Abstract
Biomaterial-related infection of joint replacements is the second most common cause of implant failure, with serious consequences. Chronically infected replacements cannot be treated without removal of the implant, as the biofilm mode of growth protects the bacteria against antibiotics. This review discusses biofilm formation on joint replacements and the important clinical phenomenon of small-colony variants (SCVs). These slow-growing phenotypic variants often remain undetected or are misdiagnosed using hospital microbiological analyses due to their unusual morphological appearance and biochemical reactions. In addition, SCVs make the infection difficult to eradicate. They often lead to recurrence since they respond poorly to standard antibiotic treatment and can sometimes survive intracellularly.
In the USA alone, 250,000 hip replacements and 400,000 knee replacements are done every year (Kurtz et al. Citation2005). These numbers are expected to double before the year 2030 because of the general increase in average age globally and growing demands for a higher quality of life.
As with all other biomaterials in humans, the use of joint replacements bears the risk of attracting infectious microorganisms. There are several theories on how infections of joint prostheses develop. Most studies on the interaction of the prosthesis with infecting organisms have shown that bacteria readily attach to devices during implantation (Lidwell et al. Citation1983). The main sources of contamination are the patient's skin and airborne particles from theater personnel (Pittet and Ducel Citation1994). The adhesion of bacteria itself is a complicated process. All biomaterials undergo changes at the surface after implantation. The earliest and probably clinically most important step is the “race for the surface”, a contest between tissue cell integration and bacterial adhesion to that same surface (Gristina Citation1987). On contact, body fluids immediately coat all surfaces with a layer of host material, mainly plasma proteins and platelets. These host proteins promote attachment of bacteria onto the implant by specific receptors and by non-specific mechanisms that may depend on their chemical composition, their hydrophobicity, and the charge of the implant surface (Bos et al. Citation1999). After their adhesion, the bacteria encase themselves in a hydrated matrix of polysaccharides and proteins (Costerton et al. Citation1999) to form a slimy layer known as a biofilm ().
Figure 1. Confocal laser scanning microscope overlay image of a 4-day-old Staphylococcus aureus biofilm on polyethylene. The sample was stained with a live/dead stain (green/red) and with Calcofluor white (blue) for slime staining. The bar represents 10 µm.
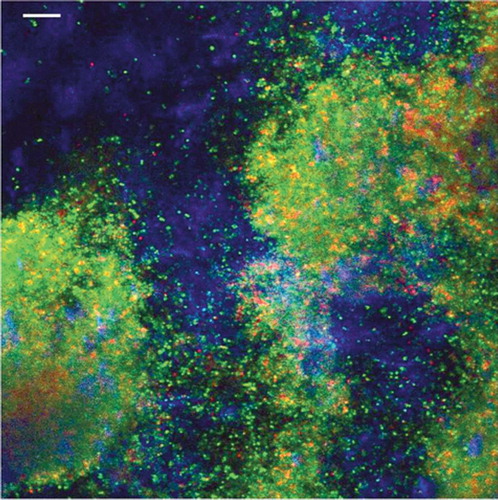
Bacteria within this protective biofilm can remain “dormant” on the surface of a prosthesis for a long time; however, when the environmental circumstances change—such as reduced host immune function—biofilm bacteria can cause a clinical infection. Generally speaking, biofilm infections will not clear until the implant has been removed and the patient must then be given antibiotic therapy. This treatment can prolong the patient's stay in hospital by several weeks, and additional surgery may be required. Infection is thus a devastating complication from the point of view of both the patient and society, as it is associated with a substantial increase in morbidity and adds significant costs to the healthcare system.
The infection rate from joint replacements has dropped dramatically since the procedure was first performed in the late 1960s, but even so about 1% of all patients develop such an infection (Josefsson and Kolmert Citation1993, Philips and Crane Citation2006). This percentage may seem small, but since these joint replacement operations are so common, it is a complication that affects a significant number of patients. Moreover, biofilm infections can cause severe problems, ranging from disfunctioning of the implanted device to lethal sepsis. Furthermore, treatment of biofilm infections is complicated, as biofilms offer enhanced protection for the infecting organisms against the natural host defenses and antibiotic therapy (Gristina and Costerton Citation1985). Bacteria in a biofilm are able to resist higher antibiotic concentrations than planktonic bacteria. In general, bacteria in a biofilm are 10–1,000 times more resistant to antibiotics than their planktonic counterparts (Widmer Citation2001, Mah et al. Citation2003). Also, some bacteria (such as Staphylococcus aureus) form small-colony variants, characterized by reduced growth rate, resistance to aminoglycosides, and possible intracellular persistence (Proctor and Peters Citation1998).
In this review we first summarize current knowledge regarding the formation of an infectious biofilm on orthopedic implants, and the mechanisms used by infectious organisms to cause a deep and chronic infection. We pay special attention to the role of strains resulting from a modification of bacterial phenotype, so-called small-colony variants (SCVs), in biofilms. Finally we review the failures in diagnosis and treatment in orthopedic surgery caused by SCVs, including possible clinical consequences.
