ABSTRACT
Introduction
The upper respiratory tract is a major route of infection for COVID-19 and other respiratory diseases. Thus, it appears logical to exploit the nose as administration site to prevent, fight, or minimize infectious spread and treat the disease. Numerous nasal products addressing these aspects have been considered and developed for COVID-19.
Areas covered
This review gives a comprehensive overview of the different approaches involving nasal delivery, i.e., nasal vaccination, barrier products, and antiviral pharmacological treatments that have led to products on the market or under clinical evaluation, highlighting the peculiarities of the nose as application and absorption site and pointing at key aspects of nasal drug delivery.
Expert opinion
From the analysis of nasal delivery strategies to prevent or fight COVID-19, it emerges that, especially for nasal immunization, formulations appear the same as originally designed for parenteral administration, leading to suboptimal results. On the other hand, mechanical barrier and antiviral products, designed to halt or treat the infection at early stage, have been proven effective but were rarely brought to the clinics. If supported by robust and targeted product development strategies, intranasal immunization and drug delivery can represent valid and sometimes superior alternatives to more conventional parenteral and oral medications.
GRAPHICAL ABSTRACT
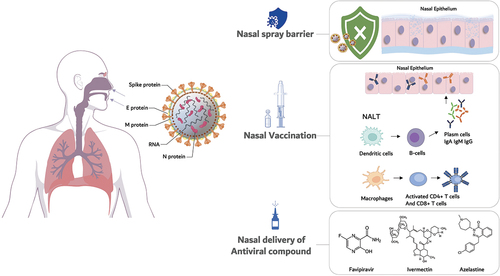
1. Introduction: the role of the nose in SARS-CoV-2 infection
In the years 2019–2023, the Severe Acute Respiratory Syndrome CoronaVirus 2 (SARS-CoV-2) triggered an unprecedented pandemic. The disease known as Corona Virus Disease 19 (COVID-19) develops initially as a respiratory infection and can progress to systemic involvement [Citation1].
SARS-CoV-2 is a single-stranded RNA, positive-sense, enveloped beta-coronavirus. The virion only contains four viral proteins: the spike (S), envelope (E), membrane (M), and nucleocapsid (N) proteins. The structural proteins S, E, and M are integrated into the virion membrane, whereas the N protein, which is coupled to the viral genomic RNA, is packed inside the virion [Citation2]. SARS-CoV-2 is mainly transmitted via respiratory particles emitted by infected subjects; large particles, i.e., droplets, are responsible for infecting nearby individuals. Aerosols have a smaller diameter (<5 μm) and infect individuals at greater distances [Citation3]. A higher viral load and replication rate have been discovered in the nasopharynx than in the oral cavity and lower airways because the penetration into the upper airways is the first step of viral infection. That is, the nose is the site of entry and primary infection of SARS-CoV-2 [Citation4]. The main entry receptors for SARS-CoV-2 following interaction with the viral Spike protein are Angiotensin-converting enzyme 2 (ACE2) and the membrane-bound serine protease TMPRSS2 () [Citation5]. ACE2 expression is the highest in the nasalciliated epithelium, and a gradient of decreasing expression from the upper to the lower airways (bronchioles and alveoli) has been detected [Citation6,Citation7].
Figure 1. Simplified depiction of the transmission and the life cycle of SARS-CoV-2 virus, along with potential immune responses elicited. SARS-CoV-2 is transmitted via respiratory droplets by infected individuals. The virus, possessing a single-stranded RNA genome wrapped in nucleocapsid (N) protein and three major surface proteins, namely membrane (M), envelope (E) and Spike, infects and replicates in the upper airways and then passes to the lungs, potentially leading to severe pneumonia. The gateway to host cell entry (magnified view) is via angiotensin-converting enzyme 2 (ACE2) interaction with cleavage of Spike in the prefusion state by proteases TMPRSS-2/furin (reproduced from [Citation5], CC-BY, ©2020 Funk, Laferrière and Ardakani).
![Figure 1. Simplified depiction of the transmission and the life cycle of SARS-CoV-2 virus, along with potential immune responses elicited. SARS-CoV-2 is transmitted via respiratory droplets by infected individuals. The virus, possessing a single-stranded RNA genome wrapped in nucleocapsid (N) protein and three major surface proteins, namely membrane (M), envelope (E) and Spike, infects and replicates in the upper airways and then passes to the lungs, potentially leading to severe pneumonia. The gateway to host cell entry (magnified view) is via angiotensin-converting enzyme 2 (ACE2) interaction with cleavage of Spike in the prefusion state by proteases TMPRSS-2/furin (reproduced from [Citation5], CC-BY, ©2020 Funk, Laferrière and Ardakani).](/cms/asset/07c15185-e9a5-42b5-baed-d4bc5adade83/iedd_a_2263363_f0001_oc.jpg)
Considering SARS-CoV-2 transmission and initial phases of the infection, it appears clear that the nose represents a unique opportunity for the prevention or the treatment of COVID-19 [Citation8]. The surface area of the nasal mucosa allows for localized drug delivery in a non-invasive way, providing also a rapid onset of the action of the product administered [Citation9]. Moreover, the mucosal tissues of the upper airways exercise a crucial protective function against both the local endogenous microbes and exogenous pathogens [Citation10]. Despite these evident advantages, the nasal route presents some well-known challenges to overcome such as the limited residence time related to the incessant mucociliary clearance and the necessity of an efficient device for depositing the drug formulation inside the nasal cavity.
The nasal cavity is lined with an epithelial mucosa and can be divided into nasal vestibule, respiratory and olfactory regions in its sagittal slice () [Citation11]. However, to interact with the epithelium, the nasal products need to penetrate the 10–15 µm-thick mucus layer, which is characterized by a network of negatively charged mucin glycoproteins, creating a structure with open spaces of dimensions varying between 20 and 1800 nm [Citation12]. Thus, some molecules could be easily withheld by mucus due to both their size and charge [Citation13]. An additional limitation to the efficacy of nasal products is represented by the mucociliary clearance, i.e., the physiological combined action of mucus-secreting cells and ciliated cells that constantly removes any material adhered to the mucus layer [Citation14].
Figure 2. The nose is part of the upper respiratory tract (left). Anatomical features include the nostrils, the nasal cavity with lower, middle and upper turbinates and the olfactory region, and the nasopharynx (right). The nasal cavity is divided by the nasal septum in two parts that are re-united in the nasopharynx. Created by Biorender.com.

The nasal cavity and epithelium features, as indicated above, as well as formulation and device, affect nasal product deposition and distribution on the target tissue and consequently its clinical efficacy [Citation15].
Nasal powders, liquid nasal sprays, nasal drops, and semi-solid formulations for nasal administration are defined in the ‘Nasal Preparations’ monograph of the European Pharmacopoeia [Citation16]. A nasal product is always a combination of formulation and device. Hence, there are several metering spray pumps for liquids, pressurized devices, and dry powder delivery devices on the market. Nasal devices can be mono-, bi-, or multidose devices, using passive or active dispersion mechanism, the latter being also propellant-aided. Doses can be pre-metered or metered at device actuation. In some cases, targeted delivery to specific regions of the nasal cavity, such as the olfactory epithelium, is claimed [Citation17–21].
The following requirements are critical in liquid formulations, may they be solutions or suspensions: solvent type and osmolarity, pH and buffering capacity, viscosity, and microbiological stability [Citation22]. In a nasal dry powder formulation, particle size, aerosolization, and dissolution are the major variables to consider. Particle sizes above 10 µm are recommended to ensure predominant nasal powder deposition and avoid inhalation into the deeper airways [Citation23]. Formulation strategies may include single-component powders of suitable particle size, but more often powder blends, agglomerated powders, or engineered multi-component particles. Despite nasal powders are few in the pharmaceutical market, the evidence of improved stability and shelf-life, greater availability, and effective administration by newly designed devices have driven extensive research toward their development [Citation24]. A complete overview of nasal drug delivery approaches and testing is beyond the scope of this review, however excellent reviews are available in literature [Citation19,Citation22,Citation25].
In the wake of the COVID-19 pandemic, all the formulation approaches mentioned above have been explored to bring forth nasal products possibly contributing to the effective fight against the spreading of the infection. The research efforts have been directed toward three main target products: nasal vaccines for immunization against SARS-CoV-2, nasal medical devices to protect from the transmission of the virus and nasal drug products delivering neutralizing or virucidal antiviral substances, as well as modulators of immune and inflammatory responses. All these strategies exploiting nasal administration will be reviewed and critically appraised in the following sections with the focus on the nose and its peculiarities as administration site. This analysis aims at evidencing their benefits and advantages and, where possible, pointing out errors or misplaced expectations in view of learning for possible future applications of nasal delivery to treat viral epidemics.
2. Nasal vaccines as a prophylactic tool for the prevention of infections
The mucosal immune system is the largest immunocompetent tissue in the human body. It consists of the lymphoid tissues and patrolling dendritic cells in all mucosal tissues of the upper and lower respiratory tract, the gastro-intestinal tract, the urogenital system, as well as of the eyes and the inner ear [Citation26]. Its activation by an antigen and a simultaneous inflammatory trigger, as caused by contact with pathogens and adjuvants, respectively, leads to an antigen-specific local immune response, which is dominant in the inductive tissue due to homing of immunocompetent cells [Citation15]. This local immune response is then distributed throughout the body following the ‘common mucosal immune system’ [Citation27]. The mucosal immune response consists of a T-cell response as well as an IgA-dominated humoral response [Citation28]. Secretory IgA is a specific antibody type which is produced within mucosal compartments and secreted onto their external surface. There, IgA dimer antibodies bind and neutralize pathogens before they enter the body, thus contributing to the prevention of infections [Citation29]. The nose is a key part of the mucosal immune system of the upper respiratory tract. The nose-associated lymphoid tissue (NALT) is concentrated in the Waldeyer’s ring consisting of the tonsils, but dendritic cells (DCs) also patrol the epithelium to take up and process foreign (antigenic) material [Citation30]. As such, the nose is a perfect target for mucosal vaccination against airborne pathogens following the same route taken by the SARS-CoV-2 virus (). However, it needs to be taken into account that mucosal surfaces, due to their constant contact with foreign material, are gatekeepers between mucosal immune activation and induction of tolerance [Citation27]. Thus, an immune-stimulating agent needs to elicit a full immune response and inevitably has to be of particulate nature [Citation31].
To date, it has become increasingly obvious that protection against virus variants (cross immunity) is strongly needed. There is evidence from nasal influenza vaccines that protection against drift variants can be more pronounced upon mucosal vaccination [Citation32,Citation33]. Especially with the evolution of the SARS-CoV-2 virus strains, a clear need for vaccines preventing the frequent breakthrough infections and achieving high levels of protection against severe disease and post-COVID symptoms has risen [Citation34]. A mucosal vaccine could be the ideal candidate to boost pre-existing systemic immunity and at the same time effectively protect against new variants [Citation35]. Nasal vaccination is non-invasive and does not require administration by trained healthcare professionals, unlike an intramuscular (i.m.) injection, which always bears the risk of injury or unsafe application. In contrast, a nasal vaccine offers ease of administration, guarantees safe handling, and will enable self-immunization by the patient alone or helped by a caretaker. As mentioned, nasal products can be either liquid or dry powder formulations [Citation22]. Having this flexibility, the formulation of a nasal vaccine can be tailored to its needs regarding stability, dosing, and administration also for immunization campaigns carried out in countries where cold-chain storage and transport are challenging. In addition to enabling vaccination in poorly accessible areas of the world and developing countries, nasal vaccination appears of special interest for children and adults that fear injections due to pain and psychological hurdles.
