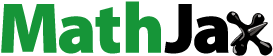
Abstract
Use of multi-angle sensors, which can perform ground observations in different view directions, provides an important technical means to acquire more comprehensive and detailed remote sensing information. This study explored the effect of angle changes on satellite-based aerosol optical depth (AOD) retrieval using 2016–2018 Multi-angle Imaging SpectroRadiometer (MISR) data in the Yangtze River Delta. The Moderate resolution Imaging Spectroradiometer (MODIS) surface reflectance determined by the MODIS V5.2 algorithm and MODIS BRDF data are used to obtain MISR surface reflectance at different angles after a spectral conversion between MODIS and MISR, and then MISR AOD with different angles were retrieved based on the MISR surface reflectance at different angles. Moreover, the errors and their formation mechanism in retrieving AOD using MISR remote sensing data at different angles were analyzed based on the Global Aerosol Robotic Network (AERONET) ground observations. We used six indicators, including correlation coefficient (R), significant level (P value), mean absolute error (MAE), mean relative error (MRE) root mean square error (RMSE) and relative mean bias (RMB), and AERONET AOD is used to verify the MISR AOD. The results show that at Taihu and Xuzhou stations, the highest R values are 0.81 (Aa AOD) and 0.75 (An AOD), and the minimum RMSE values at Taihu and Xuzhou-CUMT stations are 0.11 (Aa AOD) and 0.20 (An AOD), respectively. Incorrect surface reflectance measurement is the root cause of the angle effect in MISR AOD retrieval. With the same surface reflectance deviation, the larger the MISR observation angle, the closer the retrieved MISR AOD is to the AOD observed by AERONET. The AOD retrieval of the forward observation angle is closer to AERONET AOD than that of backward observation angle, and the retrieved error is greatest at the vertical angle (nadir). Therefore, sensors for vertical ground observation are not suitable for AOD retrieval, and sensors in non-vertical directions, especially forward to the ground, are more favorable to MISR AOD retrieval.
1. Introduction
Aerosol, an important atmospheric component, has a major impact on the climate system as it affects the radiation balance between the ground surface and the atmosphere through absorbing and scattering solar radiation (Cohen et al., Citation2010; Tang et al., Citation2017). Aerosol particles are atmospheric pollutants and can enter the human respiratory system, which can substantially impact human health. Many human respiratory diseases and cardiovascular diseases are closely related to aerosol pollutant (Monique et al., Citation2013; Lee et al., Citation2017).
Aerosol optical depth (AOD), which describes the attenuation of light, is one of several critical parameters describing the atmospheric column (Shinozuka et al., Citation2010). At present, the main methods for AOD retrieval can be divided into two categories, i.e. ground observation and satellite remote sensing (Loehnert et al., Citation2015; Yan et al., Citation2020). The former generally uses the ground monitoring network to validate the authenticity of the latter. Relative to ground observations, remote sensing monitoring of atmospheric aerosols has the characteristics of wide coverage, fast information acquisition and large amount of information, and exhibits obvious advantages in continuity, space and trend analysis.
