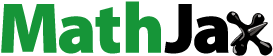
Abstract
Selenium is a trace element which can be toxic when consumed at high levels. The study was conducted to assess the possible protective role of vitamin E against selenosis in rats. Selenium was applied at different single doses (4.5, 9 and 18 mg/kg b.wt.). Vitamin E (200 mg/kg b.wt.) was given to the rats one hour before treatment with the higher selenium dose. In selenium-treated rats, selenosis was evident from the elevated level of malondialdehyde. Oxidative stress was induced from the significant altered activity levels of CAT, SOD and GPx. Furthermore, selenium-induced hepatotoxicity was developed, where the activity levels of AST, ALT and GGT were significantly increased. Hepatotoxicity was also manifested histologically. Pretreatment with vitamin E significantly alleviated the affected levels of the investigated parameters, and counteracted the hepatic histopathological changes. The results demonstrate that vitamin E supplementation provides an effective protection against oxidative damage and hepatotoxicity induced by selenosis.
1. Introduction
Selenium is a trace element that is vital for humans and other living organisms. It is involved in several biological functions since it is a component of many selenoproteins and selenoenzymes [Citation1]. Selenium is abundant in soils. Its concentrations in soils vary widely from region to region [Citation2,Citation3]. Selenium is found in different forms. Inorganic selenium compounds, selenite and selenate, dominate in soil and water, whereas organic selenium forms, selenomethionine and selenocysteine, dominate in plants [Citation4]. Selenium toxicity in foods depends on its chemical forms. Generally, organic selenium is less toxic than the inorganic selenium [Citation5,Citation6].
Selenium is a double-edged sword with beneficial effects on one side and toxic effects, when in high concentration, on the other one. According to the initiative of the Agency for Toxic Substances and Disease Registry (ATSDR) in 2003 [Citation7], the recommended dietary allowance for selenium is 55 micrograms per day (μg/d) for persons 14 years or older, with a tolerable upper intake level of 400 μg/d. Selenium deficiency leads to a variety of health problems in humans including cardiomyopathy [Citation8,Citation9]. Selenium deficiency is also involved in the pathogenesis of Keshan disease, a disease that occurs almost in children [Citation2,Citation10,Citation11]. Kashin-Beck illness is another disease of selenium deficiency in humans. This disease is caused by the selenium deficiency, along with the iodine deficiency, and leads to atrophy and necrosis of cartilage [Citation12,Citation13]. In Australia, selenium (Se) deficiency caused health implications to livestock and the implications were removed following selenium supplementation [Citation14].
Selenium is also very toxic and can induce toxicity (selenosis) to humans and animals. Acute or chronic intake of excess selenium can cause selenium toxicity. Acute selenosis in pigs occurs after an extremely high dietary intake more than 20 mg/kg body weight of selenium. Chronic selenosis occurs after consuming diets containing 5–20 ppm selenium over a longer time period [Citation15]. Selenium toxicity was also reported in livestock feeding on selenium-rich plants [Citation14,Citation16]. In humans, selenosis takes place as a result of overconsumption of nutritional supplements containing excess selenium or accidental overdose of a pharmaceutical product [Citation17]. The outbreaks of human selenium toxicity occur in populations eating crops growing on selenium accumulative soils [Citation18,Citation19]. People consumed high levels of selenium in food supplies showed clinical manifestations and symptoms of toxicity including loss of hair and nail, decay of the teeth and disturbances of the central nervous system (CNS) [Citation19,Citation20].
Vitamins are antioxidants that protect cells and tissues against oxidative stress. Among the most important antioxidant vitamins for tissue protection is vitamin E. For proper physiological function, there is a balance between the amount of free radicals generated in the body and the antioxidants necessary for protection against them [Citation21,Citation22]. This balance can be shifted resulting in oxidative stress when there are excess of free radicals and lack of antioxidant protection. The dietary and tissue balance of nutrients including vitamins are important in protecting tissues against oxidative stress.
As most previous studies of selenosis have focused on the clinical features and general health condition, the present study was conducted in order to address questions about the hepatotoxic effects of acute selenium poisoning in male albino rats, and whether the effects are dose-dependent and time-dependent. The study also aimed to assess the potential protective effects of vitamin E against selenosis. In addition, in order to have a better understanding of hepatotoxic effect of selenium and the protective role of vitamin E, determination of lipid peroxidation and antioxidant enzyme activity levels as well as histological studies of liver were carried out.
2. Materials and methods
2.1. Chemicals
Sodium Selenite and vitamin E (DL-α-Tocopherol acetate) were purchased from Sigma-Aldrich, MO, USA. Kits for liver function test were purchased from ELITech Clinical Systems, USA. Kits for lipid peroxidation test and antioxidant enzymes assay were purchased from Invitrogen, USA.
2.2. Animals
Male Wistar Albino rats, two-month-old, weighing 150–170 g were obtained from the Laboratory Animal Centre of King Abdulaziz University, Saudi Arabia. They were housed in an animal facility and kept under standard laboratory conditions at 25 ± 2°C, under control of air condition, and 12 h day/night cycle in ventilated cages. The animals were allowed to acclimatize for two weeks before starting the experiments and were observed for general health. They were given free access to a standard laboratory rat diet containing 0.06 µg selenium/g diet, and deionized water ad libitum through the whole study. All experimental procedures were approved and carried out in accordance with the Experimental Animals Research and Ethics Committee of King Abdulaziz University, Saudi Arabia (Approval no. 8012/12/8/2016), and national guidelines for the care and use of the experimental animals.
2.3. Lethality assay
The LD50 value of sodium selenite was determined according to Finney [Citation23]. Five groups of rats, each with ten rats, were used for the experiment. Five different doses of sodium selenite, 5, 10, 15, 20 and 25 mg/kg body weight were chosen and given separately to the five groups of rats by oral administration. Following treatment with selenite solution, rats were monitored for 2, 4, 6, 24 and 48 h, and the number of dead animals in each group was recorded during the experiment. The dose that killed 50% of rats (LD50) after 48 h was calculated using probit analysis with Microsoft Excel. Accordingly, LD50 was estimated to be 18 ± 1.3 mg/kg body weight.