Nonetheless, nasal vaccines have a rocky history [Citation36], and only a few vaccines have been licensed and were available before the pandemic [Citation37]. In the early 2000s, a nasal non-living influenza vaccine product containing heat-labile Escherichia coli enterotoxin (LT) as adjuvant was reported to be associated with Bell’s palsy in Switzerland and was thus withdrawn from market [Citation38]. This was allocated to the adjuvant and its neurotoxic potential. Since then, nasal vaccines were evaluated with great caution. For human use, the only marketed products are live attenuated influenza virus vaccines, namely FluMist® Quadrivalent/Fluenz Tetra (influenza A and B, AstraZeneca AB, Sodertalje, Sweden) and Nasovac (swine flu, Serum Institute of India Pvt. Ltd., Pune, India). FluMist® was originally proven more effective in protecting children against seasonal influenza, as compared to the intramuscular inactivated vaccine not creating a sound immune response [Citation32]. More recent data did not confirm the superiority of the nasal vaccine, mostly due to poor effectiveness of either option [Citation39].
The first SARS-CoV-2 vaccines, being mRNA or viral vector products for parenteral use, were effective in the induction of a systemic immune response including neutralizing antibodies. This reduced infection severeness and viral spread. However, a sterile immunity could not be achieved, which gave rise to a discussion of alternative routes of vaccination, namely the nasal mucosal route [Citation40]. The first intranasal SARS-CoV-2 vaccine candidate in clinical trial, however, which was a viral-vector-based vaccine developed by Altimmune (Gaithersburg, MD, U.S.A.) [Citation41], has been withdrawn from further clinical investigations due to disappointing immunological results [Citation42].
Also, the approved vaccine Vaxzevria® (Astra Zeneca, Cambridge, UK; University of Oxford, Oxford, UK) has been assessed in macaques and hamsters to compare nasal and intramuscular administration routes and was shown to induce a robust mucosal response to the D614G variant with higher antibody responses after intranasal administration [Citation43]. However, further recent data of a Phase 1 trial utilizing for nasal delivery the same formulation developed for parenteral use, reported disappointing results in humans [Citation44]. The antigen-specific mucosal antibody response was typically lower than after the real SARS-CoV-2 infection and was not observed in all patients. Also, systemic responses to intranasal vaccination were typically weaker than after intramuscular vaccination with the same vaccine.
Currently, there are 8 nasal vaccines against SARS-CoV-2 in ongoing clinical development [Citation7,Citation40,Citation45] with one being currently in Phase 3 randomized, placebo-controlled trials. This is the DelNS1–2019-nCoV-RBD-OPT1 based on a replicating viral vector (Wantai Biological, Beijing, China; University of Hong Kong, and Xiamen University, Xiamen, Fujian, China) [Citation46]. In addition, six COVID-19 vaccines have been approved in local markets using nasal or airways administration [Citation47]. INCOVACC® from Bharat Biotech (Hyderabad, India) is an adenoviral vector vaccine, which is formulated to be delivered as nasal drops [Citation48,Citation49]. In a recent preprint, they report immunogenicity and safety results from a clinical Phase 3 study in 3160 Indian adults. The trial was run against Covaxin® (Bharat Biotech), an intramuscular COVID-19 vaccine. Two intranasal doses of iNCOVACC® were well tolerated with no safety concern, while eliciting a superior humoral and mucosal immune response [Citation50]. CoviLiv, a live, attenuated virus (Codagenix, Farmingdale, NY, USA/Serum Institute of India, Pune, India), has been reported with positive results via a press release, claiming a strong cellular immune and mucosal antibody response against Omicron BA.2 and announcing that the CoviLiv vaccine, being administered as nasal drops, will be part of the WHO-sponsored Solidarity Trial Vaccines [Citation51]. The Razi Cov Pars (Razi Vaccine and Serum Research Institute, Karaj, Iran), a recombinant Spike protein vaccine for delivery as nasal spray, was approved in Iran. Only results from a Phase 1 clinical trial are reported, in which a combined i.m./intranasal approach was tested in one arm. Over all, the vaccine was reported to be safe and induce a strong and durable T-cell response [Citation52].
The Sputnik adenoviral vaccine (Gamaleya Research Institute of Epidemiology and Microbiology, Moscow, Russia) can be delivered intranasally as well as the Salnavac vaccine (Generium, Moscow, Russia). Both are reported to be approved for human use. However, further information on clinical trials or protection levels is missing. Finally, Convidecia IH (CanSino Biologics, Tianjin, China) has been approved from local authorities in China in September 2022 and Morocco in November 2022 for the use as booster dose after initial i.m. vaccination. This vaccine is the non-replicating adenoviral vector vaccine the same company had been developing, which is now given by inhalation using a nebulizer [Citation53]. Seven Phase 3 clinical trials have been registered for this product, but no results have been made publicly available yet. An overview of approved vaccines and more in clinical trial [Citation44,Citation54,Citation55,Citation56] based on recent available information is shown in .
Table 1. Overview of nasal (in)/inhaled COVID-19 vaccines in clinical trials or with local marketing approval.
Besides, numerous studies had been initiated at the preclinical level, but have not proceeded yet to the clinics. Of these, the live-attenuated SARS-CoV-2 vaccine being described by researchers of FU Berlin might be one of the most promising approaches as it exploits the natural immunogenicity of a full virus [Citation57,Citation58].
Despite some late approvals of nasal vaccines of SARS-CoV-2 in local markets, the overview presented here evidences a number of common unfavorable aspects that might have hampered the impact of such immunization strategy in the global fight against the COVID-19 pandemic. First and foremost, nasal vaccination studies followed those for parenteral vaccines, adopted the same formulations, and used conventional (or even outdated as in the case of nasal drops) administration devices, leading to inevitably late results, with oftentimes unimpressive efficacy and marginal clinical relevance. Only more recent studies focusing more on the combination of antigen and adjuvants in the formulations appear to have provided a better and more rational direction to the use of the nasal administration route in the immunization strategies against the virus.
Nonetheless, the interest toward nasal vaccination to prevent the earliest stages of bacterial and viral respiratory infections is not fading. In the next 5 years, the European Vaccine Initiative will coordinate the activities of the NOSEVAC Consortium, funded in May 2023 by the European Commission to develop nasal vaccines, including a bivalent one for influenza and COVID-19 [Citation59].
3. Nasal barrier products
In the pandemic scenario, irrespective of vaccination status, the first preventive measure has been to avoid contact and infection with SARS-CoV-2. In this perspective, a number of nasal ‘barrier’ products have been developed, able to limit or prevent pathogen access to the host nasal cavity epithelia. These products, mostly marketed in the form of nasal sprays, are considered useful to prevent the infection by SARS-CoV-2 [Citation60].
These nasal sprays could also be used to prevent the spread of the infection in those situations in which there is a high risk of exposure, such as public transports, crowded places, schools, and hospitals. In addition to this, such a nasal product could also be exploited for a ‘second line’ of prevention, intended as the reduction of the risk to have an increasing viral load in the nasal cavity after an infection. This latter aspect appears crucial to reduce the severity and duration of the respiratory infection symptoms [Citation61,Citation62].
Nasal barrier products do not generally claim a direct and specific action on the virus as main feature, but are often designed to create a physical barrier that hinders the interaction between pathogens and epithelial cells of the upper airways. Actually, this passive and hence non-specific mechanism of action can be considered beneficial since the efficiency of this approach is unaffected by pathogen’s mutations [Citation63]. Although some of the products described in this section contain compounds claiming some additional mechanisms of action against viruses, the antiviral action remains questionable, as demonstrated by the fact that all these nasal sprays are invariably marketed as medical devices and not as medicinal products.
3.1. Carrageenan
Carrageenans are natural sulfated polygalactans, derived from edible red seaweeds. They are classified into five groups (λ, κ, ι, ε, μ) according to their aqueous solubility and structure. Indeed, the content of sulfate esters affects their ability to form highly viscous aqueous dispersions and gels . In particular, kappa- and iota-carrageenans () form gels in contact with an aqueous solution of calcium or potassium ions, which are present in the mucosal secretions lining the human nasal cavity [Citation64]. This accounts for the exploitation of the gelling properties of iota- and kappa-carrageenans in marketed nasal sprays.
Figure 3. Chemical structure of the compounds used in nasal barrier products: A) carrageenans, B) astodrimer sodium, C) bentonite (montmorillonite), D) hydroxypropylmethyl cellulose (HPMC).

In addition to the gelling properties in physiological conditions, carrageenans have been reported to inhibit several enveloped viruses, such as human immunodeficiency virus (HIV), herpes simplex virus (HSV), human cytomegalovirus, and human rhinoviruses [Citation65–70] by preventing the binding, and therefore the entry, of the virions into the host cell [Citation71,Citation72]. This has been attributed also to carrageenan’s high similarity to the heparan sulfate, a cell-attachment factor [Citation64].
Morokutti-Kurz et al. conducted a study in which a SARS-CoV-2 Spike Pseudotyped Lentivirus was used to infect ACE2-HEK293 cells in order to test and compare the neutralization efficacy of the different classes of carrageenans [Citation73]. It was found that iota-carrageenan’s in vitro IC50 against SARS-CoV-2 was 2.6 µg/ml and the antiviral effect was attributed to the electrostatic interaction with the viral envelope. Interestingly, it was pointed out that other polymers and polysaccharides, including hydroxypropylmethyl cellulose (HPMC), were inactive. The same group also tested the polymers on Vero B4 cells infected with SARS-CoV-2, showing that the IC50 of iota-carrageenan was 1.54 µg/ml, dramatically lower than that measured for the other classes of carrageenans [Citation73].
Another group studied the preventive activity of a commercial product containing 1.2 mg/ml iota-carrageenan and 0.4 mg/ml kappa-carrageenan against SARS-CoV-2 infection. Human Airway Epithelial Cultures (HAECs) obtained from two donors were employed to simulate the entry site of the virus from a morphological and functional point of view. The nasal product was tested at a twofold dilution and put in contact with the HAECs, which were then inoculated with the virus. The staining of the nuclei and the SARS-CoV-2 Spike protein and subsequent imaging by confocal microscopy revealed that the viral infection was completely blocked by the product () [Citation74].
Figure 4. Products A (nasal product containing iota- and kappa-carrageenan) and B (oral product containing only iota-carrageenan) inhibit SARS-CoV-2 infection of primary human airway epithelial cultures (HAECs). HAECs derived from two donors (panel a and b) were exposed to PBS or product A or B and then infected with SARS-CoV-2. After 2 h, virus and treatment were removed, and cells were washed in PBS to restore the air–liquid interface. After 1, 2, and 3 days, filters were fixed and stained for SARS-CoV-2 Spike protein (green) and cell nuclei (blue) and imaged by confocal microscopy (scale bar: 100 µm). The number of infected cells per area were counted cells within microscopic images (3–5 images per time point and condition) (reproduced from [Citation74], CC-BY 4.0, ©2021 the authors, published by the American Physiological Society).
