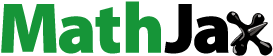
Abstract
Pembrolizumab has shown significant anticancer effects against various human cancers. The present study investigated the effects of pembrolizumab liposome and nano (naked) forms in treated lymphocytes from head and neck squamous cell carcinoma (HNSCC) patients compared to healthy individuals. The level of oxidative DNA damage induced by hydrogen peroxide (H2O2) was also investigated. A concentration of 10 µg/ml of pembrolizumab liposome was used to treat the lymphocytes in the Comet and micronucleus assays based on the preliminary dosage optimization tests. To determine the cellular pathways involved in the protective role of pembrolizumab against H2O2, several proteins involved in apoptosis (P53, P21 and Bcl-2) were assessed. Pembrolizumab significantly reduced DNA damage and decreased the number of micronuclei in lymphocytes from HNSCC patients (p < 0.01) compared with healthy individuals. The 10 µg/ml of pembrolizumab liposome significantly reduced the oxidative stress induced by H2O2 and was effective in healthy and HNSCC groups using the Comet and micronucleus assays (p < 0.001). To our knowledge, this is the first report of pembrolizumab in liposome and naked forms exhibiting a protective effect on DNA damage in the treatment of HNSCC patients.
Introduction
Head and Neck Cancer refers to a heterogenous group of tumors originating mainly from the mucosa of the upper aerodigestive tract, along with cancers of the salivary and thyroid glands (Johnson et al. Citation2020). Cancers arising from the upper aerodigestive tract are squamous cell in origin and termed Head and Neck Squamous Cell Carcinoma (HNSCC). HNSCC is a critical one among the various cancers and approximately 600,000 cases of HNSCC are diagnosed on an annual basis (Bray et al. Citation2018). The majority of the cases of squamous cell carcinomas that are diagnosed are located in the upper aero-digestive tract (Argiris, Ferris, and Rosenthal Citation2018).
HNSCC is like other cancers related to prolonged exposure to carcinogens and often have a high burden of mutations. The burden of HNSCC has commonly been associated with exposure to tobacco derived carcinogens, excessive alcohol consumption, and linked to prior infection in human oncogenic strains (Michaud et al. Citation2014). Oxidative stress alterations have been well established as a particular important factor that causes tumors of the head and neck, as these are strongly associated with oxidative damage and oxidative stress, with tobacco and alcohol described as major etiological factors. Increased rates of reactive oxygen species (ROS) have also been detected in almost all cancer cells, where they promote many aspects of tumor progression and development (Dequanter, Dok, and Nuyts Citation2017).
To effectively treat cancer, it is critical to understand its origin and how it evolves by understanding the genetic damage that can occur to ensure that effective and efficient treatment approaches are employed to prevent further damage (Alhmoud et al. Citation2020). Over the course of the last two decades, anti-cancer drugs have significantly evolved and developed to be specifically directed at tumors and metastases without causing any damage to healthy cells.
Multiple types of cancer have been shown to disable the body from destroying cancer cells through producing proteins that relate to bind to programmed death-1 (PD-1) (Francisco, Sage, and Sharpe Citation2010). The PD-1 pathway plays an important and peripheral immunosuppressive role by regulating multiple signaling pathways. However, anti- PD-1 immunotherapy has become the new standard in the treatment of patients with metastatic head and HNSCC.
Pembrolizumab (P) also known as Keytruda, is a curing antibody known to bind and inhibit PD-1 that is found on lymphocytes and has been used as a treatment option against different forms of cancer. Pembrolizumab targeting and blocking the PD-1 on the surface of immune T-cells. When PD-1 is blocked, the T-regulatory cells are stimulated to locate and eliminate cancer cells by preventing them from further developing and dividing. As such, Pembrolixumab essentially prompts the immune system to attack cancer cells. Interaction with PD-ligand 1 (PD-L1) and PD-1 has essential role to play in facilitating a tumor immune response (Toor et al. Citation2018). Moreover, based on report, Pembrolizumab was twice as effective as the standard therapy of cetuximab, which was the only approved targeted therapy for head and neck cancer at the time (Seiwert et al. Citation2015). Pembrolizumab prolonged overall survival compared with investigator’s choice of cetuximab in patients with recurrent or metastatic squamous cell carcinoma of the head and neck. The benefit of pembrolizumab compared with standard-of care therapy was greater in patients with PD-L1 expression on their tumors or in the tumor microenvironment than in those without PD-L1 expression. Pembrolizumab had a better safety profile than standard of care, with overall profiles consistent with those previously observed and no new or unexpected toxicities. The frequency of adverse events of grade 3–5 severity that were attributed to study treatment by the investigator was 2·7 times lower with pembrolizumab than with standard of care (Cohen et al. Citation2019). Liposomes are artificial vesicles synthesized from naturally derived phospholipids, with spherical, self-assembled shapes and their sizes typically range from tens of nanometers to hundreds of micrometers depending on their preparation methods and final use (Shah et al. Citation2020). They are made from nontoxic surfactants, cholesterols, and membrane proteins. Liposomes have extensively been utilized as a drug delivery system (DDS) and for biomedical and biotechnological purposes (Frick, Schwieger, and Schmidt Citation2021). The pembrolizumab liposome used as DDS in this study due to the limitation in the use of the naked pembrolizumab such as rapid biodegradation and clearance, which is reducing its efficacy. In our study, we investigate the protective effects of naked pembrolizumab and liposome on H2O2 induced oxidative stress in isolated lymphocyte cells from healthy individuals and HNSCC patients.
Materials and methods
Pembrolizumab (Cat No. 1374853-91-4), Mitomycin C (MMC) (Cat No. M0503) and fetal bovine serum (FBS) (Cat No. F7524) were purchased from Sigma-Aldrich (Gillingham, UK). RPMI 1640 Medium (Cat No. R8758), phytohaemagglutinin (PHA) (Cat No. L1668) were also obtained from Sigma-Aldrich. All other chemicals used in the different tests were from Sigma-Aldrich Company Ltd. (Sigma Chemical Ltd., Gillingham, UK). Prior to use, the pembrolizumab was re-constructed in sterile distilled water to prepare the stock solution. The concentrations-response experiments were carried out to determine the optimal doses of naked pembrolizumab and liposome and H2O2 used throughout the study. A fixed- concentration of both forms (10 µg/ml of naked pembrolizumab and 10 µg/ml of pembrolizumab liposome) were used as treatment. The classic genotoxic compound H2O2 (75 μM) was used as a positive control (PC).