Use of multi-angles is the popular trends for remote sensing sensors in acquiring more detailed and comprehensive observational information. Multi-angle Imaging SpectroRadiometer (MISR) is one instrument that provides remote sensing data for multi-angle observation. It is carried on the Terra satellite and consists of 4 bands and 9 Charge Coupled Device (CCD) cameras, which can provide continuous ground coverage at nine angles. MISR can obtain multi-angle observations at the same location within 7 minutes, which greatly improves the accuracy of retrieval information (Diner et al., Citation1991; Prasad and Singh, Citation2007). The MISR aerosol product inversion algorithm strictly selects applicable models from a series of preset models representing global aerosol types. These models include fine-mode aerosol mixtures with different particle size distributions and single scattering albedo, coarse Modal aerosol and non-spherical dust. In order to distinguish the contribution of the surface and the atmosphere from the top layer of the atmosphere, MISR uses an empirical orthogonal function to describe the change of the contribution ratio of the atmosphere and the surface radiation with different observation zenith angles, especially in the bright surface and sandy areas (Garay et al., Citation2018, Citation2020). Some existing studies have focussed on aerosol characteristics using MISR multi-band data. For example, Abdou et al. (Citation2005) conducted an comparison between MISR AOD and Moderate resolution Imaging Spectroradiometer (MODIS) AOD and verified the retrieval accuracy using Global Aerosol Robotic Network (AERONET) observations, and the results suggested that on land, MISR AOD is approximately 35% smaller than MODIS AOD in the blue band, while MISR AOD is approximately 10% smaller than MODIS AOD in the red band, and the MISR AOD values are overall close to the AERONET AOD observational values. Through a comparative study of the MISR AOD and AERONET AOD in desert areas, Martonchik et al. (Citation2004) found that MISR can retrieve AOD on the surface with relatively high reflectance of visible light and near infrared bands. Furthermore, Xu et al. (Citation2005) improved the accuracy of retrieving AOD through integrating the radiation and geometric attributes of MODIS and MISR. Wei et al. (Citation2019) proposed a regional robust high-resolution aerosol retrieval algorithm for MODIS images in eastern China. The results show that this algorithm performs well over dark vegetated and bright urban surfaces. Tian et al. (Citation2020) developed a new aerosol retrieval algorithm by considering the effects of surface BRDF using the RossThick-LiSparse model over land for MODIS images. The results show that the retrieved AOD values are highly consistent with AERONET AOD values, the data quality of AOD retrieval has been largely improved compared with those without considering the effects of surface BRDF, especially over bright urban surfaces.
The purpose of this study is to investigate the effect of angle changes on the AOD retrieval based on the data from nine angles of the MISR level 1B2 Terrain Data (MISR L1B2T) blue band in the Yangtze River Delta during the period of 2016–2018. First, the MODIS surface reflectance determined by the MODIS V5.2 algorithm and MODIS BRDF data are used to obtain MISR surface reflectance at different angles after a spectral conversion between MODIS and MISR. Subsequently, MISR AODs with different angles were retrieved based on the MISR surface reflectance at different angles. Finally, AERONET ground-based observation data was used to evaluate the accuracy of the AOD retrieval at different angles. Meanwhile, error characteristics and the mechanism of formatting the errors in retrieving AOD using MISR data at different angles were further analyzed.
2. Study area and datasets
2.1. Study area
The Yangtze River Delta area is situated in the downstream Yangtze River in the eastern coastal region of China. It is a major agricultural area and an important economic core area in China (). There are rapid urbanization and developed industrialization with the characteristics of high energy consumption and high pollution emissions (Zhou et al., Citation2013), which have caused health burdens related to severe environmental pollution, such as frequent occurrence of smog and poor air quality. The Yangtze River Delta is one of the areas where aerosols increase rapidly. Therefore, the study of the aerosol optical characteristics in the Yangtze River Delta is of great significance for the analysis of regional climate effects and atmospheric pollution prevention.
2.2. Datasets
2.2.1. MISR
The Earth's near polar orbit satellite Terra was launched on December 18, 1999 as part of NASA's Earth Observing System (EOS) program. MISR has four bands including blue, green, red, and near-infrared, with central wavelengths of 446 nm, 558 nm, 672 nm, and 867 nm, respectively (Kahn et al., Citation2007). The nine observation cameras are An, Af, Aa, Bf, Ba, Cf, Ca, Df, Da (n, f, and a represent the nadir, forward, and backward, respectively; A, B, C, and D represent four lens designs whose focal lengths increase with increasing view angle, respectively (Da: −70.5°, Ca: −60.0°, Ba: −45.6°, Aa:-26.1°, An:0.0°, Af:26.1°, Bf: 45.6°, Cf: 60.0°, Df: 70.5°) (Diner et al., Citation1998; Martonchik et al., Citation2002). The nine angles are oriented along the direction of the satellite's flight path. In general, more oblique angles provide greater sensitivity to the effects of atmospheric aerosols. Clouds and medium angles are used for surface observations. In about seven minutes, all images of the nine angles at the same location can be acquired, the swath is 376 km for the nadir camera and 413 km for the other 8 cameras (Angal et al., Citation2016). The repeat period of the MISR image is 16 days, and its spatial resolution is 275 m and 1100 m ().
Table 1. The spatial resolution of MISR data (m).
