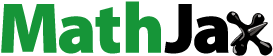
ABSTRACT
The present study aimed to investigate the efficacy of flavonoids isolated from the fruit of Terminalia chebula against the hydrogen peroxide-induced DNA damage. Five fractions were screened and were compared for their in vitro antioxidant activity by DPPH method and DNA protection activity by DNA nicking assay. All the extracts showed excellent antioxidant activity with IC50 values ranging from 11.23 ± 0.18 to 36.70 ± 0.12 µg/ml. The band intensity of supercoiled, circular and linear DNA was measured in DNA nicking assay and it is very clear that the addition of extract significantly inhibited the formation of circular and linear forms of DNA. Methanol–Water 1:1 fraction which showed the highest activity in antioxidant and DNA protection was subjected to structural characterization using different spectroscopic techniques. The structure of the compound was identified as a derivative of luteolin. The result presented in this work strongly supports the hypothesis that DNA protection activities were positively associated with the antioxidant property.
1. Introduction
DNA, the genetic material of a living organism is subjected to spontaneous attack by both exogenous and endogenous agents. UV light, ionizing radiation, toxic chemicals, viruses, smoking, etc are the main exogenous factors, while the major endogenous factors are reactive oxygen species produced from normal metabolic by-products [Citation1]. The damages induced by endogenous sources occur at a high frequency compared with exogenous damages [Citation2].
These damages can be repaired by various pathways like nucleotide excision repair, base excision repair, mismatch repair and double-strand break repair [Citation3]. Failure and defect in any of these repair pathways can lead to mutations and other health disorders like ageing, cancer, diabetes mellitus, reperfusion disorder, atherosclerosis, cataract, pulmonary dysfunction and radiation damage [Citation4].
Herbal medicines are currently in demand since the medicinal plants are easily available, cheaper and safe to be consumed as compared to modern drugs. Since the search for the cure is a long process, scientists have turned to prevention rather than a cure. Phytochemicals have a useful role in the prevention of diseases such as cancer, diabetes, cardiovascular diseases [Citation5], neurodegenerative diseases and inflammation [Citation6]. A large variety of secondary metabolites like alkaloids, terpenes, saponines and polyphenols are synthesized exclusively by plants for various purposes, such as a chemical defense against microbial attack, toxicant, and repellent for insects, and attractants for pollinators [Citation7]. Among these, flavonoids are the largest, most varied and most studied group of polyphenolic phytochemicals. Flavonoids are found to be rich in fruits, vegetables and wines [Citation8]. These are important components of both human and animal diets and are safe to be consumed [Citation9]. Flavonoids are categorized into flavonols, flavones, flavanones, isoflavones, catechins, anthocyanidins and chalcones [Citation10]. They exhibit a wide variety of biological activities like antiviral, antibacterial, anti-inflammatory, anti-carcinogenic, antiplatelet antioxidant and DNA protection activities [Citation11,Citation12].
Modern medicine has become popularized due to its precise and quick action. Even though great advances are observed in modern medicines, most of the drugs available are of high cost and toxic to the body and hence they are not safe at all. Nowadays herbal medicines are in great demand in the developing countries also not because they are inexpensive, but also for better cultural acceptability, better compatibility and minimal or no side effects [Citation13]. Natural bioactive compounds either as pure or as crude provide unique opportunities for new drug discoveries because of the structural and biological diversity of their constituents. A wide range of sophisticated technologies is available now to isolate and characterize the active components from the plant extracts and deduction of the actual mechanism behind their activities. Scientific evidence confirms the use of many flavonoids as protective agents against DNA damage [Citation14,Citation15]. Flavonoids as antioxidants can scavenge reactive oxygen species and thus prevent vascular diseases, cancer, cellular damage, etc [Citation16,Citation17]. Hence, the discovery of new compounds with excellent radical scavenging ability would be significant for some diseases relating to oxidative damage.