Small-colony variants (SCVs)
The pathogenesis of many orthopedic infections is related to the presence of microorganisms in biofilms. Bacteria that attach to a surface and grow as a biofilm are protected from the killing action of antibiotics. The protective mechanisms at work in biofilms appear to be distinct from those that are responsible for conventional antibiotic resistance (Gracia et al. Citation1997, Sheldon Citation2005). The biofilm can act as a shield for the bacteria, making it difficult for them to be reached and destroyed by antibiotic drugs. Two main mechanisms have been proposed to explain how bacteria in biofilms become resistant: (1) antibiotics do not reach bacteria present in the deeper layers of biofilms because of the difficulty in penetrating the exopolysaccharide gel (slime), and (2) bacteria in deeper layers may be in a metabolic state that makes them less susceptible to antibiotics (Costerton et al. Citation1987). Arciola et al. (Citation2005) found that exopolysaccharide-produc-ing bacterial strains showed a significantly higher occurrence of antibiotic resistance than those strains that did not produce exopolysaccharides. This may explain why only one-third of prosthetic joint infections caused by slime-positive organisms can be successfully treated with antibiotics, while all infections caused by slime-negative organisms can be cured by antibiotic therapy (Davenport et al. Citation1986).
Although bacteria in biofilms are surrounded by an exopolysaccharide matrix that can restrict the diffusion of antibiotics, this may not be the central reason for biofilm-related antibiotic resistance. Jefferson et al. (Citation2005) showed that the antibiotic vancomycin can fully penetrate the biofilm but the diffusion rate in a biofilm is significantly reduced as compared to in water. This suggests that whereas planktonic bacteria are quickly exposed to a high concentration of antibiotics, bacteria in the deeper layers of the biofilm are exposed to a gradually increasing antibiotic concentration. Darouiche et al. (Citation1994) showed good penetration of biofilms by vancomycin, but that the resident bacteria were resistant. Likewise, rifampin has been found to penetrate biofilms formed by Staphylococcus epidermidis but fails to kill the bacteria effectively (Zheng and Stewart Citation2002). These studies indicate that even though the bioifilm is not a physical barrier, it somehow does cause antibiotic resistance.
Based on their own results, Ashby et al. (Citation1994) suggested that the growth rate of the bacteria in bioifilms plays a role in antibiotic resistance. The bacteria in a bioifilm do not grow exponentially; they are influenced by high cell densities (Fux et al. Citation2005) and are in a slow-growing or stationary phase (Costerton et al. Citation1999, Fux et al. Citation2005). However, not all biofilm bacteria are resistant to antibiotics; most cells die after antibiotic therapy and only a small proportion of bacteria usually survive (Ashby et al. Citation1994, Muli and Struthers Citation1998). It has been suggested that some bacterial cells in the biofilm develop a phenotype that renders them resistant to antibiotics (Cochran et al. Citation2000, Lewis Citation2005) and that this development is triggered by environmental signals. The resistant cells are called persister cells (Stewart Citation2002, Lewis Citation2005).
Biofilm bacteria may produce persister cells that neither grow nor die in the presence of antibiotics (Cogan Citation2006). Persister cells are largely responsible for the high levels of tolerance to antibiotics seen in biofilms (Lewis Citation2005), but the environmental stimuli that turn on the switch to the persister phenotype are poorly understood. Keren et al. (Citation2004) stated that production of Escherichia coli persisters depends on the phase of growth, since maintaining the E. coli culture at early exponential phase eliminated the persister cells. Similar dynamics were observed with Pseudomonas aeruginosa and S. aureus (Keren et al. Citation2004). Using a mathematical model of biofilm dynamics, Roberts and Stewart (Citation2005) predicted that the number of persister cells increases in the biofilm despite the fact that they are unable to grow, with accumulation in regions of nutrient and oxygen depletion. In these regions, although normal cells are unable to grow, they slowly switch to the persister phenotype. Cogan (Citation2006) suggested that expression of persister cells is controlled by the growth rate and the antibiotic concentration. Persister cells can be regarded as specialized survivor cells. After the antibiotic treatment has stopped, surviving persister cells are able to revert quickly to normal cells, thus allowing the biofilm to grow again (Roberts and Stewart Citation2005).
The clinical impact of an infection depends on the capacity of the infecting organisms to form a biofilm and the ability of bacterial subpopulations to switch to the dormant metabolic state known as small-colony variants (SCVs). Although it is not known whether SCVs and persister cells express exactly the same set of genes, there are many similarities between SCVs and persister cells, especially regarding how both subpopulations are formed and their reduced sensitivity to antibiotics. Bayston Citation(2000) hypothesized that the formation of SCVs takes place when the biofilm is thick enough to serve as a barrier for oxygen and nutrients. Nutrient and oxygen limitation causes some bacteria in the lower layers of the biofilm to enter a non-grow-ing or “dormant” state: the SCVs, in which they are less susceptible to growth-dependent antibiotic killing.
