Abstract
Background Infection remains a severe complication following a total hip replacement. If infection is suspected when revision surgery is being performed, additional gentamicin is often added to the cement on an ad hoc basis in an attempt to reduce the risk of recurrent infection.
Methods and results In this in vitro study, we determined the effect of incorporating additional gentamicin on the mechanical properties of cement. We also determined the degree of gentamicin release from cement, and also the extent to which biofilms of clinical Staphylococcus spp. isolates form on cement in vitro. When gentamicin was added to unloaded cement (1–4 g), there was a significant reduction in the mechanical performance of the loaded cements compared to unloaded cement. A significant increase in gentamicin release from the cement over 72 h was apparent, with the amount of gentamicin released increasing significantly with each additional 1 g of gentamicin added. When overt infection was modeled, the incorporation of additional gentamicin did result in an initial reduction in bacterial colonization, but this beneficial effect was no longer apparent by 72 h, with the clinical strains forming biofilms on the cements despite the release of high levels of gentamicin.
Interpretation Our findings indicate that the addition of large amounts of gentamicin to cement is unlikely to eradicate bacteria present as a result of an overt infection of an existing implant, and could result in failure of the prosthetic joint because of a reduction in mechanical performance of the bone cement.
While aseptic mechanical loosening is generally reported to be the most common cause of prosthetic joint failure (Malchau et al. Citation2002), it has been shown that the incidence of infection, primarily with Staphylococcus spp. and/or Propionibacterium acnes, is greatly underestimated. Infection was implicated as the cause of joint failure in 22% of revision operations in one study (Tunney et al. Citation1998a). Revision surgery in which the infected implant is removed and replaced is usually required to eradicate the infection. During revision surgery gentamicin-loaded bone cement is used for prosthesis fixation on a routine basis, to provide a high local antibiotic concentration for elimination of any bacterial infection and to reduce the risk of infection of the newly implanted device. Using an in vitro model of antibiotic prophylaxis in hip arthroplasty, we have recently shown that when a low bacterial inoculum is present—representative of the inocula that may be present at the operative site as a result of contamination from skin—a combination of gentamicin-loaded bone cement and cefuroxime given per- and postoperatively prevented biofilm formation by clinical isolates (Tunney et al. Citation2006). These results confirmed data reported from the Norwegian Arthroplasty Register showing that the lowest risk of revision surgery following total hip replacement is when prophylactic antibiotics are used both in the cement and systemically (Espehaug et al. Citation1997, Engesaeter et al. Citation2003). However, we found using our in vitro model that if overt infection is present at the time of revision surgery, a combination of gentamicin-loaded bone cement for implant fixation and cefuroxime given systemically per- and postoperatively may not eradicate the existing infection and may not prevent infection of the newly implanted device (Tunney et al. Citation2006).
If infection is suspected when revision surgery is being performed, orthopedic surgeons frequently add additional gentamicin to the bone cement on an ad hoc basis (Hanssen and Spangehi Citation2004). The expectation is that higher levels of gentamicin will be released as a result, thereby increasing the likelihood that existing infection will be eradicated and decreasing the likelihood that the newly implanted device will become infected. Despite the fact that manufacturers of bone cement recommend that no more than twice the standard dose of gentamicin should be added, in order to prevent deterioration in mechanical properties, gentamicin is often added in quantities ranging from 1 to 4 g to each individual bone cement mix. The effect of the incorporation of additional gentamicin on the mechanical properties of the bone cement is not known; nor is it known whether it reduces or prevents bacterial colonization and biofilm formation on the bone cement surface.
The objectives of this study were therefore to determine the effect of incorporating additional amounts of gentamicin on: (1) the mechanical, physical, and thermal properties of the bone cement, (2) gentamicin release from the bone cement, and (3) biofilm formation by clinical Staphylococcus spp. isolates on the bone cement in vitro.
Material and methods
The bone cements used were unloaded Palacos R and Palacos R with gentamicin, which contains 0.5 g gentamicin in every 40 g of polymer powder (Heraeus-Kulzer, Hanua, Germany). Different quantities (1, 2, 3, and 4 g) of gentamicin were also added to the polymer powder (40 g) of unloaded Palacos R bone cement. The gentamicin used was generic-grade gentamicin (Taresh Ltd., Banbridge, UK), which was to the standard of the British Pharmacopea. The gentamicin was incorporated using a small-scale turbo blender (Rondol Technology Stone, UK) at a speed of 1500 ± 50 rpm for 30 ± 1 s. After the gentamicin had been blended into the cement powder, both the polymer powder and liquid monomer were refrigerated for 24 ± 0.5 h at a temperature of 4 ± 0.5°C. Following refrigeration, the bone cements were mixed under ambient conditions using a commercially available vacuum system (Summit Medical Ltd., Bourton on the Water, UK) according to the manufacturer's instructions. Bone cement samples for mechanical testing were prepared by injecting the mixed bone cement into PTFE molds which were allowed to cure for a minimum of 24 ± 0.1 h. The samples were then removed from the mold. Rough edges of the specimen were removed by sanding with 1,200 µm grit silicon carbide abrasive. The cement samples for measurement of the compressive properties were cylinders of 12 ± 0.1 mm length and 6 ± 0.1 mm diameter (International Standard Organisation 5833 2002). The samples for determining the bending properties were 75 ± 0.5 mm in length, 10 ± 0.1 mm in width, 3.3 ± 0.1mm in thickness (International Standard Organisation Citation2002). The fatigue samples () were “dog-bone”-shaped: 75 ± 0.5 mm in length, 5 ± 0.1 mm in width, and approximately 3.5 ± 0.1 mm in thickness with a gauge length of 25 ± 0.1 mm (Dunne et al. Citation2003). For measurement of thermal properties, the bone cement was tested directly after the mixing phase, i.e. 45–60 s after opening of the ampoule of liquid monomer. Bone cement samples for determination of gentamicin release and biofilm formation were prepared by placing the mixed cement between two glass plates; following curing, 1.0 × 1.0 ± 0.1 cm2 sections were cut and stored under sterile conditions in the dark at room temperature. Unloaded Palacos R bone cement was used as a control in all experiments.
