Abstract
Children are potentially exposed to products that contain nanoparticles (NPs). In particular, silver NPs are commonly present both in products used by and around children, primarily due to their antibacterial properties. However, very few data are available regarding the ability of silver NPs to penetrate through the oral mucosa in children. In the present work, we used baby porcine buccal mucosa mounted on vertical Franz diffusion cells, as an in vitro model to investigate penetration of silver NPs (19 ± 5 nm). Permeability experiments were performed using pristine physiologically-relevant saline solution in the receiver chamber and known concentrations of NPs or ions in the donor chamber; conditions mimicked the in vivo physiological pH conditions. After physicochemical characterization of silver nanoparticles dispersed in physiological solution, we evaluated the passage of ions and NPs through the mucosa, using single particle inductively coupled plasma mass spectrometry. A flux of 4.1 ± 1.7 ng cm−2 min−1 and a lag time of 159 ± 17 min were observed through mucosa exposed to silver nanoparticles. The latter suggests nanoparticle penetration through the baby porcine mucosa and release Ag+ ions in the receptor fluid, as confirmed by computational model. Due to physiological similarity between human and pig membranes it is reasonable to assume that a trans-oral mucosa penetration could occur in children upon contact with silver nanoparticles.
1. Introduction
Silver nanoparticles (Ag NPs) are widely sold and used in different applications that babies and young children can come into contact with. These include food packaging, containers, toothpaste and tooth brushes, alcohol free mouthwash, nasal sprays, textiles, medical devices, water purification devices and others. The wide use of Ag NPs in many applications that involve human occupational and consumer exposure is justified by their known antibacterial, antiviral and antifungal properties to prevent infections. Despite its previous use (for instance colloidal silver ‘Collargol’ has been used for medical applications and has been manufactured commercially since 1897), Ag NPs gained renewed interest when its use as antibacterial and antiviral nanotechnology became more widespread and better supported by scientific evidence (Dung et al. Citation2020; Schneider Citation2017). In addition to their benefits, there are concerns about potential human and environmental hazard (Bouwmeester et al. Citation2009; Epstein et al. Citation2014; Lead et al. Citation2018). Regarding the exposure limits already available, the US Environmental Protection Agency (EPA) (U.S. Environmental Protection Agency Chemical Assessment Citation1991) suggests a value of bulk silver of <5 μg kg−1 daily as reference dose (Rfd) for oral exposure, corresponding to a total daily amount of 350 μg of silver for a 70 kg adult. While the European Chemicals Agency (ECHA) recommended as silver Derived No Effect Level (DNEL) for human long oral exposure a value of 1.2 μg kg−1 daily, corresponding to 84 μg of silver for a 70 kg adult, slightly below the previous value (Silver - Registration Dossier - ECHA n.d.). While there are some reference values are bulk or total Ag, these are lacking for NPs. In this regard. The Scientific Committee on Consumer Safety (SCCS) recommended a maximum concentration limit of 1% w/w in products, considering reasonably foreseeable exposure conditions used in cosmetics including toothpastes and skin care products. However, the SCCS is not in the position to draw a conclusion on the safety of Ag NPs when used in oral and dermal cosmetic products due to a number of major data gaps (European Commission Citation2018). Moreover, silver intoxication (argyria) is reported in literature and has been described through oral (Chang, Khosravi, and Egbert Citation2006; Johnston et al. Citation2010) or dermal (Trop et al. Citation2006) exposure. The US Agency for Toxic Substances and Disease Registry (ATSDR) describes argyria as a ‘cosmetic issue’, since it consists mainly in a blue-gray discoloration of the skin (Agency for Toxic Substances and Disease Registry (ATSDR) Citation1999). Nevertheless, these are irreversible. There are also isolated reports of more serious neurologic, renal and hepatic complications caused by the ingestion of colloidal silver (Singh et al. Citation2011; Stepien et al. Citation2009), and there are concerns about microbiome changes from Ag NPs ingestion, affecting organism health (Li, Tang, and Xue Citation2019).
Ag NPs are therefore able to come in contact with human targets and specifically with the oral mucosa (Lansdown Citation2006; León-Silva, Fernández-Luqueño, and López-Valdez Citation2016), especially in applications from the food and personal care sectors. The oral mucosa traditionally acts as a first barrier to xenobiotics in the digestive tract (Harris and Robinson Citation1992; Wertz and Squier Citation1991). However, due to its histological structure, the oral mucosa shows a permeability 20 times higher to water (Lesch et al. Citation1989) and up to 4000 times higher to certain pharmaceuticals when compared to skin (Fox and Modak Citation1974). In addition, investigation of penetration through oral mucosa could support toxicokinetic studies related to Ag NPs exposure after oral administration, if linked with toxicity studies by the gastrointestinal tract (GIT) route (Bergin and Witzmann Citation2013). Despite much research, trans-oral sorption and penetration rates and mechanisms of NPs through mucosa are not completely understood (Roblegg et al. Citation2012). Experimental data are in most cases derived from animal or human adult donor skin and are likely to be unsuitable to predict transdermal penetration in children. The porcine lining mucosa is the most similar to the human one in terms of structure and enzyme activity and is a thoroughly investigated in-vitro model to estimate human buccal absorption and drug penetration (Lesch et al. Citation1989). Currently, NP penetration through oral mucosa is not fully quantified for adults and even fewer data are available for children (Mauro et al. Citation2015; Mauro Citation2018). Children can come into contact with Ag NPs embedded in commercial products such as pajamas, baby blankets, soft toys and also in items used around children, such as disinfectant sprays, surface wipes and kitchen cleaners (Quadros et al. Citation2013), exposing skin and the buccal cavity of children to silver ionic and NPs form. In some applications and products addressed to children and adults, the exposure range is a few mg day−1 (values calculate considering different European disinfecting sprays products for oral application – see Table S1 ESI). In addition, children’s skin and oral mucosa significantly differ from adults and so available nanotoxicological knowledge cannot predict infant’s dermal and oral sorption. Finally, infant’s transdermal exposure can lead to systemic effects, due to the differences in permeability and in the activity of metabolic pathways, potentially transforming substances which are of low toxicity in adults into more toxic substances for children (Fröhlich and Roblegg Citation2016; Tang et al. Citation2015; Tulve et al. Citation2015).