2.4. Experimental design
The sample size of rats used in the present work, according to the experimental design, was computed using the software G-power analysis (version 3.1.9.4). With a power of 80%, 0.05 level of statistical significance and effect size of 0.08, the computed total sample size was 72 rats (N = 72). The sample size per group was 12 rats.
After two weeks of acclimatization to standard laboratory conditions, rats were randomly divided into six groups, each with an equal sample size of twelve male rats (n = 12). Rats in group I received deionized water (0.00 mg sodium selenite) as a vehicle that served as control. Rats in groups II, III and IV received a single dose of sodium selenite at 0.25 LD50 (4.50 mg/kg body weight), 0.5 LD50 (9 mg/kg body weight) and LD50 (18 mg/kg body weight), respectively. Rats in group V received 200 mg/kg body weight of vitamin E [Citation24]. Rats in group VI received 200 mg/kg body weight of vitamin E and, one hour later, a single LD50 of sodium selenite (18 mg/kg body weight). Sodium selenite and/or vitamin E were given to the rats by oral gavage. At the end of experimental periods (12 and 30 h of exposure to chemicals), rats were randomly drawn from each group, i.e. the sample size for each period was six rats (n = 6), and sacrificed under ether anesthesia.
2.5. Biochemical analysis
2.5.1. Blood sampling
Blood samples were collected from the orbital sinus according to the method described by Hoff and Rlatg [Citation25]. Blood samples were divided into two parts, heparinized and non-heparinized blood. The first part of blood samples was collected in heparinized tubes and centrifuged at 1500×g for 10 min at 4°C. Aliquots of the top plasma layer were stored at −20°C for the assay of antioxidant enzymes. The second part of blood samples (non-heparinized) were left to clot for 30 min, and then centrifuged at 1500×g for 10 min at 4°C to separate serum. Aliquots of serum were stored at −20°C until analysis of malondialdehyde and liver function enzymes.
2.5.2. Determination of malondialdehyde (MDA) level as a lipid peroxidation marker
Malondialdehyde (MDA) was determined in blood serum according to Ohkawa et al. [Citation26]. In this method, thiobarbituric acid (TBA) reacts with MDA at 95°C for 30 min. The resultant product was measured at 530 nm.
2.5.3. Assays for measuring antioxidant enzymes
2.5.3.1. Assessment of catalase (CAT) activity
The activity of catalase in blood plasma was determined using hydrogen peroxide and peroxidase reagent containing peroxidase and a chromogen system according to Aebi [Citation27]. This method is based on the peroxide removal by catalase. At the end, the reaction was stopped by the peroxidase reagent and consequent reduction of remaining hydrogen peroxide to form a chromophore which was recorded at 505 nm.
2.5.3.2. Assessment of superoxide dismutase (SOD) activity
The activity of SOD in blood plasma was determined using the nitroblue tetrazolium (NBT) method described by Nishikimi et al. [Citation28]. This method is based on the ability of enzyme to inhibit the reduction of NBT by superoxide radicals. The reduction of NBT by superoxide radicals to blue formazan was followed at 560 nm.
2.5.3.3. Assessment of glutathione peroxidase (GPx) activity
The activity of GPx in blood plasma was measured using hydrogen peroxide, glutathione, glutathione reductase and NADPH according to Paglia and Valentine [Citation29]. This method is based on oxidation of glutathione upon reduction of hydrogen peroxide and regeneration of reduced glutathione by glutathione reductase. The absorbance was monitored at 340 nm.
2.5.4. Assays for measuring liver enzyme activity levels
2.5.4.1. Assessment of aspartate aminotransferase (AST) activity
The activity of AST in blood serum was measured using L-aspartate and α-ketoglutarate, malate dehydrogenase (MDH) and NADH according to Schumann et al. [Citation30]. In this method, AST catalyzes the formation of oxaloacetate and L-glutamate. Malate is formed as a result of reduction of the oxaloacetate in the presence of MDH. The rate of change in absorbance at 340 nm over 159 sec was monitored.
2.5.4.2. Assessment of alanine aminotransferase (ALT) activity
The activity of ALT in blood serum was measured using L-alanine and α-ketoglutarate, lactate dehydrogenase (LDH) and NADH according to Schumann et al. [Citation31]. In this method, ALT catalyzes the formation of pyruvate and L-glutamate. Lactate is formed as a result of reduction of the pyruvate in the presence of LDH. The rate of change in absorbance at 340 nm over 159 sec was monitored.
2.5.4.3. Assessment of gamma-glutamyltransferase (GGT) activity
The activity of GGT in blood serum was measured using L-γ-glutamyl-3-carboxy-p-nitroanilide (GLUPA-C) and glycylglycine according to Schumann et al. [Citation32]. In this method, GGT catalyzes the formation of L-γ-Glutamyl-glycylglycine and 5-amino-2-nitrobenzoate. The rate of change of absorbance of 5-amino-2-nitrobenzoate at 405 nm over 159 sec was monitored.
2.6. Histopathological examination
For histopathological examination, the control and treated rats were sacrificed after 12 or 30 h from chemical treatment under ether anesthesia. The liver was excised, washed with normal saline, fixed immediately in neutral buffered formalin, dehydrated in graded series of ethyl alcohol, cleared in chloroform and was then embedded in paraplast. Five μm-thick sections were cut using Leica RM2255 Fully Automated Rotary Microtome (Germany), stained with haematoxylin and eosin according to Bancroft and Gamble [Citation33], examined and photographed.
2.7. Statistical analyses
Kolmogorov–Smirnov test was used to determine the normal distribution of data. All variables were normally distributed, and therefore the parametric statistical analyses were applied. Two-way analysis of variances (ANOVA) was performed to evaluate the effects of treatments with chemicals (selenium alone and vitamin E in combination with selenium), experimental periods (12 and 30 h) and their interactions on the studied parameters. Two-way ANOVA was followed by Duncan’s multiple range test to estimate the significant differences among all the studied groups at various treatments and periods. Regression analysis and spearman’s correlation coefficient (r) were used to fit the relationships between the different studied parameters and the given doses of selenium at 12 and 30 h. All the results were expressed as a mean ± standard error of the mean (SEM). All data were analyzed by the aid of IBM Statistical Package for the Social Sciences, SPSS version 25 (SPSS Inc., IBM, Chicago, IL, USA).
