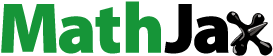
ABSTRACT
Considering the increasing incidence of fatal aluminium phosphide (ALP) poisoning and its toxicological aspects and also the paucity of our knowledge concerning the ALP effect on adipose tissue and adipokines, the present study aimed to assess the effect of acute and sub-chronic ALP inhalation exposure on adipose tissue characteristics and histological alterations in rats. This experimental study was performed on 40 male Wistar rats weighing 150–200 g. They were randomly divided into four groups (n = 10) including inhalation exposure groups to 5.67 mg/m3 (2.4 ppm) ALP for 6 h, one week and four weeks, respectively, and a control group. At the beginning and end of the study, body weight and fasting blood sugar (FBS) of animals were determined. Then, the animals were anaesthetized. The specimens of perirenal and visceral (perigonadal) adipose tissues by the routine histological process for haematoxylin and eosin (H&E) staining were determined. The results showed that the histopathological alteration of adipose tissue was significantly different between the study groups (p < 0.05). Compared to the control group, the numbers of adipocytes in perirenal area were dramatically increased in 6 h ALP exposure(p < 0.001). In agreement with the cell number changes, cell size in the perirenal area in the 6h-ALP group was markedly lower than control group (p < 0.001). Meanwhile, there was no statistically difference in the cell number of adipocytes in gonadal area between control and 6h-ALP groups(p = 0.071). Final FBS increased significantly in 6-h and 4 weeks compared to the control group (p < 0.0001). Total cholesterol and triglyceride levels decreased significantly in 4 weeks and 6h-ALP exposure in comparison with the control group (p < 0.0001) also, LDL and HDL levels in 6 h and 4 weeks’ ALP exposure and in 4 weeks ALP exposure had a significant decrease compared with the control group, respectively (p = 0.03 and p = 0.001 and p < 0.0001). ALP poisoning had a significant association with alterations in physiological and histological parameters.
1. Introduction
Aluminium phosphide (ALP), also known as rice tablet in Iran, is used throughout the world as a fumigant, rodenticide, pesticide, and insecticide for the control of insects and rodents through the fumigation of agricultural commodities, animal feeds, and processed foods [Citation1,Citation2]. In many developing countries, especially in Asia, ALP causes a high fatality rate (60–90%) [Citation3,Citation4]. A recent 8-year study showed an increased incidence of fatal ALP poisoning from 2006 to 2013 in Tehran, which included 2007 cases of phosphine poisoning-related deaths [Citation5]. The majority of ALP poisoning cases in Iran involve intentional acts of suicides although some accidental cases of ALP poisoning occur through the occupational exposure [Citation3].
After being ingested, solid phosphides, including ALPs in contact with hydrochloric acid of the stomach or following any contact with water, even moisture in the air, produce the toxic phosphine gas (PH3) that mainly leads to mitochondrial damage by inhibiting cytochrome C oxidase; thus, phosphine is considered a respiratory poison. ALP, as an extremely toxic pesticide, can inhibit the cytochrome-C oxidase activity and perturb the mitochondrial morphology. [Citation6,Citation7]. In the study conducted by Newton et al., 344 male and female Fischer rats were exposed to single 6-h acute and sub-chronic 1% phosphine in nitrogen mixture at 0, 2.5, 5, and 10 ppm for 4 and 13 weeks in their chambers [Citation8]. The results showed decreased erythrocytes, lung congestion, and increased kidney weight with coagulative necrosis of the tubular epithelium in the outer cortex observed in 70 ppm-exposed rats only. In addition, the effects were more severe in females than in males.
The poisoning with metal phosphides, exclusively ALP, has been reported worldwide, but its exact mechanism is still uncertain [Citation9–12]. Moreover, oxidative stress is another suggested mechanism involved in the poisoning [Citation13]. Hence, these events can lead to a profound circulatory collapse in cardiac myocytes and adrenal gland as the main cause of mortality and morbidity [Citation6,Citation14,Citation15]. Phosphine can form extremely reactive hydroxyl radicals and reduce peroxidase and catalase generation to inhibit lipid peroxidation [Citation13]. More studies also showed that ALP might block acetyl cholinesterase function [Citation16]. Therefore, considering the increasing incidence of fatal ALP poisoning and its toxicological aspects in Iran and the paucity of studies concerning the ALP effect on adipose tissue and adipokines, the present study aimed to assess the effect of acute and sub-chronic ALP inhalation exposure on adipose tissue characteristics and histological alterations in rats.
