ABSTRACT
Plants are considered as sessile organisms that cannot avoid adverse environmental conditions and developed complex signaling networks composed of different pathways. WRKY transcription factors (TFs) are key regulators of various plant processes, including the responses to abiotic stresses. WRKY TFs are one of the largest family of transcriptional regulators found in plants. WRKY TFs act as molecular switches that modulate the expression of stress-responsive genes. Stress-induced WRKY TFs expression modulated by a complex transcriptional regulatory network that maintain the proper balance between growth, development, and stress responses. WRKY TFs typically interact with the W-box [TGACC(A/T)] of the target gene promoter to activate the expression of downstream genes. These TFs are associated in the regulation of different physiological processes. Overexpression of various WRKY genes faces the paradox of having various significant effects. These overexpression-associated undesirable phenotypes must be identified and removed for proper plant development. The present review summarized the recent development of various WRKY TFs during adverse environmental conditions as well as their role in plant growth, development and productivity for sustainable agriculture in near future.
Introduction
Biological and environmental stressors affect phonological responses of plant growth and development. Environmental stressors, such as limited water (drought), soil salinity, toxic ions, excess light, cold, UV radiation, wind, oxidative stresses, nutritional imbalance, etc. To survive and promote to the next generation, plants must adapt to these stresses and respond to stress tolerance efficiency. Many TF families, such as WRKY, AP2 (APETLA2)/ERF (ethylene responsive factor), and NAC (NAM, ATAF1/3, and CUC1/2), are unique to plants and its important and specific functions (Jiang et al. Citation2012a; Li et al. Citation2020; Wang et al. Citation2023). With some exceptions, WRKY TFs are DNA-binding proteins initially found in plants. They are interconnected with various plant mechanisms, including plant growth and signaling of stress via auto – and cross-regulation with multiple genes and TFs. The genomes have identified – representing significant information regarding WRKY transcription factors and revealed that the WRKY TFs made a large number of genes in a variety of plants (Xiong et al. Citation2013; Ayadi et al. Citation2016; Mohanta et al. Citation2016; Liu et al. Citation2017; Li et al. Citation2020; Khoso et al. Citation2022; Du et al. Citation2023) [, ].
Figure 1. The number of WRKY transcription factors genes in plants (Khoso et al. Citation2022).
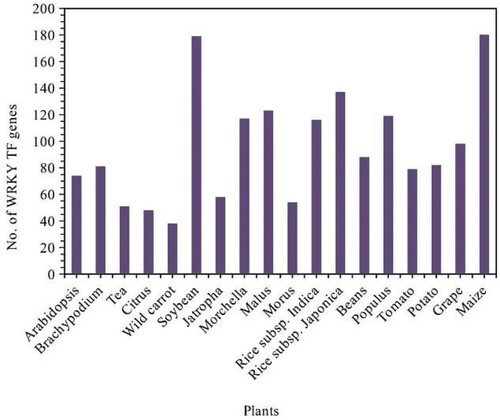
Table 1. Action mechanism of WRKY transcription factors genes in various environmental stressors.
WRKY TFs have a zinc-binding motif of CX4-5CX22-23HXH and an invariant WRKYGQK sequence known as the WRKY domain (Bakshi and Oelmüller Citation2014). Ishiguro and Nakamura (Citation1994) discovered the first member of the WRKY superfamily-SPF1 in Ipomoea batatas plants. Identifying new WRKY genes in plants by the whole-genome sequencing (WGS) of the other variety of plants, especially in model plants. Glycine max, Oryza sativa, Arabidopsis, Brassica napus, and Fragaria vesca each have 197, 100, and 74 members of the WRKY superfamily (Rushton et al. Citation2010; Fan et al. Citation2015; He et al. Citation2016; Wei et al. Citation2017; Li et al. Citation2020; Wang et al. Citation2023). The expression of WRKY TFs induced when plants are exposed to adverse environmental variables or defense signals, i.e. salicylic acid (SA) or other molecules. WRKY proteins have been linked to regulate hormone signaling, senescence, metabolism, trichome and embryo morphogenesis, plant growth and development, and defense against unfavorable environmental conditions (Bakshi and Oelmüller Citation2014; Khan et al. Citation2023).
Genetic engineering is considered an alternative to enhancing stress tolerance efficiency and has significantly contributed to modifying crops’ agronomic responses. Different genes encoding functional proteins, TFs, and proteins associated in the pathways of signal transduction have been identified as genes responding to abiotic stresses (Turan et al. Citation2012; Cohen et al. Citation2021; Khoso et al. Citation2022; Long et al. Citation2023). This review summarizes the recent developments of WRKY-TFs. Different studies have observed that the WRKY-TFs play a major role in stress tolerance capacity. Nowadays, plant genome sequencing has greatly enhanced, especially in economically important crops, and whole-genome sequencing of the WRKY genes (concerning functional plant genes) facilitates screening.
WRKY transcription factors structural characteristics and classification
The DNA-binding domain of WRKY TFs of sixty-amino acids long and has a highly conserved heptapeptide (WRKYGQK) signature motif on the N-terminus and a zinc finger-like motif on the C-terminus (Ishiguro and Nakamura Citation1994; Schmutz et al. Citation2010; Ren et al. Citation2022). WRKY TFs are categorized as a member of the WRKY-GCM1 family and a new Zn-chelating DNA binding domain. The WRKY-GCM1 domain also shared by the DBDs of Mutator transposases and Mutator-like element (MULE) transposases (Marquez and Pritham Citation2010; Wang et al. Citation2023). The GCM1 domain mostly consists of one or two N-terminal WRKY sequences and C-terminal Zn-finger motif, both of which are necessary for the binding of WRKY to the promoters W-box (C/T) TGAC (T/C) (Rushton et al. Citation2010; Wang et al. Citation2023a). Arabidopsis thaliana WRKY4 protein WRKY domain comprises a four-stranded b-sheet with a zinc-binding pocket developed by the Cys/His residues (Yamasaki et al. Citation2005) [].
Figure 2. The WRKY gene family classified into the I (I N and I C), IIa, IIb, IIc, IId, IIe, and III subfamilies (Li et al. Citation2020).
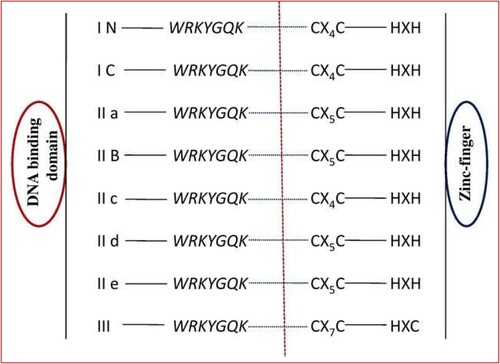
However, the hydrophobic interactions made possible by the Gly residue in the middle of the N-terminal b-strand help stabilize the structure of the b-sheet. Connections between the WRKYGQK motif-containing b-strand and 6-bp region are made, substantially compatible with the W-box (TTGACY) length. Some WRKY proteins have WRRY, WSKY, WKRY, WVKY, or WKKY motifs instead of the WRKY residues in WRKYGQK (Xie et al. Citation2005; Xing et al. Citation2023). WRKYGEK and WRKYGKK are the common variations shared by 7 and 5 domains, respectively, out of the 19 variants of the WRKY domain found in the WRKY family members in Oryza sativa plants (Zhang and Wang Citation2005) and others such as WRICGQK, WRMCGQK, WKKYGQK, WI-KYGQK, WKRYGQK, WSKYEQK, and WRKYSEK (Zhang and Wang Citation2005). The two main categories of zinc-finger-like motifs: C2H2 (C-X4-5-C-X22-23-HXH) and C2-CH (C-X7-C-X23-HXC) (Li et al. Citation2010a, Citation2020).
Based on the WRKY domains and zinc-finger motif, WRKY proteins are categorized into three groups. The zinc-finger-like motif C2H2 (C-X4-5-C-X22-23-HX1H), where X may be any amino acid, is present in Groups I and II members. C2-HC (C-X7-C-X23-HXC) zinc-finger-like motif can be found in group III WRKY proteins (Li et al. Citation2010a). This classification approach avoids the evolutionary history and duplication of genes within big families of WRKY genes and entirely focuses on the proteins’ structural properties. Different models divided into the WRKY proteins to five categories depending on phylogenetic analysis, conserved domains, and intron position of the WRKY domains: group I (I-N terminal and I-C terminal), IIa, IIb, IIc, IId, IIe, and III. Based on the inserted intron, they divided into two groups (Zhang and Wang Citation2005; Wang et al. Citation2023) [].
R-type intron WRKY (IIa and IIb subtypes) falls under the first group, and it has a splicing site between the second and third groups of the arginine codon (AGG). The V-type intron WRKY have its splicing site directly in front of the valine codon, a member of the second category. Within the zinc-finger structure, the valine situated immediately after the 6th amino acid, which follows the 2nd cysteine. V-type intron WRKY proteins primarily belong to I, IIc, IId, and III subtype WRKY TFs. A physiological process transcription can be activated or inhibited by WRKY TFs (Xie et al. Citation2005; Li et al. Citation2020). Most WRKY TFs structures besides the WRKY domain and zinc-finger-like motif, including kinase domains, TIR-NBS-LRR, leucine zippers, serine/threonine-rich, glutamine-rich, proline-rich, serine/threonine-rich, and serine/threonine-rich regions. The WRKY TFs are involved in various transcriptional regulatory activities by these architectures (Zhang and Wang Citation2005; Wang et al. Citation2023).
