Abstract
Background Since the osteogenic potential of bone marrow derived mesenchymal stem cells (BMSCs) becomes reduced with passage, establishment of culture condition that permit the rapid expansion of BMSCs while retaining their potential for differentiation is needed for clinical application. Bone morphogenetic proteins stimulate osteogenic differentiation in mesenchymal progenitor cells as well as increase stem cell numbers. Thus, we analyzed the effect of recombinant human bone morphogenetic protein-2 (rhBMP-2) on the osteogenic potential of rat BMSCs over several passages.
Material and methods Osteogenic differentiation in vitro was evaluated in terms of the alkaline phosphatase (ALP) activity and the osteocalcin (OC) concentration in the supernatants, and the expression of ALP and OC mRNA in the cultured cells. For in-vivo osteogenesis, BMSCs cultured with and without rhBMP-2 through all passages were implanted into athymic mice.
Results The levels of osteogenic markers were significantly higher in the cells of the BMP(+) group than in the cells of the BMP(–) group, although they decreased with passage irrespective of whether or not rhBMP-2 was added. Similar to the in-vitro experiments, there was a greater degree of bone and cartilage tissue formation in the BMP(+) group over all passages.
Interpretation From our results, osteogenic potential can be maintained even in BMSCs that have been passaged several times in the presence of rhBMP-2. These cells are capable of inducing and participating in bone formation and can be used for clinical applications.
Bone marrow contains a population of multipotent mesenchymal stem cells (BMSCs) that generate the progenitors of osteogenic, chondrogenic, adipocytic, and myogenic cells (Bennett and Joyner Citation1991, Beresford and Bennett Citation1992, Watitani et al. Citation1995, Ohgushi et al. Citation1996, Kadiyala et al. Citation1997, Johnstone et al. Citation1998, Pittenger et al. Citation1999). BMSCs can be isolated and cultured to large numbers from a small volume of bone marrow, and they are therefore considered to be a readily available and abundant source of cells for bone tissue engineering applications. BMSCs can be directed towards the osteogenic lineage in vitro if cultured in the presence of dexamethasone, β-glycerophosphate and ascorbic acid (Kasugai et al. Citation1991, Leboy et al. Citation1991, Ohgushi et al. Citation1996, Bruder et al. Citation1998, Maniatopoulos et al. Citation1998, Coelho and Fernandes Citation2000). Furthermore, BMSCs are appropriate as donor tissue and may be used in clinical applications because they are easily isolated from a small aspirate of bone marrow, and readily generate various cell types. We have previously shown that transplantation of cultureexpanded BMSCs into the distracted callus accelerates new bone formation and shortens the treatment period during distraction osteogenesis (Takamine et al. Citation2002, Kitoh et al. Citation2004). The proportion of BMSCs is rather low, however, and the cells generated in primary culture seem to be insufficient for clinical use of bone tissue regeneration. Expansion of the cell population by passaging several times would be necessary to obtain sufficient numbers of cells, but the osteogenic potential of BMSCs becomes reduced with passage (Coelho et al. Citation2000, Ter Brugge and Jansen Citation2002, Sugiura et al. Citation2004). There is a need to establish the culture conditions that permit rapid expansion of BMSCs while retaining their potential for differentiation.
Bioactive bone growth factors play an important role in the regeneration of bone. Numerous cytokines have been reported to induce bone formation in vivo (Ripamonti and Reddi Citation1992, Linkhart et al. Citation1996, Franceschi et al. Citation2004). Bone morphogenetic proteins (BMPs) are the strong bone inducers in allograft bone fracture healing, bone regeneration and heterotopic bone formation, and stimulate osteogenic differentiation in mesenchymal progenitor cells via the extracellular signal-regulated kinase pathway (Wozney and Rosen Citation1998). Of the BMPs, BMP-2 appears to be the most potent inducer of bone formation. A number of studies have indicated that recombinant human BMP2 (rhBMP-2) is involved in the differentiation of osteoblast precursor cells into mature osteoblasts, and that the osteoinductive effects of rhBMP-2 are exerted on the progenitor cells resident in the bone marrow (Zegzula et al. Citation1997, Ikeuchi et al. Citation2002). Moreover, rhBMP-2 not only has effects on the differentiation of BMSCs, but also induces an increase in stem cell numbers (Kino et al. Citation2003, Yoon et al. Citation2003).
