Abstract
Consumer product manufacturers utilise a spectrum of alternative ocular irritation assays, as these tests do not require the use of live animals. Despite their usefulness, no regulatory-accepted assay assesses the reversibility of ocular damage, a key criterion of GHS ocular classification, like the rabbit eye test (i.e., Draize Rabbit Eye Test [DRET]) . The Porcine Corneal Opacity Reversibility Assay (PorCORA), an ex vivo intact corneal tissue culture model, predicts the reversibility of damage by ocular irritants. Inclusion of the damage reversibility endpoint in the PorCORA supplements other alternative test methods for ocular irritation, by assessing induced eye damage and the ability of this damage to reverse (heal) without the use of live animals to distinguish between Globally Harmonised System of Classification and Labelling of Chemicals (GHS) ocular classifications. In this focused study, results of a Bovine Corneal Opacity and Permeability (BCOP) test of a laundry detergent, neat and 10% dilution, (product mixture from S.C. Johnson & Son, Inc. [SCJ]) classified the product into GHS Category 1; however, the BCOP test cannot assess the reversibility of ocular damage. The laundry detergent was evaluated using the PorCORA, where ocular damage induced by the detergent was fully reversed within seven days. Evaluation of the reversibility of ocular damage using the PorCORA in this focused study can add strength to the weight-of-evidence (WoE) analysis approach in ocular hazard assessment. This WoE approach strengthens the argument that the PorCORA can be used to supplement BCOP data, and that this laundry detergent is not an irreversible eye irritant.
Introduction
The human eye may be unintentionally exposed to chemicals via a number of scenarios, including occupational exposure (e.g. when appropriate protective eyewear isn’t used), improper use of household chemicals, or other accidental exposure to hazardous chemicals or vapours [Citation1]. Following exposure, the usual recommendation is to immediately wash the eyes [Citation2]. One of the main components in the safety assessment of many types of products is the evaluation of their potential to cause eye injury [Citation3]. Serious eye damage (eye corrosion) is defined as ‘…tissue damage in the eye, or serious physical decay of vision, following application of a test substance to the anterior surface of the eye, which is not fully reversible within 21 days of application’ [Citation4]. Eye irritation is defined as ‘…changes in the eye following the application of a test substance to the anterior surface of the eye, which are fully reversible within 21 days of application’ [Citation5]. Eye irritants are classified according to GHS based on time of reversibility. If eye irritation effects are fully reversible within 21 days, the chemical or mixture is assigned Category 2 A or if eye irritation effects are fully reversible within 7 days, the chemical or mixture is assigned Category 2B.
While several in vitro methods addressing the ocular irritation endpoint have been found to be appropriate for specific applications, no single method has been identified as a complete replacement for the Draize Rabbit Eye Test (DRET). As toxicity test methods evolve, with a 3Rs (replace, reduce, and refine) approach to eliminate DRET, the growing importance of alternative test methods is clear [Citation6,Citation7]. The recently published OECD 492B (Reconstructed Human Cornea-like Epithelium [RHCE] Test Method for Eye Hazard Identification) can discriminate between three GHS categories (Category 1, Category 2 and not classified); however, the overall accuracy is only 69.2% [Citation8]. Additionally, the RHCE Test cannot sub-discriminate between GHS Category 2 A and GHS Category 2B. Therefore, the development of additional alternative methods is critical not only for toxicologists to accurately convey product hazards to consumers but also to uphold ethical standards of avoiding unnecessary animal testing whenever possible. The 3Rs principle has resulted in the development of several predictive alternative ocular irritation tests [Citation9]. These tests are:
Eye Irritation Test (EIT, OECD 492), SkinEthic™ Human Corneal Epithelium Time-to-Toxicity Test (HCE TTT, OECD 492B), Bovine Corneal Opacity and Permeability (BCOP, OECD 437) Test, Ocular Irritection Assay (OECD 496), Short-Time Exposure (STE, OECD 491), and Defined Approaches (DAs) for Serious Eye Damage and Eye Irritation (OECD 497) [Citation8,Citation10–14].
Despite the development of these alternative methods, the DRET in live animals is currently the only fully validated method to determine reversibility or healing [Citation15], following exposure to an ocular irritant [Citation16] and to discriminate between GHS Category 2 A (eye irritation, effects fully reversible within 21 days) and GHS Category 2B (mild eye irritation, effects fully reversible within 7 days). The DRET assesses damage to specific ocular structures i.e. cornea, conjunctiva, and iris for corneal opacity (CO), iritis (IR), conjunctival redness (CR), and conjunctival chemosis (CC). According to Barroso et al [Citation17], there are different criteria to assess the damage i.e. called ‘drivers of classification.’ The author explained in vivo drivers of GHS classification for the chemicals and sub-categorized in two categories: Category 2 A (irritant to eyes) when any of the ocular effects in any animal is not fully reversible within 7 days of observation (i.e. CO, IR, CR and/or CC > 0 at 7 ≤ day < 21) and 2B (mildly irritating to eyes) when all observed ocular effects are fully reversible within 7 days of observation (i.e. CO, IR, CR and CC = 0 on day 7 and beyond) [Citation17,Citation18].
The anterior surface of the eye mainly consists of the cornea and the conjunctiva. The cornea is the most exposed area and, therefore, the most likely part of the eye to be involved in chemical exposure [Citation19]. Any chemical injury to the cornea can result in loss of vision [Citation20]. For these reasons, the cornea has been the primary tissue modelled in in vitro and alternative assays, such as Porcine Corneal Opacity Reversibility Assay (PorCORA), for assessing eye injury [Citation21]. Reversibility of corneal damage is a key factor in determining the appropriate GHS ocular irritation hazard category classification; and PorCORA provides data on the reversibility of corneal damage.