Many infections in the developed world are caused by biofilms, which has sparked renewed interest in SCVs. The clinical relevance of SCVs has been unclear for a long time. Many recent studies have shown that SCVs are largely responsible for the recalcitrance of infections caused by biofilms (von Eiff et al. Citation1997, Citation1999, Drenkard and Ausubel Citation2002, Seifert et al. Citation2003). The significance of SCVs as causative organisms in chronic and recurrent infections has been demonstrated in patients with chronic osteomyelitis (von Eiff et al. Citation1997) and cystic fibrosis (Haussler et al. Citation1999, Drenkard and Ausubel Citation2002, Kiristis et al. Citation2005). In the treatment of chronic osteomyelitis with gentamicin-loaded bone cement beads, the slow release of gentamicin from the beads into the local environment is an efficient way of selecting for SCVs (von Eiff et al. Citation1997). In a study of S. aureus strains isolated from patients with osteomyelitis, SCVs were found in about 29% of the cases (von Eiff et al. Citation1997). Likewise, the occurrence of S. aureus SCVs is observed in patients with cystic fibrosis (CF) after long-term antibiotic treatment (Kahl et al. Citation2003a). Analysis of sputum from CF patients showed that more than two-thirds were chronically colonized by S. aureus, and half of these samples were found to contain SCVs (Kahl et al. Citation2003b). Also, P. aeruginosa appears to play a role in the CF lung and P. aeruginosa SCVs were isolated from one-third of NN patients with P. aeruginosa infected lungs (Haussler et al. Citation1999). Recently, implant-related infections due to SCVs have been described, involving pacemakers (Seifert et al. Citation2003), ventriculoperitoneal shunts (Spanu et al. Citation2005), and hip replacements (Sendi et al. Citation2006).
Characteristic features of SCVs
The phenomenon of SCVs has been reported for several genera and species of bacteria, including the genera Staphylococcus () and Pseudomonas, and diverse species of Enterobacteriaceae (Bayer et al. Citation1987, Wise Citation1998, Costerton et al. Citation1999). Most SCVs isolated from clinical specimens have been identified as S. aureus. SCVs share many characteristic features, but since the greatest amount of information available relates to S. aureus, we have concentrated on S. aureus SCVs in this review.
Figure 2. Sheep blood agar plates (panels A and B) and magnified sectors (panels C and D) after overnight incubation at 37°C, showing two morphotypes of clonal isolates of Staphylococcus lugdunensis recovered from blood culturesand the infected pocket of a patient with pacemaker infection. Panels A and C show S. lugdunensis with the normal phenotype, characterized by colonies with yellow pigmentation and weak hemolysis. Panels B and D show small colony variants, characterized by tiny (pinpoint) non-pig-mented, non-hemolytic colonies. The pictures in this figure were taken with permission from Seifert H, Oltmanns D, Becker K, Wisplinghoff H, von Eiff C. Staphylococcus lugdunensis pacemaker-related infection. Emerg Infect Dis 2005 Aug;11(8):1283-6. Available from http://www.cdc.gov/ncidod/EID/vol11no08/04-1177.htm.
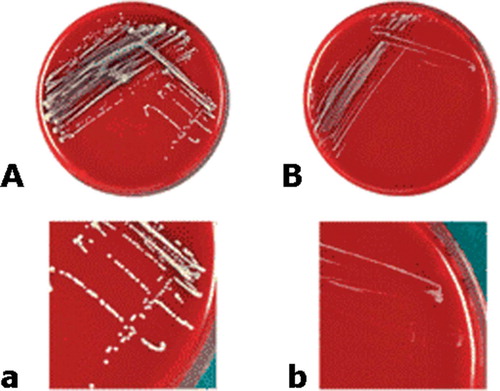
SCVs of S. aureus (Table) grow slowly and produce small colonies on standard media. These are 10 times smaller than the usual S. aureus colonies (Bergoge-Berezin Citation2000). SCVs have the ability to revert to normal growth (Musher et al. Citation1977, Lewis et al. Citation1991) but there are no data available about the genetic events responsible for the reappearance of larger colonies. In addition, S. aureus SCVs are characterized as electron transport-deficient bacteria because of their auxotrophism to hemin or menadione. Hemin/menadione auxotrophy is the inability of an organism to synthesize hemin or menadione, which is required for its growth. Hemin or menadione auxotrophs will therefore only be able to grow if hemin or menadione can be taken up from the environment. Consequently, these SCVs produce very small, mostly non-pig-mented, and non-hemolytic colonies on normal agar plates. Moreover, they show various other features that are atypical of S. aureus, including reduced coagulase production and increased resistance to aminoglycosides and cell wall-active antibiotics (von Eiff et al. Citation1997, Proctor and Peters Citation1998, Proctor et al. Citation1998, Kahl et al. Citation1998, Citation2003a, Seifert et al. Citation1999, Baumert et al. Citation2002). Furthermore, the ability of these variants to persist intracellularly within non-professional phagocytes, cells other than macrophages and neutrophils, has been described (Kahl et al. Citation1998, von Eiff et al. Citation2001, Vaudaux et al. Citation2002). S. aureus SCVs are capable of living intracellularly and consequently survive antibiotic therapy because they produce reduced levels of α-toxin, which prevents the cells from lysing (von Eiff et al. Citation2000).
Table 1. Differences in features between S. aureus and its SCVs
Failure to diagnose biofilm infections in orthopedics
Infection after joint replacement can present a diagnostic challenge, especially in late-onset infection, as the patient's pain may mimic that experienced from other common complications of the surgery. There are no preoperative tests that are consistently sensitive and specific for infection in patients who need revision surgery. Definitive diagnosis based solely on history and physical findings may prove inaccurate. The only consistent clinical finding in biofilm infections is pain at the site of the implant. However, patients with early onset of their infection typically have pain, redness, and swelling at the joint, or drainage from the wound, while patients who develop infections later in recovery complain of a gradual onset of joint pain—often without fever or other obvious signs of joint infection. There are less clinical signs and symptoms of infection in those patients. Hematological testing, radiographs and bone scans are highly variable, and all may give positive results in both aseptic loosening and infection, making these indicators of limited use. In addition, the sensitivity of preoperative aspiration of the infected joint does not exceed 75% (Barrack et al. Citation1997). Only the sum of clinical signs and symptoms, blood tests, radiography, and a microbiological workup can provide an accurate diagnosis. Investigation of suspected infection in prosthetic joint replacements remains a difficult orthopedic problem, with important therapeutic implications. It is extremely important to be able to differentiate aseptic loosening from infection, as the treatment of these two is totally different.