We analyzed the osteogenic potential of rat BMSCs, cultured with or without rhBMP-2 in the culture medium, over several passages. Osteogenic differentiation in vitro was evaluated in terms of the alkaline phosphatase (ALP) activity and the osteocalcin (OC) concentration in the supernatants, and by the expression of ALP and OC mRNA in the cultured cells. In addition, these BMSCs at various stages of differentiation were implanted subcutaneously into athymic mice, and bone formation was analyzed in vivo.
Material and methods
Cell isolation and culture
BMSCs were obtained from 4- to 6-week-old Sprague-Dawley (SD) rats and were flushed out using 3 mL of culture medium expelled from a syringe through an 18-gauge needle. The released cells were collected in a flask containing the medium. For osteoblastic differentiation, the medium was minimum essential medium alpha medium (α-MEM; Invitrogen, Grand Island, NY) supplemented with 10% fetal bovine serum (FBS; Dainippon Pharmaceutical, Osaka, Japan), 100 μL/mL 1% of penicillin-streptomycin (Invitrogen), 0.2 mM ascorbic acid (Sigma-Aldrich, St. Louis, MO), 10 mM Na-β-glycerophosphate (Sigma-Aldrich), and 10−8 M dexamethasone (Sigma-Aldrich). The bone marrow suspensions were cultured in polystyrene six-well dishes and non-adherent cells were removed from the cultures after 2 days by a series of washes in phosphatebuffered saline (PBS) and subsequent changes of medium. Adherent cells were expanded as monolayer cultures in a 5% CO2/95% air atmosphere at 37°C with medium changes every 3 days. These primary cells were referred to as passage 0 (P0). The confluent cells were dissociated with 0.025% trypsin-EDTA (Sigma-Aldrich) and subcultured in new six-well culture dishes at a plating density of 6 × 104 cells/dish. These procedures were repeated four times and the cultures were referred to as P1, P2, P3, P4, and P5. The rhBMP-2 was kindly provided by Yamanouchi Pharmaceutical Co., Ltd. (Tokyo, Japan). We prepared two groups of cells as follows: BMSCs continuously cultured with medium supplemented with 300 ng/mL rhBMP-2 (the BMP(+) group), and BMSCs cultured without rhBMP-2 (the BMP(–) group).
Osteogenic differentiation in vitro
Alkaline phosphatase and osteocalcin activity.
The culture media from the BMP(+) and BMP(–) groups were harvested every 3 days during all passages. The ALP activity and the OC concentration in the culture media were measured using the Alkaline phospha-B-test (Wako, Japan) and the Rat Osteocalcin EIA Kit (Biomedical Technologies, Stoughton, MA), according to the instruction manual. Assay of the ALP and OC was performed in 6 different samples from each of the different passages (P0–P5), and the mean values were compared between the BMP(+) and BMP(–) groups for each passage.
Total RNA isolation and reverse transcriptase polymerase chain reaction. Total RNA was isolated from individual cell layers at 7 days of subculture using Trizol reagent (Gibco-BRL, Life Technologies, Grand Island, NY). One microgram of total RNA was used as a template for reverse transcription into cDNA using the Perkin-Elmer Gold Taq Reverse Transcription System (Roche). The cDNA was amplified by PCR with oligonucleotide primers (Table). DNA amplifications were performed with an initial denaturation stage at 95°C for 8 min, followed by 28 cycles of denaturation at 95°C for 30 sec, annealing at 60°C for 30 sec, and extension at 72°C for 1 min. The final cycle ended with 7 min at 72°C (for extension). PCR products from each sample were analyzed by electrophoresis on 2% agarose gels and visualized by staining with ethidium bromide.