3. Results
3.1. Health status of animals
At the end of experiments, rats from the control group, vitamin E-treated group and vitamin E and LD50 of selenium group were in a good health state. No signs of stress, pain, illness and injury were observed. However, selenium-treated rats showed a variety of symptoms including itching, hair loss and stress.
3.2. Biochemical analysis
3.2.1. Malondialdehyde (MDA) level
The levels of liberated malondialdehyde (MDA), lipid peroxidation indicator, were significantly affected by the treatments with chemicals (selenium alone and vitamin E in combination with selenium), the experimental periods (12 and 30 h) and their interactions (P < 0.0001). In addition, according to the regression analysis, there was a positive relationship between the concentration of MDA and the given doses of selenium at 12 and 30 h with a significant correlation coefficient (Figure ). According to Duncan’s test, the MDA levels were significantly increased after 12 and 30 h post treatment with the applied doses of selenium (P < 0.05) compared with the control group. However, the levels of MDA were significantly decreased after 12 and 30 h post vitamin E and LD50 administration (Group VI) as compared to the corresponding levels obtained after treatment with LD50 alone, Group IV (Table ).
Figure 1. Relationship between the given doses of selenium (0, 4.5, 9, and 18 mg/kg body weight) and (a) the levels of malondialdehyde (MDA) and (b–d) the activities of antioxidant enzymes (CAT, SOD, and GPx) in rats after 12 h (blue line) and 30 h (red line). r, Spearman’s correlation coefficient; *, significant correlation.
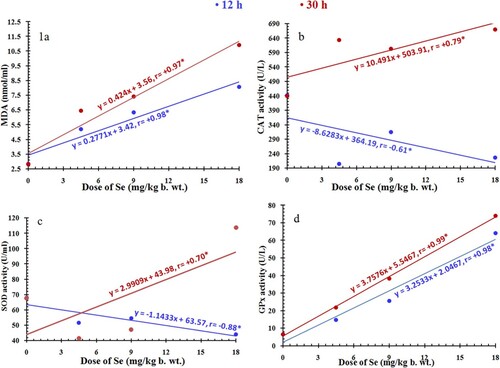
Table 1. Effect of vitamin E on serum levels of malondialdehyde (MDA) and plasma levels of catalase (CAT), superoxide dismutase (SOD), and glutathione peroxidase (GPx) activities in selenium-treated rats (n = 6).
3.2.2. Antioxidant enzymes
The activities of antioxidant enzymes were significantly affected by the treatments with chemicals, the experimental periods (12 and 30 h) and their interactions (P < 0.0001). Regression analysis showed a positive relationship between the activities of antioxidant enzymes and the given doses of selenium at 12 and 30 h except for CAT and SOD enzymes (negative relationship at 12 h) with a significant correlation coefficient (Figure ). According to Duncan’s test, the catalase and SOD activities were significantly decreased after 12 h from treatment with all used selenium doses compared with the control group. After 30 h, the catalase showed significant increased activity with all doses used, while SOD showed significant increased activity at LD50 only. In addition, the GPx activity was significantly increased after 12 and 30 h from treatment with all doses applied. Moreover, the activity levels of catalase, SOD and GPx were significantly decreased after 30 h post vitamin E and LD50 treatment as compared to the corresponding activity levels recorded after treatment with LD50 alone (Table ).
3.2.3. Liver enzymes
The activities of liver enzymes were significantly affected by the treatments with chemicals, the experimental periods (12 and 30 h) and their interactions (P < 0.0001). Regression analysis showed a positive relationship between the activities of liver enzymes and the given doses of selenium at 12 and 30 h with a significant correlation coefficient (Figure ). The activity levels of AST, ALT and GGT in selenium-treated rats were substantially influenced by the selenium doses, where they displayed significant activity levels higher than the normal levels (P < 0.05). However, the activities of liver enzymes were significantly decreased after 12 and 30 h post vitamin E and LD50 treatment as compared to the corresponding activity levels scored after treatment with LD50 alone (Table ).
Figure 2. Relationship between the given doses of selenium (0, 4.5, 9, and 18 mg/kg body weight) and the activities of liver enzymes, AST, ALT, and GGT (a–c) in rats after 12 h (blue line) and 30 h (red line). r, Spearman’s correlation coefficient; *, significant correlation.
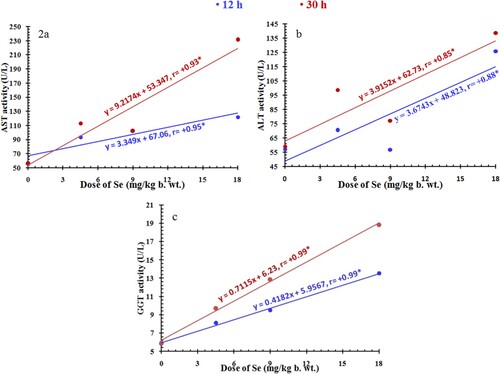
Table 2. Effect of vitamin E on serum levels of aspartate (AST), alanine (ALT) aminotransferases, and γ-glutamyltransferase (GGT) activities in selenium-treated rats (n = 6).
3.3. Histopathological observation
The histological examination of the liver sections of control group (Group I) revealed normal architecture and organization. Hepatocytes are arranged into anastomosing plates radiating from a central vein. The spaces between the plates are occupied by sinusoids. At the periphery of the hepatic lobules are the portal tracts which contain branches of the hepatic portal vein, hepatic artery and bile duct (Figure a and b). Rats given vitamin E (Group V) also showed normal liver architecture and organization.
Figure 3. Photomicrographs of sections of the liver of rats. (a and b) A control rat showing normal structure and organization. (c to f) Rats administrated with 0.25 LD50 of selenium. (g to j) Rats administrated with 0.5 LD50 of selenium. (c and d) After 12 h, the liver parenchymal cells appear almost normal. (e and f) After 30 h, microvesicular steatosis (MS) is seen in most hepatocytes. Feathery degeneration (FD) is evident in the periportal area. (g and h) After 12 h, the central vein (CV) and the portal vein (PV) appear slightly dilated and congested. (i and j) After 30 h, the central vein and the portal vein are markedly dilated and congested. PN, pyknotic nucleus; HS, hepatic sinusoids; FC, fatty change; HA, branch of hepatic artery; BD, branch of bile duct. H&E, scale bar = 50 µm.
