ABSTRACT
Introduction: Deep transcranial magnetic stimulation (dTMS) utilizes different H-coils to study and treat a variety of psychiatric and neurological conditions with identifiable brain targets. The availability of this technology is dramatically changing the practice of psychiatry and neurology as it provides a safe and effective way to treat even drug-resistant patients. However, up until now, no effort was made to summarize the different types of H-coils that are available, and the conditions for which they were tested.
Areas covered: Here we assembled all peer reviewed publication that used one of the H-coils, together with illustrations of the effective field they generate within the brain. Currently, the technology has FDA clearance for depression and European clearance for additional disorders, and multi-center trials are exploring its safety and effectiveness for OCD, PTSD, bipolar depression and nicotine addiction.
Expert commentary: Taken together with positive results in smaller scale experiments, dTMS coils represent a non-invasive way to manipulate pathological activity in different brain structures and circuits. Advances in stimulation and imaging methods can now lead to efficacious and logical treatments. This should reduce the stigma associated with mental disorders, and improve access to psychiatric treatment.
1. Introduction
Over the past half century, advances in the field of psychiatry increased the physician’s toolbox exponentially. New classes of pharmacological drugs were developed, electroconvulsive therapy (ECT) protocols were optimized [Citation1], and focal brain stimulation techniques were invented [Citation2]. Most importantly, many psychiatric symptoms have now identifiable functional alterations in specific brain regions and circuits due to advances in functional imaging [Citation3,Citation4]. Methods, such as MRI, fMRI, positron emission tomography, and single-photon emission computed tomography relate specific disorders and symptoms to specific brain areas with altered activity or volume [Citation5]. This allows techniques such as deep brain stimulation (DBS) or transcranial magnetic stimulation (TMS) to target specific networks that are known for their affected activity in a specific disorder.
One condition for which significant progress has been made is major depression disorder (MDD). MDD is a commonly occurring disorder, and with a lifetime prevalence of 17%, it ranks as the second leading cause of disability according to World Health Organization (WHO) [Citation6]. Patients diagnosed with MDD show dysregulation in several brain regions, including hypometabolism in regions, such as the dorsolateral prefrontal cortex (DLPFC) and the cingulate cortex (ACC), and abnormal overactivity in the amygdala, basal ganglia, and thalamus [Citation7]. Despite the availability of psychotherapy and over four classes and 30 distinct pharmacologic agents, a large portion (32–52%) of the MDD population are still considered treatment resistant [Citation8,Citation9]. Taken together with MDDs relapsing nature and the tolerance that may develop to formerly successful pharmacotherapy [Citation10], non-drug strategies are required for the management of this disorder. Over the years, different methods have been developed for that purpose but, side by side with their unique advantages, all methods also have their own drawbacks [Citation11]. Among the available brain stimulation technologies, ECT is still considered to be the most effective method, with over 70% remission rate [Citation12,Citation13]. Yet, the procedure is associated with side effects and continues to be the most stigmatized treatment available in psychiatry, a fact that hinders people from pursuing it [Citation1]. Thus, ECT is considered to be the ‘last resort’ and is used only for severely drug-resistant patients [Citation14]. DBS has also been shown to alleviate depressive symptoms in drug-resistant MDD patients [Citation15–Citation21]. However, DBS also has important drawbacks, as it involves a complex surgical intervention (i.e. drilling in the skull with the risk of infection), requires replacing the subcutaneous battery periodically, and has a high overall cost [Citation22]. The optimal targets for DBS are still not known, and although the background suicide rate in this population is elevated there is controversy about DBS causing suicidal ideations [Citation7,Citation23–Citation27]. Conventional TMS, being a non-invasive technique, does not have the above disadvantages and has been shown to effectively treat MDD in moderately drug-resistant patients [Citation28–Citation30]. However, conventional TMS only allows stimulation of focal targets just below the skull and, without navigation, conventional repetitive TMS (rTMS) misses the target in 27–32% of patients [Citation31,Citation32] (See Box 1).
In an attempt to address these limitations, deep TMS (dTMS) was introduced [Citation33,Citation34]. dTMS includes all the benefits of TMS (no need for hospitalization or anesthesia and negligible side effects [Citation11]) with the advantage of stimulating deeper brain targets with less focal distribution of the electric field. Similar to the conventional TMS technique, dTMS utilizes brief magnetic pulses to induce targeted neuronal depolarization in the brain using Faraday’s law of electromagnetic induction [Citation35]. Usually, trains of pulses delivered at high frequency stimulation (>5 Hz) lead to a facilitatory effect inducing increased neuronal excitability (long-term potentiation; LTP-like effect) while low frequency (~1 Hz) induces reduction in neuronal excitability (long-term depression; LTD-like effect) [Citation36,Citation37]; thus, hypo- or hyper-activity of specific brain areas can be modulated. However, conversely to conventional TMS coils (e.g. figure-of-8 or round), which directly stimulate targets up to ~1 cm beneath the surface of the skull, dTMS can simulate up to ~4 cm beneath the surface of the skull, depending on the specific H-coil being used [Citation38]. This increased stimulation depth is achieved due to the multiple windings in multiple planes inside the H-coil helmet. The magnetic fields of these windings sum together and improve the depth penetration of the electromagnetic field without the need for increased electric intensity. Although deep stimulation can also be accomplished with a large circular coil or a double cone coil, their electromagnetic field decays more rapidly and to reach significantly deep targets much higher intensities must be used on the surface [Citation36,Citation39]; intensities that can be unpleasant and potentially unsafe for the patient. Finally, the H-coils stimulate larger areas, i.e. ~17 cm3 of the brain tissue with the H1 compared to ~3 cm3 with conventional figure-8 coils, when both are operated at 120% of motor threshold (MT). This volume difference represents hundreds of millions of neurons and many more pathways, eliminates the need for imaging and neuronavigation and making the treatment less costly.
Box 1. Alternative non-pharmacological somatic treatments
Electroconvulsive therapy (ECT): The first device-based treatment to become available for psychiatric disorders. During treatment, electric current passes through electrodes placed at precise locations on the head, causing a seizure that lasts generally less than 1 min.
Magnetic seizure therapy (MST): Similar to ECT it induces a seizure, but conversely to ECT it uses magnetic pulses instead of electricity to stimulate the brain. MST appears to have less cognitive side effects than ECT.
Deep brain stimulation (DBS): A generator implanted in the chest control electrodes implanted in a specific brain area. Stimulation is continuous and its frequency and intensity can be adjusted to the individual. The targets for OCD and Parkinson’s are known, for depression they are still controversial and under investigation.
Vagus nerve stimulation (VNS): A generator implanted in the chest sends electrical pulses to the left vagus nerve. VNS was approved in 2006 for depression but is not covered by insurance due to low efficacy rates. It is covered for the treatment of refractory epilepsy.
Conventional or superficial TMS: Conventional TMS coils induces a very focal effective electric field up to 2 cm from the coil. Besides treatment, conventional TMS with neuronavigation is useful in single pulses for presurgical brain mapping.
Transcranial electrical stimulation (tES): Encompasses all forms of non-invasive application of electrical currents to the brain using electrodes on the head. tES includes, among others, cranial electrotherapy stimulation (CES) and transcranial direct current stimulation (tDCS), which induce subthreshold electric fields in the brain. tES may be helpful for non-treatment resistant patients.