Figure 1. Dimensioned drawing of rectangular cross-sectional fatigue test specimen. Drawing not to scale
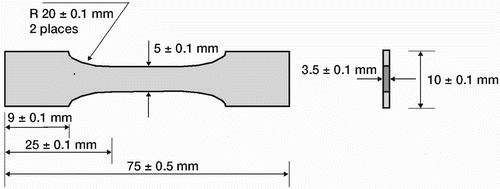
Mechanical, physical, and thermal properties
The compressive properties of each bone cement mix were determined in accordance with ISO 5833 (International Standard Organisation Citation2002) using a Lloyds materials testing machine (Lloyds Instruments, Fareham, UK), operating at a crosshead speed of 20 mm/min. Each compressive sample was tested to failure and a load vs. deformation plot recorded. A total of 30 specimens were tested for each bone cement mix.
The bending strength and modulus for each cement mix was determined using a 4-point bending test arrangement. All bending tests were conducted using the Lloyds materials testing machine operating at a crosshead speed of 5.0 mm/min. The load and deflection at mid-span were recorded to failure of each sample, and the bending modulus and bending strength were calculated according to ISO 5833 (International Standard Organisation Citation2002). 18 bone cement samples were tested for each cement mix.
The fatigue properties of each bone cement mix were assessed using a purpose-built pneumatically controlled testing machine (Zwick-Roell, Leominster, UK) and cycled continuously in load control until failure (Dunne et al. Citation2003). The cyclic stress employed was sinusoidal, at a frequency of 2 Hz. 18 samples were tested in tension-tension with a lower stress of 0.3 MPa and an upper stress of 22 MPa. All the mechanical testing was conducted in air at 23 ± 0.5°C. Each sample was tested until failure, and the maximum number of cycles at failure (Nf) was recorded.
In cemented total hip replacement surgery, data have been gathered to illustrate that fatigue is the in vivo failure mechanism of bone cement in the weak regions (Topoleski et al. Citation1993). Furthermore, fatigue is a stochastic process; thus, test data should be analyzed using appropriate probabilistic techniques (Lewis and Mladsi Citation1998). In this investigation, we tested the Nf results using a statistical technique that is widely used to study extremevalue data (the 3-parameter Weibull method) (Weibull Citation1961, Hastings and Peacock Citation1975), to ascertain estimates of three parameters: (1) b, the Weibull slope, which is a measure of the degree of dispersion or predictability of the Nf test results, such that the smaller b is, the larger is the dispersion, (2) No, the Weibull minimum fatigue life, which is the number of fatigue stress cycles above which all specimens have 100% probability of survival, and (3) Na, the Weibull characteristic fatigue life, which is the number of fatigue stress cycles at which 63.2% of the specimens survive. These estimates where then combined to quantify an overall index (I) of the material's fatigue performance (Shigley and Mischke Citation1989, Britton et al. Citation1990). A good fatigue performance (i.e. a high value of I) requires both a long fatigue life (i.e. a high value of Na) and low dispersion of the Nf results (i.e. a high value of b). Survival analysis using the Weibull relation has been used in several previous studies to analyze fatigue test data of cement specimens (Davies et al. Citation1987, Citation1989, Davies and Harris Citation1992, Lewis and Austin Citation1994, Topoleski et al. Citation1995). Other methods are available to analyze the random nature of fatigue data, such as the probability-of-fracture relationship, which permits estimation of fatigue life for a specified level of the probability of fracture (Lewis and Mladsi Citation1998). The fatigue characteristics determined using the probability-of-fracture model have been considered to be optimistic relative to those determined using the threeparameter Weibull model (Shigley and Mischke Citation1989). With regard to the selection of fatigue life of bone cement for total hip replacement surgery, it is suggested (Dunne et al. Citation2003) that conservative fatigue behavior values are preferable to optimistic ones.
We conducted fracture surface analysis on a selection of the compressive, bending, and fatigue samples from each bone cement mix using a Nikon optical microscope. The fractured surfaces were prepared for analysis by initial grinding on a grinding machine (Struers, Ballerup, Denmark) using a series of silicon carbide papers (600, 800, and 1,200 grit). Thereafter, the surfaces were polished on micro-cloths with 0.05–0.1 µm Al2O3 suspensions, gently wiped with a fine cloth to remove any scratches remaining from the grinding process, and viewed under the optical microscope.