Auxotrophism
Intraoperative specimens provide the most accurate samples for microbiological culture and are frequently used as the reference standard for diagnosing infected joint replacements. However, standard microbiological culture techniques are only moderately sensitive and specific in diagnosing infected arthroplasties. A very low inoculum, adherent bacteria, and the existence of SCVs may limit detection. Consequently, a significant proportion of these infections is probably never recognized (Philips and Kattapuram Citation1983, Tunney et al. Citation1998, Costerton et al. Citation1999, Neut et al. Citation2003). The incidence of infection in joint prostheses may therefore be grossly underestimated, and hypothetically it might be argued that inadequate microbial sampling by current hospital diagnostic methods is at least partially responsible for the high failure rates in revision surgery. Adequate sampling is extremely important in clinical practice—as, for example, septic joint prostheses require eradication of the infecting bacteria before implantation of a new prosthesis, whereas uninfected joint prostheses can be replaced immediately.
Although microscopic morphology and cell size, and Gram staining of SCVs may be normal (Haussler et al. Citation1999, Looney Citation2000), clinical microbiology laboratories may fail to detect them. Since these phenotypic variants have a low metabolism and grow very slowly on standard agar plates, they can easily be missed in a routine laboratory, where tissue samples are cultured for only 48 h. Colony formation by SCVs can often be detected on culture media after 48–72 h of culture during primary isolation (Proctor et al. Citation1995, Roggenkamp et al. Citation1998). Neut et al. (Citation2003) showed that prolonging the culture period of microbiological samples from infected joint prostheses could double the detection rate for infections, as bacteria in biofilms generally require a longer time in culture (Atkins and Bowler Citation1998). As S. aureus SCVs show generation times of up to 6 times longer than that for metabolically normal S. aureus (Proctor et al. Citation1995), cultures for detection of SCVs should be incubated for at least 6 days (Looney Citation2000). The addition of hemin and menadion to routine blood agar used for hospital culture increases the detection rate, as SCVs form normal-sized colonies and grow at the same speed as the parent strain on agar plates enriched with hemin and menadione (Kahl et al. Citation2003a). SCVs cultured from patients with persistent and relapsing infections have been shown to be auxotrophic for hemin and/or menadion (Proctor and Peters Citation1998). Supplementation of the auxotrophs with exogenous menadione or hemin resulted in normal growth and morphology, and increased hemolytic activity (Balwit et al. Citation1994). Subcultivation of SCVs showed hemin-dependent growth: formation of larger colonies (diameter 2 mm) in the presence of hemin and formation of small colonies (diameter 0.2 mm) in its absence (Roggenkamp et al. Citation1998).
Because of their particular growth characteristics, atypical morphological appearance, and unusual biochemical reactions (e.g. reduced toxin production), SCVs present a challenge to the clinical microbiologist, often resulting in misidentification (Proctor et al. Citation1995, Citation1998, Proctor and Peters Citation1998). For example, Seifert et al. (Citation2003) described case studies in which blood cultures yielded nonpigmented and non-hemolytic staphylococci, which were initially identified as coagulase-nega-tive staphylococci on the basis of a negative tube coagulation test and the API ID 32 Staph system. However, the morphological appearance of colonies was suggestive of SCVs of S. aureus, and this was confirmed by DNA amplification using the polymerase chain reaction (PCR). Likewise, using molecular methods, Spanu et al. (Citation2005) identiified S. aureus SCVs that were methicillin-resis-tant, while these had originally been identified by 3 widely used automated identification systems as being methicillin-susceptible coagulase-negative staphylococci. Thus, it is most important to take SCVs into account as a possible cause of persistent infectious diseases, particularly when no bacteria or unusual microorganisms can be found in specimens from such cases.
Reduced production of virulence factors
When there is a suspicion of an infected joint prosthesis, biochemical investigations can be performed. Erythrocyte sediment rate (ESR) and Creactive protein (CRP) levels are important laboratory parameters in the diagnosis of postoperative infection in patients. In joint prosthesis infections caused by bacteria of high virulence, high CRP and ESR values are common (Shih et al. Citation1987, Sanzén and Carlsson Citation1989), but in low-grade infections the absence of elevated levels of these two parameters does not exclude the possibility of a biofilm infection. Most recent studies tend to favor CRP over ESR, mainly because of the fact that ESR is affected by a multitude of factors (Ng Citation1998). CRP levels rise early and before the onset of clinical symptoms, and decline with the resolution of infection. Cytokines, particularly IL-6, induce synthesis of CRP in the liver (Kragsbjerg and Holmberg Citation1995). As part of their general reduction in metabolic activity, S. aureus SCVs do not produce virulence factors such as α-toxin, coagulase, or other products that are associated with stimulation of cytokine release and immune response (Bayston Citation2000). Limited release of cytokines at the site of an infection with SCVs does not cause elevated levels of CRP. Thus, measurement of the CRP level is not an adequate diagnostic tool when establishing that there is a postoperative infection caused by SCVs.