The impact of WRKY transcription factors on adverse environmental conditions
Adverse environmental conditions negatively impact on plants physio-biochemical action mechanisms (Joshi et al. Citation2016; Verma et al. Citation2022a, Citation2022b). Crop damage or loss in climate challenging regions could be averted if transgenic approach of crop improvement is applied to diverse economically important plants (Du et al. Citation2023). However, WRKYs are of prime importance as they can regulate various abiotic stresses simultaneously. Similarly a single WRKY can mediate different abiotic responses. There are some WRKYs which regulate abiotic responses. If a plant can withstand various abiotic stresses without compromising growth and productivity in field condition, agricultural revolution could be attained. By manipulating expression of a single TF like WRKY this various tolerance capacity could be developed. For this purpose there is a necessity to understand the proper structural and functional relationship of these multiple regulatory WRKY TFs. Further exploration of their function in contrasting verities against stress treatments will also help us to develop plants which may naturally sustain themselves under different stress response (Schluttenhofer and Yuan Citation2015; Phukan et al. Citation2016; Xing et al. Citation2023).
Excess light intensity (High/low temperature)
Major abiotic stress is defined by temperatures higher than an organism can tolerate. Agricultural losses are severe when temperatures are extremely high or low. To increase agricultural production, technological strategies must be developed to protect plant cells from damage by temperature fluctuations (Grover et al. Citation2013; Ohama et al. Citation2017; Cheng et al. Citation2021). Different WRKY TFs react to heat and aid plants in resisting excess light variations. In A. thaliana, excess light intensity enhances the expression of AtWRKY25 and AtWRKY26 while suppressing the expression of AtWRKY33 (Li et al. Citation2011). Overexpression of AtWRKY25 and AtWRKY26 increased tolerance to high light intensity (Li et al. Citation2011, Citation2020). The group II WRKY protein A. thaliana WRKY39 responds to various stressors (Li et al. Citation2010b). AtWRKY39 transcripts are induced by heat treatment, and AtWRKY39 positively regulated by the SA and JA signaling processes. Additionally, plants overexpressed WRKY39 showed improved thermotolerance ability (Li et al. Citation2010b; Cheng et al. Citation2021). TaWRKY70 is positively associated in high-temperature wheat plants resistance to Puccinia striiformis f. sp. Tritici (Pst), which induces stripe rust in Triticum aestivum, during which SA and ET signaling are probably activated (Wang et al. Citation2017).
TaWRKY70 transcript was enhanced significantly when exposed to high temperatures during the initial symptom expression stage of Pst infection and upregulated in plants applied with ethylene (ET), salicylic acid (SA) and cold (4°C) stresses, but reduced in plants applied with methyl jasmonate (MeJA) and high temperature (40°C) stresses. Silencing of TaWRKY70 led to more susceptibility to Pst (Wang et al. Citation2017). According to He et al. (Citation2016), TaWRKY33 transgenic lines also enhanced heat stress efficiency. Mature pollen from chilling-sensitive plants reacts negatively to cold stress. During the cold condition, AtWRKY34 expression was increased. AtWRKY34 expression is pollen-specific and adversely mediates mature Arabidopsis pollen's sensitivity to cold (Zou et al. Citation2010). During cold stress, pollen from the AtWRKY34 mutant had better vitality than the wide-type mutant (Zou et al. Citation2010). Furthermore, even in a setting conducive to normal development, AtWRKY34 overexpression led to partial sterility (Zou et al. Citation2010; Li et al. Citation2020). By interacting with NDPK3a, a Nucleoside Diphosphate Kinase (NDPK), AtWRKY34 was also discovered to be a transcription factor in the sugar induction of mitochondria (Hammargren et al. Citation2008; Wang et al. Citation2023). It is unclear if the AtWRKY34 pollen's sensitivity to cold was involved in mitochondrial metabolism linked to NDPK (, ).
Figure 3. WRKY proteins regulate the plants responses to adverse agroclimatic conditions. At: Arabidopsis thaliana, Ta: Triticum aestivum, Os: Oryza sativa, Vv: Vitis vinifera, Ptr: Poncirus trifoliata, Bc: Brassica campestris, Dg: Dendranthema grandiflorum, Zm: Zea mays, Mx: Malus xiaojinensis, Ah: Arachis hypogaea, Gh: Gossypium hirsutum, Mf: Myrothamnus flabellifolia, Bh: Boea hygrometrica, Cs: Camellia sinensis, Th: Tamarix hispida, Bo: Brassica oleracea, Gs: Glycine soja, Hv: Hordeum vulgare, Ha: Helianthus annuus, Pe: Phyllostachys edulis.
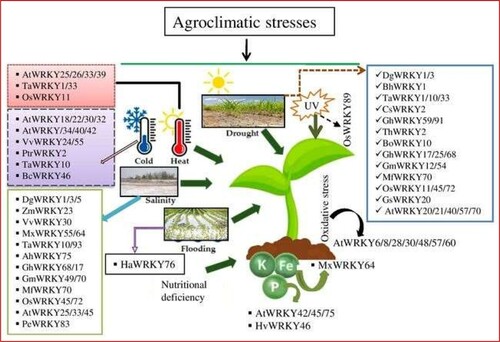
VvWRKY24 from Vitis vinifera was identified as a cold-specific responsive gene using genomic and transcriptomic studies. This gene is always induced during the cold condition but not in response to water or saline stress conditions, causes the expression of VvWRKY30 and VvWRKY52 (Wang et al. Citation2014b) among the 287 WRKY genes in Brassica napus increase during water stress. The potential role of BnaWRKY147, BnaWRKY166, and BnaWRKY210 in cold, saline, and water stress conditions, respectively (He et al. Citation2016) [].
Saline stress
Water stress often leads to excess saline conditions, which causes osmotic stress. In contrast, overexpression of either gene resists saline-stress conditions in AtWRKY25 and AtWRKY33 double mutants (Li et al. Citation2011). Similarly, Dendranthema grandiflorum WRKY genes DgWRKY1 or DgWRKY3 overexpression improves tobacco plants’ efficiency to tolerate salt stress. In transgenic tobacco plants, overexpressing DgWRKY1 or DgWRKY3, the uptake and accumulation of hydrogen peroxide (H2O2) and malondialdehyde (MDA) downregulated during saline stress, along with downregulated enzymatic activities such as catalase (CAT), superoxide dismutase (SOD), peroxidase (POD), etc. (Liu et al. Citation2013). The transgenic plants of rice that overexpressed the OsWRKY45 and OsWRKY72 also enhanced resistance to salt and drought conditions (Qiu and Yu Citation2009; Song et al. Citation2010b). The transgenic lines of rice with OsWRKY11 cDNA linked to the HSP101 promoter had significant light and water stress resistance, as indicated by the slow leaf senescence and less-impaired survival rate of healthy plant organs (Wu et al. Citation2009; Li et al. Citation2020) [].
WRKY gene from wheat (TaWRKY10) was introduced and overexpressed in tobacco plants, considerably improving its tolerance to saline and drought conditions. TaWRKY10 is a key regulator in response to saline and water stress by controlling the balance of osmotic and gene transcription associated with adverse conditions (). Proline and soluble sugar content increased, but the MDA level remained reasonably low when the transgenic lines were subjected to water and saline conditions (Wang et al. Citation2013). The transgenic plants produced when BcWRKY46 (pak choy) and HvWRKY38 (barley) are constitutively overexpressed in A. thaliana increased resistance to saline and limited water supply (Wang et al. Citation2012, Citation2013). Dehydration-responsive element binding (DREB), like soybean (GmDREB2), moss (PpDREB1), and Caragana korshinskii (CkDREB), indicated various patterns of response during abiotic stresses and ABA treatment; constitutive expression of GhWRKY17 in cotton enhances plant resistance capacity to water and saline conditions but reduce the sensitivity of ABA transcript levels, ABA-inducible genes including ABA-responsive element in Zea mays constitutive expression of ZmWRKY23 in A. thaliana likewise increases that plants ability to withstand saline condition (Jiang and Yu Citation2009; Li et al. Citation2020).
Oxidative stress
Oxidative stress is the harmful stress developed by other factors (Sun and Yu Citation2015). A careful equilibrium between ROS generation and scavenging regulates ROS-mediated signaling (Garg and Manchanda Citation2009). Reactive oxygen species in plants differentiate into four different forms, such as singlet oxygen (O2), hydroxyl radicals (OH), superoxide anion (O2•), and hydrogen peroxide. WRKY6, WRKY8, WRKY22, WRKY30, WRKY39, WRKY48, WRKY53, and WRKY75 of the WRKY TFs are enhanced in A. thaliana during H2O2 stress condition. Ascorbate peroxidase 1 (APX1) and cytosolic H2O2-scavenger enzymes are protecting chloroplasts against light stress in A. thaliana plants (Davletova et al. Citation2005). It discovered that knockout-Apx1 (KO-APX1) plants exposed to light stress conditions upregulated WRKY6, WRKY18, WRKY25, WRKY33, WRKY40, WRKY46, WRKY54, WRKY60, WRKY70, and some of the other transcription proteins, implicating APX1 in the defensive response during oxidative stress condition (Davletova et al. Citation2005; Li et al. Citation2020) [].