PorCORA, an ex vivo method which addresses injury reversibility, was internally validated using 56 chemicals that ranged from corrosive (GHS Category 1) to non-irritating (GHS not classified). This validation showed that the PorCORA had an accuracy of 88% with a positive predictive value (PNV) and negative predictive value (NPV) of 91% (Category 1), and 85% (not Category 1), respectively, using Cooper Statistics [Citation22]. Re-examination of the data using in vivo drivers of classification concept of Barroso et. al. [Citation17] and its database and methodology demonstrated invalid tests for eight chemicals or GHS classification not driven by corneal opacities. When these chemicals were removed from the dataset, the accuracy improved to 90%, with a major change in sensitivity, which increased from 80% to 87% [Citation22].
As previously stated, none of the OECD-guideline-driven alternative tests predict reversibility [Citation15]. With these facts in mind, the PorCORA was developed to provide an alternative method of measuring corneal damage and recovery for extended periods (up to and beyond 21 days) in excised porcine corneas (obtained as waste by-products from local abattoirs) in long-term tissue culture [Citation23]. The PorCORA protocol is an extension of some of the methods based on related ex-vivo corneal culture system [Citation24], which uses an air interface culture technique to ensure maintenance of the cornea with epithelial integrity [Citation25,Citation26]. During this assay’s development and validation in 2010, the goal was to optimise methods and procedures to allow porcine corneas to be reliably cultured for at least 21 days, enabling assessment of reversibility for the same duration as in the in vivo DRET. Porcine corneas are anatomically more similar to human corneas when compared to bovine or rabbit corneas, considering features such as overall corneal thickness, number of epithelial layers, curvature [Citation27], and the presence of Bowman’s layer [Citation28-31].
The purpose of this study was to determine the potential ocular damage and recovery from the application of the SCJ consumer product, a laundry detergent, using BCOP and an ex vivo PorCORA procedure. The SCJ formula is a laundry detergent with a pH of approximately 8.0 that primarily contains water, multiple cleaning agents, and enzymes (see for additional formula information). The PorCORA results were used to supplement BCOP test results (see ) by exploring the ability of the PorCORA to provide further insight into time to injury reversibility. While this focused study may not be sufficient to categorise this laundry detergent specifically as GHS Category 2 A or 2B, it offers a WoE approach that adds strength to the argument that this laundry detergent is not an irreversible eye irritant.
Table 1. SCJ detergent formulation used in the study.
Table 2. BCOP Test Results of the test substance i.e. laundry detergent, negative control i.e. sterile deionised water, and Positive Control i.e. ethanol based on the OECD test guideline 437.
Materials and methods
Test substance
A laundry detergent, SCJ product, was evaluated in the BCOP test and PorCORA. Information on the laundry detergent formulation is shown in . In the BCOP test, the positive control was ethanol (Pharmco), and the negative control was sterile, deionised water (Quality Biological). In the PorCORA, the assessment of the reversibility of potential ocular damage induced by this consumer product was performed concurrently with three control materials. Dulbecco’s phosphate-buffered saline (dPBS; Lot No. RNBG1602, Sigma-Aldrich®) was used as a negative control. Ethanol (100% EtOH; Lot No. SHBH4983V, Sigma-Aldrich®) was used as a positive control for reversible damage, and sodium hydroxide (10% NaOH from 10 M solution, Lot No. BCBR1586V, Sigma-Aldrich®) was used as a positive control for irreversible damage.
Bovine corneal opacity and permeability
Pre-test procedure and long-term culture of excised corneas
Bovine eyes were obtained from a local abattoir as a by-product of freshly slaughtered animals (Baltimore, MD). The eyes were excised and then placed in Hanks’ Balanced Salt Solution (HBSS), containing penicillin/streptomycin, and transported to the laboratory in a refrigerated container. The corneas were examined for damage. The eyes were disinfected and rinsed by immersion in sterile Dulbecco’s phosphate-buffered saline (dPBS). Corneas were excised from the eyes and were rinsed again. The corneas were then re-examined and those with defects were discarded. The corneas were excised such that a 2- to 3-mm rim of the sclera remained. The isolated corneas were then stored in a petri-dish containing HBSS until mounted in a corneal holder. The corneas were mounted in the holders with the endothelial side against the O-ring of the posterior chamber and the anterior chamber was positioned on top of the cornea. Starting with the posterior chamber, the two chambers were filled with Minimum Essential Medium without phenol red, containing 1% foetal bovine serum and 2 mM of L-glutamine (MEM [without phenol red]). The corneal holders were incubated at 32 ± 1 °C for at least one hour.
Test substance preparation and pH determination
The test substance, i.e. laundry detergent, was used neat (without dilution) and as 10% dilution. The pH of the laundry detergent (neat and 10% dilution) was determined using pH paper (EMD Millipore Corporation). The measured pH values are presented in .
Method for testing liquid or surfactant materials
After one hour of incubation, the corneal holders were removed from the incubator. The medium was removed from both chambers and replaced with fresh MEM (without phenol red). The initial opacity of each cornea was determined using an Electro Design OP-KIT opacitometer. The medium was then removed from the anterior chamber.
A volume of 750 μl of the test substance, positive control, or negative control was administered into the anterior chamber (while slightly rotating the holder to ensure uniform distribution over the corneal epithelium) of the three corneas per treatment group. The corneas were incubated at 32 ± 1 °C for 10 min with the anterior chamber facing upward to ensure that the cornea was covered with the specified treatment. Following the exposure, the epithelial side of the corneas was washed at least three times with MEM containing phenol red to ensure total removal of the control or test substance, followed by a final rinse with MEM without phenol red. The chambers were refilled with fresh MEM (without phenol red) and the opacity of each cornea was measured. The corneas were returned to the incubator for approximately two hours after which a final measure of opacity was determined.