Table 1. SP-ICP-MS measurement of receptor fluid exposed to donor fluid 1.
For the above reasons, in this work, we performed experiments to investigate the possible penetration of Ag NPs into/across the children buccal mucosa, using a standard dermatological in-vitro ex-vivo model (Franz cell), applied to oral baby porcine mucosa (Adams Citation1974; Bergman, Siegel, and Ciancio Citation1968). In particular, we coupled for the first time in this application, the Franz cell model with the use of single particle – inductively coupled plasma – mass spectrometry (SP-ICP-MS) technique and with the computational model Visual MINTEQ 3.1 (Visual MINTEQ – Visual MINTEQ – a Free Equilibrium Speciation Model Citation2021). The use of SP-ICP-MS as a powerful analytical method is growing and reached different application fields dealing with complex biological and environmental matrices (Dan et al. Citation2015; Dimitrova et al. Citation2017; Laborda et al. Citation2013; Lee et al. Citation2014; Lee and Chan Citation2015; Merrifield, Stephan, and Lead Citation2017a; Merrifield, Stephan, and Lead Citation2017b; Mitrano et al. Citation2014; Mitrano et al. Citation2012). SP-ICP-MS analysis allowed us to perform the quantitative and simultaneous estimation of Ag NPs and Ag+ ions in the receptor fluid, avoiding the limitations of ultrafiltration process. While, the computational model Visual MINTEQ 3.1 was applied in support to the SP-ICP-MS measurements, confirming the silver speciation in the receptor fluid.
2. Materials and methods
2.1. Chemicals and reagents
All chemicals used were of analytical grade. Sodium chloride, sodium hydrogen phosphate, potassium dihydrogen phosphate, glutaraldehyde (50% v/v), nitric acid (69% v/v), hydrochloric acid (36.5–38.0% v/v) and hydrogen peroxide (30%) were purchased from Sigma Aldrich (Milan, Italy), while ammonium hydroxide (25%) from J.T. Baker (Milan, Italy). Water reagent grade was produced with a Millipore purification pack system (MilliQ water). The physiological solution used as receptor fluid was prepared by dissolving 2.38 g of Na2HPO4, 0.19 g of KH2PO4 and 9 g of NaCl into 1 L of MilliQ water (final pH = 7.35). Silver NPs suspensions for this study were supplied by NanoAmor Materials Inc. (Houston, TX, USA) and were stabilized with PVP - polyvinylpirrolidone (content of silver: 25% w/w, polymer 75%). Ionic Au and Ag standard were used for calibration of ICP-MS, respectively ion mass of 197 and 107 a.m.u. Au 60 nm citrate coated NPs were used as standard for single-particle calibration and transport efficiency calculations (Table S2). All standards used were purchased from Sigma Aldrich (Milan, Italy).
2.2. Characterization of Ag NPs
Dynamic light scattering (dDLS) and Zeta potential (ZP - ζ-potELS) measurements on Ag NPs were performed using a ZetasizerNano ZS (Malvern Instruments Ltd.). Ag NPs suspension was diluted 1:5 in physiological solution and loaded into low size disposable cuvette. Zeta potential was calculated using Henry’s equation. Summary statistics were obtained using quadruplicate 3 min analysis (total analysis time = 12 min). Transmission electron microscopy (TEM) was carried out on an EM208, operating at 200 kV (Philips, Eindhoven, The Netherlands), with a high definition acquisition system based on a side-mounted TEM camera OSIS Morada and an iTEM software platform (Olympus Soft Imaging Solutions GmbH, Münster, Germany). Ag NPs, dispersed in physiological solution, were placed onto a carbon-coated grid and dried at room temperature under vacuum.
2.3. Static Franz diffusion cell system
In vitro permeation studies were performed using a Static Franz Diffusion Cell System (see Figure S1), an in vitro model for testing static diffusion through biological membranes (Franz Citation1975). In mucosal studies, the receptor compartment has a mean volume of 4.5 mL and was maintained at 37 °C by means of circulation of thermostated water in the jacket surrounding the cells throughout the experiment. This temperature value was chosen in order to reproduce oral physiological conditions. The concentration of the salt in the receiver fluids was approximately the same that can be found in the blood. The solution in each cell was continuously stirred using a Teflon-coated magnetic stirrer. Each excised sheet of mucosa was clamped between the donor and the receptor compartment, in this way the epithelium faced the donor and the connective tissue region faced the receiver compartment. The mean exposed area of the mucous membranes was 0.95 cm2. Four cells were used as controls, using physiological solution as donor fluid.