Failure in treatment of biofilm infections in orthopedics
Treatment of infected joint replacements is difficult, and usually requires appropriate surgical intervention combined with a prolonged course of antibiotic therapy. The choice of optimal management depends on the duration and pathogenesis of the infection, stability of the implant, antibiotic susceptibility of the pathogen, and condition of the soft tissue. To eradicate chronic prosthesis infection, most authors recommend removal of the implant (McDonald et al. Citation1989, Tattevin et al. Citation1999) and two-stage revision surgery. Prior to a successful reimplantation after infection, the infection should be fully eradicated through surgical debridement and removal of the prosthesis. Subsequently, high local levels of antibiotics can be achieved in the compromised tissue by implantation of chains of gentamicin-loaded bone cement beads, before a second prosthesis is actually implanted. The gentamicin concentration that can be achieved in this way is much higher than with systemic administration (Walenkamp Citation1997). These beads are usually removed after 2 weeks and the absence of cultivable microorganisms in excised soft tissue samples by routine hospital culture is taken as a sign that the infection has been eradicated, and implantation of a new prosthesis can take place. Although the use of gentamicin-loaded beads is accepted in clinical practice, the beads themselves can act as a biomaterial surface to which subpopulations of bacteria may preferentially adhere, grow and possibly even develop antibiotic resistance (Neut et al. Citation2001).
The slow release of gentamicin from the beads into the local environment is an efficient way of selecting for SCVs (von Eiff et al. Citation1997). In routine hospital culture, such minority populations are disregarded, but their presence may have consequences in the subsequent treatment of a patient after orthopedic revision surgery. Neglecting SCVs will contribute to the occurrence of reinfections after employing gentamicin-loaded beads, and this may be one of the reasons for higher infection rates after revision surgery.
Antibiotic resistance
Bacteria persisting as SCVs within biofilms may explain the recurrent nature of biofilm infections. SCVs cause persistent and relapsing infections due to their increased resistance to antibiotics. The depressed electron transport activity seen in these auxotrophic SCVs may account for their in vitro resistance to a variety of antibiotics (Proctor and Peters Citation1998). In addition, SCVs have defective catalase activity, which may interfere with oxidative metabolism and aminoglycoside uptake (Rusthoven et al. Citation1979). The low content of ATP in SCVs causes inefficient transport of aminoglycosides into the cell, resulting in increased resistance to gentamicin and other aminoglycosides (Proctor and Peters Citation1998). The minimum inhibitory concentrations of four aminoglycoside antibiotics were found to be 8- to over 16 times higher for SCVs than for the normal large colony type (Rusthoven et al. Citation1979). Moreover, the slow growth of SCVs and consequently cell-wall division reduces the effectiveness of antibiotics that act at the cell wall (Looney Citation2000). However, SCVs are susceptible to a range of antibiotics with other modes of action, the hydrophilic antibiotics tetracycline and erythromycin for example, and to the hydrophobic antibiotics rifampicin and chloramphenicol (Langford et al. Citation1989). For optimal eradication of SCVs, treatment should consist of therapy with an antibiotic that has optimal bactericidal activity against slowly growing bacteria (Vaudaux et al. Citation2006). Rifampin may be the most efficient antibiotic because of its excellent efficacy against stationary and adherent bacteria (Widmer et al. Citation1990). Although rifampin has very good bactericidal activity against slow-grow-ing bacteria, it should be given in combination with other antibiotics because of the rapid emergence of resistance when given alone (Vaudaux et al. Citation2006). The combination proposed by Sendi et al. (Citation2006), rifampin with a fluoroquinolone, has been shown to be successful when dealing with hip replacements infected with S. aureus SCVs.
Intracellular survival
S. aureus SCVs can survive inside non-profes-sional phagocytes, such as endothelial cells and osteoblasts (Hudson et al. Citation1995, von Eiff et al. Citation2000). These SCVs have a dampened production of cytotoxins and this may downregulate the induction of cell lysis or apoptosis (Proctor et al. Citation2006). Unlike the wild type, they do not kill the cells because they produce very little α-toxin (von Eiff et al. Citation2000). Their reduced virulence creates a completely new niche for these bacteria inside the host cell (Proctor Citation1994, Proctor et al. Citation1995). The intracellular location may shield these SCVs from the host defenses and exposure to antibiotics (Kahl et al. Citation1998, Vaudaux et al. Citation2002, von Eiff et al. Citation2001), thus providing one explanation for the difficulty in clearing SCVs from host tissues. In addition, S. aureus SCVs can also be phagocytosed by monocytes and macrophages. They can hide within these host cells, then revert to the highly virulent rapidly growing form and lyse the host cell once the host immune response has dropped and antibiotic therapy is complete (von Eiff et al. Citation2000). S. aureus persisting as SCVs inside host cells may also be an explanation for the recurrent nature of these biofilm infections.
Conclusions
Clinical and laboratory findings lead to the conclusion that SCVs must be actively sought after in clinical microbiology, because they grow very slowly and can easily be missed. Particularly those samples from patients who have undergone longterm antibiotic therapy (e.g. antibiotic-loaded bone cement beads or spacers) or from individuals suffering from unusually persistent or recurrent infections should be examined meticulously for SCVs. In addition, it is most important to take SCVs into account as a possible cause of persistent infectious diseases when no bacteria or unusual microorganisms are found in materials obtained from such cases. Also, due to reduced production of virulence factors by SCVs, absence of elevated levels of CRP in patients postoperatively does not exclude the possibility of a biofilm infection. An optimal treatment of SCV-mediated infections has not been established, but these bacterial forms show increased resistance to aminoglycosides and cellwall active antibiotics.