After the final opacity measurement, the medium was removed from both chambers. The posterior chamber was filled with fresh MEM (without phenol red) and 0.4% fluorescein solution was added to the anterior chamber. The corneas were then incubated in a horizontal position (anterior side up ensuring contact of the fluorescein with the cornea) for approximately 90 mins at 32 ± 1 °C. At the end of the 90-min incubation period, the medium was removed from the posterior chambers and placed into tubes numbered corresponding to the chamber numbers. A volume of 360 μl from each tube was placed into designated wells on a 96-well plate. The optical density at 490 nm was measured using a Molecular Devices Vmax kinetic microplate reader.
Fixation of corneas and histology evaluation
After the medium was removed for the permeability determination, each cornea was carefully separated from its corneal holder and transferred to an individual labelled tissue cassette. The cassettes were placed in 10% neutral buffered formalin to fix the corneal tissue for at least 24 h. A histological evaluation was performed on the corneas treated with the controls and test substance by a trained histopathologist from Institute for In Vitro Sciences (IIVS) [Citation32]. The fixed corneas were transferred to Alizee Pathology, LLC (Thurmont, MD) for embedding, sectioning, and staining. Each cornea was paraffin-embedded, bisected, and the two halves were mounted in the paraffin block. Each slide was then stained with haematoxylin and eosin.
Porcine corneal opacity reversibility assay
Pre-test procedure and long-term culture of excised corneas
Corneas were excised from disinfected porcine eyes received from a local abattoir (Spear Products Inc., Coopersburg, Pennsylvania). All procedures on mounted corneas were performed in a sterile field using sterile reagents suitable for cell culture. Porcine eyes were enucleated at the abattoir and shipped to the laboratory in a refrigerated container containing Hank’s Balanced Salt Solution (HBSS, Sigma-Aldrich®) supplemented with amphotericin B (Sigma-Aldrich®). Upon receipt, the eyes were transferred to a sterile field for corneal excision. Corneas were visually inspected for defects and those with defects were discarded (defects included abrasions, cuts, scratches, opacities, vascularisation, and pigmentation). The eyes were disinfected by immersion into a povidone-iodine solution and rinsed immediately by immersion in sterile dPBS. The eyes were then immersed in 0.1% Gentamicin (BioWhittaker, Walkersville, MD) in dPBS. Corneas were excised from the eyes and were rinsed extensively in sterile HBSS (pH 7.1). The corneas were then re-examined and those with defects (described above) were discarded. Each excised cornea was suspended epithelial-side down over a 24-well plate. Molten 1% agar (Sigma-Aldrich®) in culture medium (M199, Invitrogen, Carlsbad, CA) was added to the endothelial corneal cavities. The corneas and supporting congealed gel were inverted and transferred to sterile plastic culture dishes (four corneas per dish). Approximately 40 ml of warmed media (M199 medium and supplements) were added to the dish (leaving the corneal epithelium exposed to air). The mounted corneas were allowed to equilibrate in a humidified incubator equilibrated at 37 °C with 5% CO2 overnight before treatment. The agar/corneal tissue cultures were placed on a rocker platform modified to periodically pivot the culture dishes from a horizontal position to approximately 45 degrees to continually re-moisten the epithelium. The corneal culture medium was changed daily for the duration of the study. During culture, no microbial contamination was observed.
Dosing procedures
Twenty-four hours after the initiation of cultures, the culture medium was removed from the dishes. Corneal surfaces (n = 4 corneas per treatment) were exposed by direct application of 10 µl test substance or control for five minutes. Immediately following the five-minute exposure, the corneas were rinsed with 2 ml of sterile dPBS, mimicking real-life exposure when people initially tear up or lachrymal section and then seek out water or eye wash for rinsing after getting chemicals in their eyes. Once the corneas appeared completely rinsed off the test substance or control, the corneas were transferred to a new sterile culture dish, and 40 ml of warmed medium was added to the plate. The culture medium was replaced daily until the end of the study. The day of dose administration was designated study day 0.
Fluorescein staining and analysis
On study days 1, 2, 3, 7, 10, 14, and 21, media was removed from the plate and 2% sodium fluorescein (NaFL; Sigma-Aldrich®) in dPBS was added to the apical surface of each cornea under sterile conditions. The retention of NaFL stain was visualised using an ultraviolet transilluminator. Corneas were scored visually (See for scoring criteria and for each treatment group images and scores). The corneas were scored after fluorescein staining on specific days, in alignment with the DRET. Corneas were re-stained and monitored repeatedly in order to quantify cornea damage (indicated by retention of NaFL stain) and permit tracking of cornea healing and re-epithelialization. The mean stain-retention score and time to stain clearance were evaluated for the test substance-treated group of corneas in relation to the control groups at each NaFL staining time point to compare the ocular irritancy potential of the test substance. In this study protocol, if by study day 21 or earlier, none of the four corneas in a group retained NaFL stain, then the test substance was considered to cause only reversible ocular irritation (which can be used as supporting evidence to classify a substance into GHS Category 2). If any of the corneas in a treatment group retained stain on study day 21, the test substance was considered to cause irreversible (severe; GHS Category 1) ocular irritation. The term ‘reversible within’ when referring to study days refers to corneas for a particular group no longer retaining stain on that specific day (i.e. ‘reversible within 7 days’ means that the corneas in that particular group no longer retain stain on study day 7).
Table 3. Scoring criteria of sodium fluorescein stain retention on corneas.
Assay acceptance criteria
The results of the PorCORA were considered valid since all of the following conditions were met:
at least one of the corneas dosed with 10% NaOH (the positive control for irreversible damage) retained stain 21 days post-treatment
None of the corneas dosed with 100% EtOH (the positive control for reversible damage) retained stain 10 days post-treatment
There was no stain retention in the negative control (dPBS) corneas by study day 3
Results
Bovine corneal opacity and permeability
Histopathological evaluation
Epithelium
Negative control: The negative control-treated epithelium was composed of three layers i.e. Squamous, Wing, and Basal cell layers (). The basal cell layer was a well-formed, columnar-cell region directly attached to the basal lamina immediately anterior to the anterior limiting lamina. The basal cells were always tightly attached to each other. Several layers of wing cells covered the columnar basal layer. In both layers, the cell nuclei showed diffuse chromatin without clear nucleoli. Rare mitotic figures were seen in the basal layer. The squamous layer was flattened with limited cytoplasm and highly condensed nuclei.