Preparation and characterization of liposomes
Liposomes were prepared using the thin film rehydration method. 1,2-Dipalmitoyl-sn-glycero-3- phospho-rac-(1-glycerol) sodium salt (DPPG), 1,2-dioleoyl-sn-glycero-3-phosphoethanolamine (DOPE) and cholesterol were dissolved in dichloromethane (DCM) and methanol (3:1 v/v). The solution was then transferred to a rotary evaporator with a 25 mL round-bottomed flask. The organic solvent mixture was evaporated at 40 °C under vacuum for 6 h, to ensure no traces of the organic solvents were left. The thin film was then hydrated by 10 mL of PBS solution of pembrolizumab for 1 h at 60 °C using a bath sonicator (150 W). The sample was then subjected to four repeated freeze–thaw cycles (freezing at −20 °C and thawing at 60 °C in the bath sonicator). Furthermore, the blank liposome was prepared from the same components without adding the pembrolizumab. The average size and polydispersity index (PDI) of the liposome preparations were determined by dynamic light scattering (DLS) using Zetasizer ZS (Malvern Instruments, UK). All measurements were performed in triplicate.
Ethical approval
Ethical approval was obtained from Leeds East Research Ethics Committee (REC number: 12/YH/0464), the University of Bradford Research Ethics Sub-Committee on Research in Human Subjects (Ref: 0405/8) and the Research Support and Governance office, Bradford Teaching Hospitals, NHS Foundation (Ref: RE DA 1202). Blood samples for the study. All peripheral blood was collected after the completion of informed consent form. Blood samples from 20 healthy nonsmoking volunteers and 20 HNSCC patients by venepuncture were collected.
The characteristics of patients and healthy individuals are shown in .
Table 1. The characteristics of patients and healthy individuals are shown in Table 1.
Cell viability
Cell Counting Kit-8 (CCK-8) was used to determine the cell viability. The cells were seeded in 96-well plates at a density of 5000 cells/well and preincubated for 24 h. The cells were cultured with10µg/ml pembrolizumab and pembrolizumab liposome. Cells cultured in medium without the addition of pembrolizumab or pembrolizumab liposome were used as the negative control. After 24 h of incubation, the culture medium was replaced with 100 µL of CCK-8 solution diluted in culture medium at a ratio of 1:10 (V/V) and incubated for an additional 4 h at 37 °C. Absorbance was measured at 450 nm using a Microplate reader MRX II (Dynex Technologies, Chantilly, USA). Viability was also measured by the trypan blue exclusion test indicating intact cell membranes.
Lymphocyte isolation from whole blood
Blood was mixed in a 1:1 ratio with 0.9% normal saline and mixed gently. In a Falcon tube, diluted blood (6 mL) was carefully overlaid onto 3 mL of Lymphoprep, and the sample was transferred to the centrifuge. After centrifugation at room temperature (RT) and 600 × g for 20 min, the white layer of lymphocytes was obtained from the top of the Lymphoprep, within the plasma interface of the sample, and washed with saline in a universal tube. Then, the suspension was centrifuged at 375 × g for 15 min at RT, and the supernatant was treated with 2% Virkon before being discarded. The pellet was resuspended in 900 μL of fetal bovine serum (FBS), transferred to an Eppendorf tube containing 100 μL of DMSO. The tubes were tightly closed, mixed, then stored overnight at −20 °C before being transferred to a − 80 °C freezer for prolonged storage.
Comet assay
Lymphocytes were treated with PC of 75 μM H2O2, blank liposome, 10 µg/ml of pembrolizumab and pembrolizumab liposome in order to test the effect of pembrolizumab in a highly oxidizing environment; 10 µg/ml of pembrolizumab and pembrolizumab liposome were treated in the presence of 75 mM H2O2). An untreated sample was used as a negative control. The Comet assay was prepared as previously defined (Anderson et al. Citation2014). In brief, 100 μL of whole blood samples were incubated for 30 min at 37 °C with different test agents, to make the final volume of 1000 μL with RPMI- 1640 Medium (with sodium bicarbonate, without L-glutamine, liquid, sterile-filtered, suitable for cell culture. The samples were then incubated at 37 °C for 30 min. Following incubation, the samples were centrifuged at 3000 rpm for 5 min. Next, 100 µL of 0.5% low melting point (LMP) agarose was added and the mixture was resuspended, then 100 µL aliquots of this solution were applied in duplicate to individually labeled slides that had been coated with 1% normal melting point (NMP) agarose. The samples were covered with cover slips. The slides were immersed in lysis solution then electrophoresis was performed. The slides were covered with neutralizing buffer. The slides were coded before scoring; per slide, 100 nuclei were scored using a fluorescent microscope at 20× magnification. This was connected to a CCD camera using Komet 6 software and Kinetic Imaging (Andor Technology Ltd, Belfast). Olive tail moment (OTM) and % tail DNA were used simultaneously to decrease variability in the results.
Micronucleus assay
Five fresh blood samples from healthy and patient groups were supplemented with PHA, basic culture medium (RPMI 1640 with 25 mM HEPES and L-Glutamine), 15% FBS and 1% penicillin-streptomycin. The suspensions were left for incubation for 24 h at 37 °C in the presence of 5% CO2. In the next 24 hours, the lymphocytes from healthy individuals and HNSCC patients were treated with different treatment groups including the 50 μL of blank liposome was added to the negative control. Two positive controls were used with 10 μL of MMC (0.4 μM) used as PC 1, and 50 μL of H2O2 served as PC 2. 50 μL of 10 µg/ml of pembrolizumab and 10 µg/ml of pembrolizumab liposome were added to their respective flasks and the final flask contained 10 µg/ml of naked pembrolizumab and 10 µg/ml of pembrolizumab liposome with H2O2. 50 μL of cytochalasin-B (6 μg·mL−1, Sigma) was added to each flask and cultured at 37 °C in the presence of 5% CO2 for 44 h. The CBMN procedures were performed as described by FENECH and coworkers (Fenech Citation2007).