Contributions of authors
DN: conception and design of the study, data collection, analysis, and manuscript preparation. HvdM, SB, and HB: study design, analysis, and revision of the manuscript.
No competing interests declared.
- Arciola C R, Campoccia D, Gamberini S, Donati M E, Pirini V, Visai L, Speziale P, Montanaro L. Antibiotic resistance in exopolysaccharide-forming Staphylococcus epidermidis clinical isolates from orthopaedic implant infections. Biomaterials 2005; 26: 6530–5
- Ashby M J, Neale J E, Knott S J, Critchley I A. Effect of antibiotics on non–growing planktonic cells and biofilms of Escherichia coli. J Antimicrob Chemother 1994; 33: 443–52
- Atkins B L, Bowler I C. The diagnosis of large joint sepsis. J Hosp Infect 1998; 40: 263–74
- Balwit J M, Van Langevelde P, Vann J M, Proctor R A. Gentamicin–resistant menadione and hemin auxotrophic Staphylococcus aureus persist within cultured endothelial cells. J Infect Dis 1994; 170: 1033–7
- Barrack R L, Jennings R W, Wolfe M W, Bertot A J. The value of preoperative aspiration before total knee revision. Clin Orthop 1997, 345: 8–16
- Baumert N, Von Eiff C, Schaaff F, Peters G, Proctor R A, Sahl H G. Physiology and antibiotic susceptibility of Staphylococcus aureus small colony variants. Microb Drug Resist 2002; 8: 253–60
- Bayer A S, Norman D C, Kim K S. Characterization of impermeability variants of Pseudomonas aeruginosa isolated during unsuccessful therapy of experimental endocarditis. Antimicrob Agents Chemother 1987; 31: 70–5
- Bayston R. Biofilms and prosthetic devices. Community structure and co–operation in biofilms, D G Allison, P Gilbert, H M Lappin–Scott, M Wilson. Cambridge university press. 2000: 295–308
- Bergoge–Berezin E. Resistance and new antibiotic strategies The problem with staphylococcus. Presse Med 2000; 29: 2018–21
- Bos R, Van der Mei H C, Busscher H J. Physico–chemistry of initial microbial adhesive interactions––its mechanisms and methods for study. FEMS Microbiol Rev 1999; 23: 179–230
- Cochran W L, Suh S J, McFeters G A, Stewart P S. Role of RpoS and AlgT in Pseudomonas aeruginosa biofilm resistance to hydrogen peroxide and monochloramine. J Appl Microbiol 2000; 88: 546–53
- Cogan N G. Effects of persister formation on bacterial response to dosing. J Theor Biol 2006; 238: 694–703
- Costerton J W, Cheng K J, Geesey G G, Ladd T I, Nickel J C, Dasgupta M, Marrie T J. Bacterial biofilms in nature and disease. Annu Rev Microbiol 1987; 41: 435–64
- Costerton J W, Stewart P S, Greenberg E P. Bacterial biofilms: a common cause of persistent infections. Science 1999; 284: 1318–22
- Darouiche R O, Dhir A, Miller A J, Landon G C, Raad I I, Musher D M. Vancomycin penetration into biofilm covering infected prostheses and effect on bacteria. J Infect Dis 1994; 170: 720–3
- Davenport D S, Massanari R M, Pfaller M A, Bale M J, Streed S A, Hierholzer W J, Jr. Usefulness of a test for slime production as a marker for clinically significant infections with coagulase–negative staphylococci. J Infect Dis 1986; 153: 332–9
- Drenkard E, Ausubel F M. Pseudomonas biofilm formation and antibiotic resistance are linked to phenotypic variation. Nature 2002; 416: 740–3
- Fux C A, Costerton J W, Stewart P S, Stoodley P. Survival strategies of infectious biofilms. Trends Microbiol 2005; 13: 34–40
- Gracia E, Fernandez A, Conchello P, Lacleriga A, Paniagua L, Seral F, Amorena B. Adherence of Staphylococcus aureus slime–producing strain variants to biomaterials used in orthopaedic surgery. Int Orthop 1997; 21: 46–51
- Gristina A G. Biomaterial–centered infection; microbial adhesion versus tissue integration. Science 1987; 237: 1588–95
- Gristina A G, Costerton J W. Bacterial adherence to biomaterials and tissue: The significance of its role in clinical sepsis. J Bone Joint Surg (Am) 1985; 67: 264–73
- Haussler S, Tummler B, Weissbrodt H, Rohde M, Steinmetz I. Small–colony variants of Pseudomonas aeruginosa in cystic fibrosis. Clin Infect Dis 1999; 29: 621–5
- Hudson M C, Ramp W K, Nicholson N C, Williams A S, Nousiainen M T. Internalization of Staphylococcus aureus by cultured osteoblasts. Microb Pathog 1995; 19: 409–19
- Jefferson K K, Goldmann D A, Pier G B. Use of confocal microscopy to analyze the rate of vancomycin penetration through Staphylococcus aureus biofilms. Antimicrob Agents Chemother 2005; 49: 2467–73
- Josefsson G, Kolmert L. Prophylaxis with systematic antibiotics versus gentamicin bone cement in total hip arthroplasty. A ten–year survey of 1,688 hips. Clin Orthop 1993, 292: 210–4