Quantitative real-time PCR was carried out using a LightCycler instrument (Roche Molecular Biochemicals) according to the instruction manual. The oligonucleotide primers used in the amplification reaction were the same as above. Amplification was carried out in a final volume of 20 μL containing 3 mM MgCl2, each primer at 0.5 μM, 1 μL of the sample, and 2 μL of LightCycler-Fast Start DNA Master SYBR Green I (Roche Molecular Biochemicals). The amplification program included an initial denaturation step at 99°C for 10 min for activation of Fast Start Taq DNA polymerase, followed by 40 cycles of denaturation at 99°C for xx sec, annealing at 60°C for 5 sec, and 72°C at 15 sec for extension. Ramping times were 20°C/sec. Cycle-to-cycle fluorescence readings were plotted on the computer screen for continuous monitoring of PCR product. At the end of each run, fluorescence data were analyzed by setting a noise band to remove background fluorescence. Values obtained for external standards (Rat GAPDH Standard, LightCycler primer set; Roche) were employed to construct a reference curve and copy numbers for the sample were calculated by interpolation of the resulting reference curve. The quantitative real-time reverse transcriptase PCR analysis was performed on 3 different samples from each of the different passages (P0–P5), and the values calculated for ALP and OC were compared between the BMP(+) and BMP(–) groups for each passage.
The oligonucleotide primers (Light Cycler primer/probe Set; Roche)
In-vivo bone formation
The BMSCs cultured at different passages were trypsinized and suspended in 100 μL α-MEM, and mixed with 100 μL of 0.5% type I collagen gel (KOKENCELLGEN I-PC, Japan) at a concentration of 3.0 × 105 cells/200 μL. These cell suspensions were then loaded into diffusion chambers (0.45 μm pore size; Millipore, Bedford, MA). A mid-longitudinal skin incision was made along the backs of 8-week-old male athymic mice, and two subcutaneous pockets were formed by blunt dissection. 2 sets of cells (BMP(+) and BMP(–)) were implanted into each pocket aseptically. 6 different samples from passages P0 to P4 were implanted into a total of 30 mice. The mice were killed at 4 weeks after implantation and the newly formed tissues in the chambers were analyzed for osteogenesis radiographically and histologically. The removed chambers were immediately radiographed with soft radiographs for 1.5 min at 45 kV and 1.6 mA. After radiographic examinations, the cells in the chambers were fixed in 10% formalin and embedded in paraffin. Serial 6-μm sections were stained with Alcian blue and von Kossa for histological examination.
Statistics
The values for ALP and OC concentrations in the culture medium and for mRNA expression derived from quantitative real-time reverse transcriptase PCR analysis are expressed as the mean ± standard error of the mean (SEM). The mean values for experimental groups (BMP(+) and BMP(–)) were analyzed by unpaired Student’s t-test and values of p < 0.05 were considered significant.
Results
In-vitro osteogenic differentiation
For the cells from passages P0 to P5, the ALP activity and the OC concentration in the supernatants of the BMP(+) group were significantly higher than those of the BMP(–) group, although both osteogenic markers declined with passage irrespective of whether or not rhBMP-2 was present (). There were only basal levels of ALP and OC in the P4 and P5 supernatants of the BMP(–) group, while the corresponding levels in the BMP(+) group were better maintained, even in cells passaged several times.
Figure 1. BMSCs were plated onto 6-well plates and cultured with and without rhBMP-2. Culture media from the different passages (P0–P5) of the BMP(+) and BMP(‐) groups were assayed for alkaline phosphatase (ALP) and osteocalcin (OC) as described in Material and methods. Data shown are the means (SEM) of six different samples, and were analyzed by unpaired Student’s t-test between the two groups (*p < 0.05). Both osteogenic markers were found to have significantly higher levels in the BMP(+) group than in the BMP(-) group (n = 6).
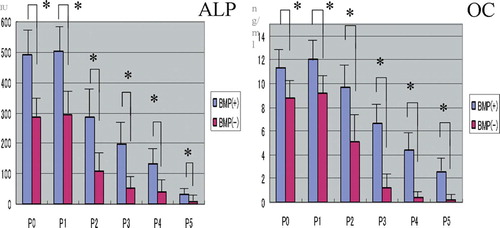
ALP and OC mRNAs were expressed in P0–P4 cells of the BMP(+) group and in P0–P3 cells of the BMP(–) group. The bands corresponding to each mRNA were, however, more intense in the BMP(+) group than in the BMP(–) group (. Quantitative real-time reverse transcriptase PCR analysis also confirmed the differences in expression levels of the two genes between the BMP(+) and BMP(–) groups (.