This study selected MISR L1B2T grade products, which have been subjected to radiation calibration and geographic projection processing. Our study area spans five paths ( from right to left are 118, 119, 120, 121, and 122 in turn). A complete path comprises of 180 blocks. 64th and 65th blocks are selected for this study area. The presence of clouds can affect the AOD retrieval, and therefore 80 scene images with less cloud amount are chosen after screening, then we use the blue band to determine the threshold for removing clouds (if cloud pixels are present) from the corresponding image. After repeated experiments, a dynamic threshold method was finally applied to the blue band to remove 9-angle cloud pixels. The HEGTool tool was employed to extract the required blocks, and the SOM projection was converted to UTM projection, and the image was resampled using ArcGIS so that the spatial resolutions of MISR data at nine angles are the same (all are 1100 m).
2.2.2. MODIS L1B data
The Terra satellite is a satellite in the Earth Observing System (EOS) program implemented by NASA, and MODIS is its main sensor. MODIS has 36 spectral bands with a spectral range of 0.4 to 14.4 μm. It provides data on three resolutions of 250, 500, and 1000 m. Due to the wide swath of MODIS, the Yangtze River Delta region is typically observed on a daily basis. MODIS provides a variety of different products. The data used in this study are MODIS L1B data after radiation calibration, including radiation data (MOD02) and geolocation data (MOD03). The radiation data are the reflectivity data of MOD02HKM from 1 to 7 bands, and the geolocation data mainly includes the longitude, latitude, solar zenith angle, solar azimuth angle, satellite view zenith angle, and satellite azimuth of each pixel in the MODIS image. The spatial resolutions are uniformly resampled to 1100 m.
2.2.3. MODIS BRDF data
The BRDF model parameter product used in this study is MCD43A1 (C6), which belongs to the MODIS Level 3 land standard product. MCD43A1 is fitted by the observation data of Terra and Aqua in 16 days. If there is clear sky observation data accumulated in at least 7 in 16 days, the surface albedo can be obtained by full BRDF inversion (Gao et al., Citation2002), and when the accumulated days are 3–7 days, the original prior information in the BRDF parameter database is corrected using the high-quality multi-angle clear-air observation data of the pixel to obtain the BRDF parameters that are consistent with the actual situation of the pixel, which is called magnitude BRDF inversion (Abelleyra and Verón, Citation2014). In addition, MCD43A1 products provide weight coefficients for an-isotropic scattering, volume scattering, and geometrical optical scattering, as well as quality control information, and the products include MODIS bands from 1 to 7, visible light (0.3–0.7 μm), near-infrared (0.7–5.0 μm) and short-wave infrared (0.3–5.0 μm).
2.2.4. AERONET
AERONET is a global network of ground-based sun photometers. The CIMEL318 instrument is utilized to observe aerosol optical characteristics. AERONET provides near-truth data for satellite and model verification at the site locations. The center wavelengths of the CIMEL318 instrument are 340 nm, 380 nm, 440 nm, 500 nm, 670 nm, 870 nm, 936 nm, 1020 nm, and 1640 nm. The measured values of the 936 nm channel are used to obtain the total water vapor content in the atmosphere. The measured values of the other channels are used to retrieve AOD. The retrieval error is about 0.01–0.02 (Eck et al., Citation1999). AERONET aerosol product quality levels are divided into three categories: Level 1.0 is the raw data without cloud screening, and level 1.5 is the cloud detection processing data, and level 2.0 data removes cloud pollution and has quality assurance data. The number of samples of data available at AERONET 2.0 level is very limited, and thus to ensure enough verification data, we uses AERONET 1.5 level data (AERONET version 3) from 2016 to 2018 to verify the results of remote sensing retrieval. We used two stations in the Yangtze River Delta region that continue to provide observation data, i.e. Taihu (31.421oN, 120.215oE) and Xuzhou-CUMT (34.217oN, 117.142oE) stations (), which were used to verify the retrieved MISR AOD.