Sun and Yu (Citation2015) stated that the AtWRKY53 gene was responsible for drought stress response. The expression of WRKY53 can also be induced upon H2O2 treatment (Miao et al. Citation2004). A mitogen-activated protein kinase kinase kinase (MEKK1, a member of mitogen-activated protein kinase MAPK family) may interact with WRKY53 directly to modulate the expression of proteins associated in antioxidantive defense, i.e. CAT1, CAT2, and CAT3 (Miao et al. Citation2007). Additionally, AtWRKY53 overexpression is extremely vulnerable to the limited availability of water conditions. By lowering the amount of H2O2, activated AtWRKY53 expression protected stomatal closure and opening by protecting guard cells to break down starch. AtWRKY53 can directly associate with the QQS promoter regions, increasing starch metabolism (Sun and Yu Citation2015).
AtWRKY46 can modulate transcription by directly binding to the W-box in promoters of the antioxidantive enzyme genes, i.e. monodehydroascorbate reductase (MDHAR), glutathione S-transferase class F14 (GSTF14), and thioredoxin H5 (TRX5) (Ding et al. Citation2014). By interacting with molecules in the MAPKKKa-MEK2-WIPK signaling cascade, AtWRKY8 promotes resistance to Phytophthora infestans, ultimately leading to the induction of apoptosis in the cells of plants (Zhang et al. Citation2012; Li et al. Citation2020). During ABA, H2O2, ABA, salt, and drought conditions, the GhWRKY68 promoter-driven b-glucuronidase activity was increased (Jia et al. Citation2015). By modifying the amount of ABA and boosting the ABA-responsive genes, GhWRKY68 can mediate the responses to salt and water stress. GhWRKY68-overexpressing plants showed decreased resistance to oxidative stress associated with ROS accumulation, downregulated enzymatic activities, and upregulated MDA levels (). They changed the ROS-related expression of genes (Jia et al. Citation2015).
Limited water supply (Drought stress)
Insufficient availability of water is one of the major environmental issues restricting the productivity of crops around the world. Stomata can close directly due to water stress, lowering transpiration and reducing plant water content (Verma et al. Citation2020a, Citation2020b, Citation2021). The development of ROS cleavage complexes in plants, such as ascorbate, glutathione, and α-tocopherol also inhibited as a result, and some soluble solutes accumulation (Tripathi et al. Citation2014; He et al. Citation2016; Wang et al. Citation2023). Different WRKY genes of rice activated by water stress using massively parallel signature sequencing (MPSS) application. An ABR (ABA-responsive elements; G-boxes in the promoter region containing ACGT) is the vital homeopathic component in pathways of ABA-mediated signaling (Khoso et al. Citation2022).
ABI signaling pathway is linked in the ABA signaling pathway via binding to the ABREs in the OsWRKY69 promoter region when the ABA signaling pathway is activated, whereas the ABL1 gene can be inhibited by water stressed conditions (Jiang et al. Citation2017; Yang et al. Citation2020). BhWRKY1 transcription factor can interact with BhGolS1 actively involved in the regulation of BhGolS1 during water stress (Wang et al. Citation2009). In Boea hygrometrica, the BhGolS1 (Galactinol synthase 1) gene is a kind of dehydrogenase and ABA-induced gene that can increase the dehydration resistance capacity. A. thaliana that overexpressed GmWRKY54 showed greater survival efficiency, suggesting that GmWRKY54 could improve Arabidopsis tolerance efficiency to water stress (Zhou et al. Citation2008; Li et al. Citation2020). Overexpression of cotton GhWRKY25 promotes the salt resistance while decreasing water stress tolerance efficiency in Arabidopsis plants (Liu et al. Citation2016). Similarly, it discovered that CsWRKY2 implicated in limited water irrigation in tea plants and exogenous application of ABA increased CsWRKY2 expression. When ABA synthesis inhibitors were applied, CsWRKY2 expression hampered (Wang et al. Citation2017) [].
Overexpressed GsWRKY20 TFs in Arabidopsis had decreased stomata density and water loss efficiency, which improved the water-stress tolerance efficiency of transgenic plants (Luo et al. Citation2013). The transcriptional factors, i.e. NAC, NF-YA, MADS-box, HSF, GRAS, and WRKY families, which upregulate few heat shock proteins (HSPs), early light-inducible (ELIP), and late embryogenesis abundant (LEA), maybe the cause of the inhibition of photosynthesis and genes related to growth under drought conditions (Gechev et al. Citation2013).
The ethylene response factor family is one of the largest family of plant-specific transcription factors associated in the development and stress response of plants, and some plants can negatively regulate resistance to water-deficit by modulating ETH response factors. ThWRKY2 in Tamarix hispidacan initiate the expression of ThERF1 (ETH-responsive factor) gene during water stress condition, which encodes a recent ETH response factor to negatively regulate adverse environmental variables such as water-deficit. Overexpression of ThERF1 in mustard enhanced the rate of transpiration, resulting in more sensitivity to water stress (Wang et al. Citation2017) [ and ]. Effective ROS elimination via the activation of directly or indirectly cellular antioxidative defense mechanisms or the activation of stress-involved gene expression, TaWRKY44, can be a positive regulator in the responses of abiotic stressors (Wang et al. Citation2015, Citation2023). Numerous physiological indices were impacted by the overexpression of GhWRKY68 in N. benthamiana, which downregulate its tolerance to salt and drought stress conditions (Jia et al. Citation2015; Li et al. Citation2020).
Other stresses
Transcription factors of WRKY are also implicated in other stresses like nutritional imbalance, UV-radiation, wind, and other stresses. The transcription factors, i.e. AtWRKY75, AtWRKY6, and AtWRKY42 influence how Arabidopsis acquires phosphate (Pi). AtWRKY75 is substantially upregulated in the plant under Pi deficit, but suppressing WRKY75 expression makes mutant plants more vulnerable to Pi stress conditions and reduces the uptake and accumulation of Pi (Devaiah et al. Citation2007; Li et al. Citation2020). In A. thaliana, the overexpression of AtWRKY6 causes plants to be more sensitive to deficient Pi and minimum Pi concentrations. Additionally, directly attach to two W-boxes of the AtPHO1 promoter and suppressing PHO1 expression, AtWRKY6 modifies activities to deficient Pi (Chen et al. Citation2009). Modulation of Pi homeostasis and crosstalk between P and Fe deficiency, rice OsWRKY74 associated with resistance to phosphate and cold stress conditions (Dai et al. Citation2016). OsWRKY74 overexpression dramatically improved resistance to Pi deficiency. In a hydroponic solution lacking in Pi, rice plants overexpressing the OsWRKY74 gene have optimum root, shoot biomass, and phosphorus concentration compared to wild-type plants (). When grown in P-deficient conditions, rice OsWRKY74-overexpressing plants showed enhanced tiller number, grain weight, and P content in greenhouse conditions (Dai et al. Citation2016; Wang et al. Citation2023) [].
According to Chen et al. (Citation2009), AtWRKY42 and AtWRKY6 work together to the W-boxes of the PHO1 promoter and suppress PHO1 production. Additionally, in Arabidopsis, AtWRKY42 controlled phosphate homeostasis. Like the phosphate1(pho1) mutant, the WRKY42-over-expressing lines were more vulnerable to deficient-inorganic phosphate stress and minimum shoot Pi contents than wild-type plants. To control the changes in the availability of Pi in the environment, AtWRKY42 controlled Pi homeostasis by containing the PHO1 and PHT1 expression (Su et al. Citation2015). Similarly, AtWRKY42 and AtWRKY45 directly upregulate expression in the Arabidopsis response to Pi deficiency. AtWRKY45 expression was noticeably induced during Pi deprivation, especially in plant roots. In Arabidopsis, AtWRKY45 overexpression boosted Pi level and accumulation, but AtWRKY45 RNA interference suppression reduced the content and accumulation of Pi (Wang et al. Citation2014a).
Numerous WRKY TFs have been associated with the positive and negative regulation of leaf senescence in various plants (Zentgraf et al. Citation2010). Transgenic rice plants overexpressing the OSWRKY89 have higher wax deposition on the surfaces of leaves and improved UV-radiation stress resistance capacity (Wang et al. Citation2007, Citation2023). 25 bp (AAGATCTAC-CATTGCTCTATAGCTT) regions between 1,188 and 1,213 upstream of the initial site of translation of OsWRKY89 gene as a UV-B response cis-regulatory element (Zhao et al. Citation2010; Li et al. Citation2020). Additionally, WRKY TFs control the senescence of leaves. For instance, AtWRKY57 inhibits leaf senescence by interacting with JA and Auxin signaling pathways, JAZ4/JAZ8 (JASMONATE ZIM-DOMAIN4/8) and IAA29 (Indole Acetic Acid 29).