2. Methods
2.1. Animals
This study was performed at the Research Center of Experimental Medicine, Birjand University of Medical Sciences in Iran, on 40 male Wistar rats weighing 150–200 g with free access to food and water ad libitum. The rats had seven days of acclimatization to the laboratory conditions. Then, they were randomly divided into four groups (n = 10 each) including, 1- acute inhalation exposure to 5.67 mg/m3 (2.4 ppm) Phosphide for long 6 hours 2- inhalation exposure to 5.67 mg/m3 (2.4 ppm) Phosphide 1.5 hours on the day for one week, 3- inhalation exposure to 5.67 mg/m3 (2.4 ppm) Phosphide 1.5 hours on the day, 5 days in a week for 4 weeks and 4- the control group.
2.2. Chemicals
Phosphide tablets were prepared from BHOSTOXIN Batch NO. 2140520. Phosphide exposure was given through inhalation in an enclosed chamber. To do so, the phosphide tablets were placed in a small box above the enclosed chamber manufactured by the researcher, as shown in Figure .
2.3. Experimental protocol
Bodyweight of the animals in the beginning and end of the study was determined using a weighing scale with 0.01 g precision. In addition, fasting blood sugar (FBS) level was determined in the beginning and end of the study using a glucometer (ACCU-CHEK active model GC-Roche) from rat-tail. At the end of the experimental study, the animals were anaesthetized with ether; then, the wet weight of perirenal and visceral (perigonadal) adipose tissues was measured using a weighing scale with 0.01 g precision. The specimens were fixed in natural buffered formalin 4%, and the paraffin blocks were cut in slices of 4 µm and prepared by a routine histological process for haematoxylin and eosin (H&E) staining. Blood samples were collected through cardiac puncture for the measurement of serum leptin by using the rat leptin kit (Zellbio, GmbH, Germany) with a minimum sensitivity of the assay of 0.09 ng/mL also, low-density lipoprotein (LDL-C), high-density lipoprotein (HDL-C), triglyceride level and total cholesterol by using Auto-analyzer Prestige 24i, Japan.
2.4. Quantitative histology of adipose tissue
The cell number (per 20,000 µm2) and cell size were determined in visceral and perirenal adipose tissues using Image Software (1.44; NIH, USA). Briefly, for each rat, three slides were evaluated using a light microscope (Upland, F1, Japan) equipped with a digital camera (Image Focus V2, Netherland). For each section 4–6, an unbiased counting frame was sampled and evaluated blindly.
2.5. Pathological investigation
Organs, including livers, lungs, pancreases, and visceral and perirenal adipose tissues, were harvested and prepared by a routine histological process with H&E staining and examined with light microscopy (Olympus Bx51).
2.6. Determination of phosphine gas concentration
For the determination of phosphine in the air of the chamber, we used the NIOSH Manual of Analytical Methods (NMAM), Fifth Edition; METHOD: 6002, Issue 3. This method employs a sorbent tube containing the silica gel (mercuric cyanide), 300 mg/150 mg sorbent, 40/60 mesh, used to capture phosphine and spectrophotometrically analyze the total phosphorus at the wavelength of 625 nm. The concentration of phosphine was calculated using the following equation:
where C is the concentration of phosphine (µg/L), Wf is the phosphine concentration found in the sample front sorbent section, Wb is the phosphine concentration found in the sample back sorbent section, Bf is the phosphine concentration found in the average media blank front sorbent section, and Bb is the phosphine concentration found in the average media blank back sorbent section and V is the sampled air volume (L).
2.7. Statistical analysis
In this study, SPSS version 18 software was used and the significance level was set at 5%. After the normal distribution of the data were assessed through the Kolmogorov–Smirnov test, the Kruskal–Wallis, and Mann–Whitney tests with post hoc Bonferroni correction were used for analyzing leptin and triglyceride variables. One-way ANOVA test was used to examine the differences in the ALP effects on groups with three exposure periods (six hours, one week, and four weeks) compared to the control group.