Figure 2. Electrophoresis of PCR products from real-time reverse transcriptase PCR analysis [OK?] of ALP and OC expression in cells at different passages (P0–P5), cultured with and without BMP-2 (A). The mRNA expression of ALP and OC was quantified as described in Material and methods (B). Data shown are the means (SEM) of 3 different samples, and were analyzed by unpaired Student’s t-test between the 2 groups (*p < 0.05). For passages P0–P4, the expression of mRNA for both osteogenic markers was significantly higher in the BMP(+) group than in the BMP(-) group (n = 3).
![Figure 2. Electrophoresis of PCR products from real-time reverse transcriptase PCR analysis [OK?] of ALP and OC expression in cells at different passages (P0–P5), cultured with and without BMP-2 (A). The mRNA expression of ALP and OC was quantified as described in Material and methods (B). Data shown are the means (SEM) of 3 different samples, and were analyzed by unpaired Student’s t-test between the 2 groups (*p < 0.05). For passages P0–P4, the expression of mRNA for both osteogenic markers was significantly higher in the BMP(+) group than in the BMP(-) group (n = 3).](/cms/asset/11c0ea27-52b7-4aeb-8160-aa9b97ae4f35/iort_a_11327302_f0002_b.jpg)
In-vivo bone formation
Calcified tissue formations in the diffusion chambers at four weeks after implantation were observed in the cells from passages P0–P4 of the BMP(+) group and in the cells from passages P0–P3 of the BMP(–) group, but the number of calcified nodules became reduced with passage in both groups ().
Figure 3. Radiographs of diffusion chambers with the cells (passages P0–P4) of the BMP(+) and BMP(-) groups at 4 weeks after transplantation. Numerous radioplaque configurations that indicate mineralized structures can be seen in the BMP(+) group compared to the BMP(-) group, although the numbers of calcifications decreased with passage in both groups. The figure shows one representative result from a total of 6 different samples at each passage.
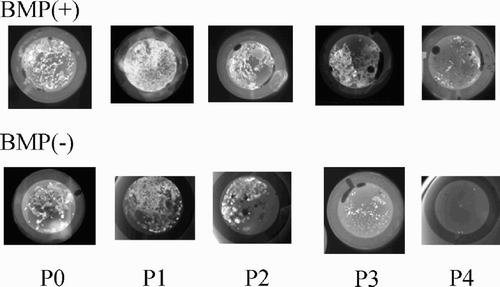
Histological examination of the diffusion chambers revealed massive bone formations inside the membrane and abundant cartilaginous matrices together with chondrocyte-like cells in the samples from passages P0–P3 of the BMP(+) group (–). In the P4 cells of the BMP(+) group, however, most of the newly formed tissues were filled with fibroblastic cells, with little evidence of osteochondrogenesis. Similarly, abundant calcium deposits were seen in the cells from passages P0– P3 of the BMP(+) group after von-Kossa staining. (–)
Figure 4. Photomicrographs of histological sections of the diffusion chambers with the BMP(+) group from passages P1(A), P2(B), P3(C), and P4(D) at 4 weeks after implantation. Massive cartilaginous tissue formations can [could?] be seen inside the chamber in the cells from passages P1–P3. (Alcian blue staining; original magnification 100×).
![Figure 4. Photomicrographs of histological sections of the diffusion chambers with the BMP(+) group from passages P1(A), P2(B), P3(C), and P4(D) at 4 weeks after implantation. Massive cartilaginous tissue formations can [could?] be seen inside the chamber in the cells from passages P1–P3. (Alcian blue staining; original magnification 100×).](/cms/asset/ee3a204b-5b7a-4bd3-9ed0-6ba377ede7d7/iort_a_11327302_f0004_b.jpg)
Figure 5. Photomicrographs of histological sections of the diffusion chambers with the BMP(+) group from passages P1(A), P2(B), P3(C), and P4(D) at 4 weeks after implantation. Abundant calcium depositions at the membrane were observed in cells from passages P1–P3. (Von Kossa staining; original magnification 100×).