1.1. dTMS in the clinic
In the United States, dTMS using the H1-coil is cleared for the treatment of unipolar major depression that has failed any number of medications. In Europe, dTMS using the H1-coil is cleared for the treatment unipolar depression, bipolar depression, negative symptoms of schizophrenia, and post-traumatic stress disorder (PTSD), while several other H-coil versions designed to target other brain networks are cleared for the treatment of Alzheimer’s disease, chronic pain, smoking cessation, obsessive compulsive disorder (OCD), autism, Parkinson’s disease, stroke rehabilitation, and multiple sclerosis (MS).
1.2. Clinical considerations
In the United States, anyone can purchase a dTMS device but it can only be used under the prescription of a physician (usually a psychiatrist or a neurologist). At the present time about 90% of all dTMS is done for the treatment of medication refractory unipolar major depressive disorder (treatment resistant depression; TRD). However, one may also treat patients for off label conditions. The assessing physician’s responsibility is to search current literature to determine if there are any new protocols for treatment of the relevant disorder, or for the treatment of comorbid conditions (e.g. depression and MS fatigue, depression and PTSD, and depression and pain).
Prior to administering dTMS to a patient, informed consent must be obtained from the patient or their legal guardian. In addition to explaining the procedure, the process includes an explanation of the risks, benefits, and alternatives. The risks or potential side effects of dTMS include headaches, facial pain, tooth pain, or neck pain usually just during the procedure but it may also occur between the procedures as well. Patients can premedicate with an analgesic, and the pain typically decreases on sequential treatments. If ear plugs are not worn or if they fall out, the repetitive loud noise from dTMS can cause permanent hearing loss or tinnitus. In rare cases, patients receiving dTMS can have generalized neuronal excitation beyond the site of stimulation resulting in a seizure. The risks of a seizure occurring are highest in patients with preexisting epilepsy, changing medication dosage without rechecking the motor threshold, alcohol intake or sleep deprivation. Seizures from dTMS have always stopped on their own and only occurred during the treatment, dTMS does not cause epilepsy. Alternatives to dTMS in the case of TRD include ECT or combinations of medications.
1.3. Treatment protocol
dTMS practice protocols are similar to the ones that are used with conventional TMS. In the case of MDD, patients are scheduled for a diagnostic evaluation with a psychiatrist that reviews their medical history, documents the current symptoms of depression, prior treatment trials, and recommends a course of dTMS, which would entail an acute course of dTMS treatments administered five days a week. Each treatment session includes 55 trains of 36 pulses (for a total of 1980 pulses per session) delivered over 20 min at 18 Hz and intensity of 120% relative to the patient’s resting motor threshold (MT) [Citation40]. The individual MT is re-measured at least once a week or when a medication is changed, by determining the minimal intensity for which a single pulse activates the hand motor cortex in 5/10 trials (evident as a movement of the limb or with electromyography). In many cases, even patients that do not improve after 30–40 sessions may reach response (50% improvement) with additional dTMS sessions [Citation40]. Alternatively, the physician can consider increasing the number of pulses. High rates of remission and response were found in severe TRD patients who were treated with 3000 dTMS pulses per session [Citation41] and 4000–6000 pulses were effective for patients with schizoaffective depressive phase and patients who failed ECT [Citation42,Citation43] . When the patient goes into remission or has a sustained improvement, the patient should continue treatment twice a week for a period of three months. Once the patient goes into remission from dTMS, they are considered future first-line dTMS patients. When these patients relapse or have recurrence of their depression, they should be immediately retreated with dTMS instead of trying a new medication. In addition, if they have several relapses a year, maintenance dTMS may be a preferred treatment. Maintenance dTMS is done once a week, after a period of 3 months of stability on twice a week treatments.
1.4. Technical considerations
The dTMS device is comprised of a rolling cart, which contains a stimulator and an air-cooling system for the coil, and a positioning arm attached to the side of the cart holding the H-coil inside of a cushioned helmet () The device can fit in the corner of a room, and any chair can be used for treatments; thus, any room can be used as a dTMS treatment room. The machine requires two NEMA 5–20 A outlets on different fuses and one 15 A outlet to plug the machine into, which almost any office room has. When the machine is used all day, the room it is in will become significantly warmer than the rest of the office and may require its own air-conditioning zone. Alternatively, the back of the cooling unit can be vented out of the treatment room.
Figure 1. dTMS system on a rolling medical cart including a cooling system, stimulator, interface module for double blind experiments, positioning arm and one helmet.
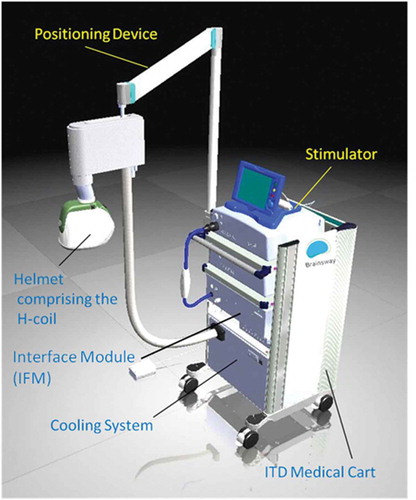
Every cart can hold several positioning arms and hence several different H-coils that target different brain areas for different indications. Every helmet can technically hold several H-coils, and during clinical trials every helmet has a corresponding sham H-coil encased in the same helmet. The sham coil induces only a negligible sub-threshold field in the brain while making an identical noise and inducing some scalp sensation [Citation44]. The research version of the dTMS device has an electronic card reader and interface module that operate on each patient’s treatment card determining whether the sham or active coil in the helmet should be used for that specific treatment. The operator uses an operator card to find the MT, and then moves the helmet to the treatment position. At this point, a randomized patient card is used to administer repetitive pulses. This maintains the double blind condition necessary for research trials, where both the operator and the patient can not tell whether an active or sham treatment is delivered (as the two coils sound and feel similar to the operator who may be holding the helmet from the outside).
1.5. The induced effects of dTMS
The H1-coil that is used for the treatment of MDD was designed to induce activation of left and right lateral and medial prefrontal cortex structures, with a preference to the left hemisphere (). The safety and efficacy of the H1-coil in MDD were demonstrated in several clinical trials (as detailed in ), including a large prospective multicenter study [Citation40], which formed the basis for US FDA approval for the treatment of MDD in the United States. The safety and efficacy of the H1-coil was also demonstrated in bipolar disorder [Citation45], negative symptoms of schizophrenia [Citation46,Citation47], and using a more medial location also in PTSD [Citation48]. Preliminary studies showed effectiveness for the H1-coil also in alcoholism (and associated dysthymia) [Citation49–Citation51] and even in migraine [Citation52] (). Several other H-coils were developed in order to affect different brain networks and hence treat different conditions (). To create , all of the peer-reviewed literature with the term deep transcranial magnetic stimulation was reviewed by each the authors using PubMed with and without a clinical trial filter. Centers that have dTMS coils are surveyed by the manufacturer for peer-reviewed publications that may not have been indexed in Pubmed as well as ongoing clinical research activity, and this data was reviewed as well. Finally, all of the ongoing single and multisite clinical trials were added. The publications were discussed by the authors as a group, and the coils were listed with corresponding disorders with the level of evidence stated explicitly in the table.
Table 1. Specifications of the different H-coils.
The effects of the H-coils are best understood through electrical field diagrams (–Citation15). These diagrams are generated by placing each coil over a plastic head model filled with saline solution and measuring the induced fields at every point with an oscilloscope. In the diagrams, red pixels indicate regions where the induced field intensity is above the threshold for neural activation (100 V/m). The maps for each coil are generated while the coil is located above the specific treatment location and the intensity is set per the relevant recommended treatment protocol. As can be observed, there is overlap in the areas that coils stimulate, and coils can be moved from their intended-designed position to another location to achieve a similar effect as another coil (i.e. moving the H7 coil from the PFC-ACC posteriorly to the motor cortex will result in a coil that effectively stimulates both lower extremities).