To verify the accuracy of retrieving AOD, we took the AOD observed by AERONET as the real value and conducted a comparison between the real value and the retrieved MISR AOD. The pixels of the AERONET site were taken as the standard, and the MISR AOD values corresponding to each site were calculated during the period of 2016–2018 (). The AOD of the AERONET site within 30 minutes before and after the satellite transit was averaged as the accurate verification value (Goloub et al., Citation1999). Since the MISR AOD value was obtained at 550 nm, and the AERONET data has no AOD observation value in this band. Therefore, this study used the Angstrom equation to fit the AERONET data to invert the AERONET AOD at 550 nm (Ångström, Citation1964). The equation is as follows:
(1)
(1)
where
represents the AOD at wavelength
represents the atmospheric turbidity index (Tian et al., Citation2018). It has a certain relationship with the total number of aerosol particles, particle spectral distribution and refractive index, and the value is between 0–0.5.
stands for clean atmosphere,
represents a relatively turbid atmosphere.
represents the wavelength index of Angstrom, and the size is mainly affected by the average radius of the aerosol. Normally, the value is between 0–2 and the average value is about 1.3. Two parameters
and
can be obtained by fitting the AOD observed at multiple bands, and then get the AOD at any wavelength under the same conditions.
3. Methodology
3.1. AOD retrieval basics
To retrieve the AOD of the sensor, the apparent reflectance of the upper atmosphere boundary of the satellite sensor needs to be known. Assuming that the underlying surface is a Lambertian surface and the atmosphere is uniform, the apparent reflectivity of the upper boundary of the atmosphere obtained by the satellite sensor can be calculated by calculation (She et al., Citation2019; Kaufman et al., Citation1997).
In practice, the acquisition of AOD is based on the assumption of different aerosol patterns and observed geometric angles. In this study, continental aerosols were selected and a questionnaire was established. AOD is obtained through a lookup table. The retrieval algorithm flow is shown in .
3.2. Determination of surface reflectance
Satellite-based aerosol monitoring is essentially the process of removing surface reflection noise from satellite signals to obtain aerosol information. When the local surface reflectance is small and relatively easy to determine, the main contribution of comes from the atmosphere, and electromagnetic radiation in the visible light band is most significantly affected by aerosols. This is the main reason why aerosol remote sensing on the ocean can achieve higher accuracy, and it is also the direct reason for choosing dense vegetation areas over the land to use red and blue bands for retrieval (Li et al., Citation2009; She et al., Citation2019).
In this research, the MODIS surface reflectance determined by the MODIS V5.2 algorithm and BRDF parameter products were used to obtain the MODIS surface reflectance at different MISR angles (He et al., Citation2015).
(2)
(2)
where
and
represent the BRDF at the MISR and MODIS angles, respectively.
and
are solar zenith angle, satellite view zenith angle and view-sun relative azimuth angle, respectively.
is the surface reflectance of MODIS under MISR observation geometry.
is the surface reflectance of MODIS obtained by MODIS V5.2 algorithm.
EquationEquation (2)(2)
(2) only obtains the MODIS surface reflectance at different MISR angles. To retrieve MISR AOD, it needs to converts to MISR surface reflectance.
Due to the spectral response functions of different satellite sensors are different, the same feature has different reflection characteristics in different sensors. To eliminate the spectral differences between the sensors, Wang et al. (Citation2012) proposed a method of correcting the reflectance of the MODIS blue band on the CCD sensor using typical ground object reflection data measured by a field spectrometer. Sun et al. (Citation2010) established a reflectance conversion model between MODIS and HJ-1/CCD data.
In this research, the standard spectral database of ENVI software was used to select spectral data of 28 typical features. The surface reflectance of different characteristics of MODIS and MISR blue bands was calculated using EquationEq. (3)(3)
(3) (Li et al., Citation2009):
(3)
(3)
where λ1 and λ2 are the upper and lower limits of the integral wavelength, respectively.
is the band response value when the sensor with a wavelength of
is surface reflectance of the corresponding band.
By fitting the surface reflectance of different characteristics of MODIS and MISR blue band obtained by EquationEq. (3)(3)
(3) , a spectral conversion model of MODIS and MISR blue band was established. The surface reflectance of MODIS and MISR has a strong correlation (), and the constructed conversion model is shown in EquationEq. (4)
(4)
(4) . It is suggested that the surface reflectance of the MISR blue band obtained by the band conversion is slightly lower than that of the MODIS blue band.