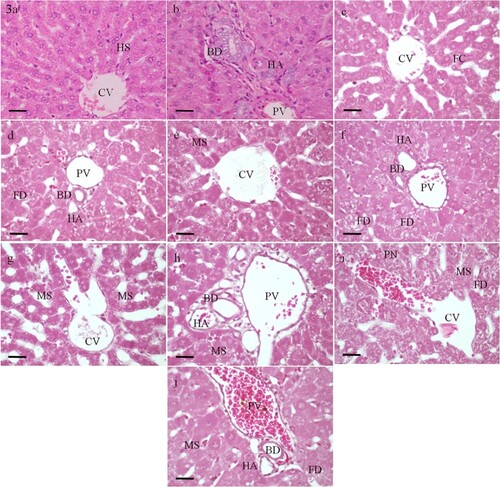
In the group II, where rats received a low dose of selenium (0.25 LD50), the liver tissues appeared almost normal (Figure c–f). Sections of the liver obtained from group III rats 12 and 30 h post treatment with 0.5 LD50 of selenium showed dilation and congestion of the central vein and the portal vein (Figure g–j). After 30 h, marked microvesicular steatosis and feathery degeneration were noticed (Figure i and j). The maximum hepatic alterations were obtained in rats treated with LD50 of selenium particularly after 30 h (Group IV). Loss of sinusoidal architecture, diffused hemorrhage and mononuclear infiltration were observed. Marked microvesicular steatosis and bile duct proliferation were recognized. The hepatocytes showed severe necrotic changes including substantial degeneration of cytoplasm and pyknosis or karyorrhexis of nuclei (Figure a–h).
Figure 4. Photomicrographs of sections of the liver obtained from rats 12 h (a to d) and 30 h (e to h) post administration with LD50 of selenium showing loss of sinusoidal architecture and diffused hemorrhage (H). Marked dilation and congestion of the central vein (CV) and the portal vein (PV) are observed. Bile duct (BD) proliferation is recognized. Marked microvesicular steatosis (MS), and pyknotic (PN) and karyorrhectic nuclei (arrow) are evident. HA, branch of hepatic artery. H&E, scale bar = 50 µm.
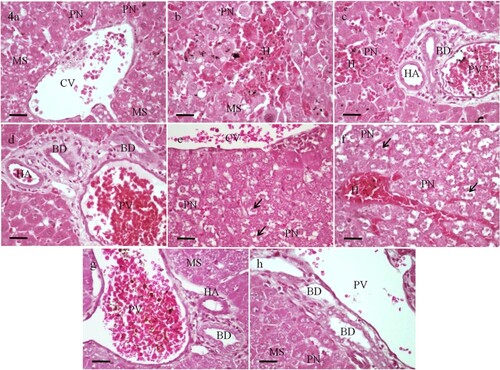
In the group VI, where rats received both vitamin E and a single LD50 of selenium, and sacrificed after 12 h, the liver showed manifestations of mild damage. Preserved radially arranged plates around the central vein were observed. The hepatocytes revealed regression in steatosis (Figure a and b). After 30 h, pretreatment with vitamin E greatly prevented the hepatic histopathological changes. The parenchyma attained nearly normal structure and organization. Hepatocellular degenerative changes were not observed (Figure c and d).
Figure 5. Photomicrographs of sections of the liver of rats received both vitamin E and a single LD50 of selenium, and sacrificed either after 12 h (a and b) or 30 h (c and d). Normal hepatic structure and organization are nearly appeared particularly after 30 h. The hepatocytes reveal regression in fatty and necrotic changes with no or little vacuoles and pyknosis. Portal triads are seen with almost normal appearance. Numerous Kupffer cells (arrow) are noticed. MS, microvesicular steatosis; CV, central vein; PV, branch of portal vein; HA, branch of hepatic artery; BD, branch of bile duct. H&E, scale bar = 50 µm.
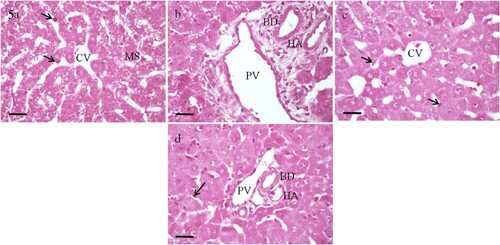
4. Discussion
The present study investigated the oxidative damage and hepatotoxicity of acute oral administrations of sodium selenite and the protective role of vitamin E against selenosis. Selenosis occurs in humans as a result of overconsumption of dietary supplements containing excess selenium or accidental overdose of selenium-containing medications. Lipid peroxidation level and antioxidant enzyme activities were measured in serum and plasma, respectively, to examine the whole health and oxidative status of animals due to excess intake of selenium. The observed symptoms in the experimental rats due to the given doses of selenium indicate its toxicity when taken in excess. Selenium-induced oxidative stress reflects the toxicity and damage of the body organs including liver in the present study [Citation34,Citation35].
4.1. Selenium and lipid peroxidation (LP)
Lipid peroxides are formed when free radical reactions occur in the cell membranes. The reactions are initiated when free radicals combine with hydrogen atoms in an unsaturated fatty acyl chain to form lipid peroxyl radicals. Lipid peroxyl radicals can further decompose to an aldehyde such as malondialdehyde [Citation36]. The result of the present study showed that treatment with sodium selenite caused a significant elevation of malondialdehyde (MDA) in a dose- and time-dependent manner. The increased level of MDA might be attributed to the generation of free radicals that reacted with the cellular and/or organelle membranes, initiating lipid peroxidation cascade reaction leading to an increased lipid peroxidation. Increase in the lipid peroxidation due to selenium intoxication is consistent with reported observations by Hoffman [Citation34] and Balogh et al. [Citation35]. They have reported an increase in LP in mallard ducklings and chicken in response to excess dietary selenite. It has been reported that increase in LP indicates tissue damage in several organs [Citation37]. Histologically, the hepatocytes of selenite-treated rats showed hepatic steatosis or fatty change. This fatty change increased in a dose- and time-dependent manner. Hepatic steatosis is one of the most common examples of liver injury. The increase of lipid peroxidation level has been associated with the development of hepatic steatosis [Citation38,Citation39].