Figure 2. Colored field maps for the H1-coil indicating the absolute magnitude electrical field in each pixel at 120% of hand motor threshold, for 10 coronal slices 1 cm apart. Red pixels indicate regions with field intensity above the threshold for neuronal activation. The figure is in-press and is open access [Citation79]. Full color available online.
![Figure 2. Colored field maps for the H1-coil indicating the absolute magnitude electrical field in each pixel at 120% of hand motor threshold, for 10 coronal slices 1 cm apart. Red pixels indicate regions with field intensity above the threshold for neuronal activation. The figure is in-press and is open access [Citation79]. Full color available online.](/cms/asset/e905d552-89fd-4e76-a711-eb244d775b5c/ierd_a_1233812_f0002_oc.jpg)
1.6. dTMS operators
The field of TMS technicians is just evolving, and it will continue to evolve as the devices and indications evolve. Currently, most dTMS operators have a bachelor’s degree and an interest in neuroscience or patient care. Operators have much more frequent contact with patients than receptionists and are similar to behavioral health technicians or nurses. The manufacturer trains and certifies operators on the device. Operators should be trained in first aid and CPR; and must be comfortable recognizing and making initial steps to deal with a seizure, because this is the only significant safety risk with dTMS, even if a very rare one [Citation80]. In addition, as they interact with depressive patients, they should be prepared for the possibility of their patients attempting suicide. This should be discussed with the operators when they are treating patients with prior suicide attempts. Operators should be observant, comforting and not alarming to the patients. Operators are also responsible for administering specific rating scales to patients and keeping accurate records of patient treatments. Finally, some off-label dTMS protocols require an intimate knowledge of patient symptoms, because the patient’s symptoms need to be provoked before the treatment (OCD, PTSD, addictions) [Citation44,Citation48,Citation72]
1.7. Financial considerations
Almost all of the TMS centers in the United States today are part of psychiatric offices, and each dTMS system can easily treat two patients per hour. Typically, one operator treats one patient at a time on one machine per room. The income from dTMS comes from the procedural fee or, in the case of a facility, from the facility and procedural fee. There are three TMS CPT codes, which are widely covered by insurance across the country for MDD patients: 90867, 90868, and 90869. Therapeutic rTMS treatment; initial, including cortical mapping, motor threshold determination, delivery and management (90867) is reported only once per treatment course. Subsequent delivery and management (90868) is reported 20–40 times per treatment course series. Subsequent motor threshold re-determination with delivery and management (90869) is reported no more frequently than once a week or when medication dosages are altered [Citation80].
Medicare and some commercial insurers have no preauthorization process and the patient can start treatment immediately. However, several commercial insurance companies require a preauthorization process at which time they approve an initial course of acute treatments with the expectation that periodic rating scales will be completed by the patient and an assessment will be done by the physician at baseline and follow up.
The patients usually come from the clinic’s existing patient population, after having failed or been intolerant to one or more antidepressant medications (because that is what commercial insurance and medicare covers). However, as dTMS devices are not that costly, it is entirely possible to have a self-pay dTMS clinic. The dTMS system costs are generally fixed. This includes the device, a maintenance plan, insurance on the device and the practice, rent, marketing fees and salary for an operator. The variable costs are relatively nominal and they include earplugs and soft caps for the patients and electricity. The dTMS system is currently available in any of three formats: purchase the system with no per use charges with a 1-year warrantee that can be extended every year for a fee. Alternatively, it is available for a 4-year lease with no per use charges with the maintenance fee included. Finally, a hesitant physician can enter a risk sharing model, where the physician pays per use charges and there is no maintenance fee.
1.8. Legal considerations
All of the patient information is available in the patient manual, and should be made available to patients, similar to the package insert that comes along with a medication. Legally this is sufficient and the FDA does not require a specific written consent for TMS. However, most US TMS centers develop brief consent forms that specify rTMS is currently only approved for resistant unipolar MDD, but used for many other conditions on an off label basis. It outlines the potential risk of a temporary seizure, reminds to use hearing protection at peril of permanent hearing loss, mentions temporary head/face/tooth/neck pain and availability of analgesic prophylactics, discusses alternative treatments, such as ECT or polypharmacy, and the fact rTMS has only been studied in humans since the 1990s, and there may be adverse long-term effects not yet known. Some consent forms also inform patients that their treatment data is kept in a separate database in an anonymous fashion for outcomes research.
2. Conclusion
Mental illnesses carry a stigma that affects patients, family members and even psychiatrists. This discrediting is present in every country, and it has not disappeared despite tremendous advances in the field of psychiatry over the last half century. One potential reason is that brain abnormalities are hidden from the naked eye, and another is that these chronic and complex conditions significantly affect behavior. Taken together with the fact that psychopharmacologic interventions do not have very clear and logical explanations for their mechanism of effect, the result is that patients are often blamed for their illness, which rarely occurs in general medical conditions [Citation81–Citation84]. However, this trend is slowly shifting its direction. Depression is much more accepted in the general population, evident by the growing number of people seeking treatment for this condition; Drug and alcohol addictions are gradually recognized as brain diseases by law-enforcement; and conditions such as ADHD or autism are diagnosed in progressively increasing rates. This shift is related to advances in functional imaging and focal brain stimulation that allows the identification and modification of altered activity in specific brain areas for specific psychiatric conditions and symptoms. This is bridging the theoretical gap between diseases of the mind-psychiatric illness, and diseases of the brain-neurological illnesses for both patients, caregivers, and the general population [Citation85,Citation86]. Thus, advances in bioengineering that enabled non-invasive modulation of altered brain activity (such as TMS and dTMS), making efficacious and logical treatments widely available to psychiatric patients, are reducing the stigma of mental illness as a whole. As a consequence, more people are now searching for treatments for their conditions, and a broader spectrum of alternatives is available to meet the specific needs of the individual.
3. Expert commentary
The most comparable technology to compare dTMS to is conventional TMS with the figure-8-shaped coils. They are both outpatient procedures with no significant adverse event profiles, only local discomfort and rare incidence of seizure. Unfortunately, there has been no head to head study comparing the two technologies. The advantages of dTMS to conventional TMS are theoretical, clinical, and practical. The theoretical advantages of dTMS are derived from the ability of the H-coil to stimulate much larger and deeper volumes of neuronal tissue. The difference is notable whether one uses mathematical modeling in spherical model of the head [Citation87,Citation88], mathematical modeling in an anatomically correct model of the head [Citation89–Citation91], or measures the depth of stimulation in saline filled head [Citation39,Citation92,Citation93]. Clinicians are aiming at a target that they cannot see without concomitant functional imaging and neuronavigation, a shotgun is likely to have greater efficacy than a rifle. The clinical advantages of dTMS are derived from comparing the results of the multisite double blinded randomized controlled trials leading to FDA clearance of the devices. The standardized effect size after 4 weeks for the H1 was 0.76, and for the figure-8 coil, it was 0.36–0.56 depending on the scale. After 4 weeks, the odds ratio for remission with the H1 was 2.83 compared to 1.1–1.16 with the [Citation40,Citation94]. The practical advantages of dTMS mainly relate to the time of treatment. The dTMS system has an active air cooling system which allows the operator to complete treatments in 20 min. It is easy to run the system continuously all day while treating two patients per hour. The motor threshold is easy to find, because of the large area of stimulation of the coil. Compared to a figure-8 coil, the shortest time of stimulation is 37.5 min, and it takes a long time to map the motor threshold. A busy office can use one dTMS system and one operator to replace two figure-8 systems and operators. Although there have not been any head to head studies yet, there are two published case series from unbiased sources demonstrating better clinical outcomes for the patients they treated with dTMS [Citation95,Citation96].