![Figure 4. Products A (nasal product containing iota- and kappa-carrageenan) and B (oral product containing only iota-carrageenan) inhibit SARS-CoV-2 infection of primary human airway epithelial cultures (HAECs). HAECs derived from two donors (panel a and b) were exposed to PBS or product A or B and then infected with SARS-CoV-2. After 2 h, virus and treatment were removed, and cells were washed in PBS to restore the air–liquid interface. After 1, 2, and 3 days, filters were fixed and stained for SARS-CoV-2 Spike protein (green) and cell nuclei (blue) and imaged by confocal microscopy (scale bar: 100 µm). The number of infected cells per area were counted cells within microscopic images (3–5 images per time point and condition) (reproduced from [Citation74], CC-BY 4.0, ©2021 the authors, published by the American Physiological Society).](/cms/asset/b32cad06-9934-4759-abc6-bd63d338c431/iedd_a_2263363_f0004_oc.jpg)
In a pilot multicenter, randomized, double-blind, placebo-controlled study conducted in 10 hospitals in Argentina, a nasal spray containing 1.7 mg/ml iota-carrageenan was administered in four daily doses for 21 days to 394 clinically healthy physicians, nurses, and other personnel managing patients hospitalized for COVID-19. The goal was to assess the spray usefulness in the prophylaxis of COVID-19 in healthcare workers vs. placebo (0.9% sodium chloride). It resulted that the carrageenan-containing nasal formulation contributed to a statistically significant reduction (p = 0.03) in the incidence of COVID-19 cases among the individuals treated (196 individuals, 1% infected) with the active formulation with respect to those receiving the placebo (198 individuals, 5% infected) [Citation75].
3.2. Astodrimer sodium
Astodrimer sodium is a highly branched dendrimer with a dimension of 3–4 nm and a molecular weight of ~16.5 kDa [Citation76]. The dendrimer structure is characterized by a core of divalent benzhydrylamine and four generations of lysine branches; the outermost branches are functionalized with naphthalene disulfonic acid groups that confer to the overall structure hydrophilicity and an anionic surface charge () [Citation77].This net negative charge results in astodrimer having similar chemical properties to iota-carrageenan [Citation78]. The anionic surface groups can bind the viral targets such as surface glycoproteins, thus hindering the interaction between the virus and the host cell and impeding the infection [Citation79]. Indeed, astodrimer sodium was originally developed to prevent vaginal infections by HSV and HIV [Citation76]. It has also been studied to prevent ocular infection by adenovirus (HAdV) [Citation80]. During the pandemic, considering the urgent need for prevention strategies against SARS-CoV-2 infection, astodrimer properties against it were studied in view of its nasal application. In a study, a nasal spray containing 1% (w/w) astodrimer sodium was evaluated as a potential barrier against SARS-CoV-2 infection in a K18-hACE2 mouse model for 7 days and its efficacy was compared to a placebo nasal spray [Citation81]. It was found that the application of the astodrimer sodium nasal spray significantly reduced the viral genome copies in the nasal secretion at week 1 post-infection. Moreover, the study also demonstrated that SARS-CoV-2 replication and the production of several pro-inflammatory cytokines (IL-6, IL-1α, IL-1β, TNFα and TGFβ, and chemokine MCP-1) were suppressed. Therefore, the treatment may also potentially limit the severe complications related to the inflammatory process developing during the infection in the upper and, more dangerously, in the deeper airways [Citation82,Citation83].
ViralezeTM is a marketed nasal spray developed by Starpharma (Abbotsford, VIC, Australia) also containing 1% (w/w) astodrimer sodium as active ingredient. The study by Paull et al. pointed out an irreversible antiviral action and a consequent block of the SARS-CoV-2 infection when the product was applied on Vero E6, Calu-3 cells and primary human airway epithelial cells HBEpC infected with the virus [Citation84]. It emerged that 10–15 min of exposure to astodrimer sodium was sufficient to reduce the viral infectivity by more than 99.9%. Finally, astodrimer sodium was applied at variable concentrations (0, 1.1, 3.3, and 10 mg/ml) to HBEpC cells 1 h before infection and cells were cultured for 4 days, after which it came out that astodrimer sodium could reduce the infection of primary cells by up to 95–98%. This inhibition, significantly higher than that determined by iota-carrageenan (17%) used as control, supported the claim that the unique structure of astodrimer sodium seems to provide benefits in counteracting viral infection if compared to other polyanionic polysaccharides like carrageenan. Mechanistically, its virucidal activity seems due to the tight binding to virus envelope proteins, including the S protein, interfering with virus-host cell interaction [Citation84].
3.3. Bentonite
Bentonite is a colloidal hydrated aluminum silicate clay which contains clay minerals belonging to the smectite groups. It forms from the devitrification process of volcanic ash falling into water [Citation85]. When in contact with water, bentonite undergoes swelling and gelling owing to montmorillonite, its major component () [Citation86]. The multilayered negatively charged structure and high specific surface of montmorillonite , enable it to absorb coronaviruses [Citation87]. As consequence, Fais et al. evaluated a possible application of a bentonite-containing nasal spray (Bentrio®, Altamira Therapeutics Inc., Hamilton Bermuda) as barrier product against SARS-CoV-2 infection, testing it on an in vitro 3D model of the primary human upper airway epithelium (MucilAir™, Epithelix, Plan-les-Ouates, Switzerland) pooled from 14 healthy adult male and female donors and reconstituted as a 3D tissue [Citation88]. Bentrio® was studied both as preventive and post-infection treatment. To test its prophylactic effect, the product was applied to the tissue 10 min before the infection and the viral replication was monitored over 4 days. Conversely, efficacy on the existing infection was assessed on the 3D model tissue infected with the virus and treated with Bentrio® after 24 h once daily for 4 days. A physiological solution was used as control. The results show that the prophylactic treatment with the nasal product significantly reduced the viral titer over 4 days. However, the reduction was higher (99%) when cells were infected with wild-type SARS-CoV-2 and lower (83%) when the Delta variant was used. When Bentrio® was applied 24 h post infection, a reduction of the viral titer by 12-folds and 3-folds on day 4 was registered, respectively for wild-type SARS-CoV-2 and Delta variant. Therefore, Fais et al. concluded that Bentrio® can act as an ‘intranasal mask,’ preventing the direct contact between the virus and nasal mucosa, trapping the virus into the bentonite-stabilized gel formed after application, thus favoring its elimination by mucociliary clearance. Finally, they observed that the model tissue used could be considered as a worst-case scenario, because when applied in vivo, the antiviral activity of the nasal product is integrated by the immune system activity and mucociliary clearance [Citation89]. Indeed, when eventually removed by the mucociliary clearance, the gel passes to the nasopharynx and the throat, still keeping the virus entrapped [Citation88], thus hindering its transmission to other people by talking or coughing. It is worth reminding that the virus spreads via the airborne droplets mainly produced in the oral cavity [Citation90,Citation91,Citation92].
3.4. Hydroxypropylmethyl cellulose
Hydroxypropylmethyl cellulose (HPMC, ) is a chemical derivative of cellulose whose capability to create a protective barrier when applied to the nose was discovered by chance. The peculiarity of HPMC-containing nasal products is that they are administered as powder and form a viscoelastic gel only after the deposition into the nasal cavity and hydration by the mucosal fluids [Citation24,Citation93].
A number of nasal spray powders containing HPMC are produced by Nasaleze International Limited (Isle of Man, UK) and are licensed as medical devices in Europe and in the USA [Citation94]. These devices provide the intranasal administration of the product in the form of a powder composed of 93%-98.5% by weight of HPMC. Owing to such high HPMC concentration, in the nasal cavity these powders take up the available fluids and turn into a gel. Thus, the extemporaneously generated gel acts as a physical and non-specific barrier against airborne germs and viruses, holding back the latter and therefore preventing their movement to the deeper airways [Citation94].
Bentley et al. tested three nasal powder products by Nasaleze on Vero A/T cells infected with SARS-CoV-2, demonstrating that the release of the virus from infected cells was inhibited in a dose-dependent manner. More precisely, the optimum dose of the powders was found to be 6.4 mg/3.5 cm2. Above this threshold concentration, the HMPC-containing powders form a gel matrix over the cell layer inhibiting the SARS-CoV-2 release from infected cells for a 72 h period independently of the concentration of HPMC present (93–98.5%). At the same time, not only the gel matrix prevented the release of the virus, but also protected the cells from infection by three different virus variants, namely England-2, Alfa, and Beta [Citation63]. Thus, HPMC-based nasal powders, despite not showing direct antiviral or virucidal activities, protect from viral infection by mechanically trapping the pathogens in an impervious viscous gel layer [Citation63].
Another commercial nasal powder containing HPMC is Taffix®, a class I medical device manufactured by Nasus Pharma (Tel Aviv, Israel) and widely marketed in the EU [Citation95], containing 89.9% (w/w) HPMC. Owing to a formulation including also citric acid and sodium citrate, Taffix® can maintain the nostril microenvironment at a stable pH of 3.5 for up to 5 h. This is functional to create a hostile microenvironment for virus survival and prevent the interaction between respiratory viruses like SARS-CoV-2 and airway epithelial cells [Citation96,Citation97].
The in vitro study performed on slices of pig nasal cavity by Mann et al., demonstrated that the physical viscous barrier created by Taffix® in contact with the nasal mucosa led to 99% reduction of the titer of both the recoverable live virus and viral RNA. The study confirmed that the gel formed within only 1 minute after the application of the spray on the tissue and preserved its characteristic viscosity for 6 h [Citation97].
The effectiveness of this nasal product was also tested in another real-life survey study involving 243 subjects from an Orthodox Jewish community engaged in a two-day period of prayers, which was considered a high-risk event in terms of viral spreading. 83 individuals used Taffix® throughout the prayer period and the following 2 weeks, while the remaining 160 volunteers did not receive the treatment. At the end of the 2-week observation period, only two of the subjects in the Taffix® group, developed the infection and it came out they had not used the product regularly. Conversely, 10 out of 160 participants in the untreated group got infected by the end of the study. Therefore, the study confirmed that HPMC powder nasal insufflators could provide an effective protection against the transmission of SARS-CoV-2 infection, pointing out a significant fourfold reduction in the risk of infection in a population treated with Taffix® regularly for 2 weeks compared to no treatment [Citation95].
It appears clear that after the beginning of the pandemic, nasal barrier products have been made rapidly available and, in a way, represented the most successful nasal product against COVID-19. Some specific features of these products have to be taken into account to critically evaluate their role in the prevention of epidemics. First and foremost, nasal barrier products are registered as medical devices, characterized by less restrictive requirements for marketing authorization. In addition, they are relatively inexpensive, easy-to-use, protection devices with very few side effects and contraindications. For this reason, they have been widely exploited during the early stage of the pandemic as a method to reduce the spread of the infection, often as adjuvants in association with other personal protective equipment. Notwithstanding, these products have no specific mechanism of action and their effectiveness, based mainly on physical action, is at least doubtful in cases of an already established infection, making them good adjuvants but not the main strategy in the fight of the spreading of a pandemic pathogen.
4. Nasal antiviral products
The quest for a cure for COVID-19 urged major drug repurposing efforts [Citation98]. Drug substances active against viruses other than SARS-CoV-2 were randomly and almost desperately tried on hospitalized, severely ill patients by systemic administration routes [Citation99]. This was not necessarily supported by the established evidence of efficacy in COVID-19, but rather motivated by experiences in SARS and MERS epidemics [Citation100]. Overall, the efficacy of these approaches was poor, as demonstrated by WHO Solidarity and Recovery trials [Citation101,Citation102]. Beside their non-specificity for SARS-CoV-2, these compounds possibly failed due to: i) poor administration timing with respect to infection stage, ii) severe side-effects and iii) a systemic dosing unable to lead effective concentrations in infected cells. Administration to the nasal cavity and upper airways appeared the right way to tackle the virus directly and timely [Citation103]. The local administration in the upper airways even of low doses of antiviral agents provides high drug concentrations at the site of ongoing viral replication, limiting systemic exposure and undesired adverse effects.