Figure 1. Negative control (sterile, deionised water), 10-min exposure, 120-min post-exposure. (A) Full thickness, magnification 42x (B) Epithelium, magnification 420x (C) Stroma directly below the anterior limiting lamina, magnification 420x (D) Endothelium, magnification 420x.
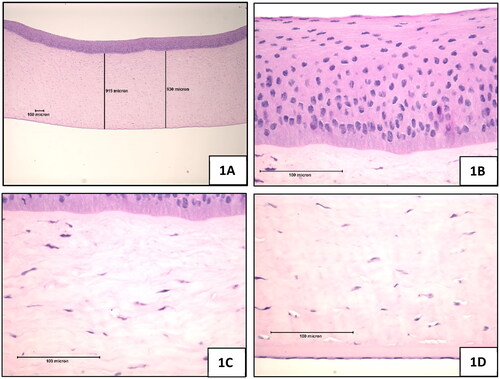
Positive control: The corneas showed the classic pattern of squamous layer coagulation (darkening) and marked vacuolisation in the wing and basal cell nuclei. The loss of adhesion between the basal cells (cell to cell) and the basal lamina was marked. The epithelium was probably not viable at the time of fixation ().
Figure 2. Positive control (100% ethanol), 10-min exposure, 120-min post-exposure. (A) Full thickness of the cornea which was appreciably thicker than the negative control treated corneas, magnification 42x (B) Epithelium, magnification 420x (C) Upper stroma showing hyperchromic staining i.e. coagulation in the zone directly below the anterior limiting lamina, stromal swelling, and the decrease in density of viable keratocytes, magnification 420x (D) Stroma near mid depth showing stromal swelling and keratocyte nuclear enlargement, magnification 420x (E) Endothelium (intact), magnification 420x.
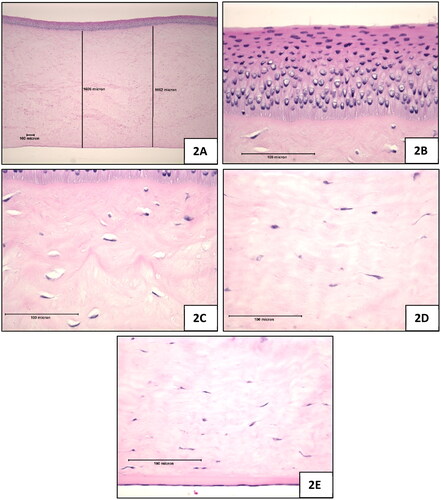
Test Substance: The epithelium exhibited a complete loss of all layers, per the expert evaluation of a trained histopathologist from IIVS ().
Figure 3. Test Substance (laundry detergent, neat), 10-min exposure, 120-min post-exposure. (A) Full thickness view of the cornea which was appreciably thicker than the negative control treated corneas, magnification 42x (B) Epithelium was lost in all sections, magnification 420x (C) Stroma directly beneath the anterior limiting lamina showing marked stromal swelling, and keratocyte degeneration, magnification 420x (D) Stroma near mid depth showing marked stromal swelling and moderate keratocyte enlargement, magnification 420x (E) lower stroma showing moderate collagen swelling without an appreciable increase in keratocyte changes, magnification 420x (F) Endothelium was generally intact, magnification 420x.
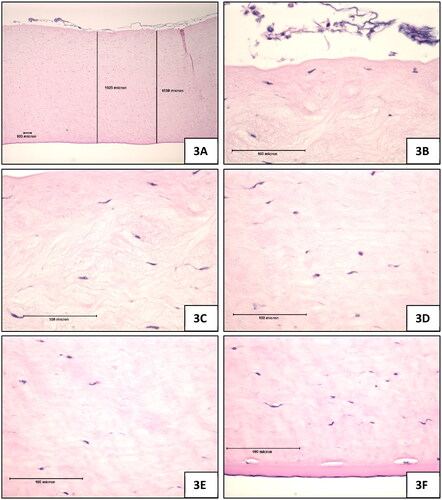
Stroma
Negative control: The cross-section of a negative control-treated cornea, showing the general thickness of the whole cornea and stroma is shown in . The stromal elements showed well-organised collagen matrix fibres with dispersed keratocytes (). Keratocyte nuclei showed a range of morphologies from moderate sized (smaller than the epithelial nuclei) with diffuse basophilic staining to narrow, elongated, and condensed with dark basophilic staining. Cytoplasmic staining, when it was visible, was moderately basophilic. Rare cells, with eosinophilic cytoplasmic staining, were observed. Collagen bundles were generally parallel and well ordered.
Positive control: Overall, the corneas were appreciably thicker than the negative control-treated corneas (). In the stroma directly below the anterior limiting lamina, the collagen matrix showed slight hyper-eosinophilic staining suggestive of some coagulation (). Below this zone, moderate/marked collagen matrix expansion extended past 50% depth. In the uppermost stroma, there was a decrease in the density of viable keratocytes as reflected by a marked increase in the frequency of keratocytes showing nuclear vacuolisation with a progression towards keratocytes showing condensed nuclei deeper into the stroma. In the stroma below mid depth, the keratocytes showed a moderate increase in the frequency of cells with enlarged nuclei and cytoplasmic eosinophilia as shown in .
Test Substance: The stroma was appreciably thicker than the negative control treated corneas (). The stroma directly beneath the anterior limiting lamina had marked stromal swelling and keratocyte degeneration (). The stroma at mid-depth had stromal swelling and moderate keratocyte enlargement (). The lower stroma had moderate collagen swelling without an appreciable increase in keratocyte changes ().
Endothelium
Negative control: Descemet’s Membrane was prominent. The endothelial layer could be seen in most sections and was reasonably well maintained as shown in .