The antagonistic role of JA and auxin in leaf senescence are consistent with the competing interactions between JAZ4/8 and IAA29 with WRKY57 and their distinct mechanisms in JA-induced senescence of the leaf (Jiang et al. Citation2014b). AtWRKY53 and AtWRKY70 are positive and negative regulators of leaf senescence in Arabidopsis plants. Two other WRKY TFs (AtWRKY 54 and AtWRKY 30) engaged in this process. According to single and double-mutant experiments, the structurally related AtWRKY54 and AtWRKY70 show similar expression patterns throughout leaf development and seem to have cooperative and partially redundant senescence activities. It has been demonstrated that AtWRKY30 and the positive regulator of AtWRKY53 interact separately with these negative senescence regulators. Since AtWRKY30 was expressed at the developmental stage of leaf senescence, the associate protein could interact with the other WRKYs in the senescence regulatory network. Expression in salicylic acid-deficient mutants and wild-type cells points to a shared but not exclusive function for SA in the senescence-related upregulation of AtWRKY30, 53, 54, and 70 (Hu et al. Citation2012).
WRKY transcription factors regulatory network during stress conditions
WRKY family members have different regulatory mechanisms. WRKY protein can be effectively interacting with W-box elements to activate or inhibit the transcription of downstream target genes. However, it can also associate other acting elements to form protein complexes, which increases the transcription binding activity (Banerjee and Roychoudhury Citation2015; Long et al. Citation2023). It regulates the defensive mechanism of plants to multiple stimuli. PcWRKY1 (Petroselinum crispum) can associate to W-box in its promoter area as well as in the PcWRKY3 promoter, according to CHIP analysis (Turck et al. Citation2004; Wang et al. Citation2023). CaWRKY6 of Capsicum frutescens may activate CaWRKY40 and make the plant more resistance to humidity and high temperature stress. Glycine max (GmWRKY27) not only inhibits the activity of downstream (GmNAC29) promoter by independent inhibition, but also cooperatively interacts with GmMYB174 to inhibit the expression of GmNAC29, thereby enhancing water and saline stress tolerance (Cai et al. Citation2015).
ABA treatment increased the expression of AtWRKY60 in wild-type A. thaliana but not in AtWRKY18 or AtWRKY40 mutants, suggesting that WRKY60 can be direct target gene of WRKY18 or WRKY40 in the ABA signaling pathway (Xu et al. Citation2006; Chen et al. Citation2010). AtWRKY25, AtWRKY26, and AtWRKY33 controlled the heat-induced response. To retain heat stress resistance, AtWRKY25 associated with AtWRKY26 and AtWRKY33. The constitutive expression of AtWRKY33 improved stress resistance efficiency (Li et al. Citation2011, Citation2020; Khan et al. Citation2023). MAPK signaling cascade's WRKY TFs, all eukaryotic species include the mitogen-activated protein kinase (MAPK) signaling cascade, which plays a significant function in the downstream signaling of ABA-dependent defensive functions in plants (de Zelicourt et al. Citation2016). It is associated with controlling plant developmental and actions to environmental stressors. Multiple phosphorylation responses in MAPK signaling cascades connect upstream receptors to downstream TFs (Ishihama and Yoshioka Citation2012). Group I WRKY TFs are activated by MAPK-dependent phosphorylation, highlighting their significance on plant immune systems (Ishihama and Yoshioka Citation2012). These TFs have a conserved motif in the N-terminal region. Without pathogen infection, the transcription factor WRKY33 interacts with MAP kinase 4 (MPK4) to create a MAMP or PAMP complex in Arabidopsis (Qiu et al. Citation2008). It is associated with the phosphorylation of MKS1, a substrate of MPK4 that triggered by pathogen infection. The production of PAD3, an enzyme necessary for developing complexes of antimicrobials activated by AtWRKY33 (Qiu et al. Citation2008; Wang et al. Citation2023a).
Additionally, AtWRKY22 and AtWRKY29 play a crucial role in MAPK-mediated tolerance to bacterial and fungal pathogens. Pathogen resistance conferred by the transient expression of the AtWRKY29 homologue gene in Arabidopsis plant leaves. The AtWRKY29 homolog AtWRKY22 can identify and link to the same promoters as AtWRKY29 and confer similar functions. Another illustration is OsWRKY30, which increases rice tolerance efficiency to drought through a cascade of phosphorylated MAPKs (Danquah et al. Citation2014). By activating RBOHB, Nicotiana benthamiana NADPH oxidase, the MAPK-WRKY pathway is necessary for ROS bursts triggered by the AVR3a effector and the INF1 pattern (Adachi et al. Citation2015). Various binding patterns and preferences of binding sites permit for the regulation of downstream target genes, providing WRKY TFs with various functions in the plant transcriptional regulation network.
WRKY TFs facilitate signal transduction for plant hormones
WRKY TFs are essential for the pathways of SA and ABA-mediated signals. When exposed to SA or jasmonic methyl acid (MeJA), AtWRKY39 activates with other proteins to control the SA and JA signaling pathways in response to high temperature (Dong et al. Citation2003; Li et al. Citation2010b). Suppressing the SA-induced expression of the protective gene Pathogenesis-Related1 (AtPR1), overexpression of AtWRKY38 or AtWRKY62 reduces plants tolerance to pathogens (Kim et al. Citation2008; Li et al. Citation2020). When OsWRKY45 expression inhibited, SA-mediated defenses against benzothiadiazole are severely compromised, whereas overexpressed, rice is much more resistant to blasts (Shimono et al. Citation2007; Wang et al. Citation2023). OsWRKY45 is essential for the responses of SA-mediated defense. Indicating its function in the SA-dependent defensive signaling system, overexpression of SA-inducible PtrWRKY89 expression of PR protein and enhances tolerance to Marssonina brunnea in poplar plants (Jiang et al. Citation2014a).
Abscisic acid (ABA) is important for integrating different stress signals and regulating subsequent stress reactions. Few WRKY TFs participate in stress-related signaling pathways that ABA mediates. Synergistic interactions with ABA and transcriptional activators of VP1 and ABI5, LtWRKY21, activate the promoter of the ABA-inducible gene HVA22 and enhance its expression (Zou et al. Citation2004). By assessment of WRKY-associated T-DNA insertion mutants, the gain-of-function mutant acquired water stress resistance efficiency (adt). Adt mutant plants receive more ABA as compared to wild-type plants. T-DNA insertion related to the WRKR57-activated expression. ChIP experiments showed that the WRKY57 might be directly associated with the W-box of the 9-cis-epoxy carotenoid dioxygenase 3 (NCED3) and Responsive to Desiccation 29A (RD29A) promoters to start the production of genes (Jiang et al. Citation2012b; Wang et al. Citation2023).
AtWRKY40 inhibits the expression of numerous ABA-inducible genes by binding to the W-box in the promoters of these genes, including AtABF4, AtABI4, AtABI5, AtDREB1A, AtMYB2, and AtRAB18 (Shang et al. Citation2010; Li et al. Citation2020). WRKY18, WRKY40, and WRKY60 interacted with ABI4 and ABI5 to control their stream expression of genes in the Arabidopsis ABA signaling pathway. It includes the central negative regulator of WRKY40 and WRKY18, which increases the suppression of ABI4 and ABI5 transcription caused by WRKY40 and WRKY60, which counteracts the effect of WRKY40 (Shang et al. Citation2010; Du et al. Citation2023). Group II WRKY gene CsWRKY46 from the cucumber (Cucumis sativus) was increased during cold and ABA application. In the nucleus, CsWRKY46 expressed, and it interacts with the W-box in the promoter of ABI5. Transgenic Arabidopsis overexpressing the CsWRKY46 gene higher seedling survival following cold stress conditions, significantly more proline content uptake and accumulation, downregulated membrane injury index, MDA content, and hypersensitivity to ABA at the stage of seed germination (Zhang et al. Citation2016b).
ABA-mediated pathway, CmWRKY1, group IIb WRKY family members screened from Chrysanthemum morifolium plants, which is critical for responding to water deficit conditions in chrysanthemum plants. Additionally, transgenic lines overexpressing CmWRKY1 improved dehydration tolerance in response to polyethylene glycol (PEG) stress conditions. The transgenic plants positively activated gene expression, controlled by ABA, and suppressed gene expression levels favorably controlled by ABA, i.e. PP2C, ABI1, and ABI2 (Fan et al. Citation2016).
Conclusion and future prospects
Plants are vulnerable to adverse environmental variables that marked influence on the plants development and productivity. WRKY TFs play a major role in plant responses to environmental stresses. One of the largest TF families, WRKY-TFs acts as molecular switches that regulate the expression of stress-sensitive genes. Plants have evolved various stress-tolerant strategies for signal transduction and regulatory networks that control various plant processes linked with plant development and stress responses. This article provides the helpful information for further investigation on the function and roles of WRKY genes playing in regulating development and physiological processes in these important stress-tolerance crops. However, different epigenetic regulation processes can modulate WRKYs and downstream genes. Advances in omic studies help to understand the WRKY genes in a variety of plant species and help to reveal the action mechanism of WRKY TFs in plant stress responses. As WRKY TFs are essential for plant metabolism, clarifying how WRKY proteins regulate metabolism is a key step in modulating crop immunity. However, genetic confirmations joined to the latest technologies are increasing to confirm the novel role of the WRKY genes, expression of WRKY-TF or downstream genes regulated by self-regulation of WRKY-TF, which helps to simplify the regulatory network of responses to abiotic stresses. Future studies should explore noncoding RNAs and epigenetic modifications involved in the regulation of WRKY-TFs. Based on current studies the role of WRKY-TFs in regulating plant responses related to abiotic stresses, particularly drought, salinity, and temperature stress, are not sufficiently detailed, particularly at the transcriptional level. Finally, the use of WRKY-TF screening for plant stress tolerance in context to increase climate change significantly improves crop yield and crop quality.