4.1. Repurposed drugs for nasal administration (small molecules)
Several repurposed drug substances with different therapeutic indications were considered for nasal delivery, highlighting how broad the initial search for useful compounds has been. Most of the available information reports of formulation studies and activity data collected in vitro, which may leave the reader disenchanted. He/she should be mindful that developing a new medicinal product, even with a known drug molecule, is a slow process, difficult to accelerate even in urgent circumstances. Then, while the race for a cure is going on, the pandemic driver declines as well as the ‘incentive’ to pursue the research.
The search of antiviral agents for systemic administration led to the relatively fast marketing authorization for remdesivir (intravenous injection) in mid 2020, followed by Paxlovid® (nirmatrelvir/ritonavir) and molnupiravir for oral use in 2022. None of these molecules was newly designed to treat SARS-CoV-2. Aiming to an antiviral effect targeted to the nasal mucosa, the only antiviral agent investigated has been favipiravir (FVP). It is an antiviral drug approved in Japan as a treatment for novel or re-emerging influenza RNA viruses [Citation104]. After metabolization into ribosyl triphosphate, FVP selectively inhibits viral RNA-dependent RNA polymerase, sharing this mechanism of action with remdesivir. Favipiravir has poor aqueous solubility and good permeability [Citation105]. Envisaging a liquid dosage form its nasal delivery, the low solubility hinders the formulation of a concentrated solution in water. Alcantara et al. were able to enhance FVP activity in vitro by microencapsulating it into chitosan/alginate nanoparticles. These polysaccharides were chosen for mucoadhesion, to prolong formulation residence at absorption site [Citation106]. Compared to free drug, owing to polymer swelling, in vitro FVP release from the nanoparticles was slowed down both at pH 7.4 and 5.5, being faster at 5.5, being 5.5–6.5 and 5.0–6.7 the pH ranges in the anterior and posterior nose, respectively [Citation107]. Protonation of the chitosan amino groups eased swelling and erosion. Furthermore, chitosan strengthened the interaction with mucus and promoted greater FVP permeation across the nasal mucosa. Finally, the antiviral activity of FVP nanoparticles was evaluated in Vero cells infected with porcine epidemic diarrhea virus, which is a good model but remains a surrogate of SARS-CoV-2. They reduced viral replication with an EC50 of 7 μg/ml, whereas free FVP did not inhibit it up to 46 μg/ml [Citation108].
The concept of nanoencapsulating favipiravir for nasal delivery is shared by Gattani and Dawre, who developed FVP-loaded poly-lactic-co-glycolic acid (PLGA) nanoparticles [Citation109]. The FVP-loaded nanoparticles were included in a thermosensitive gel to administer the FVP nanoparticles intranasally. Not unexpectedly, encapsulation within PLGA particles slowed down FVP release compared to the drug solution. Nanoparticle entrapment inside the gel sustained FVP release up to 32 h, due to gel viscosity. However, the ex vivo permeation across goat nasal mucosa evidenced the greatest permeation from the nanoparticle-loaded gel, which was the slowest formulation at releasing FVP. The authors’ explanation of a mucoadhesive action by HPMC prolonging contact time appears unconvincing as contact time in the in vitro experiment was the same for all formulations [Citation109]. Hence, despite the formulation effort, the therapeutic value of this strategy warrants further confirmation.
The above-mentioned studies are the only ones of an antiviral agent formulated for nasal delivery in COVID-19, suggesting that the strategy of nasal administration of antivirals was addressed quite late in the race for COVID-19 treatments. Before the oral products were approved, remdesivir was the only antiviral available, mostly used in hospitals at a disease stage likely missing the viral target. Its outcomes are still considered uncertain [Citation110], whereas the oral treatments for early use in the outpatient, seem effective even in the elderly [Citation111]. In addition, considering the formulations envisaged, given a volume of liquid administrable of around 150 µl per human nostril, the drug dose deliverable might be too small for the intended effect [Citation112].
Ivermectin (IVM) is an oral antiparasitic drug approved for neglected tropical diseases, like onchocerciasis, helminthiases, and scabies. In vitro studies showed that IVM may work in COVID-19 because it inhibited SARS-CoV-2 replication in infected Vero E6 cells. Activity was attributed to inhibition by IVM of importin-α/β1-mediated nuclear entry of viral proteins, blocking viral RNA replication [Citation113]. In particular, Caly and collaborators demonstrated that 5 µM ivermectin inactivated more than 90% viral RNA with an IC50 around 2 µM [Citation114]. The problem is that, to achieve the active IVM concentration in plasma, an oral dose 100 times higher than the maximum allowed in humans should be administered. Consequently, the FDA and other regulatory agencies never approved IVM for systemic use in COVID-19 [Citation115]. Local drug administration into the nose/upper airways could overcome this problem, as doses could be lowered. The concept is supported by studies indicating that IVM can accumulate in lung tissue at high concentration following inhalation [Citation116].
A liquid ivermectin nasal spray has been proposed with composition, propellant, and device typical of pressurized inhalers [Citation117]. However, from a technological perspective, the study is poorly informative. It is not declared whether the formulation was a solution or a suspension of IVM (1% w/v) in the propellant. Furthermore, the authors stated ‘delivery performance of the product was adequate,’ without mentioning actual data. The study’s main focus was the local and systemic safety and pharmacokinetics of ivermectin administered to healthy piglets by the nasal spray (1 mg per nostril).
A single dose of IVM nasal spray gave after 2 h the highest drug concentrations in the nasal tissue, i.e., the primary site of virus replication, when compared to lung and plasma levels. IVM was also detected in the lung, at lower concentrations and delayed time-to-peak. Meanwhile, at all time points plasma levels were 10 times lower than nasopharyngeal concentrations. Treatment safety was good both at systemic and local level, while nose-to-brain transport was excluded based on the absence of signs of neurotoxicity. Interestingly, repeated nasal dosing at a 12 h interval, resulted in considerably increased IVM concentrations in nasopharyngeal tissue and lungs, but not in plasma, reducing the risk of side effects. In addition, the study demonstrated that local drug application reached higher concentration in target tissues than the same dose given orally. Clearly, a proof of antiviral effectiveness in infected animals is needed to confirm the therapeutic value of this pressurized IVM nasal spray. The highest concentration measured in nasopharyngeal tissue (about 43 ng/g) was approximately 40-folds lower than the reported in vitro IC50 [Citation117].
The intranasal use of ivermectin in COVID-19 was evaluated in humans by an Egyptian team, who tested an IVM mucoadhesive nanosuspension intranasal spray in patients with mild COVID-19. The IVM nanosuspension (70 µg/ml), obtained by nanoprecipitation, was incorporated in a mucoadhesive vehicle containing HPMC, poloxamer, and alginate. According to the protocol, the treatment group received nasal IVM twice a day combined with the standard care protocol for mild COVID-19 treatment, whereas the control group received the latter only. Intranasal ivermectin added to standard care, caused rapid viral clearance and recovery from anosmia, cough, dyspnea [Citation118]. In a subsequent study on patients with persistent post-COVID-19 anosmia, the nasal IVM-treated group recovered from anosmia in 13 days compared to the 50 days of the placebo group [Citation119]. According to ClinicalTrial.gov database, there are no ongoing clinical trials on intranasal ivermectin.
Both azelastine hydrochloride and chlorpheniramine maleate are antagonists of histamine-1 receptor, formulated in over-the-counter nasal sprays for allergic rhinitis. They were tested for a potential action against SARS-CoV-2 infection. Azelastine inhibits SARS-CoV-2 by binding the ACE2 receptor, which the virus uses to enter cells [Citation120]. The in vitro EC50 of about 6.5 µM (Vero cells infected with SARS-CoV-2) was hundreds of folds lower than the concentration of the marketed spray [Citation121]. Then, a placebo-controlled trial assessed the efficacy of azelastine nasal spray (0.02% or 0.1%) to reduce viral load of COVID-19 patients treated for 11 days. The treatment was not very effective because the viral load decreased in 2 weeks independently of the treatment. A significant effect vs. placebo was observed only at day 4 of treatment with 0.1% azelastine in patients with high viral burden at enrollment [Citation122]. A new pilot study (clinicaltrials.gov Identifier NCT06008860) has been launched in July 2023 and is currently recruiting at the University of Chicago (Chicago, IL, USA) to reconfirm the usefulness of nasal azelastine (Astepro® 0.15% nasal spray) in vaccinated individuals. The effect of the treatment on symptoms, infectivity, quality of life and occupation will be hopefully clarified.
The available data about chlorpheniramine maleate (CPM) in COVID-19 come from one paper by Westover et al., reporting CPM virucidal efficacy in vitro in Vero 76 cells infected with SARS-CoV-2 [Citation123]. The authors used a 0.4% CPM nasal spray containing water, glycerin, xylitol, and sodium bicarbonate formulated by Ferrer Medical Innovations (Hallendale Beach, FL, U.SA) and Xlear Inc. (American Fork, UT, U.SA). No mechanistic information is reported, but CPM-dependent inhibition of influenza virus was attributed to interference with the viral entry into cells [Citation124]. Safety and efficacy of this CPM nasal spray were demonstrated in a 7-day pilot study on mild COVID-19 patients, instructed on how to use the device to optimize drug delivery in the nasopharynx [Citation125]. Meanwhile, Xlear Inc repurposed for adjunct treatment of COVID-19 a nasal spray product that is a medical device containing xylitol and grapefruit seed extract [Citation126,Citation127].
4.2. Biologicals
Certain chronic infections are treated with intravenous immunoglobulins (IVIG) and this strategy has been extensively explored in the treatment of COVID-19 since immunoglobulins or hyperimmune plasma were used to exploit the virus neutralizing properties of antibodies [Citation128]. Ku et al. engineered an immunoglobulin M neutralizing antibody, IgM-14, able to neutralize SARS-CoV-2 replication in vitro and in vivo in view of nasal administration. One single IgM-14 intranasal dose, given to mice 6 h before or 6 h after infection, elicited a prophylactic or therapeutic effect against viral infection, respectively. The prophylactic dose was as low as 0.044 mg/kg, compared to the 0.4 mg/kg therapeutic dose. Additionally, ex vivo organ imaging proved that the residence time of IgM-14 in the nasal cavity was prolonged (>100 h) and that IgM-14 was able to reach the lungs [Citation129].
An interesting dual targeting technological approach was applied with WKS13, a humanized monoclonal antibody (mAb) with broad spectrum activity against SARS-CoV-2 variants. The mAb was spray-dried using different nozzles to obtain particles of a size suitable for nasal and pulmonary deposition, respectively. In particular, an ultrasonic nozzle produced large ‘nasal particles,’ whereas a two-fluid nozzle allowed for the production of small particles suitable to target the lungs. A mixture of the two powders would tackle nose and lung at the same time, broadening the mAb efficacy. Dual targeting of the fine pulmonary powder when administered intranasally is achieved because of the nose’s particle-size independent filter mechanism, taking out a fraction of the pulmonary powder irrespective of size. This study presented in vivo data in hamsters infected with SARS-CoV-2 and treated with the engineered powders. However, the animals did not receive the dual targeting powder, but the individual ‘nasal’ and ‘lung’ powders, each given via the corresponding route. The neutralizing effect was not superior to the intravenous mAb, but intranasal administration was deemed valuable in terms of acceptability, non-invasiveness, and self-use by outpatients [Citation130].