(4)
(4)
The surface reflectance at the MISR angle obtained by EquationEq. (2)(2)
(2) is converted into the surface reflectance required for retrieving MISR AOD through EquationEq. (4)
(4)
(4) .
4. Result and discussion
4.1. Retrieved AOD and verification
The results of AOD retrieval of 1.1 km at nine angles in blocks of 64 and 65 of path 122 on June 12, 2018 were shown in ( is a 9-angle false color images of MISR data). It was shown that the overall aerosol concentration in the southern and northern areas of the Yangtze River Delta was relatively high on that day. The AOD of the three angles (Aa, An and Af) in the central region were higher than that of the other six angles (Da, Ca, Ba, Bf, Cf and Df). By comparing the spatial distribution of MOD04_3k AOD products () (Resampling the spatial resolution of MOD04_3k AOD products is consistent with the spatial resolution of MISR AOD), it can be seen that the retrieval results of MISR AOD are consistent with MODIS AOD in terms of spatial distribution. It can be seen from the time-series type plot of the 9 angles that the bias of the three angles of Da, Ca and Ba is larger, while the bias of the three angles of Bf, Cf and Df is smaller ().
Comparison between the retrieved MISR AOD and the ground-observed values was performed for the two sites (Taihu and Xuzhou-CUMT) (). The Taihu station contains 22 to 23 sample points, and the Xuzhou-CUMT station contains 32 to 44 sample points. At the Taihu site (), the correlation of MISR forward observation angles (including Af, Bf, Cf, Df) are generally higher than that of the backward (including Aa, Ba, Ca, Da) and the vertical angles (An), Aa exhibit the highest correlation (R = 0.81) and An has the lowest correlation (R = 0.45). At the Xuzhou-CUMT site (), the correlations of the MISR forward observation angles are relative higher than that of other angles, and the correlation at An is the highest (R = 0.75) and the correlation at Da is the lowest (R = 0.45). The fitted line of MISR forward observation angles (including Af, Bf, Cf, Df) is more closer to the 1: 1 line. Most of the scattered points are above the 1:1 line, indicating that the MISR AOD retrieval is generally higher than the AERONET observations. From verification results of MOD04_3k AOD and AERONET AOD, MOD04_3k AOD is close to the forward angle of MISR AOD ().
4.2. Error of AOD retrieval in different angles
To analyze the errors of the retrieved MISR AOD at the nine angles, the mean absolute error (MAE) and the mean relative error (MRE) were calculated for Taihu and Xuzhou-CUMT, respectively (). Equations of MAE and MRE are shown in (5) and (6), respectively. It is shown that the error of retrieving MISR AOD at Da and An angles are large, and the average absolute errors and average relative errors of the forward observation angles (Bf, Cf, and Df) are smaller than the backward observation angles and nadir angle An. Therefore, AOD retrieval of Bf, Cf and Df angles is better than that of other angles.
(5)
(5)
(6)
(6)
where
is MAE,
is the retrieved MISR AOD, and
is the AERONET AOD.
Furthermore, we calculated the average of MISR surface reflectance, apparent reflectance, MISR AOD and AERONET AOD at Taihu and Xuzhou-CUMT, in order to examine the reasons for the different errors in retrieving MISR AOD at the 9 angles. We also used the obtained the average value of apparent reflectance and AERONET AOD, which has been performed atmospheric correction by the Second Simulation of a Satellite Signal in the Solar Spectrum (6S), to calculate the surface reflectance with atmospheric correction (). It suggests that the MISR surface reflectance value determined in Sec. 3.2. is lower than that obtained by atmospheric correction. Therefore, retrieved MISR AOD is higher than the AERONET AOD. Due to the relatively small deviation between the surface reflectance at the MISR forward observation and the surface reflectance value obtained by atmospheric correction, the deviations between MISR AOD and the AERONET AOD from the forward observation angles are relatively small ().
Figure 12. The influence of geometric conditions on AOD. The Y-axis is the average of all (Surface reflectance, atmospheric correction surface reflectance, AOD, AERONET AOD and apparent reflectance) values that match the location of the site at 9 angles from 2016 to 2018.

Table 2. The difference between the MISR surface reflectance and reflectance with atmospheric correction and the deviations of MISR AOD and AERONET AOD at different angles.