3. Results
The results of ALP effects on cell number and cell size of perirenal and perigonadal adipocytes of rats are shown in Table . Compared to the control group, the number of adipocytes in perirenal area was dramatically increased in 6 h ALP exposure (one-way analysis of variance (ANOVA) followed by Tukey’s post hoc test, p < 0.001). However, there was no statistically difference in the number of adipocytes in pregonadal area between the studied groups (different time-points). In agreement with the cell number changes, the results of cell size measurement showed that, in the perirenal area, the size of adipocytes in 6h-ALP group was markedly lower than that of the control group (one-way analysis of variance (ANOVA) followed by Tukey’s post hoc test, p < 0.001). Moreover, 4 weeks ALP exposure also slightly decreased the cell size of perirenal adipocyte in comparison with the control group (one-way analysis of variance (ANOVA) followed by Tukey’s post hoc test, p = 0.021). Meanwhile, there was no statistical difference in the cell number of adipocytes in gonadal area between control and 6h-ALP groups (one-way analysis of variance (ANOVA) followed by Tukey’s post hoc test, p = 0.071),
Table 1. Effect of aluminum phosphide (ALP) on cell number and cell size of adipocytes.
The effect of ALP on different biochemical parameters of the rats is presented in Table . Compared to the control group, final FBS increased in 6 h and 4 weeks ALP exposure (one-way analysis of variance (ANOVA) followed by Tukey’s post hoc test, p < 0.0001).
Table 2. Effect of aluminium phosphide on different parameters of biochemical in rats.
Total cholesterol levels decreased significantly in 4 weeks ALP exposure in comparison with the control group (one-way analysis of variance (ANOVA) followed by Tukey’s post hoc test, p < 0.0001) and triglyceride levels decreased significantly in 6 h ALP exposure in comparison with the control group (Kruskal–Wallis test followed by Mann–Whitney post hoc test with Bonferroni correction, p = 0.03, respectively). LDL levels in 6 h and 4 weeks ALP exposure had significant decrease compared with the control group (one-way analysis of variance (ANOVA) followed by Tukey’s post hoc test, p = 0.03 and p = 0.001, respectively) and HDL levels had significant decrease in 4 weeks ALP exposure compared with control groups (one-way analysis of variance (ANOVA) followed by Tukey’s post hoc test, p < 0.0001). Also, leptin levels in 6 h and 4 weeks ALP exposure were significantly lower than the control group (Kruskal–Wallis test followed by Mann–Whitney post hoc test with Bonferroni correction, p = 0.001).
The body weight in 6 h and 4 weeks ALP exposure was significantly lower and higher than the control group, respectively (one-way analysis of variance (ANOVA) followed by Tukey’s post hoc test, p < 0.0001).
Wet weight of prerenal adipose tissue in 4 weeks ALP exposure was significantly higher than the control group (one-way analysis of variance (ANOVA) followed by Tukey’s post hoc test, p < 0.0001). Moreover, wet weight of perigonadal adipose tissue in 6 h and 4 weeks ALP exposure was significantly lower and higher than the control group, respectively (one-way analysis of variance (ANOVA) followed by Tukey’s post hoc test, p = 0.03, p = 0.008). Histopathological damages to different tissues of the rats after being exposed to ALP are shown in Figures –.
Figure 2. Effect of aluminum phosphide (ALP) on cell number and cell size of adipocytes of perirenal and perigonadal area.
