from the indicated time-point to the end of the experiment compared to experiment without decontamination.
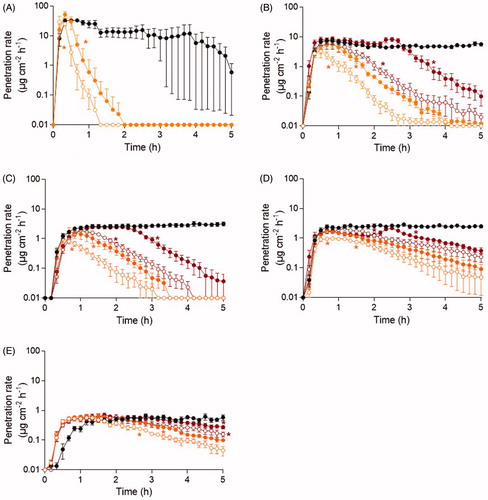
Comparison of cumulative amounts of chemicals penetrating skin resulted in no significant differences between decontamination using soapy water or water only.
4. Discussion
In the present study, skin decontamination procedures using water and soapy water were evaluated following exposure on human skin exposed in vitro to selected toxic industrial chemicals and simulants for chemical warfare agents. All chemicals in the test panel clearly penetrated the dermatomed skin when applied in neat agent concentration, although significant differences in penetration rates were observed. Theoretically, physicochemical properties such as volatility, molecular weight, and solubility in hydrophilic and lipophilic environments influence the degree of absorption in dermal tissueCitation47. Chemical absorption can be determined by analysis of chemical amounts remaining in the skin. However, to reflect the uptake of substances in the blood circulation we considered penetration through dermatomed skin to the receptor fluid as the most relevant parameter. Here, it was demonstrated that the relatively small molecule acrylonitrile displayed the shortest lag time (<10 min) and highest penetration rate among the tested compounds in agreement with the postulated correlation of low molecular weight with rapid penetration through the dermal barrierCitation48. However, due to vaporization of the liquid on the skin surface the cumulative penetrated amount of acrylonitrile declined over time despite the larger volume applied on skin compared to other chemicals in the present study, illustrating that dermal exposure of volatile liquids pose only a temporary risk for uptake in the blood circulationCitation9. However, if total immersion of the skin occurred, i.e. prolonged exposure time in an occupational setting, the risk for health effects following skin uptake of acrylonitrile would increase. Nevertheless, acrylonitrile demonstrated the greatest cumulative amount penetrated during 5 h of all compounds included in the study, which is consistent with the relatively low molecular weight. The compound with the largest molecular weight, tributylamine, showed a relatively extended lag time (approximately 30 min) compared to the other test compounds. In addition to molecular weight and volatility, lipophilic, and hydrophilic properties, often denominated as the octanol/water partition coefficient (LogP) is assumed an important determinant for penetration through the skinCitation47,Citation49,Citation50. Since a parabolic relationship between skin permeation and LogP has been demonstratedCitation51, the relatively low penetration rate of tributylamine is also consistent with the very high lipophilicity of the chemical.
To evaluate the time window for efficient decontamination, washing with water or soapy water was initiated 5, 15, 45, and 120 min after exposure. For all test substances, it was clearly demonstrated that decontamination had greater efficacy when initiated at the earliest time-point. Delayed decontamination to 120 min showed low efficacy resulting in a significant amount of agent penetrating the skin, but notably a significant reduction of penetrated compound through the skin was observed for ethyl lactate, methyl salicylate, and tributylamine. The importance of early initiated decontamination following skin exposure to chemicals has frequently been reportedCitation52–54. It should also be noted that for methyl salicylate, a temporary increase in penetration rate was observed directly following the washing procedure and a similar effect was observed for 2-butoxyethanol but only after washing with soapy water. This effect might be due to increased skin permeability induced by washing with water and soap, as previously described for other chemical compoundsCitation55–57. The underlying mechanisms of this “wash-in” effect are likely tissue hydration and eventually soap may enhance “wash-in” of substances by surfactant effects and pH changesCitation55–57. It is suggested that the “wash-in” effect depends on chemical identity and elapsed time between exposure and onset of the washing procedureCitation55–57, but no prediction model for which chemicals that attains enhanced penetration following wet decontamination has been presented.