The progression of SARS-CoV-2 infection to severe disease sees a heavy immune reaction with infiltration of monocytes to the lung, hyperinflammation and a ‘cytokine storm’ [Citation131]. T cell-mediated immunity plays an essential role to manage the infection, but when T cell responses are dysregulated, immunopathology can occur and worsen the disease. In particular, reduced frequencies of regulatory T cells (Treg) were observed in severe COVID-19 cases. Thus, induction of Treg cells at mucosal level, elicited by nasal administration of an immunomodulator, can be a therapeutic strategy for immune modulation of inflammatory diseases [Citation132,Citation133]. Foralumab, an anti-CD3 monoclonal antibody, was administered intranasally to mild and moderate COVID-19 patients and reduced lung inflammation [Citation134]. The effect of 100 µg of nasal foralumab daily for 10 days was monitored based on blood inflammatory biomarkers. Eventually, induction of IL-10-producing Treg cells that suppress inflammation was hypothesized to explain the effect. Furthermore, nasal delivery avoided the occurrence of potential systemic adverse events associated with intravenous anti-CD3 therapy.
Interferons (IFNs) act against a broad spectrum of viruses as inflammation modulators [Citation135]. In early 2020, the effects of the treatment with nebulized IFN-α2b were studied in a cohort of confirmed adult COVID-19 cases in Wuhan, China. This uncontrolled exploratory study showed that viral clearance from the respiratory tract was accelerated, while the levels of inflammatory biomarkers like IL-6 and C-reactive protein were reduced [Citation136,Citation137]. Along the same lines, an IFN-α2b nasal spray was tested in children infected by SARS-CoV-2 Omicron strain [Citation138]. Dose and frequency of the nasal IFN-α2b therapy (8,300 IU/spray) depended on child age. The treatment began within 5 days of symptom onset and lasted 3 days. Nasal interferon, combined with standard of care, shortened SARS-CoV-2 viral shedding time, i.e., the period from symptom onset to the first PCR cycle threshold > 35. This was a great achievement to cure young children by a non-invasive, acceptable route in a timely manner. In fact, being not eligible for vaccination and novel antiviral therapies, children shed the virus longer and more likely transmit it than adults, particularly the 12–24 months age group [Citation138]. Technologically, very few of the above-mentioned studies paid attention to the formulation of the biologics, which was often not specific for nasal delivery but used pre-existing formulations for injection.
4.3. Other substances
Inorganic compounds like nitric oxide and hypochlorous acid act by nonspecific mechanism on various pathogens. Nitric oxide (NO) is a signaling molecule involved in physiological and pathological processes in the human body, including innate immunity [Citation139]. In occasion of the 2002 SARS epidemic the antiviral action of NO against SARS-CoV infection was evidenced [Citation140] The exact mechanism of action was discovered later: NO reduces the palmitoylation of the viral Spike (S) protein, inhibiting membrane fusion mediated by S protein-ACE2 receptor interaction. It was also understood that 3-h exposure to NO was enough for virus inhibition [Citation141].
Several clinical trials have been conducted on intranasal NO and derivatives for the treatment of COVID-19 [Citation142,Citation143,Citation144]. SaNOtize biotech company (Vancouver, Canada) registered a nitric oxide nasal spray as medical device (NORSTM patented technology). SaNOtize claims a double action for the spray proposed as treatment or prevention tool, i.e., physical/chemical barrier and NO effect. In a Phase 3 trial vs. placebo, COVID-19 patients treated with the spray had viral load reduced by more than 94% within 24 h from treatment [Citation143]. Another Phase 2 study is ongoing to demonstrate whether NO nasal spray prevents SARS-CoV-2 infection (clinicaltrials.gov Identifier: NCT05109611).
Hypochlorous acid is a powerful oxidizing agent with antiviral, antibacterial, and antifungal properties. The virucidal effect is due to the reaction with amino acids, forming chloramines, and nitrogen-centered radical formation, damaging the viral nucleic acid [Citation145]. Sentinox by APR (Applied Pharma Research s.a., Balerna, Switzerland) is a class III medical device composed of an acidic oxidizing solution to spray containing 0.005% HClO. The recommended volume per nostril is 500 µl, deriving from five bottle squeezes, which may resemble a nasal wash procedure. In vitro, the solution showed >99.8% virucidal activity in less than 1 min on SARS-CoV-2. Tested on nasal and oral mucosae ex vivo, the solution caused no irritation [Citation146]. Finally, the efficacy of Sentinox (STX) in mild COVID-19 patients was assessed in a trial with three treatment groups: 1) spray three times/day, 2) spray five times/day, 3) untreated control. There was no greater reduction of viral load by STX compared to control. Anyway, STX spray was safe and well tolerated [Citation147].
Differently from nasal vaccines and barrier products, no antiviral drug product for nasal use has yet reached the market. During the first months of pandemic worldwide, governments, agencies, academia, and research institutions devoted substantial funding and frantic efforts to the race for a cure. This nourished the idea of an intranasal therapy with repurposed drugs, not only antivirals, as an easy and safe tool to stop the infection at early stage in the home setting (supervised by the general medicine practitioner). However, this expectation did not come true. We acknowledge three possible reasons for the lack of ultimate results and zero products at advanced clinical development or marketed. First, effective vaccines were made rapidly available and COVID-19 progressively became less severe, somehow mitigating the urgency for drug-based therapies. Secondly, the lengthy medicine development process did not really fit the time frame of the epidemic evolution. Before a product showed substantial evidence of therapeutic value, the pandemic outbreak subsided and researchers possibly lost motivation. Thirdly, another disincentive for the industry could have been the short duration of the anti-SARS-CoV-2 treatment (1–2 weeks), narrowing the window of economical investment return.
5. Expert opinion
Acknowledging the route of infection and pathogen entry in SARS-CoV-2 and other airborne diseases, it appears logical to include the respiratory system and especially the nose in the forefront of prevention and early control strategies. Among these, vaccination plays a major and very effective role and for compelling immunological reasons it should be delivered via the mucosal surfaces being involved in the infection. However, current results with nasal vaccines emphasize the need for different and more effective products. During the COVID-19 pandemic, viral vector-based products, originally developed for parenteral immunization, have been reformulated as nasal vaccines, which enabled rapid translation into clinical trials. However, it should be considered that immunologically, viral vectors are difficult to use in prime-boost or regular revaccination strategies, as envisaged for COVID-19 prevention, due to their potential autoimmunogenicity (vector directed immunity) [Citation40]. It is unclear, however, whether other vaccine types will after all be able to provoke sustainable mucosal immune responses. It is known that there is a delicate balance between protective immunity and mucosal tolerance. Vaccines need to induce sufficient inflammatory responses (either by an attenuated pathogen itself or the use of a suitable adjuvant), which trigger innate immunity to support the induction of effector cell responses [Citation36]. In that respect, joint research forces are needed to find safe and efficient mucosal adjuvants boosting safe antigens such as mRNA-based or subunit vaccines. There is some proof that mRNA-based vaccines can be effective in a prime-boost scheme [Citation148]. Based on knowledge from nasal influenza vaccines, utilizing live-attenuated viral vaccines could be a promising alternative. Currently studied vaccine formulations are further not designed for nasal delivery as the administered volume is too high for nasal retention and they do not contain mucoadhesive agents, and thus will be cleared from the nose rapidly.
The prophylactic effect of barrier products relies on an increase in viscosity and the formation of a viscous layer additional to physiological mucus, basically hindering the binding of the pathogen to the host cell surface. Considering that the nose represents the main site for SARS-CoV-2 transfection [Citation64], it is mandatory to adopt preventive measures that protect the nasal epithelium against the widest possible range of viral variants. From this perspective a non-specific and mechanical action appears the most suitable. General physical blockage has been proven an easy-to-access and quick response; more specific blockers (e.g. local inhibitors of TMPRSS2 [Citation149,Citation150]) could follow.
Finally, direct antiviral activity at the site of first contact is a reasonable, but not yet fully explored option, possibly for the reasons previously discussed. Despite some positive results, full clinical assessment of such formulations is still missing, and no new small molecules have been developed in the course of COVID-19 so far.
The learning from nasal products in COVID-19 in terms of immunological impact, local drug effects, user feedback and, importantly, the selection of formulation and delivery system will be very valuable and can be extended to other airborne diseases in the future.
Article highlights
The nose is the entry port and site of the early infection of viruses, including SARS-CoV-2.
Intranasally delivered medicinal products have been proposed to prevent, block or minimize the spread of COVID-19.
Nasal vaccines can induce a mix of local mucosal (IgA) and systemic immunity (IgG) ideal for fighting airborne pathogens.
Nasal barrier products use the deposition of materials on the nasal mucosa that non-specifically prevent virus entry in the airway epithelial cells.
Nasal antiviral products utilize a more traditional local action against the virus providing a defense at the early stages of the infection.
Overall, these products have achieved relatively modest success in the fight against SARS-CoV-2 pandemic.
Future developments should focus on products designed more specifically, both in terms of formulation and device, to exploit the unique opportunities provided by the nasal route.
Declaration of interest
R. Scherließ is listed in Nasus’ Advisory Board. G. Colombo, E. Quarta and S. Banella are inventors of an Italian patent of nasal preparations for airborne infection. F. Sonvico is listed in Polyrizon’s Advisory Board. The authors have no other relevant affiliations or financial involvement with any organization or entity with a financial interest in or financial conflict with the subject matter or materials discussed in the manuscript apart from those disclosed.
Reviewer disclosures
Peer reviewers on this manuscript have no relevant financial or other relationships to disclose.
Additional information
Funding
References
- Zhu N, Zhang D, Wang W, et al. A novel coronavirus from patients with pneumonia in China, 2019. New Engl J Med. 2020;382(8):727–733. doi: 10.1056/NEJMoa2001017
- Kirtipal N, Bharadwaj S, Kang SG. From SARS to SARS-CoV-2, insights on structure, pathogenicity and immunity aspects of pandemic human coronaviruses. Infect Genetics Evol. 2020;85:104502. doi: 10.1016/j.meegid.2020.104502
- Lee BC, Lee BU. Minimum sizes of respiratory particles carrying SARS-CoV-2 and the possibility of aerosol generation. Int J Environ Res Public Health. 2020;17(19):6960. Int J Environ Res Pu. 2021;18:11738. doi: 10.3390/ijerph17196960
- Sungnak W, Huang N, Bécavin C, et al. SARS-CoV-2 entry factors are highly expressed in nasal epithelial cells together with innate immune genes. Nat Med. 2020;26(5):681–687. doi: 10.1038/s41591-020-0868-6
- Funk CD, Laferrière C, Ardakani A. A snapshot of the global race for vaccines targeting SARS-CoV-2 and the COVID-19 pandemic. Front Pharmacol. 2020;11:937. doi: 10.3389/fphar.2020.00937
- Hou YJ, Okuda K, Edwards CE, et al. SARS-CoV-2 reverse genetics reveals a variable infection gradient in the respiratory tract. Cell. 2020;182(2):429–446.e14. doi: 10.1016/j.cell.2020.05.042
- Chavda VP, Vora LK, Pandya AK, et al. Intranasal vaccines for SARS-CoV-2: from challenges to potential in COVID-19 management. Drug Discov Today. 2021;26(11):2619–2636. doi: 10.1016/j.drudis.2021.07.021
- Kozlov M. Could a nose spray a day keep COVID away? Nature. 2022; doi: 10.1038/d41586-022-03341-z
- Lam JKW, Cheung CCK, Chow MYT, et al. Transmucosal drug administration as an alternative route in palliative and end-of-life care during the COVID-19 pandemic. Adv Drug Deliv Rev. 2020;160:234–243. doi: 10.1016/j.addr.2020.10.018
- Himi T, Takano K, Ogasawara N, et al. Mucosal immune barrier and antigen-Presenting system in human nasal epithelial cells. Adv Oto-Rhino-Laryng. 2011;72:28–30.