The particle sizes and distribution of the blended bone cement powders were established using a laser diffraction system (Sympatec HELOS/BF Particle Sizer; Sympatec, Bury, UK).
The maximum temperature and setting parameters of each bone cement mix were measured in accordance with ISO 5833 (International Standards Organisation Citation2002). A PTFE mold was used to contain the bone cement during polymerization and a nickel/chromium/aluminium k-type thermocouple was used to measure the temperatures, which were recorded using the Labtech Notebook data acquisition software package (Adept Scientific, Letchworth Garden City, UK) (Dunne and Orr Citation2002). The mixing system, test equipment, and the constituents of the cement were conditioned at 23 ± 0.5°C and at a relative humidity of not less than 40 ± 1% for at least 2 ± 0.1 h before beginning the test. Temperature data were recorded at regular intervals of 1 s for a period of 30 min. Three bone cement samples were tested for each cement mix.
Gentamicin release rates
For the measurement of gentamicin release, cement sections for each gentamicin concentration were placed in 5 mL of sterile water and stored in the dark at 37 ± 0.5°C. At designated time intervals (6, 24, 48, and 72 ± 0.1 h), each section was removed and a 1 ± 0.1 mL sample of the water was taken and stored at –20 ± 0.5°C until analyzed. The section was then placed in 5 ± 0.1 mL of fresh sterile water until the next time point. All samples were analyzed for gentamicin by high-perfor-mance liquid chromatography and mass spectrometry using a method adapted from one previously described (Keevil et al. Citation2003), with gentamicin release expressed relative to the surface area of the cement section (µg/cm2). For measurement of release rate, 5 cement sections for each gentamicin concentration were taken; 3 readings were made for each time interval and the mean of these was taken. A mean for each of the 5 cement sections was then calculated.
Bacterial strains and biofilm formation
3 strains used in the study were clinical isolates cultured as described previously from implants retrieved from patients undergoing revision hip surgery (Tunney et al. Citation1998a). The isolates chosen, Staphylococcus epidermidis (BK 2), S. aureus (DC2), and S. capitis (ED2D) were used as representatives of the bacterial species that most frequently cause orthopedic implant infection (Tunney et al. Citation1998a). The susceptibility (minimum inhibitory concentration) of the isolates growing planktonically to gentamicin had been determined previously to be < 0.125 µg/mL (BK2 and ED2D) and 1 µg/mL (DC2) (Tunney et al. Citation1998b).
Biofilms were formed for each strain as described previously (Tunney et al. Citation2006), in triplicate on unloaded and gentamicin-loaded bone cement sections over designated time intervals (6, 24, 48, and 72 ± 0.1 h). For each strain tested, 12 bone cement sections for each gentamicin concentration were placed aseptically in separate sterile bottles with 3 cement sections used for each time point. The inoculum for each of the strains was prepared by adjusting the turbidity of an overnight broth culture in nutrient broth (NB) to an optical density at 550 nm equivalent to ∼1 × 107 cfu/mL. For each strain, 10 mL of the final inoculum was added to sterile bottles and the samples were then incubated at 37 ± 0.5°C for 6, 24, 48, and 72 ± 0.1 h. At each time point and for each gentamicin concentration, 3 bone cement sections were removed and gently washed with sterile phosphate buffered saline (PBS) to remove any non-adherent bacteria. The cement sections were then placed in PBS and the bacteria retained on the section were dislodged by mild ultrasonication (5 ± 0.1 min) followed by rapid vortex mixing (30 ± 1 s). Serial tenfold dilutions were prepared, and after overnight incubation the number of colony-forming units on each cement section was counted and expressed relative to the surface area of the section (cfu/cm2). The remaining 9 cement sections for each gentamicin concentration were removed, washed using PBS, and added to separate sterile bottles containing fresh prewarmed NB, which were incubated until the next time point. At following time points (i.e. 24, 48 and 72 h), bacterial colonization to an additional 3 cement sections for each gentamicin concentration was determined. The remaining 6 cement sections were washed with sterile water, added to fresh broth and incubated until the next time point.
Statistics
Data collated from all experimental tests were evaluated for statistical significance using a oneway analysis of variance with p < 0.05 denoting significance. Post hoc tests were conducted using the Student-Newman-Keuls and Duncan methods. All the tests were conducted using commercially available software (SAS version 8.02; SAS Institute, Cary, NC).