Terminalia chebula belongs to Combretaceae family is widely distributed in tropical areas of the world. Homeostatic, antitussive, laxative, diuretic, cardiotonic and antiviral activities of T. chebula has been reported [Citation18,Citation19]. It is widely used as traditional medicine to cure several ailments such as fever, cough, gastroenteritis, skin diseases, diarrhoea, candidiasis, urinary tract infection and wound infections [Citation20]. The fruits are being enriched with phytoconstituents to cure various diseases like cancer, cardiovascular, digestive and pathogenic bacteria [Citation21]. The fruits are also reported to have antibacterial [Citation22,Citation23] antidiabetic [Citation24], antioxidant, cytoprotective and hepatoprotective activity [Citation25]. Phytochemical evaluations of T. chebula have revealed the presence of alkaloids, tannins, carbohydrates, flavonoids, phenols, terpenoids, etc [Citation26].
The present study investigated the in vitro antioxidant and DNA protection activity of flavonoids isolated from T. chebula fruit. The flavonoid fraction which showed highest activity was subjected to the structural characterization using several spectral techniques such as UV-Visible, FT-IR, 1H NMR and Mass spectra.
2. Material and methods
2.1. Preparation of extracts
Fresh fruits of T. chebula were collected from Kannur district of Kerala, India and were taxonomically identified by Dr Sujanapal P, Scientist, Kerala Forest Research Institute (KFRI), Thrissur, Kerala, India. They were washed with running water followed by double distilled water. It was air dried in the dark and was powdered coarsely. Shade-dried fruit powder of the plant was weighed and extracted with 80% methanol using the Soxhlet method, for 24 h. Decanted the methanol extract and the extract was filtered through Whatman filter paper No 1. Concentrated the solvent extract and the dried extract was stored at 4°C for further analysis.
2.2. Extraction and isolation of flavonoids from T. chebula
Eighty per cent of methanolic extracts were washed with petroleum ether to remove fatty matter. The filtrate was partitioned with diethyl ether and ethyl acetate. Diethyl ether and ethyl acetate fractions were concentrated by vacuum oven and further subjected to column chromatographic purification. Gradient elution was conducted initially with n-hexane and gradually enriched with benzene, chloroform, ethyl ether, acetone, ethanol, methanol and water successively in the increasing order of polarity [Citation27]. All the Fractions were collected dried and were tested for the presence of flavonoids [Citation28]. The fractions which showed positive results for flavonoids were used for in vitro antioxidant and DNA damage protection assays.
2.3. In vitro antioxidant activity
The isolated fractions were treated for free radical scavenging activity using DPPH radical scavenging activity [Citation29]. DPPH is a purple coloured stable free radical containing an unpaired electron. DPPH is reduced to DPPH-H while accepting a proton from antioxidant compounds. The DPPH solution loses its characteristic purple colour and the discoloration is proportional to the scavenging activity of the compound [Citation30].
The reaction mixture contained 2.8 ml of 100 µM DPPH dissolved in methanol and various concentrations of compounds in 0.2 ml distilled water. The mixture was shaken well and incubated at room temperature for 30 min. The absorbance was read at 517 nm by UV-visible spectrophotometer (Shimadzu; UV Pharmaspec-1700). Ascorbic acid was used as standard and the percentage of the DPPH scavenging effect was expressed as the percentage of inhibition from the given formula.
where A0 is the absorbance of the control and A1 is the absorbance of the sample/standard.
Lower absorbance of the reaction mixture indicated higher free radical scavenging activity.
2.4. DNA damage protection activity
The ability of different column purified fractions of plant extract to protect pEGFP-C1 plasmid DNA from harmful effects of hydroxyl radicals generated by Fenton’s reagent was evaluated by DNA nicking assay as described by Lee et al. [Citation31] with slight modifications. The plasmid DNA was mainly of the supercoiled form (S-Form). When plasmid DNA was dissolved in Fenton's reagent, it resulted in the formation of a single-stranded nicked circular form (C-Form) and double-stranded nicked linear DNA (L-Form). Exposure of plasmid DNA to Fenton's reagent causes strand breaks in DNA due to the attack of •OH radicals generated in the reaction mixture. When hydroxyl radicals react with DNA, nitrogenous bases of DNA produces base radicals and sugar radicals. These base radicals react with sugar moiety causing breakage of the sugar-phosphate backbone of nucleic acid resulting in strand break [Citation32].