Ethics statement
Authors did not use any animal or human experimental materials, and are not, therefore, subject to their ethical concerns.
Acknowledgment
The authors would like to thank the Guangxi Academy of Agricultural Sciences, Nanning, Guangxi, China, for providing the necessary facilities for this study.
Disclosure statement
No potential conflict of interest was reported by the author(s).
Additional information
Funding
Notes on contributors
Yuan Zeng
Yuan Zeng is a research assistant at Guangxi Key Laboratory of Sugarcane Genetic Improvement, Guangxi Academy of Agricultural Sciences, Nanning, Guangxi, China.
Shanshan He
Shanshan He is a research assistant at Sugarcane Research Institute, Guangxi Academy of Agricultural Sciences, Nanning, Guangxi, China.
Yan Jing
Yan Jing is a research assistant at Sugarcane Research Institute, Guangxi Academy of Agricultural Sciences, Nanning, Guangxi, China.
Yongqian Nong
Yongqian Nong is a senior researcher at Chongzuo Institute of Agricultural Sciences, Nanning, Guangxi, China
Shiyun Tang
Shiyun Tang is a senior researcher at Sugarcane Research Institute, Guangxi Academy of Agricultural Sciences, Nanning, Guangxi, China.
Zuli Yang
Zuli Yang is a senior researcher at Laibin Academy of Agricultural Sciences, Laibin, Guangxi, China
Zhiyou Su
Zhiyou Su is a senior researcher at Chongzuo Institute of Agricultural Sciences, Nanning, Guangxi, China
Meiyan Huang
Meiyan Huang is a senior researcher at Chongzuo Institute of Agricultural Sciences, Nanning, Guangxi, China
Xingwen Pan
Xingwen Pan is a senior researcher at Chongzuo Institute of Agricultural Sciences, Nanning, Guangxi, China
Ting Luo
Ting Luo is a senior researcher at Sugarcane Research Institute, Guangxi Academy of Agricultural Sciences, Nanning, Guangxi, China.
Jinju Wei
Jinju Wei is a senior researcher at Sugarcane Research Institute, Guangxi Academy of Agricultural Sciences, Nanning, Guangxi, China.
Baoqing Zhang
Baoqing Zhang is a senior researcher at Sugarcane Research Institute, Guangxi Key Laboratory of Sugarcane Genetic Improvement, Guangxi Academy of Agricultural Sciences, Nanning, Guangxi, China.
Ming Li
Ming Li is a Professor and Senior Scientist at Sugarcane Research Institute, Guangxi Key Laboratory of Sugarcane Genetic Improvement, Guangxi Academy of Agricultural Sciences, Nanning, Guangxi, China.
References
- Adachi H, Nakano T, Miyagawa N, Ishihama N, Yoshioka M, Katou Y, Yaeno T, Shirasu K, Yoshioka H. 2015. NWRKY transcription factors phosphorylated by MAPK regulate a plant immune NADPH oxidase in Nicotiana benthamiana. Plant Cell. 27:2645–2663. doi:10.1105/tpc.15.00213.
- An JP, Zhang XW, You CX, Bi SQ, Wang XF, Hao YJM. 2019. WRKY 40 promotes wounding-induced anthocyanin biosynthesis in association with md MYB 1 and undergoes md BT 2-mediated degradation. New Phytol. 224:380–395. doi:10.1111/nph.16008.
- Ayadi M, Hanana M, Kharrat N, Merchaoui H, Marzoug RB, Lauvergeat V, Rebai A, Mzid R. 2016. The WRKY transcription factor family in citrus: valuable and useful candidate genes for citrus breeding. Appl. Biochem. Biotechnol. 180:516–543. doi:10.1007/s12010-016-2114-8.
- Bakshi M, Oelmüller R. 2014. WRKY transcription factors: jack of many trades in plants. Plant Signaling Behav. 9:e27700. doi:10.4161/psb.27700.
- Banerjee A, Roychoudhury A. 2015. WRKY proteins: signaling and regulation of expression during abiotic stress responses. Sci. World J. 2015:1–17. doi:10.1155/2015/807560.
- Cai H, Yang S, Yan Y, Xiao Z, Cheng J, Wu J, Qiu A, Lai Y, Mou S, Guan D, et al. 2015. CaWRKY6 transcriptionally activates CaWRKY40, regulates Ralstonia solanacearum resistance, and confers high-temperature and high-humidity tolerance in pepper. J. Exp. Bot. 66:3163–3174. doi:10.1093/jxb/erv125.
- Chen L, Zhang L, Yu D. 2010. Wounding-induced WRKY8 is involved in basal defense in Arabidopsis. Mol. Plant Microbe Interact. 23:558–565. doi:10.1094/MPMI-23-5-0558.
- Chen YF, Li LQ, Xu Q, Kong YH, Wang H, Wu WH. 2009. The WRKY6 transcription factor modulates PHOSPHATE1 expression in response to low Pi stress in Arabidopsis. Plant Cell. 21:3554–3566. doi:10.1105/tpc.108.064980.
- Cheng Z, Luan Y, Meng J, Sun J, Tao J, Zhao D. 2021. WRKY transcription factor response to high-temperature stress. Plants. 10(10):2211. doi:10.3390/plants10102211.
- Cohen I, Zandalinas SI, Huck C, Fritschi FB, Mittler R. 2021. Meta-analysis of drought and heat stress combination impact on crop yield and yield components. Physiol Plant. 171:66–76. doi:10.1111/ppl.13203.
- Dai X, Wang Y, Zhang WH. 2016. OsWRKY74, a WRKY transcription factor, modulates tolerance to phosphate starvation in rice. J. Exp. Bot. 67:947–960. doi:10.1093/jxb/erv515.
- Danquah A, de Zelicourt A, Colcombet J, Hirt H. 2014. The role of ABA and MAPK signaling pathways in plant abiotic stress responses. Biotech. Adv. 32:40–52. doi:10.1016/j.biotechadv.2013.09.006.
- Davletova S, Rizhsky L, Liang H, Shengqiang Z, Oliver DJ, Coutu J, Shulaev V, Schlauch K, Mittler R. 2005. Cytosolic ascorbate peroxidase 1 is a central component of the reactive oxygen gene network of Arabidopsis. Plant Cell. 17:268–281. doi:10.1105/tpc.104.026971.
- Devaiah BN, Karthikeyan AS, Raghothama KG. 2007. WRKY75 transcription factor is a modulator of phosphate acquisition and root development in Arabidopsis. Plant Physiol. 143:1789–1801. doi:10.1104/pp.106.093971.
- de Zelicourt A, Colcombet J, Hirt H. 2016. The role of MAPK modules and ABA during abiotic stress signaling. Trends Plant Sci. 21:677–685. doi:10.1016/j.tplants.2016.04.004.
- Ding ZJ, Yan JY, Xu XY, Yu DQ, Li GX, Zhang SQ, Zheng SJ. 2014. Transcription factor WRKY46 regulates osmotic stress responses and stomatal movement independently in Arabidopsis. Plant J. 79:13–27. doi:10.1111/tpj.12538.
- Dong J, Chen C, Chen Z. 2003. Expression profiles of the ArabidopsisWRKY gene superfamily during plant defense response. Plant Mol. Biol. 51:21–37. doi:10.1023/a:1020780022549.
- Du P, Wang Q, Yuan DY, Chen SS, Su YN, Li L, Chen S, He XJ. 2023. WRKY transcription factors and OBERON histone-binding proteins form complexes to balance plant growth and stress tolerance. EMBO J. 42(19):e113639. doi:10.15252/embj.2023113639.
- Fan Q, Song A, Jiang J, Zhang T, Sun H, Wang Y, Chen S, Chen F. 2016. CmWRKY1 enhances the dehydration tolerance of chrysanthemum through the regulation of ABA-associated genes. PLoS One. 11:e0150572. doi:10.1371/journal.pone.0150572.
- Fan X, Guo Q, Xu P, Gong Y, Shu H, Yang Y, Shen X. 2015. Transcriptome-wide identification of salt-responsive members of the WRKY gene family in Gossypium aridum. PLoS One. 10:e0126148. doi:10.1371/journal.pone.0126148.
- Fan Z-Q, Tan X-L, Shan W, Kuang J-F, Lu W-J, Chen J-Y. 2018. Characterization of a transcriptional regulator, BrWRKY6, associated with gibberellin-suppressed leaf senescence of Chinese flowering cabbage. J. Agric. Food Chem. 66:1791–1799. doi:10.1021/acs.jafc.7b06085.
- Garg N, Manchanda G. 2009. ROS generation in plants: boon or bane? Plant Biosyst. 143:81–96. doi:10.1080/11263500802633626.
- Gechev TS, Benina M, Obata T, Tohge T, Sujeeth N, Minkov I, Toneva V. 2013. Molecular mechanisms of desiccation tolerance in the resurrection glacial relic Haberlea rhodopensis. Cell Mol. Life Sci. 70:689–709. doi:10.1007/s00018-012-1155-6.
- Grover AL, Mittal D, Negi M, Lavania D. 2013. Generating high temperature tolerant transgenic plants: achievements and challenges. Plant Sci. 205–206:38–47. doi:10.1016/j.plantsci.2013.01.005.