- Thwala LN, Préat V, Csaba NS. Emerging delivery platforms for mucosal administration of biopharmaceuticals: a critical update on nasal, pulmonary and oral routes. Expert Opin Drug Deliv. 2017;14(1):23–36. doi: 10.1080/17425247.2016.1206074
- Leal J, Smyth HDC, Ghosh D. Physicochemical properties of mucus and their impact on transmucosal drug delivery. Int J Pharm. 2017;532(1):555–572. doi: 10.1016/j.ijpharm.2017.09.018
- Newby JM, Seim I, Lysy M, et al. Technological strategies to estimate and control diffusive passage times through the mucus barrier in mucosal drug delivery. Adv Drug Deliv Rev. 2018;124:64–81. doi: 10.1016/j.addr.2017.12.002
- Marttin E, Schipper NGM, Verhoef JC, et al. Nasal mucociliary clearance as a factor in nasal drug delivery. Adv Drug Deliv Rev. 1998;29(1–2):13–38. doi: 10.1016/S0169-409X(97)00059-8
- Djupesland PG. Nasal drug delivery devices: characteristics and performance in a clinical perspective—a review. Drug Deliv Transl Re. 2013;3(1):42–62. doi: 10.1007/s13346-012-0108-9
- Nasal Preparations. European pharmacopoeia. 11.0. Strasburg: EDQM; 2022.
- Bommer R. Drug delivery: nasal route. In: Swarbrick J, editor. Encyclopedia of pharmaceutical technology. (NY): Informa Healthcare; 2006. p. 1201–1208.
- Hellfritzsch M, Scherließ R. Mucosal vaccination via the respiratory tract. Pharmaceutics. 2019;11(8):375. doi: 10.3390/pharmaceutics11080375
- Salade L, Wauthoz N, Goole J, et al. How to characterize a nasal product. The state of the art of in vitro and ex vivo specific methods. Int J Pharm. 2019;561:47–65. doi: 10.1016/j.ijpharm.2019.02.026
- Moffa A, Costantino A, Rinaldi V, et al. Nasal delivery devices: a comparative study on cadaver model. Biomed Res Int. 2019;2019:4602651. doi: 10.1155/2019/4602651
- Tai J, Han M, Lee D, et al. Different methods and formulations of drugs and vaccines for nasal administration. Pharmaceutics. 2022;14(5):1073. doi: 10.3390/pharmaceutics14051073
- Scherließ R. Nasal formulations for drug administration and characterization of nasal preparations in drug delivery. Ther Deliv. 2020;11(3):183–191. doi: 10.4155/tde-2019-0086
- CHMP. Guideline on the pharmaceutical quality of inhalation and nasal products. EMEA; 2006. Available from: https://www.ema.europa.eu/en/documents/scientific-guideline/guideline-pharmaceutical-quality-inhalation-nasal-products_en.pdf
- Tiozzo Fasiolo LT, Manniello MD, Tratta E, et al. Opportunity and challenges of nasal powders: drug formulation and delivery. Eur J Pharm Sci. 2018;113:2–17. doi: 10.1016/j.ejps.2017.09.027
- Shrewsbury SB. The upper nasal space: option for systemic drug delivery, mucosal vaccines and “nose-to-brain”. Pharmaceutics. 2023;15(6):1720. doi: 10.3390/pharmaceutics15061720
- Holmgren J, Czerkinsky C. Mucosal immunity and vaccines. Nat Med. 2005;11(S4):S45–S53. doi: 10.1038/nm1213
- Neutra MR, Kozlowski PA. Mucosal vaccines: the promise and the challenge. Nat Rev Immunol. 2006;6(2):148–158. doi: 10.1038/nri1777
- Janeway CA, Travers P, Walport M, et al. The mucosal immune system. Immunobiology: the immune system in Health and disease. 5th ed. (NY): Garland Science; 2001. Available from: https://www.ncbi.nlm.nih.gov/books/NBK27169/.
- Ainai A, Riet E, Ito R, et al. Human immune responses elicited by an intranasal inactivated H5 influenza vaccine. Microbiol Immunol. 2020;64(4):313–325. doi: 10.1111/1348-0421.12775
- Davis SS. Nasal vaccines. Adv Drug Deliv Rev. 2001;51(1–3):21–42. doi: 10.1016/S0169-409X(01)00162-4
- Csaba N, Garcia-Fuentes M, Alonso MJ. Nanoparticles for nasal vaccination. Adv Drug Deliv Rev. 2009;61(2):140–157. doi: 10.1016/j.addr.2008.09.005
- Belshe RB, Edwards KM, Vesikari T, et al. Live attenuated versus inactivated influenza vaccine in infants and young children. New Engl J Med. 2007;356(7):685–696. doi: 10.1056/NEJMoa065368
- Carter NJ, Curran MP. Live attenuated influenza vaccine (FluMist®; Fluenz™). Drugs. 2011;71(12):1591–1622. doi: 10.2165/11206860-000000000-00000
- Topol EJ, Iwasaki A. Operation nasal vaccine—lightning speed to counter COVID-19. Sci Immunol. 2022;7(74). doi: 10.1126/sciimmunol.add9947
- Tang J, Zeng C, Cox TM, et al. Respiratory mucosal immunity against SARS-CoV-2 following mRNA vaccination. Sci Immunol. 2022;7(76):eadd4853. doi: 10.1126/sciimmunol.add4853
- Lycke N. Recent progress in mucosal vaccine development: potential and limitations. Nat Rev Immunol. 2012;12(8):592–605. doi: 10.1038/nri3251
- Xu H, Cai L, Hufnagel S, et al. Intranasal vaccine: factors to consider in research and development. Int J Pharm. 2021;609:121180. doi: 10.1016/j.ijpharm.2021.121180
- Mutsch M, Zhou W, Rhodes P, et al. Use of the inactivated intranasal influenza vaccine and the risk of Bell’s palsy in Switzerland. New Engl J Med. 2004;350(9):896–903. doi: 10.1056/NEJMoa030595
- Shim E, Brown ST, DePasse J, et al. Cost effectiveness of influenza vaccine for U.S. Children live attenuated and inactivated influenza vaccine. Am J Prev Med. 2016;51(3):309–317. doi: 10.1016/j.amepre.2016.02.027
- Alu A, Chen L, Lei H, et al. Intranasal COVID-19 vaccines: from bench to bed. EBioMedicine. 2022;76:103841. doi: 10.1016/j.ebiom.2022.103841
- King RG, Silva-Sanchez A, Peel JN, et al. Single-dose intranasal administration of AdCOVID elicits systemic and mucosal immunity against SARS-CoV-2 and fully protects mice from lethal challenge. Vaccines. 2021;9(8):881. doi: 10.3390/vaccines9080881
- Altimmune. Altimmune announces update on AdCOVID(tm) Phase 1 clinical trial. 2021; Available from: https://ir.altimmune.com/news-releases/news-release-details/altimmune-announces-update-adcovidtm-phase-1-clinical-trial
- van Doremalen N, Purushotham JN, Schulz JE, et al. Intranasal ChAdOx1 nCoV-19/azd1222 vaccination reduces viral shedding after SARS-CoV-2 D614G challenge in preclinical models. Sci Transl Med. 2021;13(607):eabh0755. doi: 10.1126/scitranslmed.abh0755
- Madhavan M, Ritchie AJ, Aboagye J, et al. Tolerability and immunogenicity of an intranasally-administered adenovirus-vectored COVID-19 vaccine: an open-label partially-randomised ascending dose phase I trial. EBioMedicine. 2022;85:104298. doi: 10.1016/j.ebiom.2022.104298
- WHO. COVID-19 vaccine tracker and landscape. 2023. Available from: https://www.who.int/publications/m/item/draft-landscape-of-covid-19-candidate-vaccines
- Zhu F, Zhuang C, Chu K, et al. Safety and immunogenicity of a live-attenuated influenza virus vector-based intranasal SARS-CoV-2 vaccine in adults: randomised, double-blind, placebo-controlled, phase 1 and 2 trials. Lancet Respir Med. 2022;10(8):749–760. doi: 10.1016/S2213-2600(22)00131-X
- Pilapitiya D, Wheatley AK, Tan H-X. Mucosal vaccines for SARS-CoV-2: triumph of hope over experience. EBioMedicine. 2023;92:104585. doi: 10.1016/j.ebiom.2023.104585
- Sunagar R, Prasad SD, Ella R, et al. Preclinical evaluation of safety and immunogenicity of a primary series intranasal COVID-19 vaccine candidate (BBV154) and humoral immunogenicity evaluation of a heterologous prime-boost strategy with COVAXIN (BBV152). Front Immunol. 2022;13:1063679. doi: 10.3389/fimmu.2022.1063679
- Singh C, Verma S, Reddy P, et al. Phase III pivotal comparative clinical trial of intranasal (iNCOVACC) and intramuscular COVID-19 vaccine (Covaxin®). NPJ Vaccines. 2023;8(1):125. doi: 10.1038/s41541-023-00717-8
- Singh C, Verma S, Reddy P, et al. Immunogenicity and Tolerability of BBV154 (iNCOVACC®), an Intranasal SARS-CoV-2 Vaccine, Compared with Intramuscular Covaxin® in Healthy Adults: A Randomised, Open-Label, Phase 3 Clinical Trial. 2023. doi: 10.2139/ssrn.4342771
- Codagenix. Codagenix initiates dosing in Phase 3 efficacy trial of intranasal COVID-19 vaccine as part of WHO-Sponsored solidarity trial vaccines. 2022; Available from: https://codagenix.com/codagenix-initiates-dosing-in-phase-3-efficacy-trial-of-intranasal-covid-19-vaccine-as-part-of-who-sponsored-solidarity-trial-vaccines/
- Dodaran MS, Banihashemi SR, Es-Haghi A, et al. Immunogenicity and safety of a combined intramuscular/intranasal Recombinant Spike protein COVID-19 vaccine (RCP) in healthy adults aged 18 to 55 years old: a randomized, Double-blind, placebo-controlled, Phase I trial. Vaccines. 2023;11(2):455. doi: 10.3390/vaccines11020455
- Waltz E. China and India approve nasal COVID vaccines — are they a game changer? Nature. 2022;609(7927):450–450. doi: 10.1038/d41586-022-02851-0
- Guillen G, Limonta M, Muzio V, et al. Cuban vaccines Abdala and Mambisa Aagainst COVID-19. Int J Infect Dis. 2023;130:S9–S10. doi: 10.1016/j.ijid.2023.04.027
- Stobart CC, Rostad CA, Ke Z, et al. A live RSV vaccine with engineered thermostability is immunogenic in cotton rats despite high attenuation. Nat Commun. 2016;7(1):13916. doi: 10.1038/ncomms13916
- Lam JH, Shivhare D, Chia TW, et al. Artificial cell membrane polymersome-based intranasal beta Spike formulation as a second generation covid-19 vaccine. ACS Nano. 2022;16(10):16757–16775. doi: 10.1021/acsnano.2c06350
- Adler JM, Vidal RM, Voß A, et al. A non-transmissible live attenuated SARS-CoV-2 vaccine. Mol Ther. 2023;31(8):2391–2407. doi: 10.1016/j.ymthe.2023.05.004