2.4. Preparation of stock suspensions
In order to discriminate between Ag NPs and silver ions, released from the NPs, two different donor phases were prepared just before the experiments. The stock suspensions of donor fluid 1 and 2 were prepared, accordingly to the following procedure. For the stock 1, 200 mg of PVP-coated Ag NPs were dispersed in 100 mL of physiological solution and sonicated, at the aim to obtain a concentration of 500 mg L−1 (as silver content). Stock 2 was prepared by ultrafiltering the stock 1 (5000 rpm for 30 min by means of Amicon Ultra-4 centrifugal filters, 10 KDa MWCO), in order to separate and collect the water-soluble silver species of the stock 1. The ultrafiltered solution (stock 2) was analyzed by ICP-OES, and the concentration of ions was quantified (25 mg L−1 − 5% of the stock 1 silver content) and checked, also after the end of the experiments (240 min), to confirm that it remained stable. Stock 2 aims to reproduce the behavior of Ag ions fraction in the permeation process, comparing to stock 1 results. Five ultrafiltered aliquots (of 4 mL each) were mixed and used during the experiments as stock 2. The two donor fluids were prepared by adding 0.5 mL of the two stocks to 1 mL of physiological solution, before feeding the Franz Cell, reaching daily exposure concentrations comparable to the ones reported in some applications and products.
2.5. Preparation of oral mucosa
Due to its morphological and enzymatic similarities with the children mucosa, baby porcine oral mucosa was used for the in-vitro experiments (Adams Citation1974; Bergman, Siegel, and Ciancio Citation1968; Lesch et al. Citation1989). The membranes were provided by a local butcher's shop and obtained immediately after the pigs were sacrificed (porcine age between 4 and 8 months). During the transport to laboratory, the tissue was stored at 4 °C and then in freezer at −80 °C for a period of time up to, but not exceeding, 1 week. On the day of the experiment, the tissue was removed from the freezer and thawed in a physiologically-relevant solution (NaCl 9 wt.%), at room temperature, for approximately 30 min before the experiment. It has been shown that this method of storage does not affect the mucous barrier properties, since no change in the permeability has been previously mentioned (Nicolazzo, Reed, and Finnin Citation2003). Mucous membranes integrity was tested as suggested by Lestari (Lestari, Nicolazzo, and Finnin Citation2009). Mucosa integrity was checked even at the end of experiment, finding no alterations or damages in the oral mucosa.
2.6. Experimental set-up used for in vitro permeation studies
The experimental set-up used for testing Ag concentration in different compartments of Franz Cell System is shown in .
The first experiment was performed with the donor fluid 1 (1 mL of physiological solution and 0.5 mL of Ag NPs stock 1 suspension). At time 0, the exposure chambers of 4 Franz diffusion cells were filled with donor fluid 1 (260 µg cm−2). At selected intervals (20, 40, 60, 90, 120, 150, 180, 210, 240 min), 1 mL of the receptor fluid was removed and collected for the analysis, and immediately replaced with an equal volume of fresh physiological solution. The experiment was performed for 240 min, as suggested in other studies (Mauro et al. Citation2015; Roblegg et al. Citation2012). At the end of the experiment, the mucosal pieces were removed, washed with MilliQ water, and subsequently stored in the freezer together with donor and receptor solutions for performing ICP-OES, ICP-MS/SP-ICP-MS analysis. The experiment was repeated twice for a total of 8 Franz cells. The second experiment was performed with the donor fluid 2 (1 mL of physiological solution and 0.5 mL of the Ag ultra-filtered stock 2 solution). The exposure chambers of 4 Franz diffusion cells were filled donor fluid 2(13 µg cm−2). The other test conditions were the same of the experiment 1. The experiment was repeated twice for a total of 8 Franz cells.
2.7. Mucosal digestion
All the mucosal exposed samples were collected and stored individually in freezer at −25 °C for the following digestion and analysis. At the time of the analysis, the skin membranes were dried for 2 hours at room temperature, weight, and then acid-digested in a closed microwave system (Multiwave PRO, Anton Paar) using 2.5 mL of HNO3 (69%) and 0.5 mL of H2O2 (30%) for digestion. The obtained solutions diluted to a final volume of 10 mL with MilliQ water for the ICP-OES analysis.
2.8. Quantification of total metal content
Total Ag concentration in the solutions resulting from the mineralization of the mucosa samples were performed by ICP-OES using an Optima 8000 Spectrometer (PerkinElmer, U.S.A.), equipped with an S10 Autosampler. Analyses were conducted using a calibration curve obtained by dilution (range: 0 − 10 mg L−1) of Ag standard solution for ICP-OES analyses. The limit of detection (LOD) for Ag at the operative wavelength of 328.068 nm was 0.01 mg L−1. The precision of the measurements expressed as RSD % for the analysis was always less than 5%. An Inductive Coupled Plasma Mass Spectrometer, ICP-MS Nexion 350X with an ESI autosampler (Perkin Elmer, USA instrument), was used to determinate the total silver concentration in the donator and receiver phases, after solubilization with 1 mL of HNO3 (69%) for 10 mL of sample. The analysis was carried out in KED mode using ultra-high purity helium (flow rate of 4.8 mL min−1) to minimize polyatomic interferences. The Ag calibration curve for dissolved metal element content was performed using Ag ionic standard (0.5, 1.0, 5.0 and 10.0 μg L−1, ion mass 107 a.m.u.).