- Gu L, Ma Q, Zhang C, Wang C, Wei H, Wang H, et al. 2019b. The cotton GhWRKY91 transcription factor mediates leaf senescence and responses to drought stress in transgenic Arabidopsis thaliana. Front. Plant Sci. 10:1352. doi:10.3389/fpls.2019.01352.
- Gu L, Wei H, Wang H, Su J, Yu S. 2018. Characterization and functional analysis of GhWRKY42, a group IId WRKY gene, in upland cotton (Gossypium hirsutum L.). BMC Genet. 19:1–14. doi:10.1186/s12863-018-0653-4.
- Guo J-J, Li S, Li H-Y, Li W, Li D-H. 2021. Expression of the kale WRKY gene BoWRKY10 in transgenic tobacco confers drought stress tolerance. Russian J. Plant Physiol. 68:147–157. doi:10.1134/S1021443721010076.
- Hammargren J, Rosenquist S, Jansson C, Knorpp C. 2008. A novel connection between nucleotide and carbohydrate metabolism in mitochondria: sugar regulation of the Arabidopsis nucleoside diphosphate kinase 3a gene. Plant Cell Rep. 27:529–534. doi:10.1007/s00299-007-0486-5.
- Han D, Xu T, Han J, Liu W, Wang Y, Li X, Sun X, Wang X, Li T, Yang G. 2021a. Overexpression of MxWRKY53 increased iron and high salinity stress tolerance in arabidopsis thaliana. In Vitro Cell. Dev. Biology-Plant. 58(2):266–278. doi:10.1007/s11627-021-10241-w.
- Han D, Zhou Z, Du M, Li T, Wu X, Yu J, Zhang P, Yang G. 2020. Overexpression of a malus xiaojinensis WRKY transcription factor gene (MxWRKY55) increased iron and high salinity stress tolerance in arabidopsis thaliana. Vitro Cell. Dev. Biology-Plant. 56:600–609. doi:10.1007/s11627-020-10129-1.
- He GH, Xu JY, Wang YX, Liu JM, Li PS, Chen M, Ma YZ, Xu ZS. 2016. Drought-responsive WRKY transcription factor genes TaWRKY1 and TaWRKY33 from wheat confer drought and/or heat resistance in Arabidopsis. BMC Plant Bio. l16:116. doi:10.1186/s12870-016-0806-4.
- Hu Y, Dong Q, Yu D. 2012. ArabidopsisWRKY46 coordinates with WRKY70 and WRKY53 in basal resistance against pathogen Pseudomonas syringae. Plant Sci. 185–186:288–297. doi:10.1016/j.plantsci.2011.12.003.
- Ishiguro S, Nakamura K. 1994. Characterization of a cDNA encoding a novel DNA-binding protein, SPF1, that recognizes SP8 sequences in the 5′upstream regions of genes coding for sporamin and b-amylase from sweet potato. Mol. Gen. Genet. 244:563–571. doi:10.1007/BF00282746.
- Ishihama N, Yoshioka H. 2012. Post-translational regulation of WRKY transcription factors in plant immunity. Curr. Opin. Plant Biol. 15:431–437. doi:10.1016/j.pbi.2012.02.003.
- Jia H, Wang C, Wang F, Liu S, Li G, Guo X. 2015. GhWRKY68 reduces resistance to salt and drought in transgenic Nicotiana benthamiana. PLoS One. 10:e0120646. doi:10.1371/journal.pone.0213540.
- Jiang J, Ma S, Ye N, Jiang M, Cao J, Zhang J. 2017. WRKY transcription factors in plant responses to stresses. J. Integrative Plant Biol. 59(2):86–101. doi:10.1111/jipb.12513.
- Jiang W, Yu D. 2009. Arabidopsis WRKY2 transcription factor may be involved in osmotic stress response. Acta Bot. Yunnan. 31:427–432. doi:10.1186/1471-2229-9-96.
- Jiang Y, Duan Y, Yin J, Ye S, Zhu J, Zhang F, Lu W, Fan D, Luo K. 2014b. Genome-wide identification and characterization of the Populus WRKY transcription factor family and analysis of their expression in response to biotic and abiotic stresses. J. Exp. Bot. 65:6629–6644. doi:10.1093/jxb/eru381.
- Jiang Y, Liang G, Yang S, Yu D. 2014a. ArabidopsisWRKY57 functions as a node of convergence for jasmonic acid- and auxin-mediated signaling in jasmonic acid-induced leaf senescence. Plant Cell. 26:230–245. doi:10.1105/tpc.113.117838.
- Jiang Y, Liang G, Yu D. 2012b. Activated expression of WRKY57 confers drought tolerance in Arabidopsis. Mol. Plant. 5:1375–1388. doi:10.1093/mp/sss080.
- Jiang Y, Zeng B, Zhao H, Zhang M, Xie S, Lai J. 2012a. Genome-wide transcription factor gene prediction and their expressional tissue-specificities in maize. J. Integr. Plant Biol. 54:616–630. doi:10.1111/j.1744-7909.2012.01149.x.
- Joshi R, Wani SH, Singh B, Bohra A, Dar ZA, Lone AA, Pareek A, Singla-Pareek SL. 2016. Transcription factors and plants response to drought stress: current understanding and future directions. Front. Plant Sci. 7:1029. doi:10.3389/fpls.2016.01029.
- Kang G, Yan D, Chen X, Yang L, Zeng R. 2021. HbWRKY82, a novel IIc WRKY transcription factor from Hevea brasiliensis associated with abiotic stress tolerance and leaf senescence in arabidopsis. Physiol Plant. 171:151–160. doi:10.1111/ppl.13238.
- Khan I, Asaf S, Jan R, Bilal S, Khan AL, Kim K. 2023. Dynamic interplay of WRKY, GRAS, and ERF transcription factor families in tomato-endophytic fungal symbiosis: Insights from transcriptome and genome-wide analysis. Front. Plant Sci. 14:1181227. doi:10.3389/fpls.2023.1181227.
- Khoso MA, Hussain A, Ritonga FN, Ali Q, Channa MM, Alshegaihi RM, Meng Q, Ali M, Zaman W, Brohi RD, et al. 2022. WRKY transcription factors (TFs): molecular switches to regulate drought, temperature, and salinity stresses in plants. Front. Plant Sci. 13:1039329. doi:10.3389/fpls.2022.1039329.
- Kim KC, Lai Z, Fan B, Chen Z. 2008. ArabidopsisWRKY38 and WRKY62 transcription factors interact with histone deacetylase 19 in basal defense. Plant Cell. 20:2357–2371. doi:10.1105/tpc.107.055566.
- Li F, Li M, Wang P, Cox Jr. KL, Duan L, Dever JK, Shan L, Li Z, He P. 2017a. Regulation of cotton (Gossypium hirsutum) drought responses by mitogen-activated protein (MAP) kinase cascade-mediated phosphorylation of gh WRKY 59. New Phytol. 215:1462–1475. doi:10.1111/nph.14680.
- Li H, Xu Y, Xiao Y, Zhu Z, Xie X, Zhao H, Wang Y. 2010a. Expression and functional analysis of two genes encoding transcription factors, VpWRKY1 and VpWRKY2, isolated from Chinese wild Vitis pseudoreticulata. Planta. 232:1325–1337. doi:10.1007/s00425-010-1258-y.
- Li S, Fu Q, Chen L, Huang W, Yu D. 2011. Arabidopsis thaliana WRKY25, WRKY26, and WRKY33 coordinate induction of plant thermotolerance. Planta. 233:1237–1252. doi:10.1007/s00425-011-1375-2.
- Li S, Zhou X, Chen L, Huang W, Yu D. 2010b. Functional characterization of Arabidopsis thaliana WRKY39 in heat stress. Mol Cells. 29:475–483. doi:10.1007/s10059-010-0059-2.
- Li W, Pang S, Lu Z, Jin B. 2020. Function and mechanism of WRKY transcription factors in abiotic stress responses of plants. Plants. 9:1515. doi:10.3390/plants9111515.
- Liu QL, Zhong M, Li S, Pan YZ, Jiang BB, Jia Y, Zhang HQ. 2013. Overexpression of a chrysanthemum transcription factor gene, DgWRKY3, in tobacco enhances tolerance to salt stress. Plant Physiol. Biochem. 69:27–33. doi:10.1016/j.plaphy.2013.04.016.
- Liu Q-N, Liu Y, Xin Z-Z, Zhang D-Z, Ge B-M, Yang R-P, Wang Z-F, Yang L, Tang B-P, Zhou C-L. 2017. Genome-wide identification and characterization of the WRKY gene family in potato (Solanum tuberosum). Biochem. Systematics Ecol. 71:212–218. doi:10.1016/j.bse.2017.02.010.
- Liu X, Song Y, Xing F, Wang N, Wen F, Zhu C. 2016. GhWRKY25, a group IWRKY gene from cotton, confers differential tolerance to abiotic and biotic stresses in transgenic Nicotiana benthamiana. Protoplasma. 253:1265–1281. doi:10.1007/s00709-015-0885-3.
- Long L, Gu L, Wang S, Cai H, Wu J, Wang J, Yang M. 2023. Progress in the understanding of WRKY transcription factors in woody plants. Int. J. Biol. Macromol. 242:124379. doi:10.1016/j.ijbiomac.2023.124379.