- Kahl B, Herrmann M, Everding A S, Koch H G, Becker K, Harms E, Proctor R A, Peters G. Persistent infection with small colony variant strains of Staphylococcus aureus in patients with cystic fibrosis. J Infect Dis 1998; 177: 1023–9
- Kahl B C, Belling G, Reichelt R, Herrmann M, Proctor R A, Peters G. Thymidine–dependent small–colony variants of Staphylococcus aureus exhibit gross morphological and ultrastructural changes consistent with impaired cell separation. J Clin Microbiol 2003a; 41: 410–3
- Kahl B C, Duebbers A, Lubritz G, Haeberle J, Koch H G, Ritzerfeld B, Reilly M, Harms E, Proctor R A, Herrmann M, Peters G. Population dynamics of persistent Staphylococcus aureus isolated from the airways of cystic fibrosis patients during a 6–year prospective study. J Clin Microbiol 2003b; 41: 4424–7
- Keren I, Kaldalu N, Spoering A, Wang Y, Lewis K. Persister cells and tolerance to antimicrobials. FEMS Microbiol Lett 2004; 230: 13–8
- Kirisits M J, Prost L, Starkey M, Parsek M R. Characterization of colony morphology variants isolated from Pseudomonas aeruginosa biofilms. Appl Environ Microbiol 2005; 71: 4809–21
- Kragsbjerg P, Holmberg H. Serum concentrations of inter–leukin–6, tumour necrosis factor–a, and C–reactive protein in patients undergoing major operations. Eur J Surg 1995; 161: 17–22
- Kurtz S, Mowat F, Ong K, Chan N, Lau E, Halpern M. Prevalence of primary and revision total hip and knee arthroplasty in the United States from 1990 through 2002. J Bone Joint Surg (Am) 2005; 87: 1487–97
- Langford P R, Anwar H, Gonda I, Brown M R. Outer membrane proteins of gentamicin induced small colony variants of Pseudomonas aeruginosa. FEMS Microbiol Lett 1989; 61: 33–6
- Lewis K. Persister cells and the riddle of biofilm survival. Biochemistry (Mosc) 2005; 70: 267–74
- Lewis L A, Li K B, Gousse A, Pereira F, Pacheco N, Pierre S, Kodaman P, Lawson S. Genetic and molecular analysis of spontaneous respiratory deficient (res) mutants of Escherichia coli K–12. Microbiol Immunol 1991; 35: 289–301
- Lidwell O M, Lowbury E J, Whyte W, Blowers R, Stanley S J, Lowe D. Airborne contamination of wounds in joint replacement operations: the relationship to sepsis rates. J Hosp Infect 1983; 4: 111–31
- Looney W J. Small–colony variants of Staphylococcus aureus. Br J Biomed Sci 2000; 57: 317–22
- Mah T F, Pitts B, Pellock B, Walker G C, Stewart P S, O'Toole G A. A genetic basis for Pseudomonas aeruginosa biofilm antibiotic resistance. Nature 2003; 426: 306–10
- McDonald D J, Fitzgerald R H, Jr, Ilstrup D M. Two–stage reconstruction of a total hip arthroplasty because of infection. J Bone Joint Surg (Am) 1989; 71: 828–34
- Muli F W, Struthers J K. The growth of Gardnerella vaginalis and Lactobacillus acidophilus in Sorbarod biofilms. J Med Microbiol 1998; 47: 401–5
- Musher D M, Baughn R E, Templeton G B, Minuth J N. Emergence of variant forms of Staphylococcus aureus after exposure to gentamicin and infectivity of the variants in experimental animals. J Infect Dis 1977; 136: 360–9
- Neut D, Van de Belt H, Stokroos I, Van Horn J R, Van der Mei H C, Busscher H J. Biomaterials–associated infection of gentamicin–loaded PMMA beads in orthopaedic revision surgery. J Antimicro Chemo 2001; 47: 885–91
- Neut D, Van Horn J R, Van Kooten T G, Van der Mei H C, Busscher H J. Detection of biomaterial–associated infections in orthopaedic joint implants. Clin Orthop 2003, 413: 261–8
- Ng T. Erythrocyte sedimentation rate, plasma viscosity and C–reactive protein in clinical practice. Br J Hosp Med 1998; 58: 521–3
- Philips J E, Crane T P. The incidence of deep prosthetic infections. J Bone Joint Surg (Br) 2006; 88(7)943–8
- Philips W C, Kattapuram S V. Efficiacy of preoperative hip aspiration performed in the radiology department. Clin Orthop 1983, 179: 141–6
- Pittet D, Ducel G. Infectious risk factors related to operating rooms. Infect Control Hosp Epidemiol 1994; 15: 456–62
- Proctor R A. Microbial pathogenic factors: small colony. Infections associated with indwelling medical devices variants, A L Bisno, F A Waldvogel. American Society for Microbiology, Washington, DC 1994; 79–90
- Proctor R A, Peters G. Small colony variants in staphylococcal infections: diagnostic and therapeutic implications. Clin Infect Dis 1998; 27: 419–23
- Proctor R A, Van Langevelde P, Kristjansson M, Maslow J N, Arbeit R D. Persistent and relapsing infections associated with small–colony variants of Staphylococcus aureus. Clin Infect Dis 1995; 20: 95–102