Figure 3. Colored field maps for the H2-coil indicating the absolute magnitude electrical field in each pixel at 120% of hand motor threshold, for 16 coronal slices 1 cm apart. Red pixels indicate regions with field intensity above the threshold for neuronal activation. Full color available online.
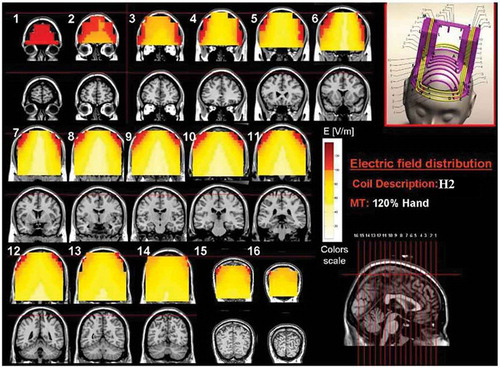
Figure 4. Colored field maps for the H3-coil indicating the absolute magnitude electrical field in each pixel at 120% of foot motor threshold, for 9 coronal slices 1 cm apart. Red pixels indicate regions with field intensity above the threshold for neuronal activation. Full color available online.
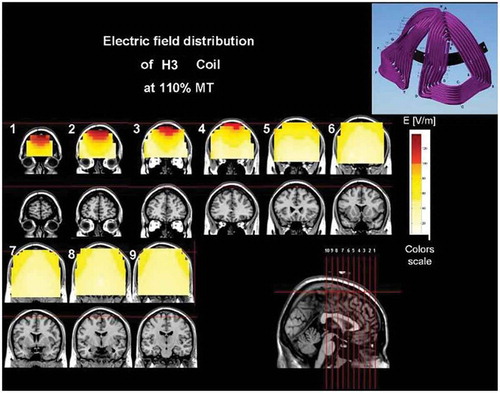
Figure 5. Colored field maps for the H4-coil indicating the absolute magnitude electrical field in each pixel at 120% of hand motor threshold, for 14 coronal slices 1 cm apart. Red pixels indicate regions with field intensity above the threshold for neuronal activation. Full color available online.
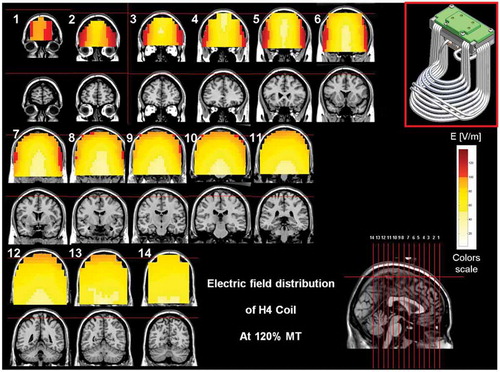
Figure 6. Colored field maps for the H5-coil indicating the absolute magnitude electrical field in each pixel at 120% of hand motor threshold, for 14 coronal slices 1 cm apart. Red pixels indicate regions with field intensity above the threshold for neuronal activation. Full color available online.
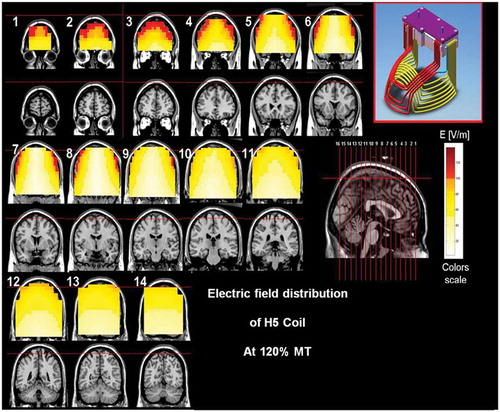
Figure 7. Colored field maps for the H6-coil indicating the absolute magnitude electrical field in each pixel at 120% of hand motor threshold, for 11 coronal slices 1 cm apart. Red pixels indicate regions with field intensity above the threshold for neuronal activation. Full color available online.
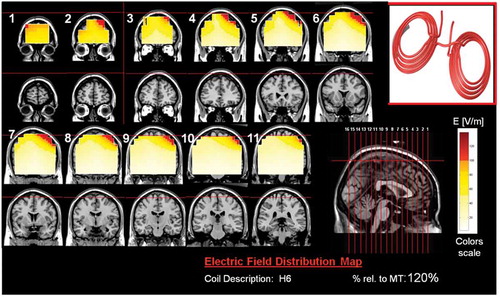
Figure 8. Colored field maps for the H7-coil indicating the absolute magnitude electrical field in each pixel at 100% of foot motor threshold, for 14 coronal slices 1 cm apart. Red pixels indicate regions with field intensity above the threshold for neuronal activation. Full color available online.
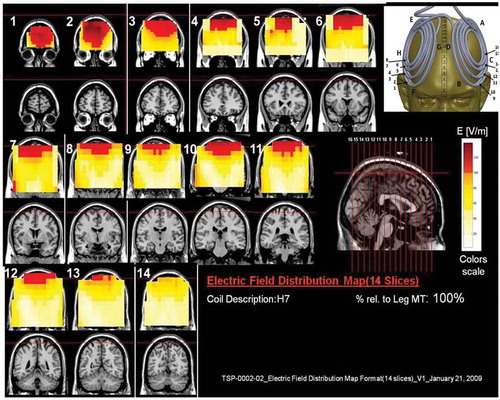
Figure 9. Colored field maps for the H8-coil indicating the absolute magnitude electrical field in each pixel at 120% of hand motor threshold, for 14 coronal slices 1 cm apart. Red pixels indicate regions with field intensity above the threshold for neuronal activation. Full color available online.
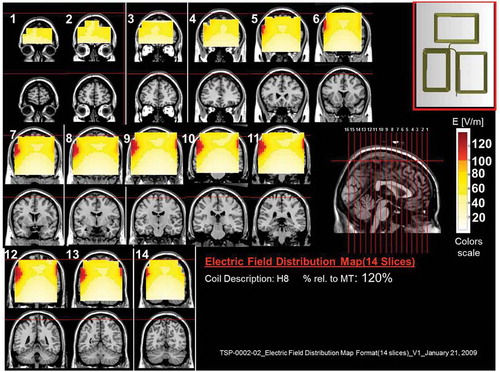
Figure 10. Colored field maps for the H9-coil indicating the absolute magnitude electrical field in each pixel at 110% of foot motor threshold, for 16 coronal slices 1 cm apart. Red pixels indicate regions with field intensity above the threshold for neuronal activation. Full color available online.
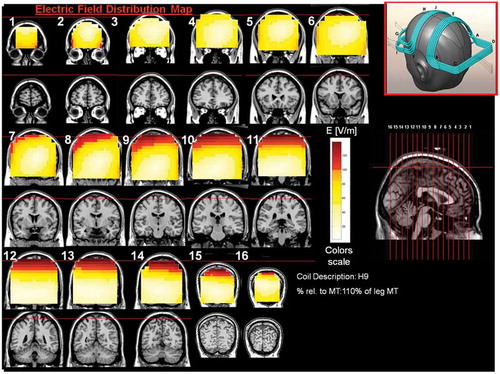
Figure 11. Colored field maps for the H10-coil indicating the absolute magnitude electrical field in each pixel at 100% of foot motor threshold, for 14 coronal slices 1 cm apart. Red pixels indicate regions with field intensity above the threshold for neuronal activation. Full color available online.