- Nouailles G, Adler JM, Pennitz P, et al. Live-attenuated vaccine sCPD9 elicits superior mucosal and systemic immunity to SARS-CoV-2 variants in hamsters. Nat Microbiol. 2023;8(5):860–874. doi: 10.1038/s41564-023-01352-8
- European Vaccine Initiative. NOSEVAC, a new European collaboration to develop nasal vaccines. 2023; Available from: https://www.euvaccine.eu/post/nosevac-a-new-european-collaboration-to-develop-nasal-vaccines
- Moakes RJA, Davies SP, Stamataki Z, et al. Formulation of a Composite nasal spray Enabling Enhanced surface Coverage and prophylaxis of SARS‐COV‐2. Adv Mater. 2021;33(26):2008304. doi: 10.1002/adma.202008304
- Ludwig M, Enzenhofer E, Schneider S, et al. Efficacy of a carrageenan nasal spray in patients with common cold: a randomized controlled trial. Respir Res. 2013;14(1):124. doi: 10.1186/1465-9921-14-124
- Eccles R, Winther B, Johnston SL, et al. Efficacy and safety of iota-carrageenan nasal spray versus placebo in early treatment of the common cold in adults: the ICICC trial. Respir Res. 2015;16(1):121. doi: 10.1186/s12931-015-0281-8
- Bentley K, Stanton RJ. Hydroxypropyl methylcellulose-based nasal sprays effectively inhibit in vitro SARS-CoV-2 infection and spread. Viruses. 2021;13(12):2345. doi: 10.3390/v13122345
- Necas J, Bartosikova L. Carrageenan: a review. Vet Med (Praha). 2013;58:187–205. doi: 10.17221/6758-VETMED
- Girond S, Crance JM, Cuyck-Gandre HV, et al. Antiviral activity of carrageenan on hepatitis a virus replication in cell culture. Res Virol. 1991;142(4):261–270. doi: 10.1016/0923-2516(91)90011-Q
- Marchetti M, Pisani S, Pietropaolo V, et al. Inhibition of herpes simplex virus infection by negatively charged and neutral carbohydrate polymers. J Chemother. 1995;7(2):90–96. doi: 10.1179/joc.1995.7.2.90
- Carlucci MJ, Scolaro LA, Damonte EB. Inhibitory action of natural carrageenans on herpes simplex virus infection of mouse astrocytes. Chemotherapy. 1999;45(6):429–436. doi: 10.1159/000007236
- Cáceres PJ, Carlucci MJ, Damonte EB, et al. Carrageenans from chilean samples of stenogramme interrupta (phyllophoraceae): structural analysis and biological activity. Phytochemistry. 2000;53(1):81–86. doi: 10.1016/S0031-9422(99)00461-6
- Zacharopoulos VR, Phillips DM. Vaginal formulations of carrageenan protect mice from herpes simplex virus infection. Clin Diagn Lab Immunol. 1997;4(4):465–468. doi: 10.1128/cdli.4.4.465-468.1997
- Stiles J, Guptill-Yoran L, Moore GE, et al. Effects of λ-carrageenan on in vitro replication of feline herpesvirus and on Experimentally Induced Herpetic Conjunctivitis in cats. Invest Ophthalmol Vis Sci. 2008;49(4):1496. doi: 10.1167/iovs.07-1245
- Buck CB, Thompson CD, Roberts JN, et al. Carrageenan is a potent inhibitor of papillomavirus infection. PLOS Pathog. 2006;2(7):e69. doi: 10.1371/journal.ppat.0020069
- Grassauer A, Weinmuellner R, Meier C, et al. Iota-carrageenan is a potent inhibitor of rhinovirus infection. Virol J. 2008;5(1):107. doi: 10.1186/1743-422X-5-107
- Morokutti-Kurz M, Fröba M, Graf P, et al. Iota-carrageenan neutralizes SARS-CoV-2 and inhibits viral replication in vitro. Plos One. 2021;16(2):e0237480. doi: 10.1371/journal.pone.0237480
- Schütz D, Conzelmann C, Fois G, et al. Carrageenan-containing over-the-counter nasal and oral sprays inhibit SARS-CoV-2 infection of airway epithelial cultures. Am J Physiol Lung Cell Mol Physiol. 2021;320(5):L750–L756. doi: 10.1152/ajplung.00552.2020
- Figueroa JM, Lombardo ME, Dogliotti A, et al. Efficacy of a nasal spray containing iota-carrageenan in the postexposure prophylaxis of COVID-19 in hospital personnel dedicated to patients care with COVID-19 disease. Int J Gen Med. 2021;14:6277–6286. doi: 10.2147/IJGM.S328486
- McCarthy TD, Karellas P, Henderson SA, et al. Dendrimers as drugs: discovery and preclinical and clinical development of dendrimer-based microbicides for HIV and STI prevention. Mol Pharm. 2005;2(4):312–318. doi: 10.1021/mp050023q
- Tyssen D, Henderson SA, Johnson A, et al. Structure activity relationship of dendrimer microbicides with dual action antiviral activity. Plos One. 2010;5(8):e12309. doi: 10.1371/journal.pone.0012309
- Castellarnau A, Heery GP, Seta A, et al. Astodrimer sodium antiviral nasal spray for reducing respiratory infections is safe and well tolerated in a randomized controlled trial. Sci Rep. 2022;12(1):10210. doi: 10.1038/s41598-022-14601-3
- Rupp R, Rosenthal SL, Stanberry LR. VivaGel (SPL7013 gel): a candidate dendrimer–microbicide for the prevention of HIV and HSV infection. Int J Nanomed. 2007;2:561–566.
- Romanowski EG, Yates KA, Paull JRA, et al. Topical astodrimer sodium, a non-toxic polyanionic dendrimer, demonstrates antiviral activity in an experimental ocular adenovirus infection model. Molecules. 2021;26(11):3419. doi: 10.3390/molecules26113419
- Paull JRA, Luscombe CA, Castellarnau A, et al. Protective effects of astodrimer sodium 1% nasal spray formulation against SARS-CoV-2 nasal challenge in K18-hACE2 mice. Viruses. 2021;13(8):1656. doi: 10.3390/v13081656
- O’Loughlin J, Millwood IY, McDonald HM, et al. Safety, tolerability, and pharmacokinetics of SPL7013 gel (VivaGel®): a dose ranging, Phase I study. Sex Transm Dis. 2010;37(2):100–104. doi: 10.1097/OLQ.0b013e3181bc0aac
- McGowan I, Gomez K, Bruder K, et al. Phase 1 randomized trial of the vaginal safety and acceptability of SPL7013 gel (VivaGel) in sexually active young women (MTN-004). AIDS. 2011;25(8):1057–1064. doi: 10.1097/QAD.0b013e328346bd3e
- Paull JRA, Heery GP, Bobardt MD, et al. Virucidal and antiviral activity of astodrimer sodium against SARS-CoV-2 in vitro. Antivir Res. 2021;191:105089. doi: 10.1016/j.antiviral.2021.105089
- Eisenhour DD, Brown RK. Bentonite and its impact on modern life. Elements. 2009;5(2):83–88. doi: 10.2113/gselements.5.2.83
- Park J-H, Shin H-J, Kim MH, et al. Application of montmorillonite in bentonite as a pharmaceutical excipient in drug delivery systems. J Pharm Invest. 2016;46(4):363–375. doi: 10.1007/s40005-016-0258-8
- Clark KJ, Sarr AB, Grant PG, et al. In vitro studies on the use of clay, clay minerals and charcoal to adsorb bovine rotavirus and bovine coronavirus. Vet Microbiol. 1998;63(2–4):137–146. doi: 10.1016/S0378-1135(98)00241-7
- Fais F, Juskeviciene R, Francardo V, et al. Drug-free nasal spray as a barrier against SARS-CoV-2 and its Delta variant: In Vitro study of safety and efficacy in human nasal airway epithelia. Int J Mol Sci. 2022;23(7):4062. doi: 10.3390/ijms23074062
- Cheng Y-S. Mechanisms of pharmaceutical aerosol deposition in the respiratory tract. AAPS Pharm Sci Tech. 2014;15(3):630–640. doi: 10.1208/s12249-014-0092-0
- Herrera D, Serrano J, Roldán S, et al. Is the oral cavity relevant in SARS-CoV-2 pandemic? Clin Oral Invest. 2020;24(8):2925–2930. doi: 10.1007/s00784-020-03413-2
- Huang N, Pérez P, Kato T, et al. SARS-CoV-2 infection of the oral cavity and saliva. Nat Med. 2021;27(5):892–903. doi: 10.1038/s41591-021-01296-8
- Papineni RS, Rosenthal FS. The size distribution of droplets in the exhaled breath of healthy human subjects. J Aerosol Med. 1997;10(2):105–116. doi: 10.1089/jam.1997.10.105
- Chavda VP, Baviskar KP, Vaghela DA, et al. Nasal sprays for treating COVID-19: a scientific note. Pharmacol Rep. 2023;75(2):249–265. doi: 10.1007/s43440-023-00463-7
- Popov TA, Emberlin J, Josling P, et al. In vitro and in vivo evaluation of the efficacy and safety of powder hydroxypropylmethylcellulose as nasal mucosal barrier. Med Devices (Auckl). 2020;13:107–113. doi: 10.2147/MDER.S236104
- Shmuel K, Dalia M, Tair L, et al. Low pH Hypromellose (Taffix) nasal powder spray could reduce SARS-CoV-2 infection rate post mass-gathering event at a highly endemic community: an observational prospective open label user survey. Expert Rev Anti-Infective Ther. 2021;19(10):1325–1330. doi: 10.1080/14787210.2021.1908127
- Hull D, Rennie P, Noronha A, et al. Effects of creating a non-specific, virus-hostile environment in the nasopharynx on symptoms and duration of common cold. Acta Otorhinolaryngol Ital. 2007;27(2):73–77.
- Mann BJ. TaffiX® nasal powder forms an effective barrier against SARS-CoV-2. Biomed J Sci Technical Res. 2021;33(3). doi: 10.26717/BJSTR.2021.33.005405
- Ng YL, Salim CK, Chu JJH. Drug repurposing for COVID-19: approaches, challenges and promising candidates. Pharmacol Therapeut. 2021;228:107930. doi: 10.1016/j.pharmthera.2021.107930
- Brüssow H. Clinical trials with antiviral drugs against COVID -19: some progress and many shattered hopes. Environ Microbiol. 2021;23(11):6364–6376. doi: 10.1111/1462-2920.15769
- Aherfi S, Pradines B, Devaux C, et al. Drug repurposing against SARS-CoV-1, SARS-CoV-2 and MERS-CoV. Future Microbiol. 2021;16(17):1341–1370. doi: 10.2217/fmb-2021-0019
- Consortium WHO Solidarity Trial. Remdesivir and three other drugs for hospitalised patients with COVID-19: final results of the WHO solidarity randomised trial and updated meta-analyses. Lancet. 2022;399:1941–1953.