RNA isolation, cDNA synthesis and reverse transcriptase PCR (RT-PCR)
Total RNA was isolated from human lymphocytes using RNeasy Mini Kit (QIAGEN), cDNA was synthesized using iScript cDNA Synthesis Kit (Bio-Rad) following manufacturer’s protocol. Quantitative PCR for determining transcripts of P53, P2, Bcl-2 and β-Actin were performed using SYBR Green Supermix (Applied Biosystems) on step one plus real-time PCR detection system (Applied Biosystems, Warrington, UK) according to the manufacturer’s protocol.
Western blots analysis
Isolated human lymphocytes were cluttered at density 1x106 cells/ml in six-well plates with RPMI 1640 medium, were then washed with PBS and lysed with 1X radio immunoprecipitation assay buffer (RIPA, Sigma) containing protease inhibitor cocktail on ice for 5 min. The total protein solutions were collected by centrifugation at 14,000 rpm for 15 min at 4 °C. The concentrations of protein were determined by the Bio-Rad Bradford assay kit. The protein suspension was separated on a 12% SDS-PAGE gel were transferred to a blotting nitrocellulose membrane for 7 minutes at a constant voltage of 25 V using the iBlot® Gel Transfer Device (Invitrogen). The membrane was incubated with blocking buffer (1X TBS, 0.1% Tween-20 with 5% w/v nonfat dry milk). Membranes were incubated with anti-P53 (1: 1,000 dilution), P21 (1: 1,000 dilution), Bcl-2 (1: 1,000 dilution) and anti-GAPDH (1:1000 dilution) antibodies following the manufacturer’s protocols. The membranes were incubated with anti-Rabbit IgG H&L (HRP) secondary antibodies (Abcam) after incubation with primary antibodies. A chemiluminescent substrate kit (ECL Plus Western blotting Detection Reagents, GE Healthcare) was used for HRP detection on the immunoblots. The relative band intensities according to the band of GAPDH were calculated by the software of Image Lab (Bio-Rad Laboratories).
Statistical analysis
The final data were expressed as mean values with standard errors, the results were analyzed using one-way ANOVA with Dunnett’s multiple comparisons test using GraphPad Prism 8.1.2. p-values were considered significant at p*<0.05, p**<0.01, p***<0.001.
Results
The viability of isolated human lymphocytes from healthy individuals and HNSCC patients in the presence of 10 µg/ml pembrolizumab or pembrolizumab liposome and 75 µM H2O2 in the culture medium was determined by detecting the activities of intracellular dehydrogenases (CCK-8 assay). The viability of lymphocytes from healthy individuals and HNSCC patients treated with different treatments was confirmed higher than 80% after 24 h treatment .
Figure 1. Cell survival rates of lymphocytes healthy individuals and HNSCC patients incubated with various treatments of 10 µg/ml pembrolizumab or pembrolizumab liposome and 75 µM H2O2, respectively. Error bars show mean ± SEM, n = 3. All the results for various treated groups were compared against the respective negative control group. NS: nonsignificant. *p < 0.05; **p < 0.01. *** p < 0.001.
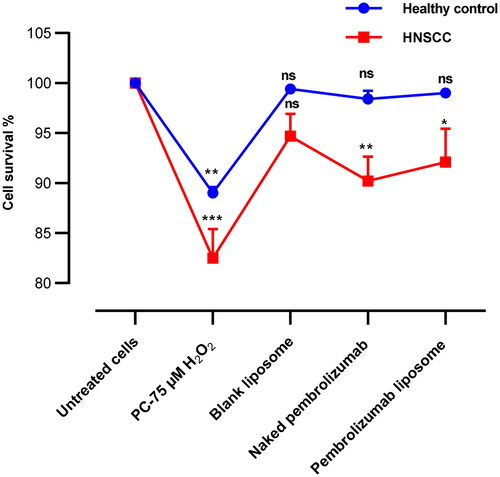
Encapsulation efficiency determination of pembrolizumab liposome
The detection of the pembrolizumab was carried out using RP-HPLC, and the retention time of pembrolizumab was 1:5 minutes as shown in the chromatogram. To measure the encapsulation efficiency of the liposomes, they were centrifuged for 30 minutes at 23 g at 4 ̊C. The supernatant was then injected in the HPLC. The encapsulation efficiency was calculated from the amount of free drug pembrolizumab in the supernatant. A calibration curve of the peak area of pembrolizumab vs. concentration in the range of 10 µg/ml to 80 µg/ml was produced. The regression equation of the line was y = 900.88X = 10172 with a correlation coefficient R2 = 0.9949 as presented in Error! Reference source not found. The peak was detected at 1.5 minutes. The encapsulation efficiency was determined by measuring the free pembrolizumab after centrifuging the sample. Area under the peak for the sample was 16601. Therefore, the concentration was: Concentration= (16601-10172)/900.88 = 7.13 µg/ml
Comparison of DNA damage by the Comet assay using naked pembrolizumab and liposome version
We next examined the effect of naked pembrolizumab and liposome version on isolated lymphocytes from healthy individuals and HNSCC patients after treated with different treatment groups using the Comet assay. Results from Olive tail moment (OTM) and % tail DNA showed that the PC group 75 µM of H2O2 has caused a significant level of DNA damage however, blank liposome and naked pembrolizumab have shown no significant difference compared to the untreated control. The pembrolizumab Liposome (10 µg/ml) by using the % tail DNA and OTM, has indicated a significant decrease in DNA damage comparable to the negative control (p < 0.01) and (p < 0.001) respectively. Supplementation of naked pembrolizumab and pembrolizumab liposome with H2O2 (75 µM), individually, has also showed a significant reduction in DNA damage compared to the PC. The results showed that both forms of pembrolizumab do not cause DNA damage in lymphocytes from HNSCC patients at basal levels. The pembrolizumab liposome has reduced this damage and has also shown more protective effect against the H2O2 damage in HNSCC patient group and .