Results
Mechanical, physical, and thermal properties
There were no statistically significant differences between the mean compressive strength and bending strength of unloaded Palacos R and Palacos R with 0.5 g gentamicin (p = 0.8 and p = 0.4, respectively). However, when gentamicin was added to the unloaded cement in quantities of 1–4 g, there was a decrease (p < 0.001) in the mean compressive and bending strengths of the loaded cements in comparison with unloaded Palacos R and Palacos R with 0.5 g gentamicin ( and ). Reductions in compressive and bending strengths of greater than 15% and 18%, respectively, in comparison to unloaded cement were apparent. Further statistical analysis revealed, however, that the decrease in compressive and bending strengths was similar for each of the additional amounts of gentamicin added. In contrast to the results for mean compressive and bending strength, there was no significant difference in the mean bending modulus between the unloaded and gentamicin-loaded bone cements (p = 0.1) (Table). In terms of resistance of the bone cement to fatigue loading, differences (p < 0.001) were noted between the mean number of cycles to failure of unloaded Palacos R and Palacos R with 0.5 g gentamicin (. Moreover, when gentamicin was added to the unloaded cement in quantities of 1–4 g, there was a significant reduction in the fatigue performance of the bone cement in comparison with unloaded Palacos R and Palacos R with 0.5 g gentamicin (p < 0.001 in both cases). We observed comparable trends for the Weibull parameter estimates, which are summarized in and and in . A 64–81% reduction in the Weibull characteristic fatigue life, Na, was noted for Palacos R bone cement loaded with 1–4 g of antibiotic as compared to unloaded cement. The Weibull slope, b, indicates the degree of scatter within a particular set of fatigue data. From it can be seen that the Weibull slope for Palacos R bone cement containing 1–4 g of gentamicin was 32–56% lower than the b value determined for unloaded Palacos R bone cement. Similar trends were observed for the fatigue performance index (I) estimates (; the performance index for Palacos R bone cement containing 1–4 g of gentamicin was 70– 87% lower than the value determined for unloaded Palacos R bone cement.
Figure 2. Compressive strength (mean ± SD) of unloaded and gentamicin-loaded Palacos R bone cements. ‘a’ denotes a p-value less than 0.001, indicating a statistically significant difference between unloaded bone cement and cements loaded with 1–4 g of gentamicin. ‘b’ denotes a p-value less than 0.001, indicating a statistically significant difference between Palacos R cement with 0.5 g of gentamicin and Palacos R cement loaded with 1–4 g of gentamicin.
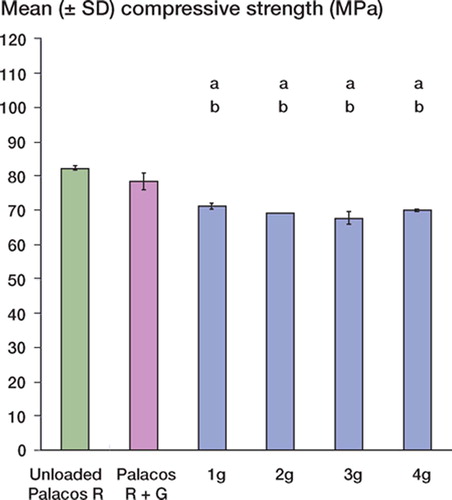
Figure 3. Bending strength (mean ± SD) of unloaded and gentamicin-loaded Palacos R bone cements. ‘a’ denotes a p-value less than 0.001, indicating a statistically significant difference between unloaded bone cement and cements loaded with 1–4 g of gentamicin. ‘b’ denotes a p-value less than 0.001, indicating a statistically significant difference between Palacos R cement with 0.5 g of gentamicin and Palacos R cement loaded with 1–4 g of gentamicin.
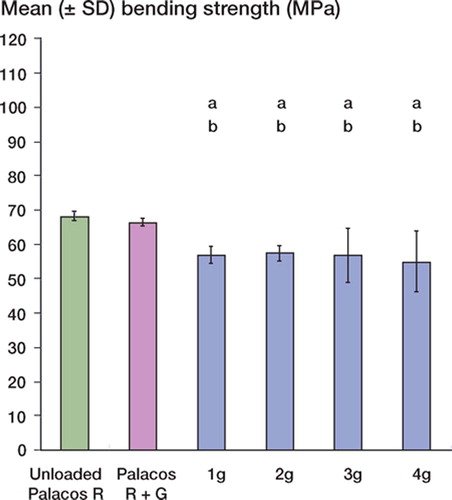
Figure 4. Fatigue data of unloaded and gentamicin-loaded Palacos R bone cements. (A.) number of cycles to failure (mean ± SD), (B.) Weibull slope, and (C.) fatigue performance. ‘a’ denotes a p-value less than 0.001, indicating a statistically significant difference between unloaded bone cement and cements loaded with 1–4 g of gentamicin. ‘b’ denotes a p-value less than 0.001, indicating a statistically significant difference between Palacos R cement with 0.5 g of gentamicin and Palacos R cement loaded with 1–4 g of gentamicin.
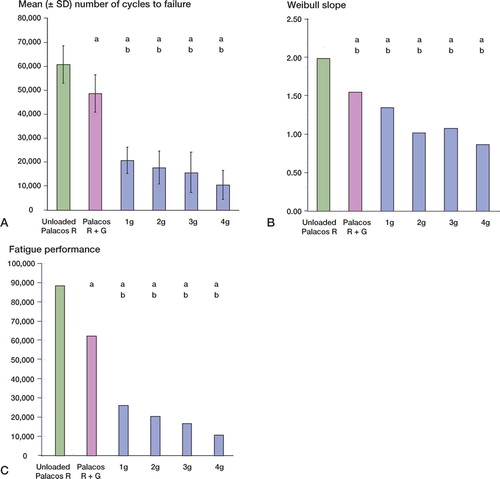
Summary of results from mechanical, physical, and thermal studies of unloaded and gentamicin-loaded bone cements
We used microscopy to visually assess the effect of incorporating additional amounts of gentamicin into the bone cement. shows a series of micrographs in which agglomerates of antibiotic can be identified as white clusters. It can be seen clearly that in the unloaded Palacos R and Palacos R with 0.5 g gentamicin, no antibiotic agglomerations are present. However, in the bone cements containing 1 or 2 g of gentamicin a small number of agglomerations are present, while there is a much larger number present in the cements containing 3 or 4 g of gentamicin. Furthermore, we observed that in addition to the finding that the number of agglomerations increased as the amount of gentamicin incorporated increased, the size of the agglomerations also increased.