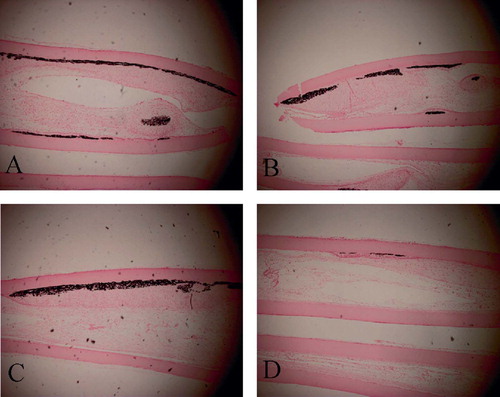
Discussion
Cell-based tissue-engineering tools and methods create new opportunities that might be useful in potential clinical applications. Autogenous BMSCs have received widespread attention because of their potential usefulness in tissue engineering (Owen Citation1988, Goshima et al. Citation1991, Sugiura et al. Citation2004, Takamine et al. Citation2002). BMSCs have osteogenic differentiation potential both in vitro and in vivo— and are therefore excellent candidates for bone tissue engineering. The present study has clearly demonstrated that culture-expanded rat BMSCs are able to become committed to osteogenic differentiation in response to rhBMP-2, which seems to enhance the recruitment, proliferation, and differentiation of mesenchymal progenitor cells and to and stimulate osteogenetic differentiation of BMSCs. It also offers an advantage regarding clinical use, since ex-vivo addition of rhBMP-2 to the culture medium seemed to be safe. A combination of BMP-2 and BMSCs would be synergistic, and cul-ture-expanded BMSCs with osteogenic potential may be able to be used for clinical application.
In-vitro expansion offers the potential to generate a large number of progenitor cells. We have previously demonstrated that in-vitro osteoblastic differentiation and in-vivo bone formation become reduced in rat BMSCs passaged several times (Sugiura et al. Citation2004). Loss of osteogenic potential of BMSCs by passage has been a dilemma in autologous cell therapy to promote bone formation. In the present study, comparing the the BMP(+) group with the BMP(–) group, elevated levels of osteogenic markers and more calcified tissue formation were observed at all passages. As a result, the osteogenic potential of the BMP (+) group was maintained even in the BMSCs that were passaged several times. Sufficiently expanded BMSCs, cultured in the presence of rhBMP-2, are capable of inducing and participating in bone formation—and can thus be used in the clinical setting to improve bone healing.
This work was supported in part by a Grant-in-Aid for Scientific Research from the Japanese Ministry of Education, Culture, Sports, Science and Technology (contract grant number 17591563).
No competing interests declared.
Contributions of authors
HI, HK and NI: designed the research. HI and FS: did the experiment and collected and analyzed the date. HI: wrote the draft manuscript. HK and NI: revised the draft manuscript.
- Bennett J H, Joyner C J. Adipocytic cells cultured from marrow have osteogenic potential. J Cell Sci 1991; 99: 131–9
- Beresford J N, Bennett J H. Evidence for an inverse relationship between the differentiation of adipocytic and osteogenic cells in rat marrow stromal cell cultures. J Cell Sci 1992; 102: 341–51
- Bruder S P, Jaiswal N, Ricalton N S. Mesenchymal stem cells in osteobiology and applied bone regeneration. Clin Orthop 1998; 355S: S247–S256
- Coelho M J, Fernandes M H. Human bone cell cultures in biocompatibility testing Part II: Effect of ascorbic acid, β-glycerophosphate and dexamethasone on osteoblastic differentiation. Biomaterials 2000; 21: 1095–102
- Coelho M J, Cabral A T, Fernandes M H. Human bone cell cultures in biocompatibility testing. Part I: Osteoblastic differentiation of serially passaged human bone marrow cells cultured in α-MEM and in DMEM. Biomaterials 2000; 21: 1087–94
- Franceschi R T, Yang S, Rutherford R B, Krebsbach P H, Zhao M, Wang D. Gene therapy approaches for bone regeneration. Cells Tissues Organs 2004; 176: 95–108