Figure 2. Comparison between the effect of naked and pembrolizumab liposome in lymphocytes from healthy individuals and HNSCC patients using OTM. Cells were treated with different various treatment groups including an untreated lymphocyte group (NC), positive control (pc) 75 µM H2O2, blank liposome, naked pembrolizumab 10 µg/ml, pembrolizumab liposome 10 µg/ml, naked pembrolizumab 10 µg/ml + 75µM H2O2, and pembrolizumab liposome 10 µg/ml +75µM H2O2. All treatment groups were compared against the respective negative control. Each treatment group from both groups was also compared against each other, represented by the horizontal lines on the graph and the statistics on top of these lines. NS: nonsignificant. *p < 0.05; **p < 0.01 ***p < 0.001.
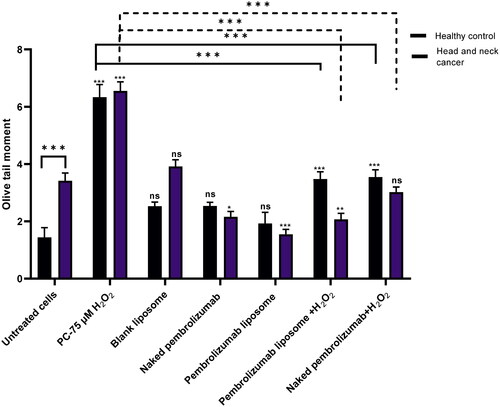
Figure 3. Comparison between the effect of naked and pembrolizumab liposome in lymphocytes from HNSCC patients and healthy individuals using % tail DNA. Cells were treated with different various treatment groups including an untreated lymphocyte group (nc), positive control (PC) 75 µM H2O2, blank liposome, naked pembrolizumab 10 µg/ml, pembrolizumab liposome 10 µg/ml, naked pembrolizumab10µg/ml + 75µM H2O2, and pembrolizumab liposome 10 µg/ml + 75µM H2O2. All treatment groups were compared against the respective negative control. Each treatment group from both groups was also compared against each other, represented by the horizontal lines on the graph and the statistics on top of these lines. NS: nonsignificant. *p < 0.05; **p < 0.01. ***p < 0.001.
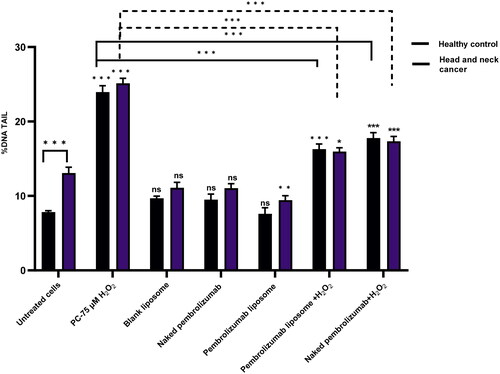
This study further demonstrated that none of the healthy volunteers − 100% of whom did not smoke – no presented confounding effects from smoking; however, the HNSCC patients clearly showed smoking-related DNA damage. The age-related confounding effect on DNA damage among the healthy versus patient groups showed significant variation. While this was linked to the sample limitations during the experiment, the result presented that DNA damage in patients with HNSCC in age ≥50 group is higher than in (20-40) healthy group. A previous study showed that damage to the DNA relates to age (Chen et al. Citation2020). Following the treatment of the lymphocytes of groups with naked and liposome pembrolizumab forms, statistically no significant differences were found among the different ages.
MNi in binucleated cells (BiNC)
Overall, the number of MNi in BiNC from HNSCC patients was way greater than those from healthy individuals in untreated cells. Both forms of pembrozinumab, naked and liposome, have induced non-significant increase in number of MNi in binucleated cells of healthy individuals and a significant decrease in MNi frequency in binucleated from HNSCC patients when compared to untreated cells. The PC groups, 0.4 µМ mitomycin (MMC) and 75 µМ of H2O2 have induced a significant increase in the number of MNi in BiNC in both the healthy and patient groups. However, co-administration of naked pembrolizumab (10 µg/ml) and liposome form of pembrolizumab effectively decreased the number of H2O2-induced MNi in BiNC of healthy individuals and patient group compared to 75 µМ of H2O2 (p < 0.001) Regardless of the groups, naked pembrolizumab significantly decreased the MNi number in binucleated cells whereas liposome pembrolizumab has demonstrated better effects in reducing the chromosomal damage at basal levels .
Figure 4. The average of BiNC MNi scored per 1000 cells from 3 individual experiments. Data are expressed as means ± SEM. Eight treatment groups included the negative control, a positive control 1(Mitomycin C, 0.4 µM MMC), positive control 2 (PC, 75 µM H2O2), naked pembrolizumab, naked pembrolizumab 10 µg/ml and 75 µM H2O2, pembrolizumab liposome, pembrolizumab liposome 10 µg/ml and 75 µM H2O2, blank liposome. All treatments were compared to their respective negative control. Each treatment group from both groups were also compared against each other, represented by the horizontal lines on the graph and the statistics on top of these lines. NS: nonsignificant. *p < 0.05; **p < 0.01. ***p < 0.001.
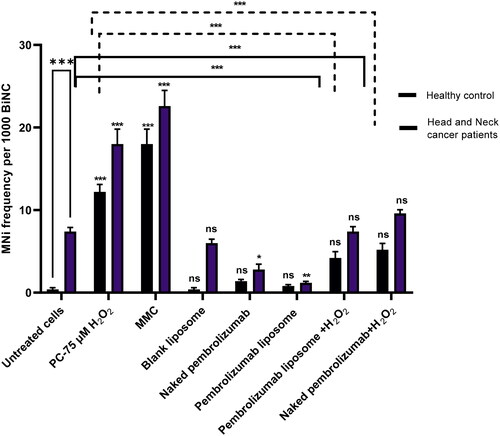
Figure 5. Level of P53, P21 and BCL2 and in lymphocytes from healthy individuals(n = 3). The data were compared against the control group and normalized against the house gene, GAPDH. Three groups included a control group, naked pembrolizumab 10 µg/ml and pembrolizumab liposome 10 µg/ml. There was no significant effect seen on P53 and P21and BCl2 expressions (ns = not significant).