In brief, the reaction mixture contained 2 µl (0.5 µg) of plasmid DNA, 5 µl of Fenton’s reagent (30 mM H2O2, 50 µM ascorbic acid and 80 µM FeCl3) followed by the addition of 20 µg of different fractions dissolved in ethanol. The final volume of the mixture was brought up to 20 µl using double-distilled water. The reaction mixtures were then allowed to incubate for 10 min at 37°C. After 10 min incubation, bromophenol blue dye was added. The reaction mixtures were loaded on 1% agarose gel and electrophoresis was carried out at 75 V for 1 h followed by ethidium bromide staining. The supercoiled, closed circular and linear forms of pEGFP-C1 were visualized and quantified using a LAS-4000 MINI Gel Documentation system.
Per cent inhibition of the DNA strand break was also calculated as,
where Sm+a is the % remaining supercoiled after treatment with mix plus agent, Sc is the % remaining supercoiled in control and Sm is the % remaining supercoiled with mix without agent [Citation33].
2.5. Structural characterization of the compound
The chemical structure of the isolated compound (Methanol–Water 1:1) was determined by UV-Visible Spectroscopy, Fourier Transform Infrared Spectroscopy (FT-IR), Proton Nuclear Magnetic Resonance Spectroscopy (1H NMR) and Mass Spectroscopy. UV-Visible spectrum was recorded on a Shimadzu UV Pharmaspec-1700 spectrophotometer using spectroscopic grade methanol as the solvent from 200 to 600 nm. Fourier transform infrared (FT-IR) spectrum of the compound was recorded in transmission mode with a Shimadzu FT-IR Spectrometer-8400S, in the wave number range of 400–4000 cm−1 using potassium bromide (KBr) pellets. Proton (1H) Nuclear Resonance (NMR) Spectrum was recorded on a Bruker Avance III, 400 MHz spectrometer. The compound was dissolved in DMSO-d6 for 1H NMR spectra. The chemical shift values were reported in ppm unit and the coupling constants (J) were in Hz. Mass Spectrum of the compound was recorded on an LCMS Waters e 2695 spectrometer equipped with an electrospray ion source (ESI). Mass spectral range, m/z 100–1000.
2.6. Statistical analysis
Each experiment was performed at least three times and results are presented as the mean ± SD. Statistical analysis was performed by Student’s t-test. Differences were considered significant at p<.05. The concentration needed for 50% inhibition (IC50) was estimated graphically by linear regression analysis.
3. Results
3.1. Isolation of flavonoids from T. chebula
Many fractions were obtained on column chromatographic separation. All the fractions were tested for the presence of flavonoids and the following fractions showed positive results. Hexane–Ether 1:4, Chloroform–Acetone 1:4, Ethyl acetate–Ethanol 6:1, Ether–Acetone 1:1 and Methanol–Water 1:1. These fractions were used for in vitro antioxidant and DNA damage protection activity assays.
3.2. In vitro antioxidant activity
Free radical scavenging capacities of the tested fractions were shown in Table .
Table 1. The free-radical scavenging activity of different fractions of T. chebula fruit and ascorbic acid (positive control).
It was observed that all the extracts possessed the effect of scavenging free radical properties. In this system, the radical scavenging activities of different fractions of T. chebula fruit and standard Ascorbic acid were in the order: Ascorbic acid (85.16%) > Methanol–Water 1:1(69%) > Ether–Acetone 1:1(68.32%) > Hexane–Ether 1:4(66.94%) > Chloroform–Acetone 1:4(56.8%) > Ethyl acetate- Ethanol 6:1(26.03%) at the dose of 20 µg/ml. The IC50 values were calculated from the regression equation. A lower IC50 value indicates greater antioxidant activity. The IC50 value of standard ascorbic acid was 9.87 ± 0.13 µg/ml. The IC50 value of Methanol–Water 1:1 fraction was found to be 11.23 ± 0.18 µg/ml indicated its highest antioxidant activity among the five fractions.