2.9. Sp-ICP-MS instrumentation and working conditions
The SP-ICP-MS analysis were run using the Nano Application Module in Syngisitx™ software (version 2.1). A pump speed 20 rpm for flush, wash and delay, and nebulizer gas flow of 1.12 mL min−1 were used for measurements. The sample uptake was 0.34 mL min−1 and a dwell time of 50 μs was used with 60 s sampling times. To calculate the transport efficiency (1.5 and 10 µg L−1) ionic Au standard was used for SP-ICP-MS ionic calibration (ion mass 197 a.m.u.). While Au 60 nm NPs citrate coated suspension were used as standard for NPs size dimension in Single-Particle software calibration material. The 60 nm Au particles were diluted in MilliQ water (18.2 MΩ cm), for SP-ICP-MS size calibration, to approximately 50,000 ± 10,000 particle mL−1. We derived this value, considering the NPs number reported in the Sigma Aldrich datasheet (1.76–2.16 1010). NPs concentration and other parameters provided by the supplier are reported in Table S2. We calculated a transport efficiency of 4.0 ± 0.2%. NPs dimension detectable by SP-ICP-MS is NPs dimension >15 nm, while Ag ions are not measured by SP-ICP-MS directly or by difference accurately for several reasons (Merrifield, Stephan, and Lead Citation2017b). Calibration curve obtained for Ag dissolution concentration is reported in Figure S2. Ag concentration data (μg cm−3) in the receptor solution were converted to the Ag amount that penetrated per skin surface unit (μg cm−2). Data analysis was performed with Excel for Windows, release 2007 and Stata Software, version 11.0 (StataCorp LP, College Station, TX, USA). Skin absorption data were reported as mean Ag total concentration ± SD. The difference among independent data was assessed by means of the Mann-Whitney test. A p value <0.05 was considered significant. Silver speciation was estimated with the use of computational model Visual MINTEQ 3.1 (Visual Basic 2012). Using as input data: pH, temperature, ions concentration and media composition, the computational model can provide as output information about possible soluble and insoluble species (Kps and ΔHf thermodynamic constants are reported in Table S4). This calculation allowed a more accurate estimation of the ions fraction present in the receptor fluid, once subtracted the amount of Ag NPs measured by SP-ICP-MS.
3. Results and discussion
3.1. Characterization of Ag NPs
The particle size distribution of Ag NPs in water and in exposure medium (donor fluid 1) was evaluated. TEM images show spherical NPs with a quite narrow distribution of size, quantified as 19 ± 5 nm (n = 150). TEM measurements were compared to DLS diameter size distribution ().
The expected difference between the TEM and DLS sizes was the result of various factors, as elsewhere reported (Blosi et al. Citation2011). In principle, the DLS technique measures the hydrodynamic size and is influenced by polydispersity and aggregation.
3.2. Ag penetration in oral mucosa
The concentration of Ag detected in the mucosa after 240 min of exposure of donor fluid 1 and 2 are reported in and Figure S3. The higher Ag concentration found after exposure to donor fluid 1 (0.32 µg cm−2), in comparison with donor fluid 2 (0.08 µg cm−2), is explained by the translocation of both Ag ions and NPs. However, in both cases, the concentration of silver able to penetrate into the mucosa is very low (less than 1%). The higher percentage of penetration is found for the donor fluid 2, indicating the higher mobility of Ag ions compared to Ag NPs. In both cases (donor fluid 1 and 2), the dose extrapolated values and reported in Table S3 were below the limits recommended by EPA and ECHA for adult population exposed to silver (see extrapolation reported in ESI). In addition, comparing our results with the penetration of Ag (NPs and ions) reported in literature for adult porcine mucosa at the similar exposure condition (Mauro et al. Citation2015), we found an higher content of silver in baby tissues. The result is consistent with differences between young and adult mucosal membranes. Oral mucosa thickness, in fact, is age-dependent (Burns et al. Citation2004) and as a consequence the skin/mucosa permeability of baby tissues is higher than in adult individuals, especially during the first year of life, justifying the observed higher accumulation of silver in the mucosa.
3.3. Ag permeation in receptor fluid
The total silver concentration detected in the receptor fluid is reported in . The permeation of silver from donor fluid 2 is negligible and not significantly different to the blank (p values > 0.05). This suggests that Ag+ ions are most likely precipitated and/or stopped and absorbed into mucosa, probably by the binding with -SH group present in the oral membrane(Iurina, Pil’tiaĭ, and Protsenko Citation1986; Toto Citation1965) and are not able to reach the receptor fluid. While for donor fluid 1 (Ag NPs + Ag+), we observed a permeation of silver in the receptor fluid with a flux of 4.1 ± 1.7 ng cm−2 min−1 and a lag time of 159 ± 17 min, most likely attributed to the NPs fraction. These results confirm the capability of PVP coating to mask the Ag NPs surface and allow a better dispersibility and stability, promoting NP permeation through tissue (Merrifield and Lead Citation2016; Tejamaya et al. Citation2012), even if in a very low concentration compare to the initial concentration (ca. 0.04% wt). Thus, this behavior could potentially promote toxic effects, through a Trojan horse mechanism, that would justify the higher reported cytotoxicity of Ag NPs if compared with the same concentration of Ag ions (Park et al. Citation2010).
Figure 4. Silver permeation in the receptor fluid. The value of silver total concentration after 240 min of exposure correspond to a silver content of 65.7 ± 16.4 µg L−1 in receptor fluid (*: values are significant different from the blank, p < 0.05).