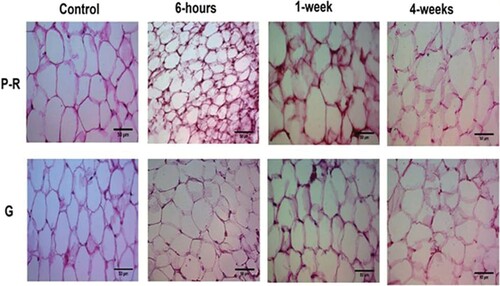
Figure 3. Liver tissue morphology in the rat in acute and sub-chronic period; (A): control group (×100) shows focal necrosis of the liver cells. The lobe of the vein is also seen in the lobular centre. Sinusoids are also dilated and there is no lupus necrosis, (B): One-week exposure (×400) severe necrosis and extensive necrosis of the liver are observed. The necrosis around the portal vein is probably due to the high amount of poison. Complex cells have increased in non-necrotic areas. Sinusoids are also disrupted. The lining of the vein of the lobular system is observed and cells have oedema, (C): 6h exposure (×100), (D): 4 weeks exposure (×100) damage to the liver cells is extensive and from the portal to the venous portal of the lobule centre, but is more intense around the vein of the lobular centre. Fatty change is also observed. Apoptosis and necrosis are observed remarkably in 10% of the liver cells, along with compensatory regenerative changes. The most important lesson of the necrosis of the bridge between the pleasures of the port, which indicates irreversible damage, leads to cirrhosis of the liver.
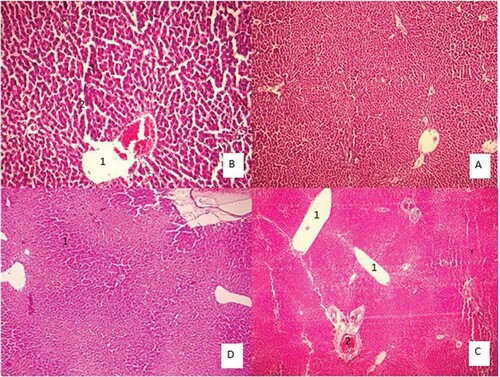
Figure 4. Lung tissue morphology in the rat in acute and sub-chronic period; (A): control group (×100), (B): One-week exposure (×400) shows alveolar walls are damaged. Contrary to the normal tissue, Type II pneumocystis increased and covers about 30% of the alveolar surface. Some alveoli are full of discharge and are seen in some types of bleeding, (C): 6h exposure (×100) shows Type II pneumocystis covers about 80% of the alveolar surface, resulting in a thickening of the alveoli. Spreading within the alveoli, severe vessel dilatation, and alveolar wall necrosis are also observed. Alveolar injury is more of an interstitial type that is in the vicinity of airways with greater intensity than near the pleural area, and (D): 4 weeks exposure (×40) shows a large number of interstitial (70% lung tissue) focuses on increasing intra-alveolar secretions. Pneumocystis Type II covers approximately 30% of the alveolar levels. Small haemorrhage can also be seen.
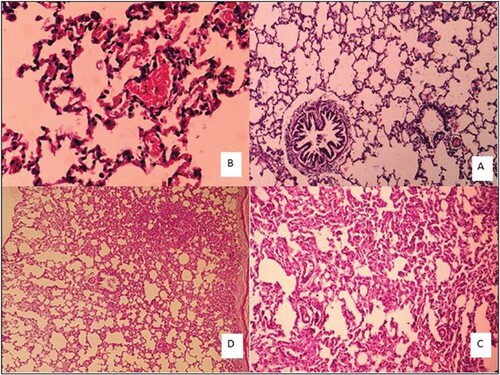
4. Discussion
4.1. Effect on histopathological characteristics
Our findings demonstrated that ALP, depending on the inhalation exposure period, exhibited significantly various effects on the histopathological process of poisoning more than the control group. Our results showed that the perirenal adipose tissues’ cell size was considerably reduced in the 6 h ALP exposure compared to the control group. In agreement with the cell size changes, the cell number of adipocytes in perirenal area was dramatically increased in 6 h ALP exposure. Also, in the present study leptin levels in 6 h and 4 weeks ALP exposure was significantly lower than the control group.