- Luan Y, Chen Z, Fang Z, Huang X, Zhao D, Tao J. 2023b. PoWRKY71 is involved in Paeonia ostii resistance to drought stress by directly regulating light-harvesting chlorophyll a/b-binding 151 gene. Hort. Res. 10(11). doi:10.1093/hr/uhad194.
- Luan Y, Chen Z, Meng J, Tao J, Zhao D. 2023a. PoWRKY17 promotes drought tolerance in Paeonia ostii by modulating lignin accumulation. Industrial Crops Products. 204:117228. doi:10.1016/j.indcrop.2023.117228.
- Luo X, Bai X, Sun X, Zhu D, Liu B, Ji W, Zhu Y. 2013. Expression of wild soybean WRKY20 in Arabidopsis enhances drought tolerance and regulates ABA signalling. J. Exp. Bot. 64:2155–2169. doi:10.1093/jxb/ert073.
- Marquez CP, Pritham EJ. 2010. Phantom, a new subclass of Mutator DNA transposons found in insect viruses and widely distributed in animals. Genetics. 185:1507–1517. doi:10.1534/genetics.110.116673.
- Miao Y, Laun T, Zimmermann P, Zentgraf U. 2004. Targets of the WRKY53 transcription factor and its role during leaf senescence in Arabidopsis. Plant Mol. Biol. 55:853–867. doi:10.1007/s11103-005-2142-1.
- Miao Y, Laun TM, Smykowski A, Zentgraf U. 2007. Arabidopsis MEKK1 can take a short cut: it can directly interact with senescence-related WRKY53 transcription factor on the protein level and can bind to its promoter. Plant Mol. Biol. 65:63–76. doi:10.1007/s11103-007-9198-z.
- Mohanta TK, Park Y-H, Bae H. 2016. Novel genomic and evolutionary insight of WRKY transcription factors in plant lineage. Sci. Rep. 6:1–22. doi:10.1038/srep37309.
- Moison M, Pacheco JM, Lucero L, Fonouni-Farde C, Rodrı́guez-Melo J, Mansilla N, et al. 2021. The lncRNA APOLO interacts with the transcription factor WRKY42 to trigger root hair cell expansion in response to cold. Mol. Plant. 14:937–948. doi:10.1016/j.molp.2021.03.008.
- Ohama N, Sato H, Shinozaki K, Yamaguchi-Shinozaki K. 2017. Transcriptional regulatory network of plant heat stress response. Trends Plant Sci. 22:53–65. doi:10.1016/j.tplants.2016.08.015.
- Phukan UJ, Jeena GS, Shukla RK. 2016. WRKY transcription factors: molecular regulation and stress responses in plants. Front. Plant Sci. 7:760. doi:10.3389/fpls.2016.00760.
- Qiu JL, Fiil BK, Petersen K, Nielsen HB, Botanga CJ, Thorgrimsen S, Palma K, Suarez-Rodriguez MC, Sandbech-Clausen S, Lichota J, et al. 2008. Arabidopsis MAP kinase 4 regulates gene expression through transcription factor release in the nucleus. EMBO J. 27:2214–2221. doi:10.1038/emboj.2008.147.
- Qiu YP, Yu DQ. 2009. Over-expression of the stress-induced OsWRKY45 enhances disease resistance and drought tolerance in Arabidopsis. Environ. Exp. Bot. 65:35–47. doi:10.1016/j.envexpbot.2008.07.002.
- Ren L, Wan W, Yin D, Deng X, Ma Z, Gao T, Cao X. 2022. Genome-wide analysis of WRKY transcription factor genes in Toona sinensis: an insight into evolutionary characteristics and terpene synthesis. Front. Plant Sci. 13:1063850. doi:10.3389/fpls.2022.1063850.
- Rushton PJ, Somssich IE, Ringler P, Shen QJ. 2010. WRKY transcription factors. Trends Plant Sci. 15:247–258. doi:10.1016/j.tplants.2010.02.006.
- Schluttenhofer C, Yuan L. 2015. Regulation of specialized metabolism by WRKY transcription factors. Plant Physiol. 167:295–306. doi:10.1104/pp.114.251769.
- Schmutz J, Cannon SB, Schlueter J, Ma J, Mitros T, Nelson W, Hyten DL, Song Q, Thelen JJ, Cheng J, et al. 2010. Genome sequence of the palaeopolyploid soybean. Nature. 463:178–183. doi:10.1038/nature08670.
- Shang Y, Yan L, Liu ZQ, Cao Z, Mei C, Xin Q, Wu FQ, Wang XF, Du SY, Jiang T, et al. 2010. The Mg-chelatase H subunit of Arabidopsis antagonizes a group of WRKY transcription repressors to relieve ABA-responsive genes of inhibition. Plant Cell. 22:1909–1935. doi:10.1105/tpc.110.073874.
- Shimono M, Sugano S, Nakayama A, Jiang CJ, Ono K, Toki S, Takatsuji H. 2007. Rice WRKY45 plays a crucial role in benzothiadiazole-inducible blast resistance. Plant Cell. 19:2064–2076. doi:10.1105/tpc.106.046250.
- Song Y, Chen LG, Zhang LP, Yu DQ. 2010b. Overexpression ofOsWRKY72 gene interferes in the abscisic acid signal and auxin transport pathway of Arabidopsis. J. Biosci. 35:459–471. doi:10.1007/s12038-010-0051-1.
- Su T, Xu Q, Zhang F-C, Chen Y, Li L-Q, Wu W-H, et al. 2015. WRKY42 modulates phosphate homeostasis through regulating phosphate translocation and acquisition in arabidopsis. Plant Physiol. 167:1579–1591. doi:10.1104/pp.114.253799.
- Sun Y, Yu D. 2015. Activated expression of AtWRKY53 negatively regulates drought tolerance by mediating stomatal movement. Plant Cell Rep. 34:1295–1306. doi:10.1007/s00299-015-1787-8.
- Tripathi P, Rabara RC, Rushton PJ. 2014. A systems biology perspective on the role of WRKY transcription factors in drought responses in plants. Planta. 239:255–266. doi:10.1007/s00425-013-1985-y.
- Turan S, Cornish K, Kumar S. 2012. Salinity tolerance in plants: breeding and genetic engineering. Aust. J. Crop Sci. 6(9):1337–1348.
- Turck F, Zhou A, Somssich IE. 2004. Stimulus-dependent, promoter-specific binding of transcription factor WRKY1 to its native promoter and the defense-related gene PcPR1-1 in Parsley. Plant Cell. 16:2573–2585. doi:10.1105/tpc.104.024810.
- Verma KK, Liu X-H, Wu K-C, Singh RK, Song QQ, Malviya MK, Song X-P, Singh P, Verma CL, Li YR. 2020a. The impact of silicon on photosynthetic and biochemical responses of sugarcane under different soil moisture levels. Silicon. 12:1355–1367. doi:10.1007/s12633-019-00228-z.
- Verma KK, Singh P, Song X-P, Malviya MK, Singh RK, Chen G-L, Solomon S, Li YR. 2020b. Mitigating climate change for sugarcane improvement: role of silicon in alleviating abiotic stresses. Sugar Tech. 22(5):741–749. doi:10.1007/s12355-020-00831-0.
- Verma KK, Song XP, Budeguer F, Nikpay A, Enrique R, Singh M, Zhang B, Wu JM, Li YR. 2022b. Genetic engineering: an efficient approach to mitigating biotic and abiotic stresses in sugarcane cultivation. Plant Signal Behav. 17(1):2108253. doi:10.1080/15592324.2022.2108253.
- Verma KK, Song XP, Lin B, Guo DJ, Singh M, Rajput VD, Singh RK, Singh P, Sharma A, Malviya MK, et al. 2021. Silicon induced drought tolerance in crop plants: physiological adaptation strategies. Silicon. 14:2473–2487. doi:10.1007/s12633-021-01071-x.
- Verma KK, Song XP, Yadav G, Degu HD, Parvaiz A, Singh M, Huang HR, Mustafa G, Xu L, Li YR. 2022a. Impact of agroclimatic variables on proteo-genomics in sugarcane (Saccharum spp.) plant productivity. ACS Omega. 7:22997–23008. doi:10.1021/acsomega.2c01395.
- Wang C, Deng P, Chen L, Wang X, Ma H, Hu W, Yao N, Feng Y, Chai R, Yang G, He G. 2013. A wheat WRKY transcription factor TaWRKY10 confers tolerance to multiple abiotic stresses in transgenic tobacco. PLoS One. 8(6):e65120. doi:10.1371/journal.pone.0065120.
- Wang C, Ye D, Li Y, Hu P, Xu R, Wang X. 2023a. Genome-wide identification and bioinformatics analysis of the WRKY transcription factors and screening of candidate genes for anthocyanin biosynthesis in azalea (Rhododendron simsii). Front. Genetics. 14:1172321. doi:10.3389/fgene.2023.1172321.
- Wang F, Hou X, Tang J, Wang Z, Wang S, Jiang F, Li Y. 2012. A novel cold-inducible gene from Pak-choi (Brassica campestriss sp. chinensis), BcWRKY46, enhances the cold, salt and dehydration stress tolerance in transgenic tobacco. Mol. Biol. Rep. 39:4553–4564. doi:10.1007/s11033-011-1245-9.