Positive control: Descemet’s Membrane was prominent. The endothelial cells were generally intact (similar to the negative control-treated corneas) as shown in .
Test Substance: Descemet’s Membrane was prominent. The endothelium was generally intact ().
Porcine Corneal Opacity Reversibility Assay
Sodium fluorescein staining
A mean stain-retention area score (0 to 4; see scoring classification in ) was determined for each treatment group of four corneas on study days 1, 2, 3, 7, 10, 14, and 21 or until no stain was retained by all the corneas per treatment group.
dPBS. The mean stain-retention score for dPBS-treated corneas was 0.8 ± 0.5 (n = 4) on study day 1 (three of four corneas retained stain), which completely reversed by study day 2, i.e. none of the 4 corneas showed stain retention (score 0), which met the assay acceptance criteria. See .
Figure 4. Representative (A) digital images of NaFL staining of the dPBS-treated corneas (n = 4) (B) scoring table. The images show corneal damage and a decrease in stain retention over time. On each day, the corneas were not in the same order. The assigned NaFL stain-retention score of the dPBS-treated corneas are presented in Table (see for the scoring scale). Areas of stain retention (yellow–brown coloured stain) are indicated with arrows, depicting damage to the corneas.
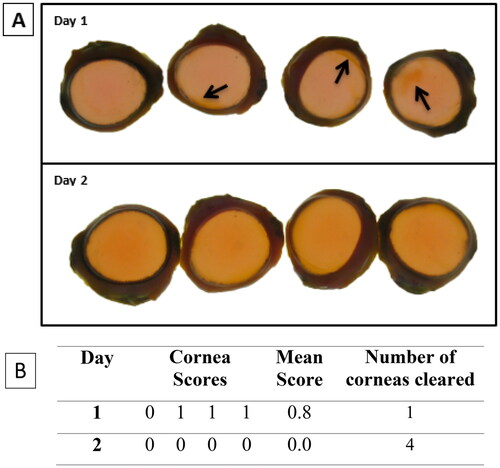
100% Ethanol. All EtOH-treated corneas showed stain retention on study day 1, which decreased to three corneas on study day 2 and two corneas study day 3. None of the four corneas retained stain on study day 7. The mean stain-retention score for EtOH-treated corneas was 3.5 ± 1.0 (n = 4) on study day 1, with a decrease to 1.0 ± 0.8 (n = 4) on study day 2. The mean score on day 3 was 1.0 ± 1.4. All EtOH-treated corneas showed complete loss of NaFL stain retention by study day 7, which met the assay acceptance criteria. See .
Figure 5. Representative (A) digital images of NaFL staining of the Ethanol-treated corneas (n = 4) (B) scoring table. The images show corneal damage and a decrease in stain retention over time. On each day, the corneas were not in the same order. The assigned NaFL stain-retention score of the 100% Ethanol-treated corneas are presented in Table (see for the scoring scale). Areas of stain retention (yellow–brown coloured stain) are indicated with arrows, depicting the damage to the corneas.
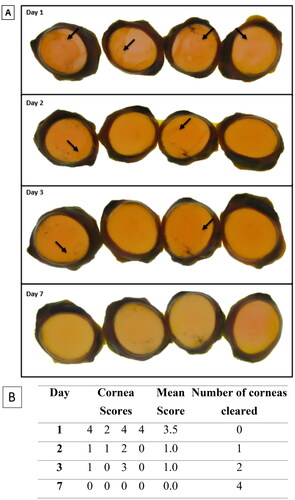
10% NaOH. All NaOH-treated corneas retained stain up to and including study day 21; all four of the corneas retained stain on the final day of the study. The NaOH-treated corneas had a high mean stain-retention score of 4.0 ± 0.0 (n = 4) on study day 1, which remained the same throughout the study and met the assay acceptance criteria. See .
Figure 6. Representative (A) digital images of NaFL staining of the NaOH-treated corneas (n = 4) (B) scoring table. The images show corneal damage and a decrease in stain retention over time. On each day, the corneas were not in the same order. The assigned NaFL stain-retention score of the 10% NaOH scores are presented in Table (see for the scoring scale). The entire surface of the corneas are covered with stain retention (yellow–brown coloured stain), depicting the damage to the corneas.
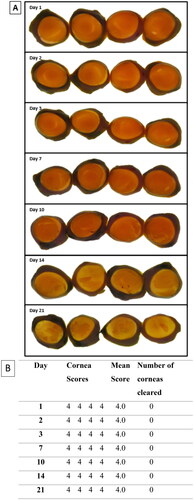
SCJ consumer product (Laundry Detergent). Three of four SCJ consumer product-treated corneas retained stain on study day 1, two corneas retained the stain on study day 2, four corneas retained the stain on study day 3 and none of corneas retained stain by study day 7. The mean stain-retention score for SCJ product-treated corneas was 0.8 ± 0.5 (n = 4) on study day 1 but decreased over the course of the study to 0.0 ± 0.0 (n = 4) by study day 7. Per the prediction model, the test substance is considered to cause reversible ocular irritation due to loss of stain retention on or before study day 21. See .
Figure 7. Representative digital images of NaFL staining of the SCJ consumer product-treated corneas (n = 4) (B) scoring table. The images show corneal damage and a decrease in stain retention over time. On each day, the corneas were not in the same order. The assigned NaFL stain-retention score of the SCJ consumer product scores are presented in Table (see for the scoring scale). Areas of stain retention (yellow–brown coloured stain) are indicated with arrows, depicting the damage to the corneas.