From our in vitro data, repeated washing with soapy water did not show significant improvement compared to washing with water alone for any of the tested compounds, indicating that addition of soap might not be of significant benefit for the decontamination efficacy. However, it should be noted that the fixed experimental washing procedure used does not correspond to operational decontamination showering. It is recently shown that both flow rate and rinsing volume in showering off the compound from the skin, as well as composition of detergents influence decontamination efficacyCitation15. Another concern of the washing procedure, which could not be addressed with the in vitro model utilized, is the risk of spreading of the contaminant to other parts of the body during showering. For lipophilic compounds such as sulphur mustard it has been shown that water washing may lead to agent spreading to other skin surfaces resulting in increased skin toxicityCitation58,Citation59. In an operational context, simple, generic, and easily accessible procedures should be most beneficial. Recently the traditional generic procedure, based on immediate showering of fully clothed individuals with high volumes of water, has been challenged by the understanding that immense washing may transfer contamination from clothes to the underlying skinCitation10,Citation13. To avoid such “wash-in” effect, Chilcott et al. recommended an immediate disrobe and dry decontamination practice prior to flushing with water for most chemicals except corrosive liquidsCitation10. For the wet decontamination, the addition of soap is commonly recommended particularly for removal of lipophilic substancesCitation10, while for other chemicals the addition of soap might be counteractive due to the “wash-in” effectCitation55–57. The lack of beneficial effect by addition of soap observed in the present study for a panel of five chemicals adds another constraint to the recommendation of using soap in decontamination protocols.
In conclusion, by using an in vitro technology for evaluation of wet decontamination procedures on human skin, it was shown that early initiated decontamination is highly important for efficient removal of chemical compounds. Initial washing with water demonstrated efficacy to remove all tested compounds with no additive effect of including soap during decontamination. These results are of relevance for the development of efficient operational decontamination procedures.
Acknowledgements
The authors would like to thank Specialistkliniken (Umeå, Sweden) for the human skin supply. This work was supported the Swedish National Board of Health and Welfare.
Disclosure statement
No potential conflict of interest was reported by the author(s).
Additional information
Funding
References
- Poet TS, McDougal JN. Skin absorption and human risk assessment. Chem Biol Interact 2002;140:19–34.
- Mattie DR, Grabau JH, McDougal JN. Significance of the dermal route of exposure to risk assessment. Risk Anal 1994;14:277–284.
- Benford DJ, Cocker J, Sartorelli P, et al. Dermal route in systemic exposure. Scand J Work Environ Health 1999;25:511–520.
- Trommer H, Neubert RH. Overcoming the stratum corneum: the modulation of skin penetration. A review. Skin Pharmacol Physiol 2006;19:106–121.
- Potts RO, Guy RH. Predicting skin permeability. Pharm Res 1992;9:663–669.
- Bos JD, Meinardi MM. The 500 Dalton rule for the skin penetration of chemical compounds and drugs. Exp Dermatol 2000;9:165–169.
- Gilpin S. The influence of vapor pressure of chemicals on dermal penetration. Rev Environ Health 2014;29:181–184.
- Grégoire S, Cubberley R, Duplan H, et al. Use of a simple in vitro test to assess loss of chemical due to volatility during an in vitro human skin absorption study. Skin Pharmacol Physiol 2019;32:117–124.
- Rouse NC, Maibach HI. The effect of volatility on percutaneous absorption. J Dermatolog Treat 2016;27:5–10.
- Chilcott RP, Larner J, Matar H. UK’s initial operational response and specialist operational response to CBRN and HazMat incidents: a primer on decontamination protocols for healthcare professionals. Emerg Med J 2019;36:117–123.