- Proctor R A, Kahl B, von Eiff C, Vaudaux P E, Lew D P, Peters G. Staphylococcal small colony variants have novel mechanisms for antibiotic resistance. Clin Infect Dis 1998; 27: S68–S74
- Proctor R A, von Eiff C, Kahl B C, Becker K, McNamara P, Herrmann M, Peters G. Small colony variants: a pathogenic form of bacteria that facilitates persistent and recurrent infections. Nat Rev Microbiol 2006; 4: 295–305
- Roberts M E, Stewart P S. Modelling protection from antimicrobial agents in biofilms through the formation of persister cells. Microbiology 2005; 151: 75–80
- Roggenkamp A, Sing A, Hornef M, Brunner U, Autenrieth I B, Heesemann J. Chronic prosthetic hip infection caused by a small–colony variant of Escherichia coli. J Clin Microb 1998; 36: 2530–4
- Rusthoven J J, Davies T A, Lerner S A. Clinical isolation and characterization of aminoglycoside–resistant small colony variants of Enterobacter aerogenes. Am J Med 1979; 67: 702–6
- Sanzén L, Carlsson A S. The diagnostic valuen of C–reactive protein in infected total hip arhtroplasties. J Bone Joint Surg (Br) 1989; 71: 638–41
- Seifert H, Von Eiff C, Fätkenheuer G. Fatal case due to methicillin–resistant Staphylococcus aureus small colony variants in an AIDS patient. Emerg Infect Dis 1999; 5: 450–3
- Seifert H, Wisplinghoff H, Schnabel P, von Eiff C. Small colony variants of Staphylococcus aureus and pacemakerrelated infection. Emerg Infect Dis 2003; 9: 1316–8
- Sendi P, Rohrbach M, Graber P, Frei R, Ochsner P E, Zimmerli W. Staphylococcus aureus small colony variants in prosthetic joint infection. Clin Infect Dis 2006; 43: 961–7
- Sheldon A T, Jr. Antibiotic resistance: a survival strategy. Clin Lab Sci 2005; 18: 170–80
- Shih L Y, Wu J J, Yang D J. Erythrocyte sedimentation rate and C–reactive protein values in patients with total hip arthroplasty. Clin Orthop 1987; 225: 238–46
- Spanu T, Romano L, D'Inzeo T, Masucci L, Albanese A, Papacci F, Marchese E, Sanguinetti M, Fadda G. Recurrent ventriculoperitoneal shunt infection caused by smallcolony variants of Staphylococcus aureus. Clin Infect Dis 2005; 41: 48–52
- Stewart P S. Mechanisms of antibiotic resistance in bacterial biofilms. Int J Med Microbiol 2002; 292: 107–13
- Tattevin P, Cremieux A C, Pottier P, Huten D, Carbon C. Prosthetic joint infection: when can prosthesis salvage be considered. Clin Infect Dis 1999; 29: 292–5
- Tunney M M, Patrick S, Gorman S P, Nixon J R, Anderson N, Davis R I, Hanna D, Ramage G. Improved detection of infection in hip replacements A currently underestimated problem. J Bone Joint Surg (Br) 1998; 80: 568–72
- Vaudaux P, Francois P, Bisognano C, Kelley W L, Lew D P, Schrenzel J, Proctor R A, McNamara P J, Peters G, Von Eiff C. Increased expression of clumping factor and fibro–nectin–binding proteins by hemB mutants of Staphylococacus aureus expressing small colony variant phenotypes. Infect Immun 2002; 70: 5428–37
- Vaudaux P, Kelley W L, Lew D P. Staphylococcus aureus small colony variants: difficult to diagnose and difficult to treat. Clin Infect Dis 2006; 43: 968–70
- Von Eiff C, Bettin D, Proctor R A, Rolauffs B, Lindner N, Winkelmann W, Peters G. Recovery of small colony variants of Staphylococcus aureus following gentamicin bead placement for osteomyelitis. Clin Infect Dis 1997; 25: 1250–1
- Von Eiff C, Vaudaux P, Kahl B C, Lew D, Emler S, Schmidt A, Peters G, Proctor R A. Bloodstream infections caused by small–colony variants of coagulase–negative staphylococci following pacemaker implantation. Clin Infect Dis 1999; 29: 932–4
- Von Eiff C, Proctor R A, Peters G. Staphylococcus aureus small colony variants: formation and clinical impact. Int J Clin Pract 2000; 115: S44–9
- Von Eiff C, Becker K, Metze D, Lubritz G, Hockmann J, Schwarz T, Peters G. Intracellular persistence of Staphylococcus aureus small–colony variants within keratinocytes: a cause for antibiotic treatment failure in a patient with Darier's disease. Clin Infect Dis 2001; 32: 1643–7
- Walenkamp G H I M. Chronic osteomyelitis. Acta Orthop Scand 1997; 68: 497–506
- Widmer A F. New developments in diagnosis and treatment of infection in orthopedic implants. Clin Infect Dis 2001; 33: S94–106
- Widmer A F, Frei R, Rajacic Z, Zimmerli W. Correlation between in vivo and in vitro efficacy of antimicrobial agents against foreign body infections. J Infect Dis 1990; 162: 96–102
- Wise R I. Small colonies (G variants) of staphylococci: isolation from cultures and infection. Ann NY Acad Sci 1998; 65: 169–74
- Zheng Z, Stewart P S. Penetration of rifampin through Staphylococcus epidermidis biofilms. Antimicrob Agents Chemother 2002; 46: 900–3