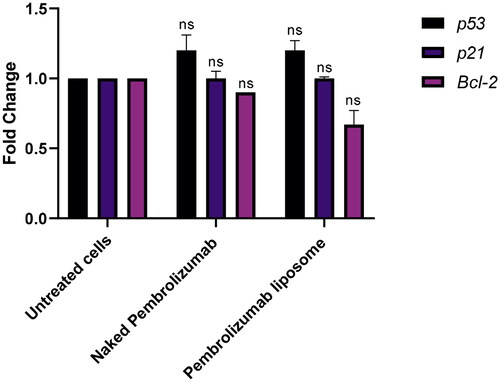
Figure 6. The effect of pembrolizumab naked and liposome forms on the gene expression levels of P53, P21 and BLC2 in lymphocytes from HNSCC patients (n = 3). The data were compared against the control group and normalized against the house gene, GAPDH. Three groups included a control group, naked pembrolizumab 10 µg/ml and pembrolizumab liposome 10 µg/ml. P53 and P21 genes were significantly upregulated; however, the BLC2 gene was significantly downregulated. (ns = not significant, *P < 0.01, **P < 0.001).
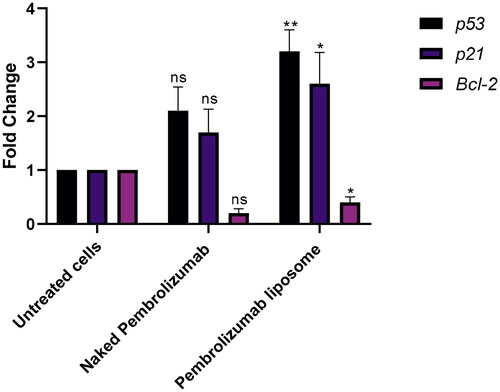
There are numerous cytological scoring parameters, including biomarkers of cell mitotic division such as mononucleated cells (MoNC), binucleated cells (BiNC), and multinucleated cells (MultiNC). From these parameters, the nuclear division index (NDI) was calculated for healthy individuals and HNSCC patients. The mean values for the NDI for all treatments were within the normal range, as presented in .
Table 2. Chromosomal instability parameters in the form of the mean of NBUDs, NPBs per 1000 BiNC and MNi frequency in MoNC. The frequency of MNi in BiNC of untreated cells was clearly higher than in lymphocytes treated with pembrolizumab liposome on HNSCC patients.
Pembrolizumab activates the P53 signaling pathway independent of DNA damage in lymphocytes
Results from the Comet and micronucleus assays showed that various concentrations of pembrolizumab do not induce statistically significant genotoxicity in lymphocytes from HNSCC patients and in those from healthy individuals. We determined the optimal concentration of pembrolizumab (10 µg/ml) to be used for subsequent studies. We then determined the effect of pembrolizumab liposome (10 µg/ml) compared to the naked form at the same concentration. Compared to the naked form, results piloted more effective and protective effects of liposome form using the Comet and micronucleus assays.
To understand the rationale behind this effect we further studied some key factors involved in the cell cycle regulation and apoptotic pathways. We determined the effects of pembrolizumab on gene expression levels of P53, P21 and Bcl-2, in lymphocytes from HNSCC patients and from healthy individuals at the mRNA level. The qPCR technique was used to analyze the expression of these genes. The qPCR data showed no effect on these genes in both forms of pembrolizumab at a same concentration of 10 µg/ml in lymphocytes from healthy individuals (). In contrast, the qPCR data revealed significant upregulation of the P53 and P21 genes, while the BLC2 gene showed a significant downregulation when assessing the impact of pembrolizumab in both its naked and liposome forms on gene expression levels of P53, P21, and BLC2 in lymphocytes from HNSCC patients (ns=not significant, *P<0.001) ().
Effect of naked and liposome pembrolizumab on protein quantitation in lymphocytes from HNSCC patients
To further explore the mechanism of the effect of naked pembrolizumab 10 µg/ml and pembrolizumab liposome on P53, P21 and Bcl-2 protein expression levels in lymphocytes from healthy individual and HNSCC patient was assessed by Western blot. The results showed that naked and liposome pembrolizumab has a non-significant effect on expressing any of these proteins in healthy individuals’ lymphocytes . In contrast, there was significant increase of P53 and P21 expression levels in lymphocytes from HNSCC patients . The P53 has increased by 1.7-fold with naked pembrolizumab 10 µg/ml and pembrolizumab liposome 10 µg/ml in lymphocytes from HNSCC patients. Furthermore, the P21 has increased to 1.8-fold for naked pembrolizumab 10 µg/ml and 1.7 for liposomal form compared to the control group.
Figure 7. A, B. The effect of naked and liposome pembrolizumab on the protein expression levels of P53, P21 and BCL2 in lymphocytes from healthy individuals(n = 3). All data from the treatment groups were compared against the control group (C) and normalized against the internal home protein, GAPDH, untreated cells, naked pembrolizumab 10 µg/ml and pembrolizumab liposome 10 µg/ml. Pembrolizumab in both forms did not show any significant effect on P53, and BCL2 protein expression (ns = not significant), (A) Immunoblot analysis of the P53, P21 and BCL2 proteins in lymphocyte from healthy individuals treated with naked pembrolizumab 10 µg /ml and pembrolizumab liposome 10 µg/ml. (B) Bar graphs exhibiting fold changes in protein expression levels. Data are represented as the mean ± SEM of three experiments.
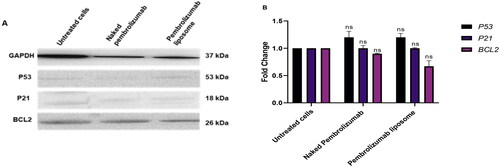
Figure 8. A, B. The effect of naked pembrolizumab 10 µg/ml and pembrolizumab liposome 10 µg/ml on the protein expression levels of P53, P21 and BCL2 in lymphocytes from HNSCC patients(n = 3). All data from the treatment groups were compared against the control group (C) and normalized against the internal home protein, GAPDH, untreated cells, naked pembrolizumab 10 µg/ml, and pembrolizumab liposome 10 µg/ml. Pembrolizumab in both forms significantly upregulated the protein expression levels of P53 and P21; however, the regulation of BCL2 expression was insignificant. (A) Immunoblot analysis of the P53, P21 and BCL2 proteins in lymphocyte from HNSCC patients treated with naked pembrolizumab 10 µg/ml and pembrolizumab liposome 10 µg/ml. (B) Bar graphs exhibiting fold changes in protein expression levels. Data are represented as the mean ± SEM of three experiments. (ns = not significant, ***P < 0.001).