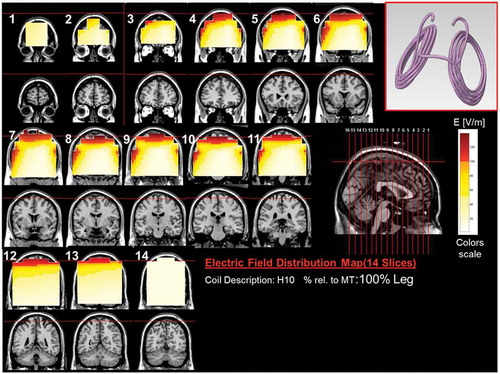
Figure 12. Colored field maps for the H11-coil indicating the absolute magnitude electrical field in each pixel at 120% of hand motor threshold, for 14 coronal slices 1 cm apart. Red pixels indicate regions with field intensity above the threshold for neuronal activation. Full color available online.
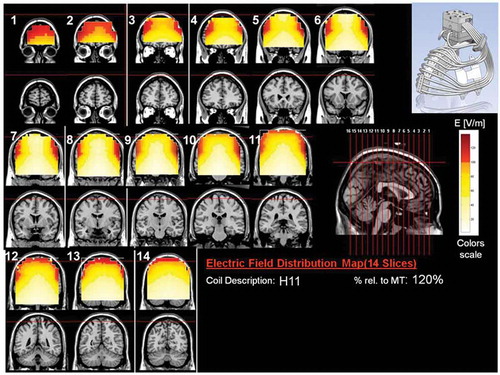
Figure 13. Colored field maps for the H12-coil indicating the absolute magnitude electrical field in each pixel at 100% of hand motor threshold, for 14 coronal slices 1 cm apart. Red pixels indicate regions with field intensity above the threshold for neuronal activation. Full color available online.
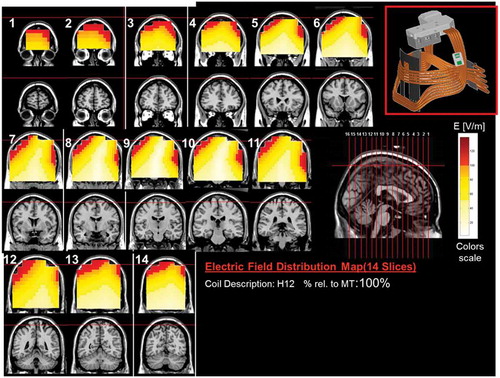
Figure 14. Colored field maps for the H13-coil indicating the absolute magnitude electrical field in each pixel at 120% of hand motor threshold, for 14 coronal slices 1 cm apart. Red pixels indicate regions with field intensity above the threshold for neuronal activation. Full color available online.
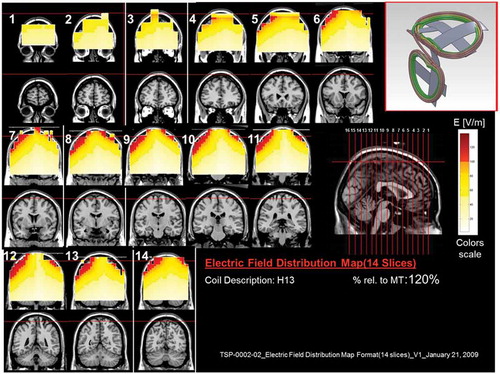
Figure 15. Colored field maps for the H14-coil indicating the absolute magnitude electrical field in each pixel at 110% of leg motor threshold, for 16 coronal slices 1 cm apart. Red pixels indicate regions with field intensity above the threshold for neuronal activation. Full color available online.
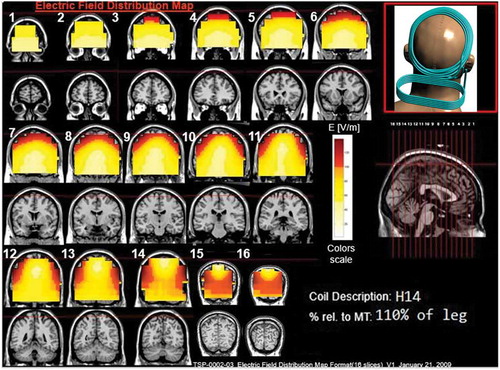
Where does dTMS fit in right now, and what coils should a dTMS center get? The primary disorders a non-research dTMS center will be treating are psychiatric. The H1-coil is the most tested coil, and every dTMS center should have one. It should be used for patients with depression who have failed any number of medications before trying ECT, and it can be used after ECT as a maintenance strategy in lieu of ECT maintenance [Citation97,Citation98]. There is early data suggesting that higher numbers of pulses may be helpful for patients with psychotic depression or schizoaffective disorder depressive phase as well as patients who failed ECT [Citation42,Citation43], the H1 is helpful for the negative symptoms of schizophrenia [Citation46,Citation62]. Additional coils with significant clinical evidence and ongoing multisite clinical trials are the H4 for patients with addiction and the H7 for patients with OCD and PTSD.
4. Five-year view
At present, the field of TMS is focused on targeting one brain area at a time, such as the left DLPFC cortex in depression or the ACC in OCD. As understanding of brain functioning advances, the more evident it is that complicated behaviors are mediated by connected neural networks [Citation85,Citation99]. Thus, it is unlikely that stimulation or inhibition of one area in the brain is the optimal approach to induce normalization of neuronal functioning in all patients. Thus, the future of non-invasive neuromodulation requires the use of a multichannel stimulator with interconnected coils [Citation100] to affect the relevant neuronal networks during tasks and at rest in pathological conditions, and to induce synchronized and optimized differential protocols to different brain regions. In that way, modification of activity can also be induced using paired associative stimulation [Citation101] rather than by trains of pulses. This procedure may greatly affect the patient’s treatment experience as it requires significantly less pulses and a much lower frequency of administration (0.2–0.25 Hz). As a result, the treatment can be painless and free of any side effects. Such a technology, a multichannel stimulator, and multisite H-coils, has been recently presented [Citation100], and preliminary results of ongoing experiments are expected in the near future.
Another issue that is expected to progress in the near future is the individualization of the treatment and the ability to predict treatment outcome prior to its initiation. More specifically, it is expected that future treatment will be based on the individual’s brain activity, measured by EEG or fMRI prior to administration of TMS. This prospect is based, at least in part, on preliminary and yet to be published data that demonstrate how specific brain activity prior to treatment predicted not only response (yes/no) but also the magnitude of that response in MDD or ADHD patients.
Key issues
dTMS is a novel non-invasive deep brain stimulation technique
dTMS has FDA clearance for the treatment of MDD
dTMS is an emerging treatment for several brain-related conditions
Different coils are used for different indications, or to target different neuroanatomical structures
Information resources
dTMS H-coils manufacturer’s web site -http://www.brainsway.com/
Declaration of interest
A. Tendler is the chief medical officer of Brainsway and has a financial interest in Brainsway, the manufacturer of the dTMS H coils. Y. Roth and A. Zangen are the inventors of the H Coil and founders of Brainsway. They have a financial interest in Brainsway, the manufacturer of the dTMS H coils. The authors have no other relevant affiliations or financial involvement with any organization or entity with a financial interest in or financial conflict with the subject matter or materials discussed in the manuscript apart from those disclosed.