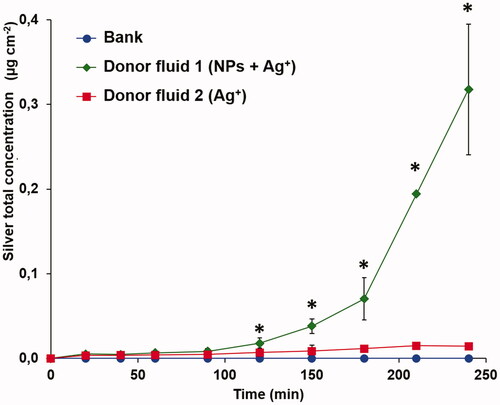
The results of SP-ICP-MS analysis of the receptor fluid, exposed to donor fluid 1, are reported in and in . As shown in , the permeation of Ag has a time lag of ca. 150 min. The size of particles detected by SP-ICP-MS, after this time (23 ± 8 nm), is comparable with TEM size, suggesting a penetration through the oral mucosa of untransformed NPs. The size distribution histograms of and show how NPs detected are distributed in terms of size and relative frequency. The observed variation of particle number has not statistically significant (p = 0.28), so we can conclude that the particles number does not seem to be affected by time of exposure (). In addition, the nonsignificant change in most frequent size and mean diameter vs time of exposure shown in , stays within the standard deviation, suggesting the absence of aggregation phenomena or of Ostwald ripening growth during the time of experiment. Computational analysis, using the speciation code MINTEQ, was also performed to estimate dissolved silver speciation in the receptor fluid (Table S4). The computational outputs suggest that the dissolved fraction in the receptor fluid, exposed to donor fluid 1, is mainly characterized by the presence of soluble species as AgCl2− and AgCl3−2 (more than 93% of total ionic silver). The small amount of insoluble AgCl hypnotized by the computational model, ca. 6%, only slightly affect the estimation of Ag NPs size on Ag ions content (difference between Ag total and Ag NPs concentration), made by SP-ICP-MS technique.
Figure 5. Ag NPs size distribution by frequency detected by SP-ICP-MS, at different time of exposure.
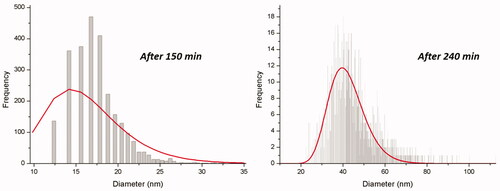
Figure 6. Size distribution of Ag NPs permeated in the receptor fluid as a function of time: ▪ most frequent size (size at the maximum of the size distribution histogram) and ♦ mean size (Z-average). There are no statistically relevant differences between the two size populations (blue and orange) (p < 0.05 only for sample at 210 min) and comparing different times (ca. p = 0.17).
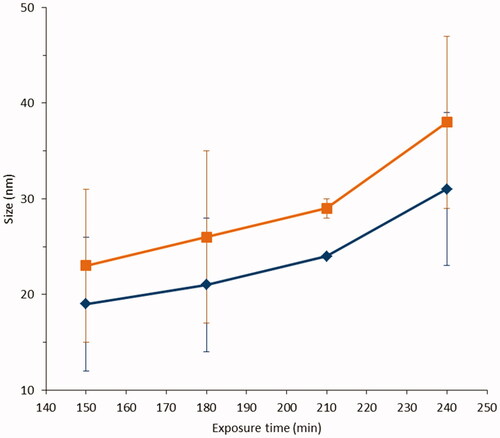
Therefore, we found that Ag NPs are able to penetrate the baby porcine oral mucosa, reaching the receptor fluid and being potentially available for translocation processes after in-vivo exposure. The increase in ions amount, from 5% in donor fluid, to ca.25% in the receptor fluid can be most likely attributed to Ag NPs surface transformation (more likely adsorption of bio-surfactants when crossing mucosa), that could justify the higher amount of ions released that stay in equilibrium with solid surface (Gardini et al. Citation2018). Due to similarity between human and pig membranes, as reported in literature (Adams Citation1974), it is reasonable to assume that a trans-oral mucosa passage could occur also in children mucosa once in contact, voluntary or accidentally, with silver NPs.
4. Conclusions
To our knowledge, this work presents one of the first attempts to evaluate Ag NP permeation and penetration through baby porcine oral mucosa to predict the effect of potential oral absorption of Ag NPs in children. Franz ex-vivo in-vitro diffusion cell was used to investigate the total amount of silver penetrating the oral mucosa and achieving the receptor fluid, during the first 240 min of exposure. The comparison between the two donor fluids, the first containing Ag NPs dispersion and the second only the ionic fraction, collected from the first donor fluid, suggested that PVP coated Ag NPs are untransformed and cross the mucosa, reaching the donor fluid, whilst Ag+ ion speciate with Cl- and cannot easily translocate. The SP-ICP-MS analysis of the receptor fluid and the use of computational model allowed us to estimate the content of Ag NPs and Ag+ ions and the NPs degree of aggregation/agglomeration, revealing that the Ag NPs reaching the donor fluid are quite well dispersed and able to release a higher concentration of ions if compared to the pristine donor fluid. Due to the similarity between human and pig oral mucosa, these findings would support in baby individuals the potential permeation of Ag NPs through oral mucosa, even at level below the reference doses recommended by existing authorities (EPA and ECHA).
N93_Supplementary_File_Ag_dermal__3_.pdf
Download PDF (440 KB)Acknowledgements
The research leading to these results has received funding from Doctoral School in Nanotechnology at University of Trieste, from FRA 2018 projects (linea E) from University of Trieste and from the EU H2020 PATROLS (Physiologically Anchored Tools for Realistic nanOmateriaL hazard aSsessment) n° 760813 Project (H2020-NMBP-2017). Funding to JRL from the US National Science Foundation (1508931) is acknowledged. The authors acknowledge Mrs Elizabeth Bair for the support provided during the design of SP-ICP-MS experiments.
Disclosure statement
No potential conflict of interest was reported by the author(s).
Correction Statement
This article has been corrected with minor changes. These changes do not impact the academic content of the article.
Additional information
Funding
References
- Adams, D. 1974. “Penetration of Water through Human and Rabbit Oral Mucosa in Vitro.” Archives of Oral Biology 19 (10): 865–IN11. doi:https://doi.org/10.1016/0003-9969(74)90048-X.
- Agency for Toxic Substances and Disease Registry (ATSDR) 1999. Silver CAS # 7440-22-4 ToxFAQs.