3.3. DNA protection assay
DNA nicking assay is employed to check the ability of fractions isolated from T. chebula against the DNA damage caused by free radicals. Figure (a) depicts the ability of the extracts to reduce Fe+3 dependent plasmid DNA nicking and Figure (b) depicts the band intensity of supercoiled, circular and linear forms. Different concentrations were tried; however, the addition of various fractions at a concentration of 20 µg/ml conferred a more significant reduction in the hydroxyl radical damage.
Figure 1. (a) Inhibitory effect of T. chebula fractions on plasmid DNA nicking. Lane 1 – DNA (pEGFP-C1 plasmid alone); Lane 2 – DNA and Fenton reagent; Lane 3 – DNA, Fenton reagent and Chloroform–Acetone 1:4; Lane 4 – DNA, Fenton reagent and Ethyl acetate–Ethanol 6:1; Lane 5 – DNA, Fenton reagent and Hexane–Ether 1:4; Lane 6 – DNA, Fenton reagent and Ether–Acetone 1:1; Lane 7-DNA, Fenton reagent and Methanol–Water 1:1. (b) Band intensity of Circular, Linear and Relaxed forms. Lane 1 – DNA (pEGFP-C1 plasmid alone); Lane 2 – DNA and Fenton reagent; Lane 3 – DNA, Fenton reagent and Chloroform–Acetone 1:4; Lane 4 – DNA, Fenton reagent and Ethyl acetate–Ethanol 6:1; Lane 5 – DNA, Fenton reagent and Hexane–Ether 1:4; Lane 6 – DNA, Fenton reagent and Ether–Acetone 1:1; Lane 7-DNA, Fenton reagent and Methanol–Water 1:1.
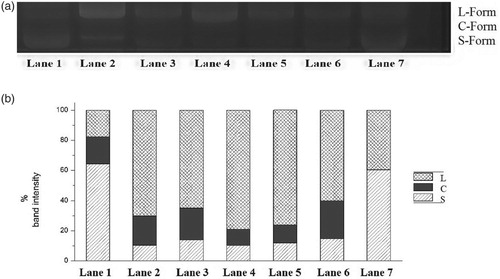
The protective activity of samples on oxidative DNA damage could be studied through observing the band intensity of the three forms DNA on gel. Addition of extract significantly inhibited the formation of circular (C) and linear (L) forms of plasmid DNA. It is clear from Figure (b), among the flavonoid fractions isolated, Methanol–Water 1:1 fraction exhibited the strongest efficiency, followed by Ether–Acetone 1:1, Hexane–Ether 1:4, Ethyl acetate–Ethanol 6:1 and Chloroform–Acetone 1:4.
In Table , we have presented the % inhibition of DNA strand scission and antioxidant activity of each flavonoid fraction at a concentration of 20 µg/ml. Amongst all the five extracts, Methanol–Water 1:1 fraction showed highest antioxidant and DNA protection activity as evidenced by maximum % inhibition in both. These results strongly support that the fractions protected DNA through the antioxidant activity. In other words, hydroxyl radicals caused DNA damage. Activity of these radicals can be reduced by antioxidants.
Table 2. % inhibition activity of different fractions at 20 µg/ml concentration.
3.4 Structural studies
The isolated compound was a yellow crystal solid mp, 240–245°C. The structural elucidation of the isolated compound was carried out using the spectroscopic methods as explained below.
Figure represents the UV-Visible spectrum of the compound. Absorption bands at 276 and 353 nm indicated the presence of aromatic ring and carbonyl group respectively. The higher value of the aromatic ring showed more electronegative groups substituted on the rings.
Figure represents the FT-IR spectrum of the compound. The following peaks observed in the spectrum correspond to the characteristic IR bands of the functional groups. Vmax in (cm−1): 3485 [phenolic O–H stretching vibration], 3082 [Aromatic C–H stretching vibration], 2912 and 2947 [C–H stretching vibrations of alkanes], 1720 [C = O cyclic ketone stretching vibrations], 1533 and 1446 [aromatic bending vibration], 1216 [aromatic C–O hydroxyl group], 1035 [aliphatic C–O hydroxyl group] and 763 and 869 [poly substituted aromatic rings].