4.2. Selenium and catalase activity
Catalase is an antioxidant enzyme that serves as one of the body’s defense systems against H2O2. The results of the present study showed that treatment with sodium selenite caused a significant decrease of catalase activity at exposure of 12 h post administration with all doses. However, after 30 h, the catalase activity increased. This might be due to the consequent accumulation of hydrogen peroxide after a period of decreased catalase activity. Escribano et al. [Citation40] have explained the decreased catalase activity by the decreased glutathione content leading to an accumulation of hydrogen peroxide. Inorganic selenium can combine with tissue thiols, such as glutathione, to produce selenotrisulphides and free radicals, such as superoxide anion [Citation41].
4.3. Selenium and Cu/Zn SOD activity
Superoxide dismutases form the first line of defense system against reactive oxygen species. They catalyze the reduction of the superoxide anion radical () to hydrogen peroxide and molecular oxygen. The results of the present study showed that treatment with sodium selenite caused a significant decrease of SOD activity in most cases. The most potent effect of sodium selenite on SOD activity was observed after 30 h with the LD50 treatment, where its activity significantly increased. The decrease in the activity of SOD might occur under the influence of accumulation of hydrogen peroxide after a period of increased activity. The increased SOD activity at the higher sodium selenite dose and longer period might be attributed to the removal of hydrogen peroxide by both CAT and GPx, and continuous free radical flux [Citation42]. For Cu/Zn SOD, there are intracellular SOD1, also called cytosolic SOD1 (found primarily in the cytoplasm and also present within the nucleus and mitochondrial intermembrane space), and extracellular SOD3 isoforms. The latter SOD form is normally anchored to the extracellular matrix (ECM) via heparan sulfate proteoglycans and collagen but can be freed up to the circulation [Citation43]. The elevated levels of plasma SOD might occur as a result of an increase in SOD1 activity possibly due to hepatic tissue damage and/or RBC lysis. Elevated levels of plasma SOD1 have been reported in patients with coronary artery disease [Citation44]. The ultimate increase in the plasma SOD level might also be attributed to SOD3 activity which could be a sign of tissue damage, heart, lung, and kidney being possible sources, and changes in ECM in the circulatory system [Citation43,Citation45,Citation46]. Identification of the isoform of the SOD elevated in the plasma due to selenosis could be a target of further investigation.
4.4. Selenium and GPx activity
Glutathione peroxidase, an antioxidant enzyme containing selenocysteine residues, works together with superoxide dismutase in the presence of reduced glutathione to transform the highly harmful reactive oxygen species and H2O2 into water [Citation47,Citation48]. In the present study, the activity of GPx in selenium-treated rats increased significantly after 12 and 30 h from treatment with all doses applied in a dose- and time-dependent manner. The obtained results agree with the findings of Balogh et al. [Citation35] who reported significant increase in GPx level in chicken consuming high dietary selenite intake. Oxidative stress has been proposed as the initiating event of embryo mortality and teratological effects in avian species exposed to selenium [Citation34,Citation49]. The increased plasma level of GPx could be a marker for pathology. For GPx, GPx3 is the circulating form in plasma, although some major fractions are anchored to the extracellular matrix (ECM) of many organs, in particular the kidney [Citation50]. The likely increase in the circulating GPx3 is a sign of kidney damage, and large scale and wide-spread changes in GPx3-ECM binding. Tham et al. [Citation51] have reported significant increase in plasma and renal GPx3 levels in dextran sodium sulfate-treated mice. The increase in the plasma GPx activity might also be due to liver/RBC GPx1 activity. In this case, the increase in GPx activity reflects tissue damage in the liver and RBC lysis. Indeed, the current histological study revealed severe damage in the liver of selenium-treated rats particularly with the higher dose and longer period. High plasma GPx1 level has been demonstrated in patients with acute cholecystitis [Citation52]. Further investigation is needed to determine whether the increase in plasma GPx activity in selenium-intoxicated rats was due to the activity of GPx1 and/or GPx3.
4.5. Liver enzymes
Liver enzymes are generally used in humans and animals as indicators of liver diseases and diagnosis as well as liver response to toxic drugs [Citation53,Citation54]. In the present study, treatment with sodium selenite significantly increased the activity of AST, ALT and GGT in most experimental groups in a dose- and time-dependent manner. The elevation in AST, ALT and GGT activities observed in selenite-treated groups could be due to hepatocellular damage particularly with the higher concentration and the longer time period. It has been reported that liver enzymes are generally increased in situations where there is a damage to the liver [Citation55–57]. When diseases or injuries affect the liver, these enzymes are released into circulation [Citation58]. Thus, the elevation in AST, ALT and GGT activities in the present study could be indicators of liver injury as a response to selenite administration.
Indeed, In the present study, selenium administration resulted in hepatic changes as indicated histologically by impaired structural organization, hepatic steatosis, feathery degeneration, dilated and congested blood vessels and disrupted hepatic sinusoids with hemorrhage formation. Leukocytic infiltration and necrotic changes have also been observed. Yang et al. [Citation59] have shown the link between nonalcoholic fatty liver disease and the increased plasma selenium levels. The observation that increased lipid peroxidation and hepatic steatosis are associated with the elevated transaminase levels is consistent with previous studies [Citation60,Citation61]. Reactive oxygen species (ROS) generated from oxidative stress can induce oxidative damage to vital cellular molecules and structures including DNA, proteins and membranes [Citation62]. Selenium toxicosis causes DNA oxidative damage by generating 8-hydroxyguanosine DNA, where guanine is the most sensitive base to free radical attack [Citation63]. Protein carbonylation, a type of protein oxidation, can be induced by both reactive oxygen species and lipid peroxidation. Protein carbonylation can mediate redox signaling processes which lead to cellular damage and pathogenesis [Citation64,Citation65]. As an effect of the oxidative stress, membranes of the cell’s organelles lose their integrity, thus lysosomal enzymes can release causing necrotic damage in tissues [Citation35]. Therefore, it is proposed that oxidative stress is linked to the organ damage following exposure to selenium intoxication.