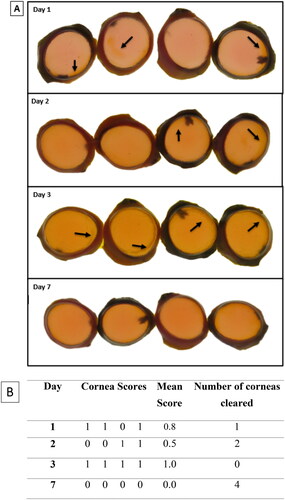
Discussion
There are several alternative methods to characterise diverse aspects of eye irritation and damage. However, at this time, there is no established method that can model the time to recovery after an injury such as in DRET. PorCORA was specifically developed to fill this void by measuring corneal damage and recovery for extended periods in excised porcine corneas (abattoir waste). When combined with other alternative assays, such as the Chorioallantoic Membrane Vascular Assay (CAMVA) for assessment of conjunctival injury and vascular damage and the BCOP test for assessment of acute corneal damage, PorCORA neatly fits as the missing piece that allows evaluation of corneal damage healing [Citation33]. Another approach by Varsho et al. [Citation34] showed a similar concept of using a dual assay approach system i.e. the combination of the EIT and BCOP test with a high degree of accuracy [Citation34]. Because corneal damage is generally the most persistent of ocular injuries, the time course measurements in the PorCORA are justifiable in modelling both reversible and non-reversible eye damage. This can be an important distinction for consumers and manufacturers of products for safety labelling, packaging, and shipping.
In a previous study, Piehl et al. [Citation23] demonstrated a temporal correlation throughout the 21-day study between damage visualised via NaFL stain and histopathological microscopic effects. The author advocated that the physiologically relevant and in vivo-like culture of the porcine corneas during the PorCORA 21-day study period provides a test system that can yield highly predictive ocular irritation and recovery information. This is due to preservation of the physiological processes of epithelial regeneration because the peripheral limbal stem cells are conserved as an intact and viable source for new corneal tissue [Citation23]. Similar to the DRET, porcine corneal tissues can be kept in culture over the course of 21 days and can model the recovery after injury. A previous study by Donahue et al., [Citation35] provided the initial idea for evaluating ocular irritation potential for personal care products in PorCORA [Citation35]. Three commercially available consumer products (a shampoo, a hair colour glaze, and a hair colourant system containing 12% hydrogen peroxide) were each tested in two PorCORA study replicates in order to assess potential ocular damage reversibility for surfactant-, propylene carbonate-, and peroxide-based formulations, respectively. A study by Ditzel et al. [Citation36] demonstrated PorCORA was used to determine the GHS Classification of a cleaner emulsifier and degreaser mixture [Citation36]. The author used PorCORA’s ability to determine GHS category 1 versus category 2 (2 A/2B) of a Fryer Cleaner and Degreaser (FC-D) mixture formula. In this study, PorCORA revealed complete healing of the cornea by study day 7, indicating reversible eye irritation and non-corrosivity.
In the BCOP test, following a 10-min exposure to the laundry detergent (neat), the In Vitro Irritation Score (IVIS) was 29.8. While the score itself was not high enough to warrant GHS Category 1 classification (IVIS was less than 55), histopathology revealed a complete loss of layers of the epithelium of the detergent (neat)-treated corneas (see ), which supported the low opacity values with high permeability values. The histopathology results and high permeability indicate that the detergent (neat) may cause severe injury. Further investigation with PorCORA provided valuable data on injury reversibility, which added to the weight of evidence that Category 1 may not be an appropriate category for the test substance. represents NaFL staining area over time by each treatment group. The conflicting data between BCOP and PorCORA highlight the influence of anatomical differences between bovine and porcine eyes on ocular irritation assessment. This emphasises the need for carefully selected species for ocular toxicity testing. Porcine corneas share a relatively similar size and shape with human corneas. The curvature and dimensions of porcine corneas are closer to those of humans compared to many other animal species such as bovine. This similarity allows for a better approximation of the human ocular surface area and curvature, which is important for accurate testing and research outcomes.
Figure 8. NaFL staining area over time by treatment group. Each line represents the progression towards loss of stain retention or re-epithelialization of a treatment group (n = 4 corneas) and each data point indicates the mean assigned damage score (0–4; maximum = 4) per treatment group on the indicated day post-dose (study day 1 through day 21). Error bars represent the standard error of the mean.
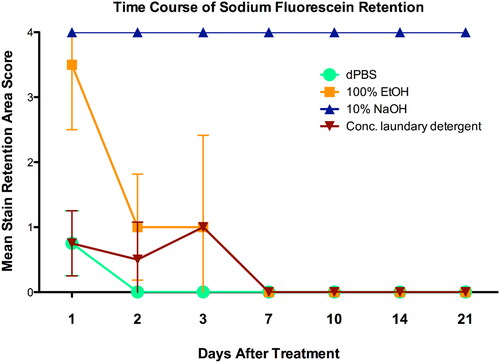
The negative control (dPBS) results of the PorCORA confirmed the successful physiological maintenance of porcine corneas in the culture system. As expected, dPBS-treated corneas in the study exhibited minimal damage which is attributable to the excision and handling process during the introduction of the corneas into the culture. This minimal damage implies that the damage caused by dPBS is less severe or of a lower magnitude via qualitative judgement when compared to the positive controls and the test substance. All dPBS-exposed corneas demonstrated complete loss of stain retention by study day 3, meeting assay acceptance criteria. All reversible positive control (100% ethanol)-treated corneas exhibited stain retention on the first day post-dosing (study day 1) and a progressive decrease in stain retention which fully cleared by study day 7, demonstrating reversible corneal damage. These results are comparable to the mean of 3.3 ± 1.15 days (n = 3) for corneal healing after exposure to 100% ethanol in the in vivo DRET [Citation35]. In the BCOP test, the In Vitro Irritation Score (IVIS) of 100% ethanol (positive control) was 42, an anticipated moderately irritating, non-corrosive response. The original PorCORA development study results for 100% ethanol indicated a mean of 5.0 ± 1 days (n = 32) to clear NaFL stain (data not shown). Therefore, the results obtained in the current study for 100% ethanol exposure are within the range of experimental variability. In contrast to the ethanol results, all four irreversible positive control (10% NaOH) treated corneas retained stain by the end of the 21-day study period, demonstrating clear non-reversibility of the ocular damage. The NaOH-treated corneal results of this study corroborate the results of previous PorCORA studies (data not shown) that also indicated sustained NaFL stain retention in 10% NaOH-exposed corneas on study day 21. In addition, historical Draize data for 10% NaOH indicate sustained corneal effects in rabbits on study day 21 [Citation37]. A progressive decrease in stain retention was observed among all test substance-treated corneas, culminating in complete loss of stain retention for all corneas by study day 7. Therefore, to supplement the results gathered in the BCOP test, strength is added to the WoE that the substance could be considered a reversible eye irritant.