Figure represents the 1H NMR spectrum of the compound. The following characteristic peaks are observed in the spectrum. Phenolic O–H appeared at 9.2 ppm with the integration of 4.4 estimated the presence of four phenolic groups in the compound. The peaks observed from 6.9–7.4 ppm with the total integration 4.5 indicated the presence of four aromatic hydrogens. The following peaks are observed for the aliphatic, cyclo-aliphatic and alkenyl hydrogens (Figure ).
5.744 (Ha), 5.724 (Hb), 5.156 (Hc), 5.052 (Hd), 4.411 (He), 1.155 (Hf), 2.989 (Hg), 2.955 (Hh), 2.930 (Hi), 2.923 (Hj), 2.895 (Hk), 2.886 (Hl), 2.874 (Hm), 2.866 (Hn), 2.847 (Ho), 2.837 (Hp), 2.716 (Hq), 2.706 (Hr), 2.676 (Hs), 2.667 (Ht) and the end methyl group hydrogens at 1.1 ppm (Hf).
From the above three spectra (viz: UV-Vis, FT-IR, and NMR), the following information was derived. The parent compound contained more than one aromatic ring with different substitutions, a carbonyl group, and an unsaturation. Phenolic and hydroxyl –OH were also present. It also contained methylene hydrogen and an end methyl group. Based on this, we studied the mass spectrum and elucidated the proposed structure. The Explanation of the peaks obtained in the mass spectrum is shown in Table .
Table 3. Description of mass spectrum.
Thus, according to the mentioned data and by comparison with the literature [Citation34], compound identified as a derivative of luteolin. Figure represent the chemical structure of the compound.
4. Discussion
Free radicals are constantly generated in the living system as a result of normal cell metabolism. But their overproduction creates an imbalance between the generation of Reactive Oxygen Species and the antioxidant enzymes, which can lead to the oxidative stress and finally the damage to essential biomolecules such as DNA, nucleic acids, lipids and proteins [Citation35]. Many health disorders such as cardiovascular disease, cancer, liver disease, diabetes, ageing and neurodegenerative diseases are also associated with oxidative stress [Citation30]. Many studies prove that the uncontrolled production and action of hydroxyl radicals, the most damaging free radical among others, have adverse effects within the body. Hydroxyl radical is derived from hydrogen peroxide in the presence of transition metals such as iron and copper which in turn is derived from superoxide radical through the action of superoxide dismutase enzyme [Citation36]. Therefore antioxidant based studies are treated as a subject of serious study and have received much attention in the medical field also. All organisms have antioxidant defenses, including antioxidant enzymes and antioxidant dietary components, to remove or repair damaged molecules [Citation37]. If ROS are not removed by such constituents they may cause irreversible oxidative damage. Many synthetic antioxidants such as butylated hydroxyanisole (BHA) and butylated hydroxytoluene (BHT) are capable of protecting cells from such oxidative damage, but they are not well accepted since they cause liver damage and cancer [Citation38]. Therefore much research is going on to find out the alternative remedy from non-toxic natural resources with strong antioxidant capacity.
The flavonoid fractions isolated from T. chebula fruit possessed the effect of scavenging free radical properties. The flavonoids exert their antioxidative action either through scavenging or chelating properties. In the scavenging mechanism, flavonoids are oxidized by radicals resulting in a more stable, less reactive free radical. In other words, the hydroxyl groups of flavonoids are highly reactive which make free radicals inactive and stable. Flavonoids also behave as antioxidants by chelation of transition metals, thereby removing a casual factor involved in the formation of free radicals [Citation39]. In the present study, the highest activity was observed in Methanol–Water 1:1 fraction followed by Ether–Acetone 1:1fraction.