- Bergin, Ingrid L., and Frank A. Witzmann, 2013. “Nanoparticle Toxicity by the Gastrointestinal Route: Evidence and Knowledge Gaps.” International Journal of Biomedical Nanoscience and Nanotechnology 3 (1/2): 163. doi:https://doi.org/10.1504/IJBNN.2013.054515.
- Bergman, Stuart, Ivens A. Siegel, and Sebastian Ciancio, 1968. “Absorption of Carbon-14 Labeled Lidocaine through the Oral Mucosa.” Journal of Dental Research 47 (6): 1184. doi:https://doi.org/10.1177/00220345680470063001.
- Blosi, M., S. Albonetti, M. Dondi, C. Martelli, and G. Baldi, 2011. “Microwave-Assisted Polyol Synthesis of Cu Nanoparticles.” Journal of Nanoparticle Research 13 (1): 127–138. doi:https://doi.org/10.1007/s11051-010-0010-7.
- Bouwmeester, Hans, Susan Dekkers, Maryvon Y. Noordam, Werner I. Hagens, Astrid S. Bulder, Cees de Heer, Sandra E. C. G. ten Voorde, Susan W. P. Wijnhoven, Hans J. P. Marvin, and Adriënne J. A. M. Sips, 2009. “Review of Health Safety Aspects of Nanotechnologies in Food Production.” Regul Toxicol Pharmacol 53 (1): 52–62. doi:https://doi.org/10.1016/j.yrtph.2008.10.008.
- Burns, D. A., S. M. Breathnach, N. Cox, and C. E. Griffiths. 2004. Rook’s Textbook of Dermatology. Rook’s Textbook of Dermatology. 7th ed. Vol. 1–4. USA: Blackwell Publishing.
- Chang, Anne Lynn S., Vista Khosravi, and Barbara Egbert, 2006. “A Case of Argyria after Colloidal Silver Ingestion.” Journal of Cutaneous Pathology 33 (12): 809–811. doi:https://doi.org/10.1111/j.1600-0560.2006.00557.x.
- Dan, Yongbo, Honglan Shi, Chady Stephan, and Xinhua Liang, 2015. “Rapid Analysis of Titanium Dioxide Nanoparticles in Sunscreens Using Single Particle Inductively Coupled Plasma–Mass Spectrometry.” Microchemical Journal 122: 119–126. doi:https://doi.org/10.1016/j.microc.2015.04.018.
- Dimitrova, Nadya, Jesse R. Zamudio, Robyn M. Jong, Dylan Soukup, Rebecca Resnick, Kavitha Sarma, and Amanda J. Ward, 2017. “Public Access NIH Public Access.” PLoS One. 32 (7): 736–740. doi:https://doi.org/10.1371/journal.pone.0178059.
- Dung, Tran Thi Ngoc, Vu Nang Nam, Tran Thi Nhan, Trinh Thi Bich Ngoc, Luu Quang Minh, Bui Thi To Nga, Van Phan Le, and Dang Viet Quang, 2020. “Silver Nanoparticles as Potential Antiviral Agents against African Swine Fever Virus.” Materials Research Express 6 (12): 1250g9. IOP Publishing: 1250g9. doi:https://doi.org/10.1088/2053-1591/ab6ad8.
- Epstein, Michelle, Igor Emri, Philippe Hartemann, Peter Hoet, Norbert Leitgeb, Luis Martínez Martínez, Ana Proykova, et al. 2014. “Scientific Committee on Emerging and Newly Identified Health Risks SCENIHR Opinion on Nanosilver: Safety.” Health and Environmental Effects and Role in Antimicrobial Resistance: 1–64. doi:https://doi.org/10.2772/76851.
- European Commission 2018. Directorate General for Health and Food Safety. Opinion on Colloidal Silver (Nano). LU: Publications Office. https://doi.org/https://doi.org/10.2875/586035.
- Fox, Charles L., and Shanta M. Modak, 1974. “Mechanism of Silver Sulfadiazine Action on Burn Wound Infections.” Antimicrobial Agents and Chemotherapy 5 (6): 582–588. doi:https://doi.org/10.1128/AAC.5.6.582.
- Franz, Thomas J. 1975. “Percutaneous Absorption. On the Relevance of in Vitro Data.” The Journal of Investigative Dermatology 64 (3) Elsevier: 190–195. doi:https://doi.org/10.1111/1523-1747.EP12533356.
- Fröhlich, Eleonore, and Eva Roblegg, 2016. “Oral Uptake of Nanoparticles: Human Relevance and the Role of in Vitro Systems.” Archives of Toxicology 90 (10): 2297–2314. doi:https://doi.org/10.1007/s00204-016-1765-0.
- Gardini, D., M. Blosi, S. Ortelli, C. Delpivo, O. Bussolati, M. G. Bianchi, M. Allegri, E. Bergamaschi, and A. L. Costa, 2018. “Nanosilver: An Innovative Paradigm to Promote Its Safe and Active Use.” NanoImpact 11 (Regul. Toxicol. Pharmacol. 76 2016): 128–135. doi:https://doi.org/10.1016/j.impact.2018.06.003.
- Harris, David, and Joseph R. Robinson, 1992. “Drug Delivery via the Mucous Membranes of the Oral Cavity.” Journal of Pharmaceutical Sciences 81 (1): 1–10. doi:https://doi.org/10.1002/JPS.2600810102.
- Iurina, N. A., V. I. Pil'tiaĭ, and V. D. Protsenko, 1986. “[Optico-Structural Machine Analysis of the Nuclear Chromatin and Cytoplasmic SH-Groups of Epitheliocytes of the Oral Mucosa of Healthy Subjects and in Peptic Ulcer of the Stomach and Duodenum].” Arkhiv Anatomii, Gistologii i Embriologii 91 (10): 67–70.