It is worth noting that the difference between the MISR surface reflectance at An angle and the An surface reflectance obtained from atmospheric correction and the difference between the MISR surface reflectance at Df angle and the Df surface reflectance obtained from atmospheric correction are similar. However, compared with AERONET AOD, MISR AOD at An angle deviates greatly from AERONET AOD than MISR AOD at Df angle (). To investigate the impact of MISR surface reflectance deviation on MISR AOD errors at different angles, the average values of AERONET AOD and MISR surface reflectance obtained from the atmospheric corrections of Taihu and Xuzhou-CUMT sites were used to simulate the apparent reflectance through the 6S. When the surface reflectances at the nine angles of Taihu and Xuzhou-CUMT sites were respectively reduced by 0.0458 and 0.0519, the AOD of MISR was retrieved using the simulated apparent reflectance (). When the same decrease in surface reflectance at the nine angles, the AOD values in different angles are different, and they are no longer the same value (average of AERONET AOD). Therefore, the inaccurate determination of surface reflectance is the root cause of MISR AOD retrieval angle effect. With the same surface reflectance deviation, the larger the MISR observation angle, the closer the retrieved MISR AOD to the AERONET observed AOD. Moreover, the AOD of the forward observation angles is better than that of the backward observation angles. The AOD error is greatest at the vertical angle (An). Therefore, sensors for vertical ground observation are not suitable for AOD retrieval, and sensors for non-vertical observation, especially forward ground observation, are more favorable for AOD retrieval.
5. Conclusions
This study explored the effects of angle changes on AOD satellite remote sensing retrieval in the Yangtze River Delta using 2016–2018 MISR data. Based on the MODIS surface reflectance determined by the MODIS V5.2 algorithm and MODIS BRDF data, we provide the first surface reflectances of MISR at different angles in retrieving MISR AOD through the spectral conversion between MODIS and MISR. Moreover, the errors and their formation mechanism in retrieving AOD from MISR data at different angles were analyzed using the AERONET ground-based observation data. The results suggested that the inaccurate determination of ground surface reflectance is the root cause of the angle effect in retrieving AOD using MISR. At the same surface reflectance deviation, the larger the MISR observation angle, the closer the retrieved MISR AOD to the AERONET observed AOD. The retrieved MISR AOD at forward observation angles is significantly better than that at backward observation angles, and the maximum AOD error is found at a vertical angle (An). Therefore, sensors for vertical ground observation are not suitable for AOD retrieval, and sensors for non-vertical observation, especially forward ground observation, are more favorable for the MISR AOD retrieval. These findings have important significance in AOD retrieval using multi-angle remote sensing observations.
Acknowledgments
All data used in this study are available upon request to the corresponding author ([email protected]). This MISR data used in this study are acquired from https://eosweb.larc.nasa.gov/project/misr/misr_table. The MODIS data are acquired from https://misr.jpl.nasa.gov/getData/accessData. The AERONET data are acquired from https://aeronet.gsfc.nasa.gov.
Disclosure statement
No potential conflict of interest was reported by the authors.
Additional information
Funding
References
- Abdou, W. A., Diner, D. J., Martonchik, J. V., Bruegge, C. J., Kahn, R. A. and co-authors. 2005. Comparison of coincident multiangle imaging spectroradiometer and moderate resolution imaging spectroradiometer aerosol optical depths over land and ocean scenes containing aerosol robotic network sites. J. Geophys. Res. 110, 1–12.
- Abelleyra, D. and Verón, S. R. 2014. Comparison of different Brdf correction methods to generate daily normalized Modis 250m time series. Remote Sens. Environ. 140, 46–59. doi:https://doi.org/10.1016/j.rse.2013.08.019
- Angal, A., Xiong, X. and Wu, A. 2016. Monitoring the on-orbit calibration of terra MODIS reflective solar bands using simultaneous terra MISR observations. IEEE Trans. Geosci. Remote Sens., 1–12.
- Ångström, A. 1964. The parameters of atmospheric turbidity. Tellus. 16, 64–75.
- Cohen, J. B., Wang, C. and Prinn, R. G. 2010. The impact of detailed urban-scale processing on the aerosol direct effect and its impacts on the climate. In: AGU Fall Meeting. Abstracts.