Some previous studies have shown that inhalation of phosphine can cause lipid peroxidation and cellular injury through oxidative damage, changes in cell morphology, weight loss and/or weight gain [Citation17]. Because adipose tissue besides being an energy storage site, it is considered as a significant site for toxicant bioaccumulation as toxicants are not released until lipolysis occurs [Citation18]. Also, in one study reporting no increased body mass after persistent organic pollutant (POP) exposure [Citation19] and in contrast, in the study performed by Arsenescu et al. has shown that with the administration of polychlorinated biphenyls (PCB-77) to mice occurred adipocyte hypertrophy and greater body weight [Citation20]. Additionally, adipose tissue, as an endocrine organ, has a major function for secretion of a wide range of proteins which are known to act both locally and systemically termed adipokines that they participate in body weight regulation and glucose and lipid homeostasis including leptin, adiponectin and [Citation21].
Leptin has direct and indirect effects on adipocyte metabolism resulting adipocyte size also, the capability to modify adipose tissue mass by influencing the number of cells and recent clinical trials have shown the correlation between adipocyte volume and leptin secretion and serum leptin concentrations; therefore, changes in adipocyte characteristics in our study can also be attributed to leptin levels which, in turn, are influenced by phosphine inhalation. In the study incubation of adult adipose tissue explants with Bisphenol A (BPA) inhibited adiponectin [Citation22] and in contrast, increase in leptin and adiponectin expression was reported in the study by Taxvig et al. on 3T3-L1 cells incubated with BPA [Citation23].
The body weight in 6 h and 4 weeks ALP exposure was significantly lower and higher than the control group respectively. Adipose tissue has a central role in the regulation of body weight [Citation18]; therefore, in 6 h ALP exposure group in which were adipocytes smaller than the control group resulting body weight was significantly lower than the control group. Against, in 4 weeks ALP exposure wet weight of prerenal and perigonadal adipose tissue was higher than the control group with higher body weight than the control group.
The histological image provided specific changes in most organs including the adipose, liver, lungs, and pancreatic tissues, and led to an irreversible lesion of necrosis. However, we obtained adequate evidence of significant histological damage to rats; for example, lung tissue morphology showed alveolar injury and small haemorrhage and liver tissue indicated extensive damage from the portal to the venous portal of the centre lobule that leads to cirrhosis of the liver. Anand et al. [Citation11] reported that stomach and renal medulla showed distended and congested appearance in the majority of cases in the ALP group.
Furthermore, haemorrhage and necrosis in lung and liver tissues of the exposed groups were noted [Citation2,Citation24]. Several pieces of evidence demonstrate that ALP leads to circulatory failure in most critical organs such as gastrointestinal, hepatic, respiratory, renal, and cardiac organs [Citation9,Citation10,Citation25–27]. Olurin et al. [Citation11] indicated that the liver tissue showed mild inflammatory changes at minimum concentrations; at the maximum concentration of exposure, there was noticeable inflammation through hydropic degeneration; moreover, kidney tissues indicated inflammatory condition only at the highest dose of exposure [Citation28, Citation29] illustrated that animals’ exposure to ALP leads to hazardous alterations in some histological and biochemical characteristics including liver and kidney. Furthermore, they confirmed that ALP treatment significantly reduces GSH (glutathione), GST (glutathione S-transferase), and CAT (catalase) in plasma. Also, α-lipoic acid combined with ALP could decrease the toxic effects in major parameters [Citation29].
4.2. Effect on biochemical parameters
Our results showed that compared to the control group, final FBS increased in 6 h and 4 weeks ALP exposure.
Several pieces of evidence show that leptin plays a primary role in the regulation of glucose homeostasis, independent of actions on food intake, energy expenditure or body weight and leptin or leptin receptor deficiency associated with perturbed glucose metabolism [Citation30,Citation31]. Therefore, increased FBS in our study is possibly associated with reduced leptin levels. Our result is in agreement with several studies on persistent organic pollutants (POPs) including Phthalate Esters and BPA [Citation32,Citation33] and is in contrast with the findings of Yousef et al. that showed decrease in glucose level of rats treated with aluminium phosphide [Citation34].
Total cholesterol, triglyceride, and LDL and HDL levels decreased significantly in 6 h or 4 weeks or both ALP exposure groups in comparison with the control group. Phosphine has a strong inhibitory effect on cytochrome c oxidase and mitochondrial respiratory chain which causes ATP depletion, disruption of cell homoeostasis, and finally cell death. Reactive oxygen species (ROS) and lipid peroxidation are involved in phosphine toxicity [Citation35].