Additional information
Funding
References
- Payne NA, Prudic J. Electroconvulsive therapy: part I. A perspective on the evolution and current practice of ECT. J Psychiatr Pract. 2009;15(5):346–368.
- George MS. New methods of minimally invasive brain modulation as therapies in psychiatry: TMS, MST, VNS and DBS. Zhonghua Yi Xue Za Zhi = Chinese Medical Journal; Free China Ed. 2002;65(8):349–360.
- Runge VM, Aoki S, Bradley WG Jr., et al. Magnetic resonance imaging and computed tomography of the brain-50 years of innovation, with a focus on the future. Invest Radiol. 2015;50(9):551–556.
- Slomka PJ, Pan T, Berman DS, et al. Advances in SPECT and PET hardware. Progress in Cardiovascular Diseases. 2015;57(6):566–578.
- Mayberg HS Neuroimaging and psychiatry: the long road from bench to bedside. The Hastings Center report, Spec No, S31–36 (2014).
- Ferrari AJ, Charlson FJ, Norman RE, et al. Burden of depressive disorders by country, sex, age, and year: findings from the global burden of disease study 2010. Plos Medicine. 2013;10(11):e1001547.
- McLaughlin NC, Stewart C, Greenberg BD. Deep brain stimulation for obsessive-compulsive disorder and major depressive disorder. Psychiatric Neurotherapeutics. Springer. 2016;141–163. eBook ISBN 978-1-59745-495-7, doi: 10.1007/978-1-59745-495-7.
- Al-Harbi KS. Treatment-resistant depression: therapeutic trends, challenges, and future directions. Patient Preference and Adherence. 2012;6:369–388.
- Rush AJ, Trivedi MH, Wisniewski SR, et al. Acute and longer-term outcomes in depressed outpatients requiring one or several treatment steps: a STAR. Am J Psychiatry. 1905-1917;163(11):2006.
- Fava GA, Offidani E. The mechanisms of tolerance in antidepressant action. Progress in Neuro-Psychopharmacology & Biological Psychiatry. 2011;35(7):1593–1602.
- Cusin C, Dougherty DD. Somatic therapies for treatment-resistant depression: ECT, TMS, VNS, DBS. Biology of Mood & Anxiety Disorders. 2012;2:14.
- Dukakis KTLS. Shock: the healing power of electroconvulsive therapy; 1 edition: Avery, New York; 2006.
- Husain MM, Rush AJ, Fink M, et al. Speed of response and remission in major depressive disorder with acute electroconvulsive therapy (ECT): a Consortium for Research in ECT (CORE) report. J Clin Psychiatry. 2004;65(4):485–491.
- Lisanby SH. Electroconvulsive therapy for depression. N Engl J Med. 1939-1945;357(19):2007.
- Aouizerate B, Cuny E, Bardinet E, et al. Distinct striatal targets in treating obsessive-compulsive disorder and major depression. J Neurosurg. 2009;111(4):775–779.
- Jimenez F, Velasco F, Salin-Pascual R, et al. A patient with a resistant major depression disorder treated with deep brain stimulation in the inferior thalamic peduncle. Neurosurgery. 2005;57(3):585-593; discussion 585-593.
- Kennedy SH, Giacobbe P, Rizvi SJ, et al. Deep brain stimulation for treatment-resistant depression: follow-up after 3 to 6 years. Am J Psychiatry. 2011;168(5):502–510.
- Malone DA Jr., Dougherty DD, Rezai AR, et al. Deep brain stimulation of the ventral capsule/ventral striatum for treatment-resistant depression. Biol Psychiatry. 2009;65(4):267–275.
- Mayberg HS, Lozano AM, Voon V, et al. Deep brain stimulation for treatment-resistant depression. Neuron. 2005;45(5):651–660.
- Sartorius A, Kiening KL, Kirsch P, et al. Remission of major depression under deep brain stimulation of the lateral habenula in a therapy-refractory patient. Biol Psychiatry. 2010;67(2):e9–e11.
- Schlaepfer TE, Cohen MX, Frick C, et al. Deep brain stimulation to reward circuitry alleviates anhedonia in refractory major depression. Neuropsychopharmacology. 2008;33(2):368–377.
- McIntosh E, Gray A, Daniels J, et al. Cost‐utility analysis of deep brain stimulation surgery plus best medical therapy versus best medical therapy in patients with Parkinson’s: economic evaluation alongside the PD SURG trial. Movement Disorders. 2016;31:1173–1182.
- Bergfeld IO, Mantione M, Hoogendoorn ML, et al. Deep brain stimulation of the ventral anterior limb of the internal capsule for treatment-resistant depression: a randomized clinical trial. JAMA Psychiatry. 2016;73(5):456–464.
- Choi KS, Riva-Posse P, Gross RE, et al. Mapping the “depression switch” during intraoperative testing of subcallosal cingulate deep brain stimulation. JAMA Neurology. 2015;72(11):1252–1260.
- Coenen VA, Schlaepfer TE, Goll P, et al. The medial forebrain bundle as a target for deep brain stimulation for obsessive-compulsive disorder. CNS Spectr. 2016;1–8. doi:10.1017/S1092852916000286.
- Weintraub D, Duda JE, Carlson K, et al. Suicide ideation and behaviours after STN and GPi DBS surgery for Parkinson’s disease: results from a randomised, controlled trial. Journal of Neurology, Neurosurgery, and Psychiatry. 2013;84(10):1113–1118.
- Mayberg HS, Riva-Posse P, Crowell AL. Deep brain stimulation for depression: keeping an eye on a moving target. JAMA Psychiatry. 2016;73(5):439–440.
- Ms G, Post RM. Daily left prefrontal repetitive transcranial magnetic stimulation for acute treatment of medication-resistant depression. Am J Psychiatry. 2011;168(4):356–364.
- Herwig U, Fallgatter AJ, Hoppner J, et al. Antidepressant effects of augmentative transcranial magnetic stimulation: randomised multicentre trial. Br J Psychiatry. 2007;191:441–448.
- Lisanby SH, Husain MM, Rosenquist PB, et al. Daily left prefrontal repetitive transcranial magnetic stimulation in the acute treatment of major depression: clinical predictors of outcome in a multisite, randomized controlled clinical trial. Neuropsychopharmacology. 2009;34(2):522–534.
- George MS, Lisanby SH, Avery D, et al. Daily left prefrontal transcranial magnetic stimulation therapy for major depressive disorder: a sham-controlled randomized trial. Archives of General Psychiatry. 2010;67(5):507–516.
- Johnson KA, Baig M, Ramsey D, et al. Prefrontal rTMS for treating depression: location and intensity results from the OPT-TMS multi-site clinical trial. Brain Stimulation. 2013;6(2):108–117.
- Roth Y, Zangen A, Hallett M. A coil design for transcranial magnetic stimulation of deep brain regions. Journal of Clinical Neurophysiology: Official Publication of the American Electroencephalographic Society. 2002;19(4):361–370.
- Zangen A, Roth Y, Voller B, et al. Transcranial magnetic stimulation of deep brain regions: evidence for efficacy of the H-coil. Clinical Neurophysiology: Official Journal of the International Federation of Clinical Neurophysiology. 2005;116(4):775–779.
- George MS, Belmaker RH. Transcranial magnetic stimulation in clinical psychiatry. American Psychiatric Publishing; 2006.
- Pell GS, Roth Y, Zangen A. Modulation of cortical excitability induced by repetitive transcranial magnetic stimulation: influence of timing and geometrical parameters and underlying mechanisms. Progress in Neurobiology. 2011;93(1):59–98.