Figure 5. Micrographs of unloaded and gentamicin-loaded bone cements in which antibiotic agglomerates can be identified as white clusters (arrows show examples). The micrographs of the unloaded Palacos R and Palacos R with 0.5 g gentamicin show no evidence of antibiotic agglomerations. The bone cements containing 1 g or 2 g of gentamicin show a small number of agglomerations within the cement microstructure, and a much larger number is present in the cements containing 3 g or 4 g of gentamicin.
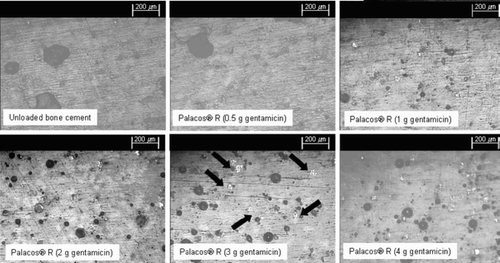
Incorporation of different amounts of gentamicin had no significant effect on either the mean particle diameter (p = 0.02) or mean particle distribution (p = 0.9); both of these parameters were similar for unloaded cement and for cement loaded with the different amounts of gentamicin (Table). Similarly, the incorporation of additional gentamicin had no effect on the thermal properties of the bone cement, with no significant differences in the setting time (p = 0.2) or the maximum exotherm temperature (p = 0.1) for the unloaded and gentamicin-loaded curing cements (Table).
Gentamicin release rates
summarizes gentamicin release as a function of time for unloaded Palacos R and Palacos R loaded with gentamicin. For all the bone cements tested, gentamicin release was most rapid during the first 6 h and continued at a much lower rate thereafter. Incorporation of additional amounts of gentamicin in the bone cement resulted in a significant difference in the total amount of gentamicin released over 72 h (p < 0.001).
Bacterial biofilm formation
We found strain-dependent differences in bacterial colonization on Palacos R bone cement containing different amounts of gentamicin (). Generally, the level of colonization with Staphylococcus spp. detected was lower (p < 0.001) on Palacos R with gentamicin (0.5–4 g) than on the unloaded cement. For S. epidermidis (, colonization at 6, 24, and 48 h was less (p < 0.001) on Palacos R with gentamicin (0.5 g) than on unloaded cement, but this beneficial effect was less significant at 72 h (p < 0.01). Although the incorporation of 1–4 g additional gentamicin prevented colonization at 6 h, by 72 h only the bone cement containing 4 g of additional gentamicin prevented colonization by S. epidermidis. biofilms developed in similar numbers on unloaded cement and cement loaded with 1, 2, or 3 g of additional gentamicin. In contrast, although the incorporation of additional gentamicin reduced or prevented colonization by the S. aureus () and . capitis () strains tested at 6 and 24 h, none of the bone cements containing additional gentamicin prevented colonization by these two strains at 48 and 72 h.
Figure 7. Colonization (mean ± SD) of unloaded and gentamicin-loaded bone cements by clinical prosthetic hip isolates: (A.) S. epidermidis, (B.) S. aureus, and (C.) S. capitis. ‘a’, ‘b’ and ‘c’ denote p-values less than 0.001, 0.01, and 0.05, respectively, indicating a statistically significant difference between unloaded bone cement and cements loaded with 1–4 g of gentamicin. ‘d’ and ‘e’ denote p-values less than 0.01 and 0.05, respectively, indicating a statistically significant difference between Palacos R cement with gentamicin and Palacos R cement loaded with 1–4 g of gentamicin.
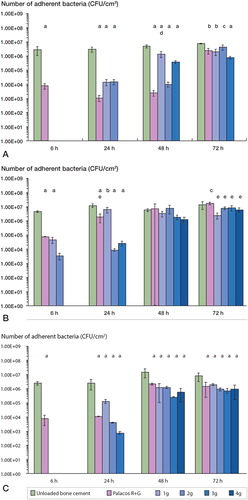
Discussion
Although manufacturers of bone cement currently recommend that additional gentamicin should not be added to bone cement in order to prevent detrimental effects on mechanical properties, orthopedic surgeons frequently add this antibiotic to bone cement in large quantities when infection is suspected at the time of revision surgery (Hanssen and Spangehi Citation2004). Although previous investigators have quantified the effect on mechanical properties of adding supplementary gentamicin to bone cement (Lautenschlager et al. Citation1976, Nelson et al. Citation1978, He et al. Citation2002, DeLuise and Scott Citation2004), none of the studies published to date have determined whether this practice is effective in the treatment and prevention of further infection. Consequently, in this study we investigated the effect of incorporating additional amounts of gentamicin on: (1) the mechanical, physical, and thermal properties of the bone cement, (2) gentamicin release from the bone cement, and (3) in vitro biofilm formation on bone cement by clinical isolates of Staphylococcus spp.