DNA is subjected to constant assault from both environmental factors and normal metabolic processes. The DNA protection ability of fractions isolated from T. chebula by scavenging the free radicals was also estimated. Oxidative damage to plasmid DNA caused by •OH radicals produced from Fenton's reagent was measured in terms of conversion of supercoiled to nicked circular and linear forms. All the fractions showed prominent DNA protection activity, Methanol–Water 1:1 being the best. Till now no DNA protection activity was reported for flavonoids isolated from the fruit of T. chebula. It is evident from Table , DNA protection capacity of fractions have a linear relationship with antioxidant capacity. Asker et al. [Citation40] reported that flavonoids of plants are directly related to DNA protection activity, either by chelating the transition metal or by inhibiting the enzymes involved in the initiation reaction of DNA break. Methanol -Water 1:1 fraction was characterized as luteolin (a flavone) derivative. Being a natural product, luteolin derivative would be more effective compared to the commercial or other materials in terms of cost, efficiency, etc. On comparison with the previous studies, it can be found that the supercoiled percentage remaining in the case of isolated luteolin derivative (60.5%) is higher than the other standard antioxidants like catechin, luteolin, apigenin, quercetin and Kaempferol [Citation41]. Cumulative studies have suggested that dietary flavonoids reduced DNA damage through inhibition of Reactive Oxygen Species. It has also been reported that flavonoids can form complexes with DNA, thus protecting it from oxidative damage [Citation42]. There are only few research papers available in the literature about the study and use of luteolin for DNA protection. One of the main Ayurvedic medicines available in the market using luteolin as one of the components, “Algonot Neuroprotek”. These types of medicines are natural and are durable. There is no reported side effect. The study of DNA protection is an advanced care to be taken before the damage of DNA. The studies based on luteolin derivative from T. chebula for the protection of DNA are comparably recent. There may be chemical reactions to recover the component and reuse them. However, there is no such report available in the literature.
5 Conclusion
This study is a detailed report on the antioxidant and DNA protection ability of flavonoid fractions isolated from the fruit of T. chebula and the structural characterization of the best among them. All the fractions were found having strong antioxidant and DNA protection activity. Methanol–Water 1:1 fraction showed the excellent activity in both assays and its structure was elucidated as a derivative of luteolin. Definitive clinical studies are needed to fully understand the underlying mechanism of their role on the DNA damage protection and antioxidant activity. A decrease in oxidative damage upon flavonoid intervention might be interpreted as a decrease in cancer risk. Therefore, future work should include research into which the isolated compound could be used for the anti-carcinogenic effects. In vivo studies are under progress to confirm the non-toxic activity of the active component on the animal. It can be a good option to be used as a drug after the completion of in vivo studies.
Disclosure statement
No potential conflict of interest was reported by the authors.
ORCID
K. Soumya http://orcid.org/0000-0001-7594-4012
Jesna James http://orcid.org/0000-0003-2999-3141
V. B. Sameer Kumar http://orcid.org/0000-0002-0895-0241
Additional information
Funding
References
- Andreassi MG, Botto N. DNA damage as a new emerging risk factor in atherosclerosis. Trends Cardiovas med. 2003;13:270–275. doi: 10.1016/S1050-1738(03)00109-9
- De Bont R, Van Larebeke N. Endogenous DNA in humans: a review of quantitative data. Mutagenesis. 2004;19:169–185. doi: 10.1093/mutage/geh025
- Christmann M, Tomicic MT, Roos WP, et al. Mechanisms of human DNA repair: an update. Toxicology. 2003;193:3–34. doi: 10.1016/S0300-483X(03)00287-7
- Phaniendra A, Jestadi DB, Periyasamy L. Free radicals: properties, sources, targets, and their implication in various diseases. Ind J Clin Biochem. 2015;30:11–26. doi: 10.1007/s12291-014-0446-0
- Kubola J, Siriamornpun S. Phenolic contents and antioxidant activities of bitter gourd (Momordica charantia L.) leaf, stem and fruit fraction extracts in vitro. Food Chem. 2008;110:881–890. doi: 10.1016/j.foodchem.2008.02.076
- Banerjee A, Dasgupta N, De B. In vitro study of antioxidant activity of Syzygium cumini fruit. Food Chem. 2005;90:727–733. doi: 10.1016/j.foodchem.2004.04.033
- Chew YL, Goh JK, Lim YY. Assessment of in vitro antioxidant capacity and polyphenolic composition of selected medicinal herbs from Leguminosae family in Peninsular Malaysia. Food Chem. 2009;116:13–18. doi: 10.1016/j.foodchem.2009.01.091
- Sudheesh S, Presannakumar G, Vijayakumar S, et al. Hypolipidemic effect of flavonoids from Solanum melongena. Plant Foods Hum Nutr. 1997;51:321–330. doi: 10.1023/A:1007965927434
- Cai Q, Rahn RO, Zhang R. Dietary flavonoids, quercetin, luteolin, and genistein, reduce oxidative DNA damage and lipid peroxidation and quench free radicals. Cancer Lett. 1997;119:99–107. doi: 10.1016/S0304-3835(97)00261-9
- Jose J, Dhanya AT, Haridas KR, et al. Structural characterization of a novel derivative of myricetin from Mimosa pudica as an anti-proliferative agent for the treatment of cancer. Biomed Pharmacother. 2016;84:1067–1077. doi: 10.1016/j.biopha.2016.10.020
- Jose J, Sudhakaran S, Sumesh KKTM, et al. Study of In vitro Immunomodulatory effect of flavonoid isolated from Phyllanthus niruri on human Blood Lymphocytes and Evaluation of its antioxidant Potential. IJPPR. 2014;6:284–289.