- Diner, D. J., Bruegge, C. J., Martonchik, J. V., Bothwell, G. W., Danielson, E. D. and co-authors. 1991. A multi-angle imaging spectroradiometer for terrestrial remote sensing from the earth observing system. Int. J. Imaging Syst. Technol. 3, 92–107. John Wiley & sons Inc, USA, NJ. doi:https://doi.org/10.1002/ima.1850030206
- Diner, D. J., Beckert, J. C., Reilly, T. H., Bruegge, C. J., Conel, J. E. and co-authors. 1998. Multi-angle imaging SpectroRadiometer (MISR) instrument description and experiment overview. IEEE Trans. Geosci. Remote Sens. 36, 1072–1087. doi:https://doi.org/10.1109/36.700992
- Eck, T. F., Holben, B. N., Reid, J. S., Dubovik, O., Smirnov, A. and co-authors. 1999. Wavelength dependence of the optical depth of biomass burning, urban, and desert dust aerosols. J. Geophys. Res. 104, 31333–31349. doi:https://doi.org/10.1029/1999JD900923
- Gao, F., Jin, Y., Schaaf, C. B. and Strahler, A. H. 2002. Bidirectional ndvi and atmospherically resistant BRDF inversion for vegetation canopy. IEEE Trans. Geosci. Remote Sens. 40, 1269–1278.
- Garay, M. J., Bull, M. A., Nastan, A. M., Witek, M. L., Seidel, F. C. and co-authors. 2018. Data Product Specification for the MISR Level 2Aerosol Product.
- Garay, M. J., Witek, M. L., Kahn, R. A., Seidel, F. C., Limbacher, J. A. and co-authors. 2020. Introducing the 4.4 km spatial resolution multi-angle imaging SpectroRadiometer (MISR) aerosol product. Atmos. Meas. Tech. 13, 593–628. doi:https://doi.org/10.5194/amt-13-593-2020
- Goloub, P., Tanre, D., Deuze, J. L., Herman, M., Marchand, A. and co-authors. 1999. Validation of the first algorithm applied for deriving the aerosol properties over the ocean using the polder/adeos measurements. IEEE Trans. Geosci. Remote Sens. 37, 1586–1596. doi:https://doi.org/10.1109/36.763270
- He, J., Zha, Y., Zhang, J., Gao, J., Li, Y. and co-authors. 2015. Retrieval of aerosol optical thickness from hj-1 CCD data based on MODIS-derived surface reflectance. Int. J. Remote Sens. 36, 882–898. doi:https://doi.org/10.1080/01431161.2014.999171
- Kahn, R. A., Garay, M. J., Nelson, D. L., Yau, K. K., Bull, M. A. and co-authors. 2007. Satellite-derived aerosol optical depth over dark water from MISR and MODIS: Comparisons with aeronet and implications for climatological studies. J. Geophys. Res. 112, D18205. doi:https://doi.org/10.1029/2006JD008175.
- Kaufman, Y. J., Tanré, D., Remer, L. A., Vermote, E. F., Chu, A. and co-authors. 1997. Operational remote sensing of tropospheric aerosol over land from EOS moderate resolution imaging spectroradiometer. J. Geophys. Res. 102, 17051–17067. doi:https://doi.org/10.1029/96JD03988
- Lee, H. H., Iraqui, O., Gu, Y., Yim, S. H. L. and Wang, C. 2017. Impacts of particulate pollution from fossil fuel and biomass burnings on the air quality and human health in Southeast Asia. In: AGU Fall Meeting. Abstracts.
- Li, Z., Zhao, X., Kahn, R., Mishchenko, M., Remer, L. and co-authors. 2009. Uncertainties in satellite remote sensing of aerosols and impact on monitoring its longterm trend: A review and perspective. Ann. Geophys. 27, 2755–2770. doi:https://doi.org/10.5194/angeo-27-2755-2009
- Loehnert, U., Ebell, K. and Orlandi, E. 2015. Improving the retrieval of atmospheric stability indices by combining ground-based and satellite remote sensing. In: AGU Fall Meeting. Abstracts.