- Matar H, Pinhal A, Amer N, et al. Decontamination and management of contaminated hair following a CBRN incident. Toxicol Sci 2019;171:269–279.
- Chilcott RP. Managing mass casualties and decontamination. Environ Int 2014;72:37–45.
- Chilcott RP, Larner J, Durrant A, et al. Evaluation of US Federal Guidelines (Primary Response Incident Scene Management [PRISM]) for mass decontamination of casualties during the initial operational response to a chemical incident. Ann Emerg Med 2019;73:671–684.
- Dennerlein K, Jäger T, Göen T, et al. Evaluation of the effect of skin cleaning procedures on the dermal absorption of chemicals. Toxicol in Vitro 2015;29:828–833.
- Pavlikova R, Misik J, Cabal J, et al. In vitro skin decontamination of paraoxon - wet-type cleansing effect of selected detergents. Cutan Ocul Toxicol 2018;37:77–83.
- Bunge AL, Persichetti JM, Payan JP. Explaining skin permeation of 2-butoxyethanol from neat and aqueous solutions. Int J Pharm 2012;435:50–62.
- Buchter A, Peter H. Clinical toxicology of acrylonitrile. G Ital Med Lav 1984; 6:83–86.
- James T, Wyke S, Marczylo T, et al. Chemical warfare agent simulants for human volunteer trials of emergency decontamination: a systematic review. J Appl Toxicol 2018;38:113–121.
- WHO. Acrylonitrile. Concise International Chemical Assessment Document 39; 2002.
- De Smedt T, De Cremer K, Vleminckx C, et al. Acrylonitrile exposure in the general population following a major train accident in Belgium: a human biomonitoring study. Toxicol Lett 2014;231:344–351.
- Leng G, Gries W. Biomonitoring following a chemical incident with acrylonitrile and ethylene in 2008. Toxicol Lett 2014;231:360–364.
- WHO. 2-Butoxyethanol. Concise International Chemical Assessment Document 10. 1998. ISBN 92 4 153010 3
- ATSDR. Toxicological profile for 2-butoxyethanol and 2-butoxyethanol acetate. US Department of Health and Human Services, Public Health Service, Agency for for Toxic Substances and Disease Registry; 1996.
- ECHA. Tributylamine 2020 [updated February 17, 2020]. Available from: https://www.echa.europa.eu/sv/web/guest/registration-dossier/-/registered-dossier/13327/7/3/4
- C B. Chemical stockpile disposal program: final programmatic environmental impact statement; 1988.
- Abou-Donia MB, Siracuse B, Gupta N, et al. Sarin (GB, O-isopropyl methylphosphonofluoridate) neurotoxicity: critical review. Crit Rev Toxicol 2016; 46:845–875.
- Beaton R, Stergachis A, Oberle M, et al. The Sarin Gas Attacks on the Tokyo subway – 10 years later/lessons learned. Traumatology 2005;11:103–119.
- Yanagisawa N, Morita H, Nakajima T. Sarin experiences in Japan: acute toxicity and long-term effects. J Neurol Sci 2006;249:76–85.
- UN. Report on the Alleged Use of Chemical Weapons in the Ghouta Area of Damaskus on 21 August 2013; 2013.
- Shakarjian MP, Heck DE, Gray JP, et al. Mechanisms mediating the vesicant actions of sulfur mustard after cutaneous exposure. Toxicol Sci 2010;114:5–19.
- Balali-Mood M, Hefazi M. Comparison of early and late toxic effects of sulfur mustard in Iranian veterans. Basic Clin Pharmacol Toxicol 2006;99:273–282.
- UN. Report of the OPCW fact-finding mission in Syria regarding the incident of 16 September 2016 as reported in the note verbale of the Syrian Arab republic number 113. S/2017/400.
- Thors L, Koch B, Koch M, et al. In vitro human skin penetration model for organophosphorus compounds with different physicochemical properties. Toxicol in Vitro 2016;32:198–204.