Addition of gentamicin to the bone cement in quantities greater than 0.5 g had a detrimental effect on the mechanical performance of the cement as measured by compressive, bending, and fatigue properties. These findings are similar to the results of previous studies (Lautenschlager et al. Citation1976, Nelson et al. Citation1978, Davies et al. Citation1989, He et al. Citation2002, DeLuise and Scott Citation2004). For example, Lautenschlager et al. (Citation1976) reported that adding up to 10 g of gentamicin to Simplex P bone cement caused a gradual, proportional decrease in the bulk mechanical properties of compressive and diametral tensile strengths. Similarly, Nelson et al. (Citation1978) found that although the addition of up to 2 g of gentamicin to Simplex P bone cement did not affect the tensile properties, the compressive strength of the cement was, however, adversely affected. Furthermore, He et al. (Citation2002) examined the relationship between the amount of gentamicin added to Palacos R bone cement and the compressive properties of the cement and reported that gentamicin concentrations of more than 3–4% resulted in a considerable reduction in the static compressive properties of the cement. Davies et al. (Citation1989) reported that the addition of 1 g of gentamicin to Palacos R cement significantly reduced the fatigue life. It is interesting to note from our static mechanical test data that the compressive and bending strength of Palacos R cement was immediately and significantly reduced by the incorporation of 0.5–1 g of antibiotic in Palacos R cement; this was followed by a gradual reduction in strength with increasing quantities of gentamicin. A similar trend was noted in the study by Lautenschlager et al. (Citation1976). It is postulated that the antibiotic is incorporated into the cement matrix as discrete particles not usually associated with the ever-present porosity, which appear to act as additional defects that significantly lower the compressive and bending strength of the cement above a threshold level of antibiotic loading, i.e. ≤ 0.5 and ≥ 1 g. It appears that beyond this level of loading, additional antibiotic blended into the polymer cement powder does not contribute significantly to the failure of Palacos R cement when subjected to a static load. The bending modulus was largely insensitive to additional quantities of antibiotic being incorporated into the polymer cement powder. Similar findings have been reported by other groups (Lautenschlager et al. Citation1976, He et al. Citation2002). A hypothesis to explain this finding would be that the antibiotic does not play an active role in the mechanical performance of the cement when it is being statically loaded within its elastic region. However, it should be noted that this explanation is only valid for the quantities of antibiotic investigated in our study. We postulate that at levels greater than 4 g of gentamicin per 40 g of cement polymer powder, a reduction in bending modulus would be observed.
The morphology of the fractured surfaces of the cured cements revealed clusters of gentamicin agglomerations or inclusions of large size, which could act as stress concentrations within the bone cement, thereby reducing the compressive, bending, and fatigue properties of the bone cement when subjected to static and dynamic loads. These antibiotic agglomerations were absent or present in very low numbers on the fractured surfaces of the cured cements containing a low dose of gentamicin (≤ 0.5 g). However, when a higher dose of gentamicin (≤ 0.5 and ≥ 4 g) was added, the antibiotic particles clustered together to form relatively large agglomerates on the fractured surfaces of the cured cements, which acted as stress concentrations and thereby reduced the compressive and bending properties of the cement. Furthermore, we postulate that the high level of dispersion (low Weibull slope, b) and low fatigue performance estimate of the Palacos R cement containing 1–4 g of gentamicin when subjected to fatigue loading may be related to the high incidence of antibiotic agglomerates at amounts of gentamicin of 1 g or more. Interestingly, these results contrast with the data from the particle size analysis. He et al. (Citation2002) have reported a similar observation; they observed that at low amounts of antibiotic (≤ 1 g), gentamicin was dispersed into isolated domains with some gentamicin particles completely surrounded by the cement matrix, whereas others were connected to the surface of the cement. At high antibiotic concentrations (amounts ≥ 1 g), the gentamicin domains were numerous and closely interconnected, thus forming agglomerations or inclusions.
We also found no significant differences between the thermal properties of unloaded Palacos R and antibiotic-loaded Palacos R bone cements. The maximum temperatures, setting temperatures, and times at maximum and setting temperatures determined in this investigation are similar to values reported for Palacos R bone cement in a previous study (Ginebra et al. Citation2001). Furthermore, other authors have also found no significant difference in the polymerization reactions for gentamicin-loaded Cemex XL bone cements mixed using a number of different methods (Lewis et al. Citation2005).
A number of studies have shown that the possibility of a hip prosthesis failing due to loosening increases as a result of a reduction in the mechanical properties of the bone cement used to support the prosthesis within the bone cavity (Murphy and Prendergast Citation1999, Dunne et al. Citation2003). Thus, incorporation of additional gentamicin into the bone cement could only be justified if its increased release from the cement resulted in both the eradication of any infection present at the surgical site and the prevention of infection of the new implant. Our findings show that incorporation of additional amounts of gentamicin did indeed result in a significant increase in gentamicin release from the bone cement. Furthermore, when an overt infection was simulated using clinical isolates of Staphylococcus spp. at an inoculum similar to that present as a result of an infected hip implant, the incorporation of additional gentamicin did result in an initial decrease in bacterial adherence relative to the level achieved with standard Palacos R supplied with gentamicin. However, this beneficial effect was no longer apparent by 72 h, as the clinical isolates formed biofilms on the bone cements despite the release of high levels of gentamicin. The susceptibility of the isolates growing planktonically to gentamicin, as determined by the minimum inhibitory concentration (Tunney et al. Citation1998b), did not affect the ability of the isolates to colonize and subsequently grow in a biofilm on the bone cement. biofilms were formed by all three isolates despite differences in their planktonic susceptibility to gentamicin. Furthermore, the concentration of gentamicin released by each of the bone cements was much higher than the concentration required to kill the isolates growing planktonically. Thus, the incorporation of additional gentamicin does not appear to be an effective method for the treatment of overt infection at the time of revision surgery. Moreover, incorporation of high levels of gentamicin might result in the prolonged release of low levels of the antibiotic, which could result in increased resistance of bacteria isolated from hip joints to gentamicin (Tunney et al. Citation1998b, Thomes et al. Citation2002).