- Group RC, Horby PW, Mafham M, et al. Lopinavir–ritonavir in patients admitted to hospital with COVID-19 (RECOVERY): a randomised, controlled, open-label, platform trial. Lancet. 2020;396(10259):1345–1352. doi: 10.1016/S0140-6736(20)32013-4
- Lamers MM, Haagmans BL. SARS-CoV-2 pathogenesis. Nat Rev Microbiol. 2022;20(5):270–284. doi: 10.1038/s41579-022-00713-0
- Shiraki K, Daikoku T. Favipiravir, an anti-influenza drug against life-threatening RNA virus infections. Pharmacol Therapeut. 2020;209:107512. doi: 10.1016/j.pharmthera.2020.107512
- Sajadian SA, Ardestani NS, Esfandiari N, et al. Solubility of favipiravir (as an anti-COVID-19) in supercritical carbon dioxide: an experimental analysis and thermodynamic modeling. J Supercrit Fluids. 2022;183:105539. doi: 10.1016/j.supflu.2022.105539
- Djekic L. Novel mucoadhesive polymers for nasal drug delivery. In: Pathak Y Yadav H editors. In Nasal drug delivery formulations, developments, challenges, and solutions. Cham:Springer. 2023; p. 189–234. doi: 10.1007/978-3-031-23112-4_11
- Washington N, Steele RJC, Jackson SJ, et al. Determination of baseline human nasal pH and the effect of intranasally administered buffers. Int J Pharm. 2000;198(2):139–146. doi: 10.1016/S0378-5173(99)00442-1
- Alcantara KP, Nalinratana N, Chutiwitoonchai N, et al. Enhanced nasal deposition and anti-coronavirus effect of favipiravir-loaded mucoadhesive chitosan–alginate nanoparticles. Pharmaceutics. 2022;14(12):2680. doi: 10.3390/pharmaceutics14122680
- Gattani V, Dawre S. Development of favipiravir loaded PLGA nanoparticles entrapped in in-situ gel for treatment of covid-19 via nasal route. J Drug Deliv Sci Tec. 2023;79:104082. doi: 10.1016/j.jddst.2022.104082
- Grundeis F, Ansems K, Dahms K, et al. Remdesivir for the treatment of COVID‐19. Cochrane Database Syst Rev. 2023;2023(1):CD014962. doi: 10.1002/14651858.CD014962.pub2
- Tsai Y, Wu J, Liu T, et al. Clinical effectiveness of oral antiviral agents in older patients with COVID‐19 based on real‐world data. J Med Virol. 2023;95(6):e28869. doi: 10.1002/jmv.28869
- Chung S, Peters JM, Detyniecki K, et al. The nose has it: opportunities and challenges for intranasal drug administration for neurologic conditions including seizure clusters. Epilepsy Behav Reports. 2023;21:100581. doi: 10.1016/j.ebr.2022.100581
- Timani KA, Liao Q, Ye L, et al. Nuclear/Nucleolar localization properties of C-terminal nucleocapsid protein of SARS coronavirus. Virus Res. 2005;114(1–2):23–34. doi: 10.1016/j.virusres.2005.05.007
- Caly L, Druce JD, Catton MG, et al. The FDA-approved drug ivermectin inhibits the replication of SARS-CoV-2 in vitro. Antivir Res. 2020;178:104787. doi: 10.1016/j.antiviral.2020.104787
- NIH. Ivermectin | COVID-19 treatment guidelines. Available from: https://www.covid19treatmentguidelines.nih.gov/therapies/miscellaneous-drugs/ivermectin/
- Arshad U, Pertinez H, Box H, et al. Prioritization of anti‐SARS‐Cov‐2 drug repurposing opportunities based on plasma and target site concentrations derived from their established human pharmacokinetics. Clin Pharmacol Ther. 2020;108(4):775–790. doi: 10.1002/cpt.1909
- Errecalde J, Lifschitz A, Vecchioli G, et al. Safety and pharmacokinetic assessments of a novel ivermectin nasal spray formulation in a pig model. J Pharm Sci. 2021;110(6):2501–2507. doi: 10.1016/j.xphs.2021.01.017
- Aref ZF, Bazeed SEES, Hassan MH, et al. Clinical, biochemical and molecular evaluations of ivermectin mucoadhesive nanosuspension nasal spray in reducing upper respiratory symptoms of mild COVID-19. Int J Nanomed. 2021;16:4063–4072. doi: 10.2147/IJN.S313093
- Aref ZF, Bazeed SEES, Hassan MH, et al. Possible role of ivermectin mucoadhesive nanosuspension nasal spray in recovery of post-COVID-19 anosmia. Infect Drug Resist. 2022;15:5483–5494. doi: 10.2147/IDR.S381715
- Reznikov LR, Norris MH, Vashisht R, et al. Identification of antiviral antihistamines for COVID-19 repurposing. Biochem Bioph Res Commun. 2021;538:173–179. doi: 10.1016/j.bbrc.2020.11.095
- Konrat R, Papp H, Kimpel J, et al. The Anti-Histamine Azelastine, identified by computational drug repurposing, inhibits infection by major variants of SARS-CoV-2 in cell cultures and reconstituted human nasal tissue. Front Pharmacol. 2022;13:861295. doi: 10.3389/fphar.2022.861295
- Klussmann JP, Grosheva M, Meiser P, et al. Early intervention with azelastine nasal spray may reduce viral load in SARS-CoV-2 infected patients. Sci Rep-UK. 2023;13(1):6839. doi: 10.1038/s41598-023-32546-z
- Westover J, Ferrer G, Vazquez H, et al. In vitro virucidal effect of intranasally delivered chlorpheniramine maleate compound against severe acute respiratory syndrome coronavirus 2. Cureus. 2020;12:e10501. doi: 10.7759/cureus.10501
- Xu W, Xia S, Pu J, et al. The antihistamine drugs carbinoxamine maleate and chlorpheniramine maleate exhibit potent antiviral activity against a broad spectrum of influenza viruses. Front Microbiol. 2018;9:2643. doi: 10.3389/fmicb.2018.02643
- Sanchez-Gonzalez M, Westover J, Rizvi S, et al. Intranasal chlorpheniramine maleate for the treatment of COVID-19: translational and clinical evidence. Med Res Arch. 2022;10(3):10. doi: 10.18103/mra.v10i3.2752
- Go CC, Pandav K, Sanchez-Gonzalez MA, et al. Potential role of xylitol plus grapefruit seed extract nasal spray solution in COVID-19: case series. Cureus. 2020;12:e11315. doi: 10.7759/cureus.11315
- Cannon ML, Westover JB, Bleher R, et al. In vitro analysis of the anti-viral potential of nasal spray constituents against SARS-CoV-2. bio Rxiv. 2020.408575. doi: 10.1101/2020.12.02.408575
- Nguyen AA, Habiballah SB, Platt CD, et al. Immunoglobulins in the treatment of COVID-19 infection: proceed with caution! Clin Immunol. 2020;216:108459. doi: 10.1016/j.clim.2020.108459
- Ku Z, Xie X, Hinton PR, et al. Nasal delivery of an IgM offers broad protection from SARS-CoV-2 variants. Nature. 2021;595(7869):718–723. doi: 10.1038/s41586-021-03673-2
- Seow HC, Cai J-P, Pan HW, et al. Neutralisation of SARS-CoV-2 by monoclonal antibody through dual targeting powder formulation. J Control Release. 2023;358:128–141. doi: 10.1016/j.jconrel.2023.04.029
- Vabret N, Britton GJ, Gruber C, et al. Immunology of COVID-19: Current state of the science. Immunity. 2020;52(6):910–941. doi: 10.1016/j.immuni.2020.05.002
- Ochi H, Abraham M, Ishikawa H, et al. Oral CD3-specific antibody suppresses autoimmune encephalomyelitis by inducing CD4+CD25−LAP+ T cells. Nat Med. 2006;12(6):627–635. doi: 10.1038/nm1408
- Wu HY, Maron R, Tukpah A-M, et al. Mucosal anti-CD3 monoclonal antibody attenuates collagen-induced arthritis that is associated with induction of LAP+ regulatory T cells and is enhanced by administration of an emulsome-based Th2-skewing adjuvant. J Immunol. 2010;185(6):3401–3407. doi: 10.4049/jimmunol.1000836
- Moreira TG, Matos KTF, Paula GSD, et al. Nasal administration of anti-CD3 monoclonal antibody (foralumab) reduces lung inflammation and blood inflammatory biomarkers in mild to moderate COVID-19 patients: a pilot study. Front Immunol. 2021;12:709861. doi: 10.3389/fimmu.2021.709861
- Cao L, Zhang L, Zhang X, et al. Types of interferons and their expression in plant systems. J Interf Cytokine Res. 2022;42(2):62–71. doi: 10.1089/jir.2021.0148
- Zhou Q, Chen V, Shannon CP, et al. Interferon-α2b treatment for COVID-19. Front Immunol. 2020;11:1061. doi: 10.3389/fimmu.2020.01061
- Zhou Q, MacArthur MR, He X, et al. Interferon-α2b treatment for COVID-19 is associated with improvements in lung abnormalities. Viruses. 2020;13(1):44. doi: 10.3390/v13010044
- Zhou J, Chen X, Lu Y, et al. Interferon-α-2b nasal spray for treating SARS-CoV-2 Omicron variant-infected children. J Clin Immunol. 2023;43(5):862–864. doi: 10.1007/s10875-023-01452-4
- Bogdan C. Nitric oxide and the immune response. Nat Immunol. 2001;2(10):907–916. doi: 10.1038/ni1001-907
- Akerstrom S, Mousavi-Jazi M, Klingstrom J, et al. Nitric oxide inhibits the replication cycle of severe acute respiratory syndrome coronavirus. J Virol. 2005;79(3):1966–1969. doi: 10.1128/JVI.79.3.1966-1969.2005
- Åkerström S, Gunalan V, Keng CT, et al. Dual effect of nitric oxide on SARS-CoV replication: viral RNA production and palmitoylation of the S protein are affected. Virology. 2009;395(1):1–9. doi: 10.1016/j.virol.2009.09.007
- Winchester S, John S, Jabbar K, et al. Clinical efficacy of nitric oxide nasal spray (NONS) for the treatment of mild COVID-19 infection. J Infection. 2021;83(2):237–279. doi: 10.1016/j.jinf.2021.05.009
- Tandon M, Wu W, Moore K, et al. SARS-CoV-2 accelerated clearance using a novel nitric oxide nasal spray (NONS) treatment: a randomized trial. Lancet Reg Health Southeast Asia. 2022;3:100036. doi: 10.1016/j.lansea.2022.100036
- Tada R, Yamazaki H, Nagai Y, et al. Intranasal administration of sodium nitroprusside augments antigen-specific mucosal and systemic antibody production in mice. Int Immunopharmacol. 2023;119:110262. doi: 10.1016/j.intimp.2023.110262
- Boecker D, Zhang Z, Breves R, et al. Antimicrobial efficacy, mode of action and in vivo use of hypochlorous acid (HOCl) for prevention or therapeutic support of infections. GMS Hyg Infect Control. 2023;18:Doc07. doi: 10.3205/dgkh000433
- Giarratana N, Rajan B, Kamala K, et al. A sprayable acid-oxidizing solution containing hypochlorous acid (AOS2020) efficiently and safely inactivates SARS-Cov-2: a new potential solution for upper respiratory tract hygiene. Eur Arch Oto-Rhino-L. 2021;278(8):3099–3103. doi: 10.1007/s00405-021-06644-5
- Panatto D, Orsi A, Bruzzone B, et al. Efficacy of the Sentinox spray in reducing viral load in mild COVID-19 and its virucidal activity against other respiratory viruses: results of a randomized controlled trial and an in vitro study. Viruses. 2022;14(5):1033. doi: 10.3390/v14051033
- Lapuente D, Fuchs J, Willar J, et al. Protective mucosal immunity against SARS-CoV-2 after heterologous systemic prime-mucosal boost immunization. Nat Commun. 2021;12(1):6871. doi: 10.1038/s41467-021-27063-4
- Hoffmann M, Hofmann-Winkler H, Smith JC, et al. Camostat mesylate inhibits SARS-CoV-2 activation by TMPRSS2-related proteases and its metabolite GBPA exerts antiviral activity. EBio Medicine. 2021;65:103225. doi: 10.1016/j.ebiom.2021.103255
- Breining P, Frølund AL, Højen JF, et al. Camostat mesylate against SARS-CoV-2 and COVID-19-Rationale, dosing and safety. Basic Clin Pharmacol Toxicol. 2021;128(2):204–212. doi: 10.1111/bcpt.13533