- Daubert T. Danner RP. Physical and thermodynamic properties of pure chemicals data compilation. Washington, DC: Taylor and Francis; 1989.
- National Technical Information Service Vol. OTS0571605.
- National Service Information Service. Vol. OTS0534646.
- Company DC. The glycol ethers handbook. Midland, MI: The Dow Chemical Company; 1990. p. 97.
- Dodd DE, Snellings WM, Maronpot RR, et al. Ethylene glycol monobutyl ether: acute, 9-day, and 90-day vapor inhalation studies in Fischer 344 rats. Toxicol Appl Pharmacol 1983;68:405–414.
- Dow Chemical Company Reports. Vol. MSD-46.
- Clary J, Feron V, van Velthuijsen J. Safety assessment of lactate esters. Regul Toxicol Pharmacol 1998;27:88–97.
- Opdyke DL, Letizia C. Monographs on fragrance raw materials. Food Chem Toxicol 1982;20:633–852.
- Esters BM. Pattýs industrial hygiene and toxicology. New York: John Wiley & Sons; 1994. pp. 3058–3059.
- Carpenter CP, Weil CS, Smyth HF. Range-finding toxicity data: list 8. Toxicol Appl Pharmacol 1974;28:313–319.
- Deutsche Gesundheitswesen. Vol. 15. 1960. p. 2179.
- National Technical Information Service Vol. PB158-508.
- National Technical Information Service Vol. PB158-507.
- Brown MB, Lau CH, Lim ST, et al. An evaluation of the potential of linear and nonlinear skin permeation models for the prediction of experimentally measured percutaneous drug absorption. J Pharm Pharmacol 2012;64:566–577.
- Magnusson BM, Anissimov YG, Cross SE, et al. Molecular size as the main determinant of solute maximum flux across the skin. J Invest Dermatol 2004;122:993–999.
- Chen CP, Chen CC, Huang CW, et al. Evaluating molecular properties involved in transport of small molecules in Stratum Corneum: a quantitative structure-activity relationship for skin permeability. Molecules 2018;23:911.
- Lian G, Chen L, Han L. An evaluation of mathematical models for predicting skin permeability. J Pharm Sci 2008;97:584–598.
- Grice J, Moghimi H, Ryan E, et al. Non-formulation parameters that affect penetrant-skin-vehicle interactions and percutaneous absorption. In: Percutaneous penetration enhancers drug penetration into/through skin. New York: Springer; 2017.
- Thors L, Lindberg S, Johansson S, et al. RSDL decontamination of human skin contaminated with the nerve agent VX. Toxicol Lett 2017;269:47–54.
- Joosen MJ, van den Berg RM, de Jong AL, et al. The impact of skin decontamination on the time window for effective treatment of percutaneous VX exposure. Chem Biol Interact 2016; 267:48–56.
- Noury B, Coman G, Blickenstaff N, et al. In vitro skin decontamination model: comparison of salicylic acid and aminophylline. Cutan Ocul Toxicol 2015;34:124–131.
- Moody RP, Maibach HI. Skin decontamination: importance of the wash-in effect. Food Chem Toxicol 2006;44:1783–1788.
- Zhu H, Jung EC, Phuong C, et al. Effects of soap-water wash on human epidermal penetration. J Appl Toxicol 2016;36:1526.
- Misik J, Pavlikova R, Josse D, et al. In vitro skin permeation and decontamination of the organophosphorus pesticide paraoxon under various physical conditions – evidence for a wash-in effect. Toxicol Mech Methods 2012;22:520–525.
- Chilcott RP, Jenner J, Hotchkiss SA, et al. In vitro skin absorption and decontamination of sulphur mustard: comparison of human and pig-ear skin. J Appl Toxicol 2001;21:279–283.
- Wormser U, Brodsky B, Sintov A. Skin toxicokinetics of mustard gas in the guinea pig: effect of hypochlorite and safety aspects. Arch Toxicol 2002;76:517–522.