In addition, stain is used repeatedly over the 21-day DRET to transiently label damaged tissue and monitor corneal injury recovery [Citation38]. In PorCORA, NaFL stain retention is monitored as an indicator of corneal injury. For the purposes of comparing PorCORA-based corneal effects to the historical Draize database, loss of NaFL stain retention in the PorCORA can be considered corneal ‘clearing.’ Therefore, the reversibility of ocular irritation/damage as indicated by PorCORA evidence implies the actual repair of the eye structure via corneal re-epithelialization. The results of this study support the continued use of PorCORA as a suitable alternative ocular irritation and reversibility assay that, in concert with other alternative assays, may be used to replace the in vivo DRET.
Conclusion
The development of novel alternative ocular toxicity test methods, such as the PorCORA, is crucial for toxicologists to accurately assess hazards and provide a means to do so, without the use of live animal testing (in this case, an ‘ex-vivo’ test). This work demonstrates the application of the PorCORA to evaluate the reversibility of eye injury induced by a laundry detergent (neat) with a pH of approximately 8.0 (primarily containing water, multiple cleaning agents, and enzymes) and is a focused study demonstrating the potential ability to assess mixtures and surfactants as well as enzyme formulations.
Reversibility or the ability of ocular tissues to heal following injury is a key determinant of GHS classification of ocular irritants. Herein, PorCORA was successfully applied to gain more information about the hazard of a detergent product mixture. Corneas treated with the mixture were found to be clear of fluorescein stain retention by seven (7) days post-treatment, in contrast to at or after 21 days, it was concluded that the detergent is a reversible ocular irritant, (comparable to ethanol), and not comparable to 10% NaOH, an ocular corrosive. Overall, this work provides an example of the application of the PorCORA as an alternative test method to determine the reversibility of eye injury in mixtures; and for use as a contributing factor to a ‘weight-of-evidence analysis approach’ in product hazard assessment.
Acknowledgements
This PorCORA work was conducted at MB Research Laboratories (MRL). MRL was solely responsible for the study design, the data collection, analysis, and interpretation of data, and the preparation of the final study report. BCOP test was performed at Institute for In Vitro Sciences (IIVS). The authors acknowledge the contributions of staff members at MB Research Laboratories who provided technical and quality assurance services in support of this PorCORA work.
Disclosure statement
No potential conflict of interest was reported by the author(s).
Additional information
Funding
References
- Herber RF, Duffus JH, Christensen JM, et al. Risk assessment for occupational exposure to chemicals. A review of current methodology (IUPAC technical report). Pure Appl Chem. 2001;73(6):993–1031. doi:10.1351/pac200173060993.
- Scazzola R, Boeije G, Bøtker Pedersen E, et al. Eye hazard classification according to UN GHS/EU CLP and the severity of eye symptoms caused by accidental exposures to detergents and cleaning products. Regul Toxicol Pharmacol. 2019;105:69–76. doi:10.1016/j.yrtph.2019.04.004.
- Parish ST, Aschner M, Casey W, et al. An evaluation framework for new approach methodologies (NAMs) for human health safety assessment. Regul Toxicol Pharmacol. 2020;112:104592. doi:10.1016/j.yrtph.2020.104592.
- Wang Q, Li X, Yang H, et al. In silico prediction of serious eye irritation or corrosion potential of chemicals. RSC Adv. 2017;7(11):6697–6703. doi:10.1039/C6RA25267B.
- Luechtefeld T, Maertens A, Russo DP, et al. Analysis of draize eye irritation testing and its prediction by mining publicly available 2008-2014 REACH data. ALTEX. 2016;33(2):123–134.
- Fischer I, Milton C, Wallace H. Toxicity testing is evolving!. Toxicol Res. 2020;9(2):67–80. doi:10.1093/toxres/tfaa011.
- Vij P, Donahue DA, Burke KP, et al. Lack of skin sensitization hazard potential for alpha-glycosyl isoquercitrin (AGIQ) utilizing the local lymph node assay. Toxicol Rep. 2022;9:1291–1296. doi:10.1016/j.toxrep.2022.05.021.
- OECD 2022. Test no. 492B: Reconstructed human cornea-like epithelium (RHCE) Test method for eye hazard identification, OECD guidelines for the testing of chemicals. Section 4. Paris: OECD Publishing.
- Guhad F. Introduction to the 3Rs (refinement, reduction, and replacement). Contemp Top Lab Anim Sci. 2005;44(2):58–59.
- OECD 2019. Test no. 492: Reconstructed human cornea-like epithelium (RhCE) test method for identifying chemicals not requiring classification and labelling for eye irritation or serious eye damage, OECD guidelines for the testing of chemicals. Section 4. Paris: OECD Publishing.
- OECD 2020. Test no. 437: Bovine corneal opacity and permeability test method for identifying i) Chemicals inducing serious eye damage and ii) chemicals not requiring classification for eye irritation or serious eye damage, OECD guidelines for the testing of chemicals. Section 4. Paris: OECD Publishing.
- OECD 2019. Test no. 496: in vitro macromolecular test method for identifying chemicals inducing serious eye damage and chemicals not requiring classification for eye irritation or serious eye damage, OECD guidelines for the testing of chemicals. Section 4. Paris: OECD Publishing.