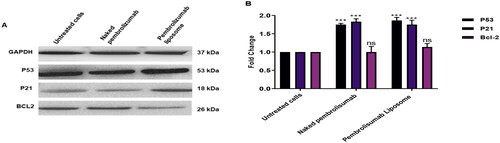
The results have shown that naked pembrolizumab 10 µg/ml and pembrolizumab liposome 10 µg/ml can fight the cancer cells by stimulating the P53 expression levels and P21. It will have a protective and antitumor effect by stimulating DNA damage response (DDR). Thus, it will contribute to survival through the repair of DNA damage. Furthermore, results from WB were consistent with the results of gene expression from the qPCR.
Discussion
Treatment of head and neck squamous cell carcinoma is currently a therapeutic challenge. The treatment options consist of surgery, radiotherapy plus or minus chemotherapy. Early stage tumors can be treated by single modality such as primary surgery or radiotherapy alone. Later stage HNSCC is usually treated by multimodal therapy, a combination of surgery plus radiotherapy with or without chemotherapy. Chemotherapy functions by generating DNA damage. Though such treatments generate dose limiting toxicities in normal tissues, they are often efficacious. Different in vivo and in vitro studies have presented the promising applications of pembrolizumab in the field of health to treat inoperable or metastatic melanoma, meta-static non-small cell lung cancer (NSCLC) in certain cases (Goldberg et al. Citation2016), a first-line treatment for metastatic bladder cancer in patients who cannot receive cisplatin-based chemotherapy and have high levels of PD-L1(Sundahl et al. Citation2018), as a second-line treatment for HNSCC, as the treatment of pediatric and adult patients with refractory classical Hodgkin lymphoma (CHL), and recurrent locally advanced or metastatic esophageal squamous cell carcinoma (Sundahl et al. Citation2018).
The pembrolizumab was approved by Food and Drug Administration (FDA) in 2017 for any unresectable or metastatic solid tumor with certain genetic anomalies such as mis-match repair deficiency or microsatellite instability (Sung, Chow, and Cho Citation2021). The FDA approved pembrolizumab for high-risk, early-stage, triple-negative breast cancer (TNBC) combined with chemotherapy as neoadjuvant treatment, and then used as a single agent as adjuvant treatment after surgery (Schmid et al. Citation2020; Sung, Chow, and Cho Citation2021).
This study is the first to investigate the effect of naked pembrolizumab and pembrolizumab liposome on DNA damage and expression levels of P53, P21 and Bcl-2 genes and proteins in human lymphocytes originating from 20 healthy individuals and 20 HNSCC patients. Our study shows that the treated lymphocytes from healthy individuals with naked pembrolizumab and pembrolizumab liposome did not induce significant DNA damage compared to the untreated cells ( and ). In contrast, naked and liposome of the pembrolizumab showed a significant reduction in the values of OTM and %tail DNA in HNSCC patients’ lymphocytes compared to the untreated cells (p < 0.01) and (p < 0.001) respectively. The OTM showed greater reduction in DNA damage by the pembrolizumab liposome than the naked pembrolizumab (p < 0.05). The basal DNA damage of the percent tail DNA induced by naked pembrolizumab showed no significant reduction, while liposomal showed significant reduction (p < 0.01) ( and ). In agreement with previous study where pembrolizumab liposome has often demonstrated superior protective benefits to naked form, the larger particle analogue. This might occur as a result of numerous therapeutic substances, including proteins and nucleotides, being stabilized by the increased solubility of liposome-coated pembrolizumab in an aqueous media. Their composition, size, and surface charge all contribute to their successful outcomes (Bozzuto and Molinari Citation2015). Liposomes can enclose a variety of hydrophilic and hydrophobic biomolecules, shielding them from contact with the outside world. In addition to preventing the drug’s contact with healthy cells, liposomes prevent the drug’s metabolism before it reaches the target cells (Schmid et al. Citation2020).
To determine if a pembrolizumab liposome and naked form is genotoxic, we further evaluated the presence of micronuclei of the chromosome fragments produced from DNA breakage and whole chromosomes produced by disruption of the mitotic apparatus. In this study, specific consideration was made for the presence of MNi in the treatment groups because MNi are indicators of chromosomal breakage, loss, rearrangement, necrosis, and apoptosis. MNis are cytoplasmic bodies generated as a consequence of disorders during cell cycle division. Subsequently, when the centric fragments or whole chromosomes are incapable of traveling to opposite poles during anaphase, the nuclear envelope forms around the lagging chromosomes and fragments, which gradually take on the morphology of an interphase nucleus, which is smaller than the main nuclei in the cell (Fenech Citation2007). Furthermore, MNi in BiNC only showed the damage after treatment, which decreased the probability of scoring the preexisting damage (Li et al. Citation2012).
Results showed that the frequency of MNi in the lymphocytes from healthy individuals and HNSCC patients decreased when treated with naked pembrolizumab and liposome pembrolizumab compared to the untreated cells from each group. Whereas the liposome pembrolizumab showed a significant re-duction in MNi frequency in lymphocytes more than the naked pembrolizumab . The NDI values were normal for all experiments (). Results also showed that naked pembrolizumab and the liposome form effectively protects DNA damage and reduced the frequency of MNis compared lymphocytes from healthy individuals and HNSCC patients form H2O2-induced oxidative stress. Interestingly, the liposome pembrolizumab had exhibited higher reduction in DNA damage more than naked pembrolizumab.