- Goshima V M, Goldberg J, Caplan A I. The osteogenic potential of culture-expanded rat marrow mesenchymal cells assayed in vivo in calcium phosphate ceramic block. Clin Orthop 1991; 262: 298–311
- Ikeuchi M, Dohi Y, Horiuchi K. Recombinant human bone morphogenetic protein-2 promotes osteogenesis within atelopeptide type I collagen solution by combination with rat cultured marrow cells. J Biomed Mater Res 2002; 60: 61–9
- Johnstone B, Hering T M, Caplan A I. In vitro chondrogenesis of bone marrow-derived mesenchymal progenitor cell. Exp Cell Res 1998; 238: 265–72
- Kadiyala S, Young R G, Thieda M A. Cultured-expanded canine mesenchymal stem cells posses osteochondrogrnic potential in vivo and in vitro. Cell Transplant 1997; 6: 125–34
- Kasugai S, Todescan R, Nagata T. Expression of bone matrix proteins associated with mineralized tissue formation by adult rat bone marrow cells in vitro: inductive effects of dexamethasone on the osteoblastic phenotype. J Cell Physiol 1991; 147: 111–20
- Kino K, Mineta T, Fukui M, Fujii T, Akita S. Bone morphogenetic protein 2 induces placental growth factor in mesenchymal stem cells. Wound Repair Regen 2003; 11: 354–60
- Kitoh H, Kitakoji T, Tsuchiya H, Ishiguro N. Transplantation of marrow derived mesenchymal stem cells and platelet rich plasma during distraction osteogenesis –A preliminary result of three cases. Bone 2004; 35: 892–8
- Leboy P H, Beresford J, Devlin C. Dexamethasone induction of osteoblast mRNA in rat marrow stromal cell cultures. J Cell Physiol 1991; 146: 370–8
- Linkhart T A, Mohan S, Baylink D J. Growth factors for bone growth and repair: IGF, TGF beta and BMP. Bone 1996; 19: 1S–12S
- Maniatopoulos C, Sodek J, Melcher A H. Bone formation in vitro by stromal cells obtained from bone marrow of young adults rats. Cell Tissue Res 1998; 254: 317–30
- Ohgushi H, Dohi Y, Katuda T. In vitro bone formation by rat marrow cell culture. J Biomed Mater Res 1996; 32: 333–40
- Owen M. Marrow stromal stem cell. J Cell Sci 1988; 10: 63–76
- Pittenger M F, Mackay A M, Beck S C. Multilineage potential of adult human mesenchymal stem cells. Science 1999; 284: 143–7
- Ripamonti U, Reddi A H. Growth and morphogenetic factors in bone induction: role of osteogenin and related bone morphogenetic proteins in craniofacial and periodontal bone repair. Crit Rev Oral Biol Med 1992; 3: 1–14
- Sugiura F, Kitoh H, Ishiguro N. Osteogenic potential of rat mesenchymal stem cells after several passages. Biochm Biophys Res Commun 2004; 316: 233–9
- Takamine Y, Tsuchiya H, Kitakoji T, Kitoh H, Ishiguro N. Distraction osteogenesis enhanced by osteoblastlike cells and collagen gel. Clin Orthop 2002; 399: 240–6
- Ter Brugge P J, Jansen J A. In vitro osteogenic differentiation of rat bone marrow cells subcultured with and without dexamethasone. Tissue Eng 2002; 8: 321–31
- Watitani S, Saito T, Caplan A I. Myogenic cells derived from rat bone marrow mesenchymal stem cells exposed to 5azacytidine. Muscle Nerve 1995; 18: 1417–26
- Wozney J M, Rosen V. Bone morphogenetic protein and bone morphogenetic protein gene family in bone formation and repair. Clin Orthop 1998; 346: 26–37
- Yoon S, Kim K, Li J, Park J, Akamaru T, Elmer W A, Hutton W C. The effect of bone morphogenetic protein-2 on rat intervertebral disc cells in vitro. Spine 2003; 28: 1773–80
- Zegzula H D, Buck D C, Brekke J, Wozney J M, Hollinger J O. Bone formation with use of rhBMP-2 (recombinanthuman bone morphogenetic protein-2). J Bone Joint Surg (Am) 1997; 79: 1778–90