Similar results have been reported in previous studies, which examined the incorporation of a second antibiotic, rather than additional gentamicin, to prevent bacterial adherence and biofilm formation. For example, it has been reported using a flowthrough system that bone cements loaded with a combination of gentamicin and clindamycin or fusidic acid are more effective in preventing biofilm formation by two Staphylococcus spp. isolates than bone cement containing only gentamicin (Neut et al. Citation2005). However, none of the combinations tested completely prevented biofilm formation by either of the isolates tested. In a further study using a prosthesis-related interfacial gap model, these authors reported no significant difference in the growth of gentamicin-resistant coagulase-negative Staphylococcus spp. in the interfacial gap prepared using bone cements containing gentamicin only and bone cements containing a combination of gentamicin and either clindamycin or fusidic acid (Neut et al. Citation2006).
In using the results from our in vitro study, some points must be borne in mind. First, we used only one bone cement formulation. It is well known that the properties of acrylic bone cement are formu-lation-specific (Lewis Citation1997). Secondly, only one type of antibiotic was used, with a specific particle size (28.46 ± 1.20 µm) and a specific morphology. Thirdly, we used only one mixing method to prepare the bone cement. It is well known that the mixing method has a significant influence on the properties of the cured bone cement (Lewis Citation1997). Fourth, a consistent, repeatable, mechanical blending regime was used to ensure full dispersion of the antibiotic within the polymer cement powder. It is anticipated that such a method of blending the antibiotic into the polymer cement powder would not be available in conventional surgical theaters. Fifth, it is anticipated that the method adopted for the determination of the degree of bacterial colonization on the unloaded and gentamicin-loaded bone cements by clinical prosthetic hip isolates is representative of what occurs in a post-surgical situation. Sixth, the release of gentamicin from the bone cement when immersed in sterile water at 37°C is an accurate representation of the in vivo dynamic of this antibiotic. However, this method has been adopted in a number of previous studies (Keevil et al. Citation2003, Tunney et al. Citation2006). A final point for consideration is that the activity and stability of the gentamicin was not monitored after release from the bone cement.
In summary, our study has shown that although the incorporation of additional gentamicin in Palacos R bone cement results in an increase in gentamicin release, the bone cements loaded with additional gentamicin are unlikely to eradicate an overt infection if present—or prevent infection of the newly implanted device at the time of revision surgery. Furthermore, as the incorporation of gentamicin in amounts greater than 0.5 g significantly reduces the mechanical properties of the bone cement, orthopedic surgeons should carefully consider whether there is any clinical benefit to this practice, given that it could result in premature failure of the prosthetic joint.
Contributions of authors
ND: data analysis and writing of the paper. JH: static mechanical testing. PMcA: static mechanical, thermal, and physical property testing. KT: dynamic mechanical testing. RK: measurement of gentamicin release and degree of biofilm formation. SP: data analysis and writing of the paper. MT: data analysis and writing of the paper.
No external funding was received for this study. The authors have no professional or financial affiliations that may be perceived to have biased the presentation.
No competing interests declared.