Expression of P53 is the most frequent molecular event in human cancers. Many commonly lost tumor-suppressor genes, including P53 and P21 function in DNA damage response and/or DNA repair pathways, which are among the most often involved pathways in human cancers (Kulaberoglu, Hergovich, and Gómez Citation2021). The underlying mechanisms by which liposome and naked pembrolizumab elicit P53, P21 and Bcl-2 genes and proteins expressions was also investigated. Results showed that no genetic effects were seen on P53, P21 and Bcl-2 at the level of gene and protein expression in lymphocytes from healthy individuals. In contrast, significantly decreased levels of P53, P21 and Bcl-2 were seen in lymphocytes from HNSCC patients (*P < 0.01, **P < 0.001). The major findings of this study suggest that tumor cell apoptosis is strongly inhibited by P53 and P21 and influenced by Bcl-2 which, presumably, inhibits tumor cell apoptosis.
In general, liposome pembrolizumab (10 µg/ml) has shown better protective effects than its larger particle counterpart, naked form at concentration. This is possibly due to liposome-coated pembrolizumab’s induced solubility in an aqueous medium stabilizing different therapeutic agent such as nucleotides and proteins. Their size, surface charge and composition are favorable conditions for their effective results. Many hydrophilic and hydrophobic biomolecules can be encapsulated in liposomes and protected from interactions with the external environment.
Liposomes induce the drug’s therapeutic index through the inhibition of the drug’s metabolism before reaching the target cells and protect the drug’s interaction with the healthy cells (Alhawmdeh et al. Citation2021). It has been well known that the liposome form of any therapeutic drug shows enhanced pharmacokinetic properties and biodistribution than its naked form. The morphology of liposomes is similar to that of the cellular membranes, making them an ideal drug carrier system (Barenholz and Peer Citation2012). Multi-functional liposomes have been developed for diagnosis and therapy of disease simultaneously have been considered the next generation of nano-therapeutics. Such liposomes have been discovered and are at an early research stage for PC treatment (Deng et al. Citation2012) and will become a standard practice in the future. Liposomal formulations have shown promising results in drug delivery for various types of cancer. However, passive targeting of liposomes depends on the pathophysiological properties at the tumor site and has limitations that include decreased efficacy and off target toxicity (Kroll et al. Citation2009). This has led many researchers to focus on more specific forms of targeting liposomes, such as active targeting. Active targeting uses molecular approaches to directly target tumor cells via interactions with tumor-specific marker (Raj et al. Citation2016). The liposomal formulation requires further studies to investigate long term stability, loading levels and release kinetics to use it for in vivo study.
Conclusions
In conclusion, the data obtained from this study showed that naked and liposome pembrolizumab might potentially reduce the oxidative DNA damage in lymphocytes from HNSCC patients. Overall data from the present study proposes that pembrolizumab in both forms could protect against HNSCC through DNA damage mechanisms. Thus, liposomes pembrolizumab can be used as a useful drug in cancer cell delivery systems in various conditions.
Acknowledgments
The authors would like to thank the clinical staff of Bradford Royal Infirmary Hospital and St. Luke’s Hospital for their assistance in this study. The Sponsorship by Libyan embassy of a PhD studentship to Nagah Bobtina is gratefully acknowledged. The sponsor played no part in the conduct of the work or the writing of the manuscript.
Disclosure statement
No potential conflict of interest was reported by the author(s).
Correction Statement
This article has been corrected with minor changes. These changes do not impact the academic content of the article.
Additional information
Funding
References
- Alhawmdeh, M., M. Isreb, A. Aziz, B. K. Jacob, D. Anderson, and M. Najafzadeh. 2021. “Interferon-γ Liposome: A New System to Improve Drug Delivery in the Treatment of Lung Cancer.” ERJ Open Research 7 (3): 00555–2020. https://doi.org/10.1183/23120541.00555-2020
- Alhmoud, J. F., J. F. Woolley, A. E. Al Moustafa, and M. I. Malki. 2020. DNA Damage/Repair Management in Cancers. Cancers (Basel) 12 (4): 1050. https://doi.org/10.3390/cancers12041050
- Anderson, D., M. Najafzadeh, R. Gopalan, N. Ghaderi, A. J. Scally, S. T. Britland, B. K. Jacobs, et al. 2014. “Sensitivity and Specificity of the Empirical Lymphocyte Genome Sensitivity (LGS) Assay: implications for Improving Cancer Diagnostics.” FASEB Journal: Official Publication of the Federation of American Societies for Experimental Biology 28 (10): 4563–4570. https://doi.org/10.1096/fj.14-254748
- Argiris, A., R. L. Ferris, and D. I. Rosenthal. 2018. Head and Neck Cancers: Evidence-Based Treatment. Springer Publishing Company.