Besides, it has been demonstrated that trichlorfon could reduce hepatic hormone-sensitive lipase, LDL, and APO-B100 by hepatic pathways in lipid metabolism and that it was correlated with the accumulation of lipids in the liver [Citation36]. Triglyceride is hydrolyzed in the mitochondria of liver cells by cyclic AMP-regulated lipases. Lipase is hormone-sensitive and can lipolyze the enzyme of lipid metabolism and mobilize TG and cholesterol ester stores in numerous tissues [Citation37]. Ching-Hung [Citation37] found that phosphine and antioxidant effects on GSH and GSSG levels are strongly paralleled by the rise in lipid peroxidation in the brain, liver, and lungs [Citation37]. The ALP produces cellular superoxide and peroxide radicals and leads to further cellular loss and lipid peroxidation. SOD (superoxide dismutase), MDA (Malondialdehyde), and catalase have a strong link with death [Citation38]. ALP is involved in complex IV of the electron transport chain (ETC) to slow down the electron flow throughout the ETC, while ALP results were more remarkable in vitro than in vivo. ALP can block glycerophosphate dehydrogenase, which leads to hydrogen generation [Citation39].
Generally, the phosphine gas is absorbed by simple diffusion in the gastrointestinal tract and mostly excreted by the kidneys and lungs [Citation40]. Even more, ALP poisoning by disrupting the mitochondrial process can inhibit cellular respiration and cholinesterase and induce oxidative stress in numerous mammalian and nematodes [Citation41,Citation42]. On the other hand, the blocking of cytochrome oxidase, peroxidase, and catalase can lead to the hydrogen peroxide formation and elevated MDA and SOD [Citation43,Citation44]. Moreover, numerous chromosomal abnormalities might alter the total antioxidant capacity level due to ALP poisoning [Citation45]. The ALP-induced generation of reactive oxygen species (ROS) could be associated with energy insufficiency [Citation46,Citation47].
5. Conclusion
Our study indicated that the inhalation exposure of experimental animals (rats) to ALP as time-dependent manner induced marked histopathological alterations in some organs including adipose tissue characteristics (cell number and cell size), liver, lung and pancreas also, some biochemical parameters (including FBS, lipid profile, and leptin levels). Therefore, due to the lack of antidotes and effective therapeutic strategies for fatal toxicological aspects of ALP poisoning it seems, further research, about adipose tissue function considering it can be as a significant site for accumulation of lipophilic compounds, is essential.
Acknowledgements
The authors of this study gratefully acknowledge the Research Council of Birjand University of Medical Sciences for the financial support [Grant number 4286]. The contribution of the Student Research Committee is also sincerely appreciated.
Disclosure statement
No potential conflict of interest was reported by the author(s).
Additional information
Funding
References
- Sudakin D. Occupational exposure to aluminium phosphide and phosphine gas? A suspected case report and review of the literature. Hum Exp Toxicol. 2005;24(1):27–33.
- Mehrpour O, Dolati M, Soltaninejad K, et al. Evaluation of histopathological changes in fatal aluminum phosphide poisoning. Indian J Forensic Med Toxicol. 2008;2(2):34–36.
- Navabi SM, Navabi J, Aghaei A, et al. Mortality from aluminum phosphide poisoning in Kermanshah Province, Iran: characteristics and predictive factors. Epidemiol Health. 2018;40:e2018022–e.
- Alinejad S, Zamani N, Abdollahi M, et al. A narrative review of acute adult poisoning in Iran. Iran J Med Sci. 2017;42(4):327.
- Etemadi-Aleagha A, Akhgari M, Iravani FS. Aluminum phosphide poisoning-related deaths in Tehran, Iran, 2006 to 2013. Medicine. 2015;94(38): e1637.
- Bumbrah GS, Krishan K, Kanchan T, et al. Phosphide poisoning: a review of literature. Forensic Sci Int. 2012;214(1):1–6.
- Oghabian Z, Mehrpour O. Treatment of aluminium phosphide poisoning with a combination of intravenous glucagon, digoxin and antioxidant agents. Sultan Qaboos Univ Med J. 2016;16(3):e352–e355.