- Martonchik, J. V., Diner, D. J., Crean, K. A. and Bull, M. A. 2002. Regional aerosol retrieval results from MISR. IEEE Trans. Geosci. Remote Sens. 40, 1520–1531. doi:https://doi.org/10.1109/TGRS.2002.801142
- Martonchik, J. V., Diner, D. J., Kahn, R. A., Gaitley, B. J. and Holben, B. N. 2004. Comparison of MISR and AERONET aerosol optical depths over desert sites. Geophys. Res. Lett. 31, 171–184.
- Monique, W., Amanda, V., Krassimir, B., Sabrina, L., Prue, T. and co-authors. 2013. Metal and silicate particles including nanoparticles are present in electronic cigarette cartomizer fluid and aerosol. PLoS One. 8, e57987.
- Prasad, A. K. and Singh, R. P. 2007. Comparison of MISR-MODIS aerosol optical depth over the Indo-Gangetic basin during the winter and summer seasons (2000–2005). Remote Sens. Environ. 107, 109–119. doi:https://doi.org/10.1016/j.rse.2006.09.026
- She, L., Xue, Y., Yang, X., Leys, J., Guang, J. and co-authors. 2019. Joint retrieval of aerosol optical depth and surface reflectance over land using geostationary satellite data. IEEE Trans. Geosci. Remote Sens. 57, 1489–1501. doi:https://doi.org/10.1109/TGRS.2018.2867000
- Shinozuka, Y., Redemann, J., Livingston, J. M., Russell, P. B. and Mcarthur, L. J. B. 2010. Airborne observation of aerosol optical depth during ARCTAS: Vertical profiles, inter-comparison, fine-mode fraction and horizontal variability. Atmos. Chem. Phys. 10, 18315–18363.
- Sun, L., Sun, C. K., Liu, Q. H. and Zhong, B. 2010. Aerosol optical depth retrieval by HJ-1/CCD supported by MODIS surface reflectance data. Sci. China Earth Sci. 53, 74–80. doi:https://doi.org/10.1007/s11430-010-4134-5
- Tang, W., Yang, K., Qin, J., Niu, X., Lin, C. and co-authors. 2017. A revisit to decadal change of aerosol optical depth and its impact on global radiation over china. Atmos. Environ. 150, 106–e115. doi:https://doi.org/10.1016/j.atmosenv.2016.11.043
- Tian, X., Liu, S., Sun, L. and Qiang, L. 2018. Retrieval of aerosol optical depth in the arid or semiarid region of Northern Xinjiang, China. Remote Sens. 10, 197. doi:https://doi.org/10.3390/rs10020197
- Tian, X., Liu, Q., Gao, Z., Wang, Y. and Wei, J. 2020. Improving MODIS aerosol estimates over land with the surface BRDF reflectances using the three-dimensional discrete cosine transform and RossThick-LiSparse models. IEEE Transactions on Geoscience and Remote Sensing.
- Wang, Z., Li, Q., Wang, Q., Li, X., Chen, L. and co-authors. 2012. HJ-1 terrestrial aerosol data retrieval using deep blue algorithm. J. Remote Sens. 16, 596–610.
- Wei, J., Li, Z., Peng, Y., Sun, L. and Yan, X. 2019. A regionally robust high-spatial-resolution aerosol retrieval algorithm for MODIS images over Eastern China. IEEE Trans. Geosci. Remote Sens. 57, 4748–4757. doi:https://doi.org/10.1109/TGRS.2019.2892813
- Xu, Q., Obradovic, Z., Han, B., Li, Y., Braverman, A. and co-authors. 2005. Improving aerosol retrieval accuracy by integrating AERONET, MISR and MODIS data. In: 8th IEEE International Conference on Information Fusion, 10, 1–7.
- Yan, X., Luo, N., Liang, C., Zang, Z., Zhao, W. and co-authors. 2020. Simplified and fast atmospheric radiative transfer model for satellite-based aerosol optical depth retrieval. Atmos. Environ. 224, 117362. doi:https://doi.org/10.1016/j.atmosenv.2020.117362
- Zhou, S., Dai, J. and Bu, J. 2013. City size distributions in China 1949 to 2010 and the impacts of government policies. Cities. 32, S51–S57. doi:https://doi.org/10.1016/j.cities.2013.04.011