- Barenholz, Y., and D. Peer. 2012. “Liposomes and Other Assemblies as Drugs and Nano-Drugs: From Basic and Translational Research to the Clinics.” Journal of Controlled Release: Official Journal of the Controlled Release Society 160 (2): 115–116. https://doi.org/10.1016/j.jconrel.2012.03.025
- Bozzuto, G., and A. Molinari. 2015. “Liposomes as Nanomedical Devices.” International Journal of Nanomedicine 10: 975–999. https://doi.org/10.2147/IJN.S68861
- Bray, F., J. Ferlay, I. Soerjomataram, R. L. Siegel, L. A. Torre, and A. Jemal. 2018. “Global Cancer Statistics 2018: GLOBOCAN Estimates of Incidence and Mortality Worldwide for 36 Cancers in 185 Countries.” CA: a Cancer Journal for Clinicians 68 (6): 394–424. https://doi.org/10.3322/caac.21492
- Chen, Y., A. Geng, W. Zhang, Z. Qian, X. Wan, Y. Jiang, and Z. Mao. 2020. “Fight to the Bitter End: DNA Repair and Aging.” Ageing Research Reviews 64: 101154. https://doi.org/10.1016/j.arr.2020.101154
- Cohen, E. E. W., D. Soulières, C. Le Tourneau, J. Dinis, L. Licitra, M.-J. Ahn, A. Soria, KEYNOTE-040 investigators, et al. 2019. “Pembrolizumab versus Methotrexate, Docetaxel, or Cetuximab for Recurrent or Metastatic Head-and-Neck Squamous Cell Carcinoma (KEYNOTE-040): a Randomised, Open-Label, Phase 3 Study.” Lancet (London, England) 393 (10167): 156–167. https://doi.org/10.1016/S0140-6736(18)31999-8
- Deng, L., X. Ke, Z. He, D. Yang, H. Gong, Y. Zhang, X. Jing, J. Yao, and J. Chen. 2012. “A MSLN-Targeted Multifunctional Nanoimmunoliposome for MRI and Targeting Therapy in Pancreatic Cancer.” International Journal of Nanomedicine 7: 5053–5065. https://doi.org/10.2147/IJN.S34801
- Dequanter, D., R. Dok, and S. Nuyts. 2017. “Basal Oxidative Stress Ratio of Head and Neck Squamous Cell Carcinomas Correlates with Nodal Metastatic Spread in Patients under Therapy.” OncoTargets and Therapy 10: 259–263. https://doi.org/10.2147/OTT.S118980
- Fenech, M. 2007. “Cytokinesis-Block Micronucleus Cytome Assay.” Nature Protocols 2 (5): 1084–1104. https://doi.org/10.1038/nprot.2007.77
- Francisco, L. M., P. T. Sage, and A. H. Sharpe. 2010. “The PD-1 Pathway in Tolerance and Autoimmunity.” Immunological Reviews 236 (1): 219–242. https://doi.org/10.1111/j.1600-065X.2010.00923.x
- Frick, M., C. Schwieger, and C. Schmidt. 2021. “Liposomes as Carriers of Membrane-Associated Proteins and Peptides for Mass Spectrometric Analysis.” Angewandte Chemie (International ed. in English) 60 (20): 11523–11530. https://doi.org/10.1002/anie.202101242
- Goldberg, S. B., S. N. Gettinger, A. Mahajan, A. C. Chiang, R. S. Herbst, M. Sznol, A. J. Tsiouris, et al. 2016. “Pembrolizumab for Patients with Melanoma or Non-Small-Cell Lung Cancer and Untreated Brain Metastases: early Analysis of a Non-Randomised, Open-Label, Phase 2 Trial.” The Lancet. Oncology 17 (7): 976–983. https://doi.org/10.1016/S1470-2045(16)30053-5
- Johnson, D. E., B. Burtness, C. R. Leemans, V. W. Y. Lui, J. E. Bauman, and J. R. Grandis. 2020. “Head and Neck Squamous Cell Carcinoma.” Nature Reviews. Disease Primers 6 (1): 92. https://doi.org/10.1038/s41572-020-00224-3
- Kroll, A., M. H. Pillukat, D. Hahn, and J. Schnekenburger. 2009. “Current in Vitro Methods in Nanoparticle Risk Assessment: limitations and Challenges.” European Journal of Pharmaceutics and Biopharmaceutics: official Journal of Arbeitsgemeinschaft Fur Pharmazeutische Verfahrenstechnik e.V 72 (2): 370–377. https://doi.org/10.1016/j.ejpb.2008.08.009
- Kulaberoglu, Y., A. Hergovich, and V. Gómez. 2021. “The Role of p53/p21/p16 in DNA Damage Signaling and DNA Repair.” In Genome Stability, 257–274. Elsevier.
- Li, Y., D. H. Chen, J. Yan, Y. Chen, R. A. Mittelstaedt, Y. Zhang, A. S. Biris, R. H. Heflich, and T. Chen. 2012. “Genotoxicity of Silver Nanoparticles Evaluated Using the Ames Test and in Vitro Micronucleus Assay.” Mutation Research 745 (1–2): 4–10. https://doi.org/10.1016/j.mrgentox.2011.11.010
- Michaud, D. S., S. M. Langevin, M. Eliot, H. H. Nelson, M. Pawlita, M. D. McClean, and K. T. Kelsey. 2014. “High-Risk HPV Types and Head and Neck Cancer.” International Journal of Cancer 135 (7): 1653–1661. https://doi.org/10.1002/ijc.28811
- Raj, R., P. Mongia, S. Kumar Sahu, and A. Ram. 2016. “Nanocarriers Based Anticancer Drugs: Current Scenario and Future Perceptions.” Current Drug Targets 17 (2): 206–228. https://doi.org/10.2174/1389450116666150722141607
- Schmid, P., R. Salgado, Y. H. Park, E. Muñoz-Couselo, S. B. Kim, J. Sohn, S.-A. Im, et al. 2020. “Pembrolizumab plus Chemotherapy as Neoadjuvant Treatment of High-Risk, Early-Stage Triple-Negative Breast Cancer: results from the Phase 1b Open-Label, Multicohort KEYNOTE-173 Study.” Annals of Oncology: Official Journal of the European Society for Medical Oncology 31 (5): 569–581. https://doi.org/10.1016/j.annonc.2020.01.072
- Seiwert, T. Y., R. I. Haddad, S. Gupta, R. Mehra, M. Tahara, R. Berger, S.-H. Lee, B. Burtness, D. T. Le, and K. Heath. 2015. Antitumor Activity and Safety of Pembrolizumab in Patients (Pts) with Advanced Squamous Cell Carcinoma of the Head and Neck (SCCHN): Preliminary Results from KEYNOTE-012 Expansion Cohort. American Society of Clinical Oncology.
- Shah, S., V. Dhawan, R. Holm, M. S. Nagarsenker, and Y. Perrie. 2020. “Liposomes: Advancements and Innovation in the Manufacturing Process.” Advanced Drug Delivery Reviews 154–155: 102–122. https://doi.org/10.1016/j.addr.2020.07.002
- Sundahl, N., S. Rottey, D. De Maeseneer, and P. Ost. 2018. “Pembrolizumab for the Treatment of Bladder Cancer.” Expert Review of Anticancer Therapy 18 (2): 107–114. https://doi.org/10.1080/14737140.2018.1421461
- Sung, W. W., J. C. Chow, and W. C. Cho. 2021. “Tumor Mutational Burden as a Tissue-Agnostic Biomarker for Cancer Immunotherapy.” Expert Review of Clinical Pharmacology 14 (2): 141–143. https://doi.org/10.1080/17512433.2021.1865797
- Toor, S., A. Syed Khaja, I. Alkurd, and E. Elkord. 2018. “In-Vitro Effect of Pembrolizumab on Different T Regulatory Cell Subsets.” Clinical and Experimental Immunology 191 (2): 189–197. https://doi.org/10.1111/cei.13060