- Johnston, Helinor J., Gary Hutchison, Frans M. Christensen, Sheona Peters, Steve Hankin, and Vicki Stone, 2010. “A Review of the in Vivo and in Vitro Toxicity of Silver and Gold Particulates: Particle Attributes and Biological Mechanisms Responsible for the Observed Toxicity.” Critical Reviews in Toxicology 40 (4): 328–346. doi:https://doi.org/10.3109/10408440903453074.
- Laborda, Francisco, Javier Jiménez-Lamana, Eduardo Bolea, and Juan R. Castillo, 2013. “Critical Considerations for the Determination of Nanoparticle Number Concentrations, Size and Number Size Distributions by Single Particle ICP-MS.” Journal of Analytical Atomic Spectrometry 28 (8): 1220–1232. doi:https://doi.org/10.1039/c3ja50100k.
- Lansdown, Alan B. G. 2006. “Silver in Healthcare: Its Antimicrobial Efficacy and Safety in Use.” Biofunctional Textiles and the Skin 33: 1–8.
- Lead, Jamie R., Graeme E. Batley, Pedro J. J. Alvarez, Marie-Noële Croteau, Richard D. Handy, Michael J. McLaughlin, Jonathan D. Judy, and Kristin Schirmer, 2018. “Nanomaterials in the Environment: Behavior, Fate, Bioavailability, and Effects-An Updated Review.” Environmental Toxicology and Chemistry 37 (8): 2029–2063. doi:https://doi.org/10.1002/etc.4147.
- Lee, Sungyun, Xiangyu Bi, Robert B. Reed, James F. Ranville, Pierre Herckes, and Paul Westerhoff, 2014. “Nanoparticle Size Detection Limits by Single Particle ICP-MS for 40 Elements.” Environmental Science and Technology 48 (17): 10291–10300. doi:https://doi.org/10.1021/es502422v.
- Lee, Wan Waan, and Wing Tat Chan, 2015. “Calibration of Single-Particle Inductively Coupled Plasma-Mass Spectrometry (SP-ICP-MS).” Journal of Analytical Atomic Spectrometry 30 (6): 1245–1254. doi:https://doi.org/10.1039/C4JA00408F.
- León-Silva, Sein, Fabián Fernández-Luqueño, and Fernando López-Valdez, 2016. “Silver Nanoparticles (AgNP) in the Environment: A Review of Potential Risks on Human and Environmental Health.” Water, Air, & Soil Pollution 227 (9): 1–20. doi:https://doi.org/10.1007/s11270-016-3022-9.
- Lesch, C. A., C. A. Squier, A. Cruchley, D. M. Williams, and P. Speight, 1989. “The Permeability of Human Oral Mucosa and Skin to Water.” Journal of Dental Research 68 (9): 1345–1349. doi:https://doi.org/10.1177/00220345890680091101.
- Lestari, Maria L. A. D., Joseph A. Nicolazzo, and Barrie C. Finnin, 2009. “A Novel Flow through Diffusion Cell for Assessing Drug Transport across the Buccal Mucosa in Vitro.” Journal of Pharmaceutical Sciences 98 (12): 4577–4588. doi:https://doi.org/10.1002/JPS.21772.
- Li, Jiangyan, Meng Tang, and Yuying Xue, 2019. “Review of the Effects of Silver Nanoparticle Exposure on Gut Bacteria.” Journal of Applied Toxicology : JAT 39 (1): 27–37. doi:https://doi.org/10.1002/jat.3729.
- Mauro, Marcella, Matteo Crosera, Carlotta Bianco, Francesca Bellomo, Massimo Bovenzi, Gianpiero Adami, and Francesca Larese Filon, 2015. “In Vitro Permeability of Silver Nanoparticles through Porcine Oromucosal Membrane.” Colloids and Surfaces. B, Biointerfaces 132: 10–16. doi:https://doi.org/10.1016/j.colsurfb.2015.04.061.
- Mauro. 2018. “Nanoparticles Skin Exposure and Absorption: Differences between Children and Adults.” http://paper.researchbib.com/view/paper/177545.
- Merrifield, R. C., and J. R. Lead, 2016. “Preparation and Characterization of Three-Layer, Isotopically Labelled Core-Shell Nanoparticles; a Tool for Understanding Mechanisms of Bioavailability.” NanoImpact 2: 54–60. doi:https://doi.org/10.1016/j.impact.2016.06.003.
- Merrifield, Ruth C., Chady Stephan, and Jamie Lead, 2017a. “Determining the Concentration Dependent Transformations of Ag Nanoparticles in Complex Media: Using SP-ICP-MS and Au@Ag Core-Shell Nanoparticles as Tracers.” Environmental Science & Technology 51 (6): 3206–3213. doi:https://doi.org/10.1021/acs.est.6b05178.
- Merrifield, Ruth C., Chady Stephan, and Jamie R. Lead, 2017b. “Single-Particle Inductively Coupled Plasma Mass Spectroscopy Analysis of Size and Number Concentration in Mixtures of Monometallic and Bimetallic (Core-Shell) Nanoparticles.” Talanta 162: 130–134. doi:https://doi.org/10.1016/j.talanta.2016.09.070.
- Mitrano, D. M., J. F. Ranville, A. Bednar, K. Kazor, A. S. Hering, and C. P. Higgins, 2014. “Tracking Dissolution of Silver Nanoparticles at Environmentally Relevant Concentrations in Laboratory, Natural, and Processed Waters Using Single Particle ICP-MS (SpICP-MS).” Environmental Science: Na 1 (3): 248–259. doi:https://doi.org/10.1039/C3EN00108C.