- Newton PE, Schroeder RE, Sullivan JB, et al. Inhalation toxicity of phosphine in the rat: acute, subchronic, and developmental. Inhal Toxicol. 1993;5(2):223–239.
- Chugh S, Ram S, Singhal H, et al. Significance of heart rate response in shock due to aluminium phosphide poisoning. J Assoc Physicians India. 1989;37(11):708–709.
- Proudfoot AT. Aluminium and zinc phosphide poisoning. Clin Toxicol. 2009;47(2):89–100.
- Anand R, Sharma D, Verma D, et al. Mitochondrial electron transport chain complexes, catalase and markers of oxidative stress in platelets of patients with severe aluminum phosphide poisoning. Hum Exp Toxicol. 2013;32(8):807–816.
- Ayobola A. Assessment of lipid peroxidation and activities of antioxidant enzymes in phosphide-powder resi-due exposed rats. J Drug Metab Toxicol. 2012;3(5):132.
- Nakhaee S, Mehrpour O, Balali-Mood M. Does N-acetyl cysteine have protective effects in acute aluminum phosphide poisoning? Indian J Crit Care Med. 2017;21:8.
- Costa RO, Ferreiro E, Oliveira CR, et al. Inhibition of mitochondrial cytochrome c oxidase potentiates Aβ-induced ER stress and cell death in cortical neurons. Mol Cell Neurosci. 2013;52:1–8.
- Maitai C, Njoroge D, Abuga K, et al. Investigation of possible antidotal effects of activated charcoal, sodium bicarbonate, hydrogen peroxide and potassium permanganate in zinc phosphide poisoning. East Centr Afr J Pharma Sci. 2002;5(2):38–41.
- Tehrani H, Halvaie Z, Shadnia S, et al. Protective effects of N-acetylcysteine on aluminum phosphide-induced oxidative stress in acute human poisoning. Clin Toxicol. 2013;51(1):23–28.
- Amiri H, Vaseie L, Habibollahi P, et al. Rice tablet: an overview to common material in Iran. J Res Clin Med. 2016;4(2):77–81.
- Jackson E, Shoemaker R, Larian N, et al. Adipose tissue as a site of toxin accumulation. Compr Physiol. 2011;7(4):1085–1135.
- La Merrill M, Birnbaum LS. Childhood obesity and environmental chemicals. Mount Sinai J Med. 2011;78(1):22–48.
- Arsenescu V, Arsenescu RI, King V, et al. Polychlorinated biphenyl-77 induces adipocyte differentiation and proinflammatory adipokines and promotes obesity and atherosclerosis. Environ Health Perspect. 2008;116(6):761–768.
- Kershaw EE, Flier JS. Adipose tissue as an endocrine organ. J Clin Endocrinol Metab. 2004;89(6):2548–2556.
- Hugo ER, Brandebourg TD, Woo JG, et al. Bisphenol A at environmentally relevant doses inhibits adiponectin release from human adipose tissue explants and adipocytes. Environ Health Perspect. 2008;116(12):1642–1647.
- Taxvig C, Dreisig K, Boberg J, et al. Differential effects of environmental chemicals and food contaminants on adipogenesis, biomarker release and PPARγ activation. Mol Cell Endocrinol. 2012;361(1-2):106–115.
- Anand R, Kumari P, Kaushal A, et al. Effect of acute aluminum phosphide exposure on rats – a biochemical and histological correlation. Toxicol Lett. 2012;215(1):62–69.
- Chugh S, Ram S, Mehta L, et al. Adult respiratory distress syndrome following aluminium phosphide ingestion. Report of 4 cases. J Assoc Physicians India. 1989;37(4):271–272.
- Darbari A, Kumar A, Chandra G, et al. Tracheo-oesophageal fistula with oesophageal stricture due to aluminium phosphide (Celphos tablet) poisoning. Indian J Chest Diseases Allied Sci. 2007;49(4):241.
- Farzaneh E, Ghobadi H, Akbarifard M, et al. Prognostic factors in acute aluminium phosphide poisoning: a risk-prediction nomogram approach. Basic Clin Pharmacol Toxicol. 2018;123(3):347–355.