4.6. Supplementation of vitamin E
Vitamin E is commonly used as one of the most potent antioxidants. In the present study, vitamin E (DL-α-Tocopherol acetate) was used to examine its potential protection against selenium intoxication. The current data showed that vitamin E significantly alleviated the toxic effects of sodium selenite and lowered the elevated lipid peroxidation levels. Vitamin E supplementation improved the oxidant buffering capacity and significantly altered the affected activity levels of antioxidant enzymes to be close to the normal levels. The elevated liver transaminase levels have been significantly lowered following vitamin E supplementation. The histological examination of liver after vitamin E administration showed a great reduction in hepatic steatosis, feathery degeneration, lobular inflammation and necrotic changes. Thus, the current data reflect the expected effects of vitamin E as an antioxidant which can help prevent the oxidative stress-mediated liver injury. The antioxidant effect of vitamin E is attributed to the hydroxyl group which can donate hydrogen to reduce the destructive free radicals [Citation66]. Various levels of post-transcriptional and/or post-translational controls of vitamin E have been suggested by Ryan et al. [Citation67] as a mechanism for regulation of antioxidant enzymes.
The current data are in agreement with previous works showing that vitamin E supplementation protects lipids from oxidative damage, and improves the antioxidant capacity and liver functions in a variety of experiments and diseases. In nonalcoholic steatohepatitis (NASH), vitamin E therapy significantly reduced the oxidative stress, lowered serum transaminase levels and attenuated hepatic steatosis [Citation61]. Moreover, chickens fed a high-oxidant diet and supplemented with vitamin E were able to stabilize the increased hepatic transaminase levels [Citation68]. In addition, some studies have proved that vitamin E can regulate the entry of fatty acids into the liver [Citation69]. In human studies, vitamin E has been used to treat hepatic steatosis, with reported improvement in liver transaminase levels [Citation60,Citation70]. The study of mitigation of selenium toxicity by vitamin E through assessment of liver oxidative stress, protein oxidation and the immunological status as well as analysis of the immunohistochemical and ultrastructural features of liver remains an open subject for further investigations.
5. Conclusion
The present results reflect the toxicity of selenium to the liver tissue when taken in excess. This may be attributed to the increased generation of free radicals and disturbance of antioxidant enzymes. The range between dietary deficiency and toxicity is narrow, therefore, proper selenium concentration in food is important when considering supplementation in human population. The data demonstrate that supplementation with vitamin E was effective for decreasing lipid peroxidation, attenuating the oxidative stress and lowering the elevated transaminase levels. This suggests that vitamin E as a free radical scavenger can provide an effective protection in animals and humans against oxidative damage and hepatotoxicity induced by selenosis.
Acknowledgements
The authors are grateful to the Department of Biology, College of Science, Taibah University, for the support during the investigation. The authors also would like to thank the Department of Biology, Faculty of Science, King Abdulaziz University, for the continuous cooperation. Great thanks to Prof. Wael Alhalawany, Ain Shams University, Department of Zoology, Cairo, Egypt, for his critical reading of the manuscript and suggestions.
Disclosure statement
No potential conflict of interest was reported by the author(s).
Correction Statement
This article has been republished with minor changes. These changes do not impact the academic content of the article.
References
- Rotruck JT, Pope AL, Ganther HE, et al. Selenium: biochemical role as a component of glutathione peroxidase. Science. 1973;179:588–590. doi: 10.1126/science.179.4073.588
- Yang G, Chen J, Wen Z, et al. The role of selenium in Keshan disease. Adv Nutr Res. 1984;6:203–231. doi: 10.1007/978-1-4613-2801-8_8
- Barceloux DG. Selenium. J Toxicol Clin Toxicol. 1999;37:145–172. doi: 10.1081/CLT-100102417
- Nuttall KL. Evaluating selenium poisoning. Ann Clin Lab Sci. 2006;36:409–420.
- Xia Y, Hill KE, Byrne DW, et al. Effectiveness of selenium supplements in a low-selenium area of China. Am J Clin Nutr. 2005;81:829–834. doi: 10.1093/ajcn/81.4.829
- Papadomichelakis G, Zoidis E, Pappas AC, et al. Dietary organic selenium addition and accumulation of toxic and essential trace elements in liver and meat of growing rabbits. Meat Sci. 2018;145:383–388. doi: 10.1016/j.meatsci.2018.07.022
- Gerberding JL. Agency for toxic substances and disease registry (ATSDR). Toxicological profile for selenium. Atlanta (GA): Department of Health and Human Services, Public Health Service; 2003.
- Goyer RA. Toxic effects of metals. In: MO Amdur, JD Doull, CD Klaassen, editor. Casarett and doull’s toxicology. New York: Pergamon Press; 1991. p. 623–680.
- Munguti CM, Alrifai M, Shaheen W. A rare cause of cardiomyopathy: a case of selenium deficiency causing severe cardiomyopathy that improved on supplementation. Cureus. 2017;9:e1627.
- Li Q, Liu M, Hou J, et al. The prevalence of Keshan disease in China. Int J Cardiol. 2013;168:1121–1126. doi: 10.1016/j.ijcard.2012.11.046
- Zhang X, Wang T, Li S, et al. A spatial ecological study of selenoprotein P and Keshan disease. Epid. 2019;51:150–158.
- Moreno-Reyes R, Suetens C, Mathieu F, et al. Kashin-Beck disease and iodine deficiency in Tibet. Int Orthop. 2001;25:164–166. doi: 10.1007/s002640000216
- Yao Y, Pei F, Kang P. Selenium, iodine, and the relation with Kashin-Beck disease. Nutrition. 2011;27:1095–1100. doi: 10.1016/j.nut.2011.03.002
- Tinggi U. Essentiality and toxicity of selenium and its status in Australia: a review. Toxicol Lett. 2003;137:103–110. doi: 10.1016/S0378-4274(02)00384-3
- Kim Y, Mahan DC. Comparative effects of high dietary levels of organic and inorganic selenium on selenium toxicity of growing-finishing pigs. J Anim Sci. 2001;79:942–948. doi: 10.2527/2001.794942x
- Ceballos-Marquez A, Barkema HW, Stryhn H, et al. Milk selenium concentration and its association with udder health in Atlantic Canadian dairy herds. J Dairy Sci. 2010;93:4700–4709. doi: 10.3168/jds.2010-3313
- Aldosary BM, Sutter ME, Schwartz M, et al. Case series of selenium toxicity from a nutritional supplement. Clin Toxicol. 2012;50:57–64. doi: 10.3109/15563650.2011.641560
- Irland BG, Martens P, Barbara R, et al. Selenium status in preschool children receiving a Brazil nut-enriched diet. Nutrition. 2015;31:1339–1343. doi: 10.1016/j.nut.2015.05.005
- Dhillon KS, Dhillon SK, Bijay-Singh. Genesis of seleniferous soils and associated animal and human health problems. Adv Agron. 2019;154:1–80. doi: 10.1016/bs.agron.2018.11.001
- Yang G, Yin S, Zhou R, et al. Studies of safe maximal daily dietary Se-intake in a seleniferous area in China. Part II: relation between Se-intake and the manifestation of clinical signs and certain biochemical alterations in blood and urine. J Trace Elem Electrolytes Health Dis. 1989;3:123–130.