- Voineskos D, Daskalakis ZJ. A primer on the treatment of schizophrenia through repetitive transcranial magnetic stimulation. Expert Review of Neurotherapeutics. 2013;13(10):1079–1082.
- Roth Y, Zangen A. Reaching deep brain structures: the H-coils. Transcranial Magnetic Stimulation. Neuromethods. 2014;89:57–65.
- Roth Y, Amir A, Levkovitz Y, et al. Three-dimensional distribution of the electric field induced in the brain by transcranial magnetic stimulation using figure-8 and deep H-coils. J Clin Neurophysiol. 2007;24(1):31–38.
- Levkovitz Y, Isserles M, Padberg F, et al. Efficacy and safety of deep transcranial magnetic stimulation for major depression: a prospective multicenter randomized controlled trial. World Psychiatry. 2015;14(1):64–73.
- Berlim MT, Van den Eynde F, Tovar-Perdomo S, et al. Augmenting antidepressants with deep transcranial magnetic stimulation (DTMS) in treatment-resistant major depression. The World Journal of Biological Psychiatry. 2014;15(7):570–578.
- Tendler A, Sisko E, DeLuca M, et al. Efficacy of deep transcranial magnetic stimulation in patients whose depression is resistant to electroconvulsive therapy. Brain Stimulation. 2016;9(5):e1.
- Tendler A, Sisko E, DeLuca M, et al. Deep transcranial magnetic stimulation for schizoaffective depressive phase. Brain Stimulation. 2016;9(5):e2.
- Dinur-Klein L, Dannon P, Hadar A, et al. Smoking cessation induced by deep repetitive transcranial magnetic stimulation of the prefrontal and insular cortices: a prospective, randomized controlled trial. Biological Psychiatry. 2014;76(9):742–749.
- Harel EV, Zangen A, Roth Y, et al. H-coil repetitive transcranial magnetic stimulation for the treatment of bipolar depression: an add-on, safety and feasibility study. The World Journal of Biological Psychiatry. 2011;12(2):119–126.
- Levkovitz Y, Rabany L, Harel EV, et al. Deep transcranial magnetic stimulation add-on for treatment of negative symptoms and cognitive deficits of schizophrenia: a feasibility study. Int J Neuropsychopharmacol. 2011;14(7):991–996.
- Rosenberg O, Roth Y, Kotler M, et al. Deep transcranial magnetic stimulation for the treatment of auditory hallucinations: a preliminary open-label study. Ann Gen Psychiatry. 2011;10:3.
- Isserles M, Shalev AY, Roth Y, et al. Effectiveness of deep transcranial magnetic stimulation combined with a brief exposure procedure in post-traumatic stress disorder–a pilot study. Brain Stimulation. 2013;6(3):377–383.
- Ceccanti M, Inghilleri M, Attilia ML, et al. Deep TMS on alcoholics: effects on cortisolemia and dopamine pathway modulation. A pilot study. Canadian Journal of Physiology and Pharmacology. 2015;93(4):283–290.
- Girardi P, Rapinesi C, Chiarotti F, et al. Add-on deep transcranial magnetic stimulation (dTMS) in patients with dysthymic disorder comorbid with alcohol use disorder: a comparison with standard treatment. The World Journal of Biological Psychiatry. 2015;16(1):66–73.
- Rapinesi C, Curto M, Kotzalidis GD, et al. Antidepressant effectiveness of deep transcranial magnetic stimulation (dTMS) in patients with major depressive disorder (MDD) with or without alcohol use disorders (AUDs): a 6-month, open label, follow-up study. Journal of Affective Disorders. 2015;174:57–63.
- Rapinesi C, Del Casale A, Scatena P, et al. Add-on deep Transcranial Magnetic Stimulation (dTMS) for the treatment of chronic migraine: a preliminary study. Neuroscience Letters. 2016;623:7–12.
- Harel EV, Rabany L, Deutsch L, et al. H-coil repetitive transcranial magnetic stimulation for treatment resistant major depressive disorder: an 18-week continuation safety and feasibility study. The World Journal of Biological Psychiatry. 2014;15(4):298–306.
- Harvey P-O, Van Den Eynde F, Zangen A, et al. Neural correlates of clinical improvement after deep transcranial magnetic stimulation (DTMS) for treatment-resistant depression: a case report using functional magnetic resonance imaging. Neurocase. 2015;21(1):16–22.
- Isserles M, Rosenberg O, Dannon P, et al. Cognitive–emotional reactivation during deep transcranial magnetic stimulation over the prefrontal cortex of depressive patients affects antidepressant outcome. Journal of Affective Disorders. 2011;128(3):235–242.
- Levkovitz Y, Harel EV, Roth Y, et al. Deep transcranial magnetic stimulation over the prefrontal cortex: evaluation of antidepressant and cognitive effects in depressive patients. Brain Stimulation. 2009;2(4):188–200.
- Rosenberg O, Isserles M, Levkovitz Y, et al. Effectiveness of a second deep TMS in depression: a brief report. Progress in Neuro-Psychopharmacology and Biological Psychiatry. 2011;35(4):1041–1044.
- Rosenberg O, Shoenfeld N, Zangen A, et al. Deep TMS in a resistant major depressive disorder: a brief report. Depress Anxiety. 2010;27(5):465–469.
- Rosenberg O, Zangen A, Stryjer R, et al. Response to deep TMS in depressive patients with previous electroconvulsive treatment. Brain Stimulation. 2010;3(4):211–217.
- Bersani FS, Girardi N, Sanna L, et al. Deep transcranial magnetic stimulation for treatment-resistant bipolar depression: a case report of acute and maintenance efficacy. Neurocase. 2013;19(5):451–457.
- Rapinesi C, Bersani FS, Kotzalidis GD, et al. Maintenance deep transcranial magnetic stimulation sessions are associated with reduced depressive relapses in patients with unipolar or bipolar depression. Frontiers in Neurology. 2015;6:1-5. doi: 10.3389/fneur.2015.00016.
- Rabany L, Deutsch L, Levkovitz Y. Double-blind, randomized sham controlled study of deep-TMS add-on treatment for negative symptoms and cognitive deficits in schizophrenia. Journal of Psychopharmacology. 2014;28(7):686–690.
- Salviati M, Bersani FS, Calabria LF, et al. Deep transcranial magnetic stimulation in a woman with chronic tinnitus: clinical and FMRI findings. Seeking relief from a symptom and finding vivid memories by serendipity. Brain Stimulation. 2014;7(3):492.
- Avirame K, Stehberg J, Todder D. Benefits of deep Transcranial Magnetic Stimulation in Alzheimer disease: case series. J ECT. 2016 Jun;32(2):127-133. doi: 10.1097/YCT.0000000000000286.
- Coppi E, Ferrari L, Nuara A, et al. 71. Repetitive Transcranial Magnetic Stimulation (rTMS) applied with H-coil in Alzheimer’s disease: a placebo-controlled, double-blind, pilot study. Clinical Neurophysiology. 2016;127(4):e148–e149.
- Cohen OS, Orlev Y, Yahalom G, et al. Repetitive deep transcranial magnetic stimulation for motor symptoms in Parkinson’s disease: A feasibility study. Clinical Neurology and Neurosurgery. 2016;140:73–78.
- Torres F, Villalon E, Poblete P, et al. Retrospective evaluation of deep Transcranial Magnetic Stimulation as add-on treatment for Parkinson’s disease. Frontiers in Neurology. 2015;6:1–13. doi: 10.3389/fneur.2015.00210.