- Olurin KB, Mbaka GO, Agbato OA. Histopathological effect of sub-lethal concentration of aluminum phosphide (phostoxin) on Clarias gariepinus juveniles. Pesquisa Vet Bras. 2016;36(7):574–580.
- Yousef MI, Soliman NF, El-Demerdash FM. Aluminium phosphide-induced hepato-nephrotoxicity and oxidative damage in rats: the protective effect of α-lipoic acid. Open Conf Proc J. 2015:18-23.
- Denroche HC, Huynh FK, Kieffer TJ. The role of leptin in glucose homeostasis. J Diabetes Investig. 2012;3(2):115–129.
- Hussain Z, Khan JA. Food intake regulation by leptin: mechanisms mediating gluconeogenesis and energy expenditure. Asian Pac J Trop Med. 2017;10(10):940–944.
- Sun Q, Cornelis MC, Townsend MK, et al. Association of urinary concentrations of bisphenol A and phthalate metabolites with risk of type 2 diabetes: a prospective investigation in the Nurses’ Health Study (NHS) and NHSII cohorts. Environ Health Perspect. 2014;122(6):616–623.
- Svensson K, Hernández-Ramírez RU, Burguete-García A, et al. Phthalate exposure associated with self-reported diabetes among Mexican women. Environ Res. 2011;111(6):792–796.
- Yousef MI, Soliman NF, El-Demerdash FM, editors. Aluminium phosphide-induced hepato-nephrotoxicity and oxidative damage in rats: the protective effect of α-lipoic acid. Open Conf Proc J; 2015.
- Rashedinia M, Jamshidzadeh A, Mehrabadi AR, et al. Prevention of phosphine-induced cytotoxicity by nutrients in HepG2 cells. Indian J Med Res. 2016;144(4):560.
- Xu W, Liu W, Shao X, et al. Effect of Trichlorfon on Hepatic Lipid Accumulation in Crucian Carp Carassius auratus gibelio. J Aquat Anim Health. 2012;24(3):185–194.
- Yeaman SJ. Hormone-sensitive lipase – new roles for an old enzyme. Biochem J. 2004;379(1):11–22.
- Moghadamnia AA. An update on toxicology of aluminum phosphide. DARU Journal of Pharmaceutical Sciences. 2012;20(1):25.
- Salimi A, Paeezi M, Yousefsani BS, et al. Inhibition of glucose-6-phosphate dehydrogenase protects hepatocytes from aluminum phosphide-induced toxicity. Pestic Biochem Physiol. 2017;143:141–146.
- Rigobello MP, Scutari G, Boscolo R, et al. Induction of mitochondrial permeability transition by auranofin, a Gold (I)-phosphine derivative. Br J Pharmacol. 2002;136(8):1162–1168.
- Shakeri S, Mehrpour O. Aluminum phosphide poisoning in animals. Int J Med Toxicol Forensic Med. 2014;5(2 (Spring)):81–97.
- Mittra S, Peshin S, Lall S. Cholinesterase inhibition by aluminium phosphide poisoning in rats and effects of atropine and pralidoxime chloride. Acta Pharmacol Sin. 2001;22(1):37–39.
- Azad A, Lall S, Mittra S. Effect of N-acetylcysteine and L-NAME on aluminium phosphide induced cardiovascular toxicity in rats. Acta Pharmacol Sin. 2001;22(4):298–304.
- Hsu C-H, Chi B-C, Liu M-Y, et al. Phosphine-induced oxidative damage in rats: role of glutathione. Toxicology. 2002;179(1-2):1–8.
- Türkez H, Toğar B. Aluminum phosphide-induced gene-tic and oxidative damages in rats: attenuation by Laurus nobilis leaf extract. Toxicol Ind Health. 2013;29(7):579–583.
- Alessio HM, Blasi ER. Physical activity as a natural antioxidant booster and its effect on a healthy life span. Res Q Exerc Sport. 1997;68(4):292–302.
- Saleki S, Ardalan FA, Javidan-Nejad A. Liver histopathology of fatal phosphine poisoning. Forensic Sci Int. 2007;166(2-3):190–193.