- Wang H, Cheng X, Yin D, Chen D, Luo C, Liu H, Huang C. 2023. Advances in the research on plant WRKY transcription factors responsive to external stresses. Mol. Biol. 45:2861–2880. doi:10.3390/cimb45040187.
- Wang H, Hao J, Chen X, Hao Z, Wang X, Lou Y, Peng Y, Guo Z. 2007. Overexpression of rice WRKY89 enhances ultraviolet B tolerance and disease resistance in rice plants. Plant Mol. Biol. 65:799–815. doi:10.1007/s11103-007-9244-x.
- Wang H, Xu Q, Kong YH, Chen Y, Duan JY, Wu WH, Chen YF. 2014a. Arabidopsis WRKY45 transcription factor activates PHOSPHATE TRANSPORTER1;1 expression in response to phosphate starvation. Plant Physiol 164:2020–2029. doi:10.1104/pp.113.235077.
- Wang J, Tao F, An F, Zou Y, Tian W, Chen X, Xu X, Hu X. 2017. Wheat transcription factor TaWRKY70 is positively involved in high-temperature seedling plant resistance to Puccinia striiformi sf. sp. tritici. Mol. Plant Pathol. 18:649–661. doi:10.1111/mpp.12425.
- Wang M, Vannozzi A, Wang G, Liang YH, Tornielli GB, Zenoni S, Cavallini E, Pezzotti M, Cheng ZM. 2014b. Genome and transcriptome analysis of the grapevine (Vitis vinifera L.) WRKY gene family. Hort. Res. 1:14016. doi:10.1038/hortres.2014.16.
- Wang W, Xin H, Wang M, Ma Q, Wang L, Kaleri NA, Wang Y, Li X. 2016. Transcriptomic analysis reveals the molecular mechanisms of drought-stress-induced decreases in Camellia sinensis leaf quality. Front. Plant Sci. 7:786. doi:10.3389/fpls.2016.00385.
- Wang X, Zeng J, Li Y, Rong X, Sun J, Sun T, Li M, Wang L, Feng Y, Chai R, et al. 2015. Expression of TaWRKY44, a wheat WRKY gene, in transgenic tobacco confers multiple abiotic stress tolerances. Front. Plant Sci. 6:615. doi:10.3389/fpls.2015.00615.
- Wang Z, Zhu Y, Wang L, Liu X, Liu Y, Phillips J, Deng X. 2009. A WRKY transcription factor participates in dehydration tolerance in Boea hygrometrica by binding to the W-box elements of the galactinol synthase (BhGolS1) promoter. Planta. 230:1155–1166. doi:10.1007/s00425-009-1014-3.
- Wei S, Ma X, Pan L, Miao J, Fu J, Bai L, Zhang Z, Guan Y, Mo C, Huang H, Chen M. 2017. Transcriptome analysis of Taxillusi chinensis (DC.) danser seeds in response to water loss. PLoS One. 12(1):e0169177. doi:10.1371/journal.pone.0169177.
- Wu X, Shiroto Y, Kishitani S, Ito Y, Toriyama K. 2009. Enhanced heat and drought tolerance in transgenic rice seedlings overexpressing OsWRKY11 under the control of HSP101 promoter. Plant Cell Rep. 28:21–30. doi:10.1007/s00299-008-0614-x.
- Xiang X-Y, Chen J, Xu W-X, Qiu J-R, Song L, Wang J-T, Tang R, Chen D, Jiang C-Z, Huang Z. 2021. Dehydration-induced WRKY transcriptional factor MfWRKY70 of myrothamnus flabellifolia enhanced drought and salinity tolerance in arabidopsis. Biomolecules. 11(2):327. doi:10.3390/biom11020327.
- Xie Z, Zhang ZL, Zou X, Huang J, Ruas P, Thompson D, Shen QJ. 2005. Annotations and functional analyses of the rice WRKY gene superfamily reveal positive and negative regulators of abscisic acid signaling in aleurone cells. Plant Physiol. 137:176–189. doi:10.1104/pp.104.054312.
- Xing B, Wan S, Su L, Riaz MW, Li L, Ju Y, Zhang W, Zheng Y, Shao Q. 2023. Two polyamines -responsive WRKY transcription factors from Anoectochilus roxburghii play opposite functions on flower development. Plant Sci. 327:111566. doi:10.1016/j.plantsci.2022.111566.
- Xiong W, Xu X, Zhang L, Wu P, Chen Y, Li M, Jiang H, Wu G. 2013. Genome-wide analysis of the WRKY gene family in physic nut (Jatropha curcas L.). Gene. 524:124–132. doi:10.1016/j.gene.2013.04.047.
- Xu X, Chen C, Fan B, Chen Z. 2006. Physical and functional interactions between pathogen-induced Arabidopsis WRKY18, WRKY40, and WRKY60 transcription factors. Plant Cell. 18:1310–1326. doi:10.1105/tpc.105.037523.
- Yamasaki K, Kigawa T, Inoue M, Tateno M, Yamasaki T, Yabuki T, Aoki M, Seki E, Matsuda T, Tomo Y, et al. 2005. Solution structure of an Arabidopsis WRKY DNA binding domain. Plant Cell. 17:944–956. doi:10.1105/tpc.104.026435.
- Yan H, Jia H, Chen X, Hao L, An H, Guo X. 2014. The cotton WRKY transcription factor GhWRKY17 functions in drought and salt stress in transgenic Nicotiana benthamiana through ABA signaling and the modulation of reactive oxygen species production. Plant Cell Physiol. 55:2060–2076. doi:10.1093/pcp/pcu133.
- Yang Z, Chi X, Guo F, Jin X, Luo H, Hawar A, Chen Y, Feng K, Wang B, Qi J, et al. 2020. SbWRKY30 enhances the drought tolerance of plants and regulates a drought stress-responsive gene, SbRD19, in sorghum. J. Plant Physiol. 246–247:153142. doi: 10.1016/j.jplph.2020.153142.
- Zentgraf U, Laun T, Miao Y. 2010. The complex regulation of WRKY53 during leaf senescence of Arabidopsis thaliana. Eur. J. Cell Biol. 89:133–137. doi:10.1016/j.ejcb.2009.10.014.
- Zhang H, Li D, Wang M, Liu J, Teng W, Cheng B, Huang Q, Wang M, Song W, Dong S, et al. 2012. The Nicotiana benthamiana mitogen-activated protein kinase cascade and WRKY transcription factor participate in Nep1(Mo)-triggered plant responses. Mol. Plant Microbe Int. 25:1639–1653. doi:10.1094/MPMI-11-11-0293.
- Zhang M, Liu Y, Cai H, Guo M, Chai M, She Z, Ye L, Cheng Y, Wang B, Qin Y. 2020. The bZIP transcription factor GmbZIP15 negatively regulates salt-and drought-stress responses in soybean. Int. J. Mol. Sci. 21(20):7778. doi:10.3390/ijms21207778.
- Zhang Y, Wang L. 2005. The WRKY transcription factor superfamily: Its origin in eukaryotes and expansion in plants. BMC Evol. Biol. 5:1. doi:10.1186/1471-2148-5-1.
- Zhang Y, Yu H, Yang X, Li Q, Ling J, Wang H, Gu X, Huang S, Jiang W. 2016b. CsWRKY46, a WRKY transcription factor from cucumber, confers cold resistance in transgenic-plant by regulating a set of cold-stress responsive genes in an ABA-dependent manner. Plant Physiol. Biochem. 108:478–487. doi:10.1016/j.plaphy.2016.08.013.
- Zhao CJ, Hao ZN, Wang HH, Chen XJ, Guo ZJ. 2010. Identification of a novel regulatory element region responded to UV-B in rice WRKY89 promoter. Prog. Biochem. Biophys. 37:671–677. doi:10.3724/SP.J.1206.2009.00666.
- Zhou QY, Tian AG, Zou HF, Xie ZM, Lei G, Huang J, Chen SY. 2008. Soybean WRKY-type transcription factor genes, GmWRKY13, GmWRKY21, and GmWRKY54, confer differential tolerance to abiotic stresses in transgenic Arabidopsis plants. Plant Biotechnol. J. 6:486–503. doi:10.1111/j.1467-7652.2008.00336.x.
- Zhu D, Hou L, Xiao P, Guo Y, Deyholos MK, Liu X. 2019. VvWRKY30, a grape WRKY transcription factor, plays a positive regulatory role under salinity stress. Plant Sci. 280:132–142. doi:10.1016/j.plantsci.2018.03.018.
- Zhu H, Jiang Y, Guo Y, Huang J, Zhou M, Tang Y, Sui J, Wang J, Qiao L. 2021. A novel salt inducible WRKY transcription factor gene, AhWRKY75, confers salt tolerance in transgenic peanut. Plant Physiol. Biochem. 160:175–183. doi:10.1016/j.plaphy.2021.01.014.
- Zou C, Jiang W, Yu D. 2010. Male gametophyte-specific WRKY34 transcription factor mediates cold sensitivity of mature pollen in Arabidopsis. J. Exp. Bot. 61:3901–3914. doi:10.1093/jxb/erq204.
- Zou X, Seemann JR, Neuman D, Shen QJ. 2004. A WRKY gene from creosote bush encodes an activator of the abscisic acid signaling pathway. J. Biol. Chem. 279:55770–55779. doi:10.1074/jbc.M408536200.