- McDowell LR, Wilkinson N, Madison R, et al. Vitamins and minerals functioning as antioxidants with supplementation considerations. Florida Ruminant Nutrition Symposium. Best Western Gateway Grand. Gainesville, FL, 30-31 January. 2007: 1–17. https://conference.ifas.ufl.edu/ruminant/index07.html.
- Lobo V, Patil A, Phatak A, et al. Free radicals, antioxidants and functional foods: impact on human health. Pharmacogn Rev. 2010;4:118–126. doi: 10.4103/0973-7847.70902
- Finney D. Probit analysis. 3rd ed. Cambridge: Cambridge University Press; 1971.
- Naghibi B, Ghafghazi T, Hajhashemi V, et al. The effect of vitamin E in prevention of vancomycin-induced nephrotoxicity in rats. Res Pharm Sci. 2006;2:104–111.
- Hoff J, Rlatg L. Methods of blood collection in the mouse. J Lab Anim. 2000;29:47–53.
- Ohkawa H, Ohishi N, Yagi K. Assay for lipid peroxides in animal tissues by thiobarbituric acid reaction. Anal Biochem. 1979;95:351–358. doi: 10.1016/0003-2697(79)90738-3
- Aebi H. Catalase in vitro. Methods Enzymol. 1984;105:121–126. doi: 10.1016/S0076-6879(84)05016-3
- Nishikimi M, Appaji N, Yagi K. The occurrence of superoxide anion in the reaction of reduced phenazine methosulfate and molecular oxygen. Biochem Biophys Res Commun. 1972;46:849–854. doi: 10.1016/S0006-291X(72)80218-3
- Paglia DE, Valentine WN. Studies on the quantitative and qualitative characterization of erythrocyte glutathione peroxidase. J Lab Clin Med. 1967;70:158–169.
- Schumann G, Bonora R, Ceriotti F, et al. IFCC primary reference procedures for the measurement of catalytic activity concentrations of enzymes at 37°C. Part 5. Reference procedure for the measurement of catalytic concentration of aspartate aminotransferase. Clin Chem Lab Med. 2002;40:725–733.
- Schumann G, Bonora R, Ceriotti F, et al. IFCC primary reference procedures for the measurement of catalytic activity concentrations of enzymes at 37°C. Part 4. Reference procedure for the measurement of catalytic concentration of alanine aminotransferase. Clin Chem Lab Med. 2002;40:718–724.
- Schumann G, Bonora R, Ceriotti F, et al. IFCC primary reference procedures for the measurement of catalytic activity concentrations of enzymes at 37°C. Part 6. Reference procedure for the measurement of catalytic concentration of γ-glutamyltransferase. Clin Chem Lab Med. 2002;40:734–738.
- Bancroft JD, Gamble M. Theory and practice of histological techniques. 6th ed. London: Churchill Livingstone; 2007.
- Hoffman DJ. Role of selenium toxicity and oxidative stress in aquatic birds. Aquat Toxicol. 2002;57:11–26. doi: 10.1016/S0166-445X(01)00263-6
- Balogh K, Weber M, Erdélyi M, et al. Investigation of lipid peroxide and glutathione redox status of chicken concerning on high dietary selenium intake. Acta Biol Hung. 2007;58:269–279. doi: 10.1556/ABiol.58.2007.3.3
- Yin H, Xu L, Porter N. Free radical lipid peroxidation: mechanisms and analysis. Chem Rev. 2011;111:5944–5972. doi: 10.1021/cr200084z
- Sener G, Jahovic N, Tosun O, et al. Melatonin meliorates ionizing radiation-induced oxidative organ damage in rats. Life Sci. 2003;74:563–572. doi: 10.1016/j.lfs.2003.05.011
- Feldstein AE, Lopez R, Tamimi TA, et al. Mass spectrometric profiling of oxidized lipid products in human nonalcoholic fatty liver disease and nonalcoholic steatohepatitis. J Lipid Res. 2010;51:3046–3054. doi: 10.1194/jlr.M007096
- Bell LN, Molleston JP, Morton MJ, et al. Hepatic lipid peroxidation and cytochrome P-450 2E1 in pediatric nonalcoholic fatty liver disease and its subtypes. J Clin Gastroenterol. 2011;45:800–809. doi: 10.1097/MCG.0b013e31821377e4
- Escribano A, Amor M, Pastor S, et al. Decreased glutathione and low catalase activity contribute to oxidative stress in children with α−1 antitrypsin deficiency. Thorax. 2015;70:82–93. doi: 10.1136/thoraxjnl-2014-205898
- Seko Y, Imura N. Active oxygen generation as a possible mechanism of selenium toxicity. Biomed Environ Sci. 1997;10:333–339.
- Amri F, Ghouili I, Amri M, et al. Neuroglobin protects astroglial cells from hydrogen peroxide-induced oxidative stress and apoptotic cell death. J Neurochem. 2017;140:151–169. doi: 10.1111/jnc.13876
- Folz RJ, Crapo JD. Extracellular superoxide dismutase (SOD3): tissue-specific expression, genomic characterization, and computer-assisted sequence analysis of the human EC SOD gene. Genomics. 1994;22:162–171. doi: 10.1006/geno.1994.1357
- Peng J-R, Lu T-T, Chang H-T, et al. Elevated levels of plasma superoxide dismutases 1 and 2 in patients with coronary artery disease. Biomed Res Int. 2016;2016, Article ID 3708905, 9 pages.
- Sandstrom J, Nilsson P, Karlsson K, et al. 10-fold increase in human plasma extracellular superoxide dismutase content caused by a mutation in heparin-binding domain. J Biol Chem. 1994;269:19163–19166.