- Tapas AR, Sakarkar DM, Kakde RB. Flavonoids as Nutraceuticals: a review. Trop J Pharm Res. 2008;7:1089–1099. doi: 10.4314/tjpr.v7i3.14693
- Pal SK, Shukla Y. Herbal medicine: current status and the future. APJCP. 2003;4:281–288.
- Close DC, McArthur C. Rethinking the role of many plant phenolics–protection from photodamage not herbivores? Oikos. 2002;99:166–172. doi: 10.1034/j.1600-0706.2002.990117.x
- Silva JP, Gomes AC, Coutinho OP. Oxidative DNA damage protection and repair by polyphenolic compounds in PC12 cells. Eur J Pharmacol. 2008;60:50–60. doi: 10.1016/j.ejphar.2008.10.046
- Ferreira IC, Baptista P, Vilas-Boas M, et al. Free-radical scavenging capacity and reducing power of wild edible mushrooms from northeast Portugal: Individual cap and stipe activity. Food Chem. 2007;100:1511–1516. doi: 10.1016/j.foodchem.2005.11.043
- Mahesh AR, Ranganath MK, Harish Kumar DR. Enrichment of flavonoids from the methanolic extract of Boerhaavia diffusa Roots by Partitioning Technique. Res J Chem Sci. 2013;3:43–47.
- Lee D, Boo KH, Woo JK, et al. Anti bacterial and anti-viral activities of extracts from Terminalia chebula barks. J Korean Soc Appl Biol Chem. 2011;54:295–298.
- Saleem A, Husheem M, Harkonen P, et al. Inhibition of cancer cell growth by crude extract and the phenolics of Terminalia chebula retz. fruit. J Ethnopharmacol. 2002;81:327–336. doi: 10.1016/S0378-8741(02)00099-5
- Rathinamoorthy R, Thilagavathi G. Terminalia chebula - review on pharmacological and biochemical studies. Int J PharmTech Res. 2014;6:97–116.
- Kannan P, Ramadevi SR, Hopper W. Antibacterial activity of Terminalia chebula fruit extract. AfrJMicrobiolRes. 2009;3:180–184.
- Bag A, Bhattacharyya SK, Pal NK, et al. In vitro antimicrobial potential of Terminalia chebula fruit extracts against multidrug–resistant uropathogens. Asian Pac J Trop Biomed. 2012;2:S1883–S1887. doi: 10.1016/S2221-1691(12)60514-0
- Malekzadeh F, Ehsanifar H, Shahamat M, et al. Antibacterial activity of black myrobalan (Terminalia chebula Retz) against Helicobacter pylori. Int J Antimicrob Agents. 2001;18:85–88. doi: 10.1016/S0924-8579(01)00352-1
- Kumar GP, Arulselvan P, Kumar DS, et al. Anti-diabetic activity of fruits of Terminalia chebula on streptozotocin induced diabetic rats. J Health Sci. 2006;52:283–291. doi: 10.1248/jhs.52.283
- Saha S, Verma RJ. Antioxidant activity of polyphenolic extract of Terminalia chebula Retzius fruits. J Taibah Univ Sci. 2016;10:805–812. doi: 10.1016/j.jtusci.2014.09.003
- Jane SS, Divakar KM. A Comparative Phytochemical analysis of various Biotypes of Terminalia chebula Retz. fruits of Western Ghats. IOSR JPBS. 2016;2:01–04.