- OECD 2020. Test no. 491: Short time exposure in vitro test method for identifying i) Chemicals inducing serious eye damage and ii) chemicals not requiring classification for eye irritation or serious eye damage, OECD guidelines for the testing of chemicals. Section 4. Paris: OECD Publishing.
- OECD 2021. Guideline no. 497: Defined approaches on skin sensitisation, OECD guidelines for the testing of chemicals. Section 4. Paris: OECD Publishing.
- Barile FA. Validating and troubleshooting ocular in vitro toxicology tests. J Pharmacol Toxicol Methods. 2010;61(2):136–145. doi:10.1016/j.vascn.2010.01.001.
- Wilson SL, Ahearne M, Hopkinson A. An overview of current techniques for ocular toxicity testing. Toxicology. 2015;327:32–46. doi:10.1016/j.tox.2014.11.003.
- Barroso J, Pfannenbecker U, Adriaens E, et al. Cosmetics Europe compilation of historical serious eye damage/eye irritation in vivo data analysed by drivers of classification to support the selection of chemicals for development and evaluation of alternative methods/strategies: the draize eye test reference database (DRD). Arch Toxicol. 2017;91(2):521–547. doi:10.1007/s00204-016-1679-x.
- Earl LK, Dickens AD, Rowson MJ. A critical analysis of the rabbit eye irritation test variability and its impact on the validation of alternative methods. Toxicol in Vitro. 1997;11(3):295–304. doi:10.1016/s0887-2333(97)00016-7.
- Spector J, Fernandez WG. Chemical, thermal, and biological ocular exposures. Emerg Med Clin North Am. 2008;26(1):125–136, vii. doi:10.1016/j.emc.2007.11.002.
- Willmann D, Fu L, Melanson SW. Corneal injury. StatPearls; 2017.
- Huhtala A, Salminen L, Tähti H, et al. Corneal models for the toxicity testing of drugs and drug releasing materials. Topics Multifunct Biomater Dev. 2008;1(2):1–23.
- Carathers MR, Vij P, Delacruz E, et al. The porcine corneal opacity and reversibility assay (PorCORA) and assessment of the drivers of classification with regards to ocular damage. Toxicol Suppl Toxicol Sci. 2018;162(1):119. doi:10.13140/RG.2.2.11147.57127.
- Piehl M, Gilotti A, Donovan A, et al. Novel cultured porcine corneal irritancy assay with reversibility endpoint. Toxicol in Vitro. 2010;24(1):231–239. doi:10.1016/j.tiv.2009.08.033.
- Foreman DM, Pancholi S, Jarvis-Evans J, et al. A simple organ culture model for assessing the effects of growth factors on corneal re-epithelialization. Exp Eye Res. 1996;62(5):555–564. doi:10.1006/exer.1996.0065.
- Richard NR, Anderson JA, Weiss JL, et al. Air/liquid corneal organ culture: a light microscopic study. Curr Eye Res. 1991;10(8):739–749. doi:10.3109/02713689109013868.
- Tanelian DL, Bisla K. A new in vitro corneal preparation to study epithelial wound healing. Invest Ophthalmol Vis Sci. 1992;33(11):3024–3028.
- Elsheikh A, Alhasso D, Rama P. Biomechanical properties of human and porcine corneas. Exp Eye Res. 2008;86(5):783–790. doi:10.1016/j.exer.2008.02.006.
- Obata H, Tsuru T. Corneal wound healing from the perspective of keratoplasty specimens with special reference to the function of the bowman layer and descemet membrane. Cornea. 2007;26(9 Suppl 1):S82–S9. doi:10.1097/ICO.0b013e31812f6f1b.
- Van den Berghe C, Guillet MC, Compan D. Performance of porcine corneal opacity and permeability assay to predict eye irritation for water-soluble cosmetic ingredients. Toxicol in Vitro. 2005;19(6):823–830. doi:10.1016/j.tiv.2005.04.006.
- Wilson SE, Hong JW. Bowman’s layer structure and function: critical or dispensable to corneal function? A hypothesis. Cornea. 2000;19(4):417–420. doi:10.1097/00003226-200007000-00001.
- U.N. Globally. Harmonized system of classification and labelling of chemicals (GHS). New York, Geneva: U.N. Globally; 2021. https://unece.org/sites/default/files/2021-09/GHS_Rev9E_0.pdf
- OECD 2016. Guidelines for histopathological evaluation of bovine corneas as an endpoint of the bovine corneal opacity and permeability (BCOP) assay. Institute for in vitro sciences, Inc.
- Vij P, Carathers M, Yasso B, et al. Resolving corrosive/severe irritant ocular classifications using an alternative dual ex vivo assay system. Toxicolog Suppl Toxicol Sci. 2017;156(1):414. doi:10.13140/RG.2.2.17334.16966.
- Varsho BJ, Vij P, Sergeyev D, et al. Identifying eye irritants (GHS category 2) using validated, nonanimal tests. Int. J. Toxicol. 2020;39(1):59. doi:10.13140/RG.2.2.15341.87520.
- Donahue DA, Avalos J, Kaufman LE, et al. Ocular irritation reversibility assessment for personal care products using a porcine corneal culture assay. Toxicol in Vitro. 2011;25(3):708–714. doi:10.1016/j.tiv.2010.12.008.
- Ditzel E, Vij P, Sergeyev D, et al. Use of porcine corneal opacity reversibility assay (PorCORA) to determine the GHS classification for an irritating mixture (cleaner emulsifier and degreaser). Toxicolog Suppl Toxicol Sci. 2019;168(1):438. doi:10.13140/RG.2.2.33125.06880.
- ECETOC. 1998. Eye irritation – reference chemicals data bank. Technical Report No. 48 (2), European Centre for Ecotoxicology and Toxicology of Chemicals, Brussels.
- Draize JH. Methods for the study of irritation and toxicity of substances applied topically to the skin and mucous membranes. J Pharmacol Exp Ther. 1944;82:377–390.