- Britton J, McInnes P, Ledoux W, Retief D. Shear bond strength of ceramic orthodontic brackets. Am J Orthod Dent Orthop 1990; 98(1)348–53
- Davies J, Harris W. The effect of addition of methylene blue on the fatigue strength of Simplex P bone cement. J Appl Biomater 1992; 3(1)81–5
- Davies J, O'Connor D, Greer J, Harris W. Comparison of the mechanical properties of Simplex, P., Zimmer Regular and LVC bone cements. J Biomed Mater Res 1987; 21(1)719–30
- Davies J, O'Connor D, Burke D, Harris W. Influence of antibiotic impregnation on the fatigue life of Simplex P and Palacos R acrylic bone cement, with and without centrifugation. J Biomed Mater Res 1989; 23(1)379–97
- DeLuise M, Scott C. Addition of hand-blended generic Tobramycin in bone cement: Effect on mechanical strength. Orthopedics 2004; 27(12)1289–91
- Dunne N, Orr J. Curing characteristics of acrylic bone cement. J Materials Science: Materials in Medicine 2002; 13(1)17–22
- Dunne N, Orr J, Mushipe M, Eveleigh R. The relationship between porosity and fatigue characteristics of bone cement. Biomaterials 2003; 24(2)239–45
- Engesaeter L, Lie S, Espehaug B, Furnes O, Vollset S, Havelin L. Antibiotic prophylaxis in total hip arthroplasty-Effect of antibiotic prophylaxis systemically and in bone cement on the revision rate of 22,170 primary hip replacements followed 0–14 years in the Norwegian arthroplasty register. Acta Orthop Scand 2003; 74(6)644–51
- Espehaug B, Engesaeter L, Vollset S, Havelin L, Langeland N. Antibiotic prophylaxis in total hip arthroplasty-Review of 10,905 primary cemented total hip replacements reported to the Norwegian arthroplasty register, 1987 to 1995. J Bone Joint Surg (Br) 1997; 79: 590–5
- Ginebra M, Morejon L, Delgad J, Manero J, Gil F, Artola A, Goni I, Gurruchaga M, Vaquez B, San Roman J, Planell J (2001) Effect of size distribution of the PMMA beads on the fatigue crack propagation of acrylic bone cements. Transactions of the 16th European Conference on Biomaterials, LondonUK, September, 2001, T20
- Hanssen A, Spangehi M. Practical applications of antibi-otic-loaded bone cement for treatment of infected joint replacements. Clin Orthop 2004, 427: 79–85
- Hastings N, Peacock J. Statistical distributions. Butterworth, London 1975
- He Y, Trotignon J, Loty B, Tcharkhtchi A, Verdu J. Effect of antibiotics on the properties of poly(methylmethacrylate)-based bone cement. J Biomed Mater Res 2002; 63(6)800–6
- International Standards Organisation 5833: Implants for surgery – Acrylic resin cement 2002.
- Keevil B, Lockhart S, Cooper D. Determination of tobramycin in serum using liquid chromatography-tandem mass spectrometry and comparison with a fluorescence polarisation assay. J Chromatography B 2003; 794: 329–35
- Lautenschlager E, Jacobs J, Marshall G, Meyer P. Mechanical properties of bone cements containing large doses of antibiotic powders. J Biomed Mater Res 1976; 10(6)929–38
- Lewis G. The properties of acrylic bone cement: a state-of-the-art-review. J Biomed Mater Res: Appl Biomater 1997; 38: 155–82
- Lewis G, Austin G. Mechanical properties of vacuum mixed acrylic bone cement. J Appl Biomater 1994; 5(1)307–14
- Lewis G, Mladsi S. Effect of sterilisation method on properties of Palacos R acrylic bone cement. Biomaterials 1998; 19: 117–24
- Lewis G, Janna S, Bhattaram A. Influence of the method of blending antibiotic powder with an acrylic bone cement powder on physical, mechanical, and thermal properties of the cured cement. Biomaterials 2005; 26: 4317–25
- Malchau H, Herberts P, Eisler T, Garellick G, Soderman P. The Swedish Total Hip Replacement Register. J Bone Joint Surg (Am) 2002; 84: 2–20
- Murphy B, Prendergast P. Measurement of non-linear microcrack accumulation rate in polymethylmethacrylate bone cement under cyclic loading. J Materials Science: Materials in Medicine 1999; 10(12)779–81
- Nelson R, Hoffman R, Burton T. Effect of antibiotic additions on the mechanical properties of acrylic cement. J Biomed Mater Res 1978; 12(4)473–90
- Neut D, de Groot E, Kowalski R, van Horn J, van der Mei H, Busscher H. Gentamicin loaded bone cement with clindamycin or fusidic acid added: biofilm formation and antibiotic release. J Biomed Mater Res A 2005; 73(2)165–70
- Neut D, Hendriks J, van Horn J, Kowalski R, van der Mei H, Busscher H. Antimicrobial efficacy of gentamicin-loaded acrylic bone cements with fusidic acid or clindamycin added. J Orthop Res 2006; 24(2)291–9
- Shigley J, Mischke C. Mechanical engineering design5. McGraw-Hill, New York 1989
- Thomes B, Murray P, Bouchier-Hayes D. Development of resistant strains of Staphylococcus epidermidis on genta-micin-loaded bone cement in vivo. J Bone Joint Surg (Br) 2002; 84(5)758–60
- Topoleski L, Ducheyne P, Chuckler J. Microstructural pathway of fracture in poly (methylmethacrylate) bone cement. Biomaterials 1993; 14: 1165–72
- Topoleski L, Ducheyne P, Chuckler J. The effects of centrifugation and titanium fibre reinforcement on fatigue failure mechanisms in poly (methylmethacrylate) bone cement. J Biomed Mater Res 1995; 29(1)299–307
- Tunney M, Patrick S, Gorman S, Nixon, Anderson N, Davis R, Hanna D, Ramage G. Improved detection of infection in hip replacements. A currently underestimated problem. J Bone Joint Surg (Br) 1998a; 80: 568–72
- Tunney M, Ramage G, Patrick S, Nixon J, Murphy P, Gorman S. Antimicrobial susceptibility of bacteria isolated from orthopaedic implants following revision hip surgery. Antimicrob Agents Chemother 1998b; 42(11)3002–5
- Tunney, M, Dunne N, Einarsson G, McDowell A, Kerr A, Patrick S. Bacterial biofilm formation by bacteria isolated from retrieved failed prosthetic hip implants in an in vitro model of hip arthroplasty antibiotic prophylaxis. J Orthop Res 2006; 12(1)1–9
- Weibull W. Fatigue testing and analysis of results. Macmillian, New York 1961