- Sudheesh S, Vijayalakshmi NR. Flavonoids from Punica granatum—potential antiperoxidative agents. Fitoterapia. 2005;76:181–186. doi: 10.1016/j.fitote.2004.11.002
- Temidayo AR. Extraction and Isolation of flavonoids present in the methanolic extract of Leaves of Acanthospermum HispidiumDc, Glob. J Med Plant Res. 2013;1:111–123.
- Dasgupta N, De B. Antioxidant activity of Piper betle L. leaf extract in vitro. Food Chem. 2004;88:219–224. doi: 10.1016/j.foodchem.2004.01.036
- Verma K, Shrivastava D, Kumar G. Antioxidant activity and DNA damage inhibition in vitro by a methanolic extract of Carissa carandas (Apocynaceae) leaves. J Taibah Univ Sci. 2015;9:34–40. doi: 10.1016/j.jtusci.2014.07.001
- Lee JC, Kim HR, Kim J, et al. Antioxidant property of an ethanol extract of the stem of Opuntia ficus-indica var. Saboten. J Agric Food Chem. 2002;50:6490–6496. doi: 10.1021/jf020388c
- Golla U, Gajam PK, Bhimathati SS. Evaluation of diuretic and laxative activity of hydro-alcoholic extract of Desmostachya bipinnata (L.) Stapf in rats. J Integr Med. 2014;12:372–378. doi: 10.1016/S2095-4964(14)60029-7
- Kizil M, Yavuz M. Protective activity of ethanol extract of three Achillea species against lipid peroxidation, protein oxidation and DNA damage in vitro. Acta Aliment. 2010;39:457–470. doi: 10.1556/AAlim.39.2010.4.7
- Klika KD, Saleem A, Sinkkonen J, et al. The structural and conformational analyses and antioxidant activities of chebulinic acid and its thrice- hydrolyzed derivative, 2,4-chebuloyl-β-D-glucopyranoside, isolated from the fruit of Terminalia chebula. ARKIVOC. 2004;7:83–105.
- Diaz P, Jeong SC, Lee S, et al. Antioxidant and anti-inflammatory activities of selected medicinal plants and fungi containing phenolic and flavonoid compounds. Chin Med. 2012;7:1–9. doi: 10.1186/1749-8546-7-26
- Dizdaroglu M, Jaruga P. Mechanisms of free radical induced damage to DNA. Free Radic Res. 2012;46:382–419. doi: 10.3109/10715762.2011.653969
- Ak T, Gulcin I. Antioxidant and radical scavenging properties of curcumin. Chem Biol Interact. 2008;174:27–37. doi: 10.1016/j.cbi.2008.05.003
- Kasangana PB, Haddad PS, Stevanovic T. Study of polyphenol content and antioxidant capacity of Myrianthus arboreus (Cecropiaceae) root bark extracts. Antioxidants. 2015;4:410–426. doi: 10.3390/antiox4020410
- Nijveldt RJ, van Nood E, van Hoorn DE, et al. Flavonoids: a review of probable mechanisms of action and Potential applications. Am J Ciln Nutr. 2001;74:418–425. doi: 10.1093/ajcn/74.4.418
- Van Acker SA, Tromp MN, Griffioen DH, et al. Structural aspects of antioxidant activity of flavonoids. Free Radic Biol Med. 1996;20:331–342. doi: 10.1016/0891-5849(95)02047-0
- Zhu L, Chen J, Tan J, et al. Flavonoids from Agrimonia pilosa Ledeb: free radical scavenging and DNA oxidative damage protection activities and analysis of bioactivity-structure relationship based on molecular and electronic structures. Molecules. 2017;26:195. doi: 10.3390/molecules22030195
- P. Tiwari, Mishra, Role of flavonoids in DNA damage and carcinogenesis prevention. J Carcinog Mutagen. 2017;08:4–9. doi: 10.4172/2157-2518.1000297