- Stocker R, Keaney Jr JF. Role of oxidative modifications in atherosclerosis. Physiol Rev. 2004;84:1381–1478. doi: 10.1152/physrev.00047.2003
- Griffith OW. Biologic and pharmacologic regulation of mammalian glutathione synthesis. Free Radic Biol Med. 1999;27:922–935. doi: 10.1016/S0891-5849(99)00176-8
- Fitzpatrick AM, Teague WG, Holguin F, et al. Airway glutathione homeostasis is altered in children with severe asthma: evidence for oxidant stress. J Allergy Clin Immunol. 2009;123:146–152. doi: 10.1016/j.jaci.2008.10.047
- Spallholz JE, Hoffman DJ. Selenium toxicity: cause and effects in aquatic birds. Aquat Toxicol. 2002;57:27–37. doi: 10.1016/S0166-445X(01)00268-5
- Olson GE, Whitin JC, Hill KE, et al. Extracellular glutathione peroxidase (Gpx3) binds specifically to basement membranes of mouse renal cortex tubule cells. Am J Physiol Renal Physiol. 2010;298:F1244–F1253. doi: 10.1152/ajprenal.00662.2009
- Tham DM, Whitin JC, Cohen HJ. Increased expression of extracellular glutathione peroxidase in mice with dextran sodium sulphate-induced experimental colitis. Pediatr Res. 2002;51:641–646. doi: 10.1203/00006450-200205000-00016
- Kärkkäinen J, Aspinen S, Harju J, et al. Plasma glutathione peroxidase (GPX1) levels and oxidative stress in gallstone patients operated with two different cholecystectomy techniques: a randomized study with special reference to cancer patients. Anticancer Res. 2017;37:6921–6927.
- Kim K, Park J. Antihepatic effect of Artemisia iwayomogi methanol extract on acute hepatic injury by carbon tetrachloride in rat. Korean J Vet Res. 1994;34:619–626.
- Malomo SO. Toxicological implications of ceftriaxone administration in rats. Nig J Biochem Mol Biol. 2000;15:33–38.
- Schumann G, Aoki R, Ferrero CA, et al. IFCC primary reference procedures for the measurement of catalytic activity concentrations of enzymes at 37°C. Clin Chem Lab Med. 2006;44:1146–1155. doi: 10.1515/CCLM.2006.212
- Makhlouf R, Makhlouf I. Evaluation of the effect of Spirulina against Gamma irradiation induced oxidative stress and tissue injury in rats. Int J Appl Sci Eng Res. 2012;1:152–164.
- Abdelhalim MA, Moussa SA. The biochemical changes in rats’ blood serum levels exposed to different gamma radiation doses. African J Pharm Pharmacol. 2013;7:785–792. doi: 10.5897/AJPP2013.3434
- Adaramoye OA, Awogbindin I, Okusaga JO. Effect of kolaviron, a biflavonoid complex from Garcinia kola seeds on ethanol-induced oxidative stress in liver of adult Wistar rats. J Med Food. 2009;12:584–590. doi: 10.1089/jmf.2008.0138
- Yang Z, Yan C, Liu G, et al. Plasma selenium levels and nonalcoholic fatty liver disease in Chinese adults: a cross-sectional analysis. Sci Rep. 2016;6:1–8. doi: 10.1038/s41598-016-0001-8
- Dufour JF, Oneta CM, Gonvers JJ, et al. Swiss Association for the study of the liver. Randomized placebo-controlled trial of ursodeoxycholic acid with vitamin E in nonalcoholic steatohepatitis. Clin Gastroenterol Hepatol. 2006;4:1537–1543. doi: 10.1016/j.cgh.2006.09.025
- Nan YM, Wu WJ, Fu N, et al. Antioxidants vitamin E and 1-aminobenzotriazole prevent experimental non-alcoholic steatohepatitis in mice. Scand J Gastroenterol. 2009;44:1121–1131. doi: 10.1080/00365520903114912
- Cadet J, Bellon S, Douki T, et al. Radiation-induced DNA damage: formation, measurement, and biochemical features. J Environ Pathol Toxicol Oncol. 2004;23:33–43. doi: 10.1615/JEnvPathToxOncol.v23.i1.30
- Kim Y, Jhon D, Lee K-Y. Involvement of ROS and JNK1 in selenite-induced apoptosis in Chang liver cells. Exp Mol Med. 2004;36:157–164. doi: 10.1038/emm.2004.22
- Dalle-Donne I, Scaloni A, Giustarini D, et al. Proteins as biomarkers of oxidative/nitrosative stress in diseases: the contribution of redox proteomics. Mass Spectrom Rev. 2005;24:55–99. doi: 10.1002/mas.20006
- Baraibar MA, Ladouce R, Friguet B. Proteomic quantification and identification of carbonylated proteins upon oxidative stress and during cellular aging. J Proteomics. 2013;92:63–70. doi: 10.1016/j.jprot.2013.05.008
- Peh HY, Tan WS, Liao W, et al. Vitamin E therapy beyond cancer: tocopherol versus tocotrienol. Pharmacol Ther. 2016;162:152–169. doi: 10.1016/j.pharmthera.2015.12.003
- Ryan MJ, Dudash HJ, Docherty M, et al. Vitamin E and C supplementation reduces oxidative stress, improves antioxidant enzymes and positive muscle work in chronically loaded muscles of aged rats. Exp Gerontol. 2010;45:882–895. doi: 10.1016/j.exger.2010.08.002
- Lu T, Harper AF, Zhao J, et al. Effects of a dietary antioxidant blend and vitamin E on fatty acid profile, liver function, and inflammatory response in broiler chickens fed a diet high in oxidants. Poult Sci. 2014;93:1658–1666. doi: 10.3382/ps.2013-03827
- Podszun MC, Grebenstein N, Spruss A, et al. Dietary alpha-tocopherol and atorvastatin reduce high-fat-induced lipid accumulation and down-regulate CD36 protein in the liver of guinea pigs. J Nutr Biochem. 2014;25:573–579. doi: 10.1016/j.jnutbio.2014.01.008
- Foster T, Budoff MJ, Saab S, et al. Atorvastatin and antioxidants for the treatment of nonalcoholic fatty liver disease: the St Francis heart study randomized clinical trial. Am J Gastroenterol. 2011;106:71–77. doi: 10.1038/ajg.2010.299