- Mitrano, Denise M., Angela Barber, Anthony Bednar, Paul Westerhoff, Christopher P. Higgins, and James F. Ranville, 2012. “Silver Nanoparticle Characterization Using Single Particle ICP-MS (SP-ICP-MS) and Asymmetrical Flow Field Flow Fractionation ICP-MS (AF4-ICP-MS).” Journal of Analytical Atomic Spectrometry 27 (7): 1131–1142. doi:https://doi.org/10.1039/c2ja30021d.
- Nicolazzo, Joseph A., Barry L. Reed, and Barrie C. Finnin, 2003. “The Effect of Various in Vitro Conditions on the Permeability Characteristics of the Buccal Mucosa.” Journal of Pharmaceutical Sciences 92 (12): 2399–2410. doi:https://doi.org/10.1002/JPS.10505.
- Park, Eun-Jung, Jongheop Yi, Younghun Kim, Kyunghee Choi, and Kwangsik Park, 2010. “Silver Nanoparticles Induce Cytotoxicity by a Trojan-Horse Type Mechanism.” Toxicology in Vitro : An International Journal Published in Association with BIBRA 24 (3): 872–878. doi:https://doi.org/10.1016/J.TIV.2009.12.001.
- Quadros, Marina E., Raymond Pierson, Nicolle S. Tulve, Robert Willis, Kim Rogers, Treye A. Thomas, and Linsey C. Marr, 2013. “Release of Silver from Nanotechnology-Based Consumer Products for Children.” Environmental Science & Technology 47 (15): 8894–8901. doi:https://doi.org/10.1021/es4015844.
- Roblegg, Eva, Eleonore Fröhlich, Claudia Meindl, Birgit Teubl, Michaela Zaversky, and Andreas Zimmer, 2012. “Evaluation of a Physiological in Vitro System to Study the Transport of Nanoparticles through the Buccal Mucosa.” Nanotoxicology 6 (4): 399–413. doi:https://doi.org/10.3109/17435390.2011.580863.
- Schneider, Gregor 2017. “Antimicrobial Silver Nanoparticles – Regulatory Situation in the European Union.” Materials Today: Proceedings 4: S200–S207. doi:https://doi.org/10.1016/j.matpr.2017.09.187.
- Silver - Registration Dossier - ECHA n.d. https://echa.europa.eu/it/registration-dossier/-/registered-dossier/16155/7/1.
- Singh, C., S. Friedrichs, M. Levin, R. Birkedal, K. a Jensen, G. Pojana, and W. Wohlleben. 2011. NM-Series of Representative Manufactured Nanomaterials Characterisation and Test Item Preparation. doi:https://doi.org/10.2787/55008.
- Stepien, Karolina M., Rob Morris, Sandy Brown, Andrew Taylor, and Linda Morgan, 2009. “Unintentional Silver Intoxication following Self-Medication: An Unusual Case of Corticobasal Degeneration.” Annals of Clinical Biochemistry 46 (Pt 6): 520–522. doi:https://doi.org/10.1258/acb.2009.009082.
- Tang, Song, Wang, Mao, Kaylyn E. Germ, Hua Mao Du, Wen Jie Sun, Wei Min Gao, and Gregory D. Mayer, 2015. “Health Implications of Engineered Nanoparticles in Infants and Children.” World Journal of Pediatrics : WJP 11 (3): 197–206. doi:https://doi.org/10.1007/s12519-015-0028-0.
- Tejamaya, Mila, Isabella Römer, Ruth C. Merrifield, and Jamie R. Lead, 2012. “Stability of Citrate, PVP, and PEG Coated Silver Nanoparticles in Ecotoxicology Media.” Environmental Science and Technology 46 (13): 7011–7017. doi:https://doi.org/10.1021/es2038596.
- Toto, Patrick D. 1965. “The Relation of Acid Mucopolysaccharides and Sulfhydryl Groups to Diseased Oral Mucosa.” Oral Surgery, Oral Medicine.” Oral Surgery, Oral Medicine, and Oral Pathology 20 (5): 600–606. doi:https://doi.org/10.1016/0030-4220(65)90104-0.
- Trop, Marija, Michael Novak, Siegfried Rodl, Bengt Hellbom, Wolfgang Kroell, and Walter Goessler, 2006. “Silver-Coated Dressing Acticoat Caused Raised Liver Enzymes and Argyria-like Symptoms in Burn Patient.” The Journal of Trauma 60 (3): 648–652. doi:https://doi.org/10.1097/01.ta.0000208126.22089.b6.
- Tulve, Nicolle S., Aleksandr B. Stefaniak, Marina E. Vance, Kim Rogers, Samuel Mwilu, Ryan F. LeBouf, Diane Schwegler-Berry, Robert Willis, Treye A. Thomas, and Linsey C. Marr, 2015. “Characterization of Silver Nanoparticles in Selected Consumer Products and Its Relevance for Predicting children's potential exposures.” International Journal of Hygiene and Environmental Health 218 (3): 345–357. doi:https://doi.org/10.1016/j.ijheh.2015.02.002.
- U.S. Environmental Protection Agency Chemical Assessment. 1991. “Silver CASRN 7440-22-4 |IRIS|US EPA, ORD.” https://iris.epa.gov/ChemicalLanding/&substance_nmbr=99.
- Visual MINTEQ – Visual MINTEQ – a Free Equilibrium Speciation Model. 2021. Accessed January 12. https://vminteq.lwr.kth.se/.
- Wertz, P. W., and C. A. Squier, 1991. “Cellular and Molecular Basis of Barrier Function in Oral Epithelium.” Critical Reviews in Therapeutic Drug Carrier Systems 8 (3): 237–269.