- Enticott PG, Fitzgibbon BM, Kennedy HA, et al. A double-blind, randomized trial of deep repetitive transcranial magnetic stimulation (rTMS) for autism spectrum disorder. Brain Stimulation. 2014;7(2):206–211.
- Enticott PG, Kennedy HA, Zangen A, et al. Deep repetitive transcranial magnetic stimulation associated with improved social functioning in a young woman with an autism spectrum disorder. The Journal of ECT. 2011;27(1):41–43.
- Spagnolo F, Volonté M, Fichera M, et al. Excitatory deep repetitive transcranial magnetic stimulation with H-coil as add-on treatment of motor symptoms in Parkinson’s disease: an open label, pilot study. Brain Stimulation. 2014;7(2):297–300.
- Shahar H, Alyagon U, Lazarovits A, et al. Right prefrontal deep tms effects on attention symptoms: behavioral outcomes and electrophysiological correlates. European Psychiatry. 2015;30:841.
- Carmi L, Al Yagon U, Dar R, et al. Deep Transcranial Magnetic Stimulation (Tms) in Obsessive Compulsive Disorder (Ocd) patients. European Psychiatry. 2015;30(Supplement 1):794.
- Dalla Libera D, Colombo B, Coppi E, et al. P19.19 Effects of high-frequency repetitive transcranial magnetic stimulation (rTMS) applied with H-coil for chronic migraine prophylaxis. Clinical Neurophysiology. 2011;122:S145–S146.
- Onesti E, Gabriele M, Cambieri C, et al. H‐coil repetitive transcranial magnetic stimulation for pain relief in patients with diabetic neuropathy. European Journal of Pain. 2013;17(9):1347–1356.
- Chieffo R, De Prezzo S, Houdayer E, et al. Deep repetitive transcranial magnetic stimulation with H-coil on lower limb motor function in chronic stroke: a pilot study. Archives of Physical Medicine and Rehabilitation. 2014;95(6):1141–1147.
- Chieffo R, Ferrari F, Battista P, et al. Excitatory deep transcranial magnetic stimulation with H-coil over the right homologous Broca’s region improves naming in chronic post-stroke aphasia. Neurorehabil Neural Repair. 2014;28(3):291–298. doi: 10.1177/1545968313508471.
- Vazana U, Veksler R, Pell G, et al. Glutamate-mediated blood-brain barrier opening: implications for neuroprotection and drug delivery. Journal of Neuroscience. (inpress2016);36:7727–7739.
- Gersner R, Oberman L, Sanchez M, et al. H-coil repetitive transcranial magnetic stimulation for treatment of temporal lobe epilepsy: a case report. Epilepsy Behav Case Rep. 2016;5:52–56.
- Tendler A, Roth Y, Barnea-Ygael N, et al. How to use the H1 deep Transcranial Magnetic Stimulation coil for conditions otherthan depression. Journal of Visualized Experiments (Jove). In press (2016).
- Perera T, George MS, Grammer G, et al. The clinical TMS society consensus review and treatment recommendations for TMS therapy for major depressive disorder. Brain Stimulation. 2016;9:336–346.
- Gray AJ. Stigma in psychiatry. J R Soc Med. 2002;95(2):72–76.
- Hawke LD, Parikh SV, Michalak EE. Stigma and bipolar disorder: a review of the literature. Journal of Affective Disorders. 2013;150(2):181–191.
- Shrivastava A, Bureau Y, Rewari N, et al. Clinical risk of stigma and discrimination of mental illnesses: need for objective assessment and quantification. Indian J Psychiatry. 2013;55(2):178–182.
- Shrubb S. Individuals who experience mental health problems describe stigma and discrimination as one of the biggest barriers they face in their journey of recovery. Ment Health Today. 2009;9.
- Downar J, Daskalakis ZJ. New targets for rTMS in depression: a review of convergent evidence. Brain Stimulation. 2013;6(3):231–240.
- Zhang H, Chen Z, Jia Z, et al. Dysfunction of neural circuitry in depressive patients with suicidal behaviors: a review of structural and functional neuroimaging studies. Progress in Neuro-Psychopharmacology & Biological Psychiatry. 2014;53:61–66.
- Deng Z-D, Lisanby SH, Peterchev AV. Electric field depth-focality tradeoff in transcranial magnetic stimulation: simulation comparison of 50 coil designs. Brain Stimulation. 2013;6(1):1–13.
- Deng Z-D, Lisanby SH, Peterchev AV. Coil design considerations for deep transcranial magnetic stimulation. Clinical Neurophysiology. 2014;125(6):1202–1212.
- Fiocchi S, Longhi M, Ravazzani P, et al. Modelling of the electric field distribution in deep Transcranial Magnetic Stimulation in the adolescence, in the adulthood, and in the old age. Computational and Mathematical Methods in Medicine. 2016;2016, Article ID 9039613, Available from: http://dx.doi.org/10.1155/2016/9039613
- Guadagnin V, Parazzini M, Fiocchi S, et al. Deep Transcranial Magnetic Stimulation: modeling of different coil configurations. IEEE Trans Biomed Eng. 2016;63(7):1543–1550.
- Guadagnin V, Parazzini M, Fiocchi S, et al. Electric field estimation in deep transcranial magnetic stimulation. Brain Stimulation. 2015;8(2):327.
- Fadini T, Matthäus L, Rothkegel H, et al. H-coil: induced electric field properties and input/output curves on healthy volunteers, comparison with a standard figure-of-eight coil. Clinical Neurophysiology: Official Journal of the International Federation of Clinical Neurophysiology. 2009;120(6):1174–1182.
- Roth Y, Pell GS, Chistyakov AV, et al. Motor cortex activation by H-coil and figure-8 coil at different depths. Combined motor threshold and electric field distribution study. Clinical Neurophysiology: Official Journal of the International Federation of Clinical Neurophysiology. 2014;125(2):336–343.
- O’Reardon JP, Solvason HB, Janicak PG, et al. Efficacy and safety of transcranial magnetic stimulation in the acute treatment of major depression: a multisite randomized controlled trial. Biological Psychiatry. 2007;62(11):1208–1216.
- Manevitz A, Halper J, Yagi C, et al. Review of 200 patients (7,000 treatments) clinical outcomes of Transcranial Magnetic Stimulation (TMS)therapy performed in a naturalistic clinical setting using the Brainsway™ (H coil) and Neurostar™ stimulators: a biopsychosocial integrated clinical care approach. Brain Stimulation. 2015;8(5):e3–e4.
- Viner L, Viner J. Comparison of TMS outcomes in complex major depressive disorder: Brainsway and Neurostar. Brain Stimulation. 2016;9(5):e5–e6.
- Cristancho MA, Helmer A, Connolly R, et al. Transcranial magnetic stimulation maintenance as a substitute for maintenance electroconvulsive therapy: a case series. J Ect. 2013;29(2):106–108.
- Noda Y, Daskalakis ZJ, Ramos C, et al. Repetitive transcranial magnetic stimulation to maintain treatment response to electroconvulsive therapy in depression: a case series. Front Psychiatry. 2013;4:73.
- Sale MV, Mattingley JB, Zalesky A, et al. Imaging human brain networks to improve the clinical efficacy of non-invasive brain stimulation. Neuroscience & Biobehavioral Reviews. 2015;57:187–198.
- Roth Y, Levkovitz Y, Pell GS, et al. Safety and characterization of a novel multi-channel TMS stimulator. Brain Stimulation. 2014;7(2):194–205.
- Stefan K, Kunesch E, Cohen LG, et al. Induction of plasticity in the human motor cortex by paired associative stimulation. Brain. 2000;123(3):572–584.