Abstract
Introduction Bisphosphonates have been proposed to delay or prevent loosening of joint replacement implants by reducing bone resorption. It is known, however, that implant motion prevents the bone anchorage necessary to maintain secure implant fixation.
Methods We used our experimental implant model with controlled motion to evaluate the relative effects of implant motion and bisphosphonate. We implemented our established 8-week experimental revision protocol to obtain a bony and soft tissue setting of revision joint replacement in 16 dogs. At 8 weeks, we had stabilized half of the implants. The other half of the implants continued pistoning. Half of the dogs were exposed to alendronate (oral).
Results Stabilization of the revision implant was more effective at improving fixation (higher shear strength) than administering alendronate. As expected, the fibrous membrane remained under unstable conditions, even with alendronate. With alendronate and stabilized implants, increased bone was observed near the sclerotic shell of the revision cavity, but it was reduced with alendronate when the implant was unstable.
Interpretation Our findings suggest that it may be difficult for alendronate administration alone to rescue implants that are already loose. In implants that have not progressed to loosening, alendronate may increase bone density at the border with the sclerotic shell, but the effect of this bone in delaying eventual loosening is not known.
Revision joint replacement implants have shorter longevity, poorer functional outcome, higher costs, and longer rehabilitation times than primary implants. Implant longevity requires durable fixation to the bone. In the revision setting, implant fixation is impaired for reasons that include bone loss due to the process of loosening—with poorer mechanical fixation in the revision cavity (Dohmae et al. Citation1988)—and higher levels of inflammatory cytokines with impaired bone healing.
Bisphosphonates impair the activity of osteoclasts, and thereby reduce bone resorption (Cecchini et al. Citation1987). These anti-resorptive agents have become established clinical tools for prevention and treatment of osteoporosis and fractures (Tucci et al. Citation1996, Rodan Citation1997, Black Citation2000, Fleisch Citation2002, Bauer et al. Citation2006)
Several strategies have been suggested for the use of bisphosphonates in the revision setting. With one strategy, revision surgery could be delayed in an implant at risk of loosening by the use of bisphosphonates. The aim of this strategy is to reduce resorption associated with peri-implant osteolysis (Schanbhag et al. Citation1997). With another strategy, primary implant longevity could be improved (and eventual revision prevented) by the use of bisphosphonates immediately postoperatively. The aim of this second strategy is to reduce peri-implant resorption caused by necrosis secondary to cutting and preparing the bony bed for insertion of the primary implant. In a third strategy, bone stock could be restored during revision surgery by local application of bisphosphonate and allograft, and thereby potentially maintain bone stock as a durable interface grows (Jeppsson et al. Citation2003).
In the present study we investigated the first strategy—of preventing the progression of osteolysis when an implant may already be at risk of loosening. We implemented our 8 week protocol to engender a setting of revision joint replacement (Bechtold et al. Citation2001a, Citationb, Soballe et al. Citation2003, Citation2004, Vestermark et al. Citation2004). At this 8 week time point, when a bone and tissue reaction of a clinically loose implant had occurred, we exposed half of the implants to systemic alendronate. Control implants received no alendronate. Then we exposed half of the implants to alendronate, with control implants receiving no alendronate.
Implant motion has been shown to be a major factor in directing the tissue response at the implant interface (Aspenberg et al. Citation1992, Søballe et al. Citation1992, Citation1993, Aspenberg and Herbertsson Citation1996, Bechtold et al. Citation2002) but the effect of bisphosphonate under controlled motion conditions is not known. Since implants that fail due to loosening have inherent relative motion, the influence of implant motion with bisphosphonate is important to determine. Since our experimental model allows relative implant motion to be controlled, we could examine the effect of implant interface motion both separately and combined with the effect of alendronate.
Following implementation of the revision protocol, we tested the following hypotheses based on mechanical and histomorphometric measures representing the integrity of the interface. (1) An implant with no relative motion at the revision bone-implant interface (i.e. an implant that has been stabilized) has better fixation than an implant with continuing relative motion at the revision bone-implant interface, regardless of alendronate status. (2) A revision implant with systemic alendronate exposure has better fixation than a revision implant without systemic alendronate exposure. (3) The improvement in fixation with alendronate is greater for revision implants that have been stabilized than for those with continued motion.
Methods
All procedures in this study were approved by the Animal Care and Use Committee of the Minneapolis Medical Research Foundation.
The revision protocol is based on the Søballe micromotion device (clinically a loose, pistoning implant) (). Plasma-sprayed titanium alloy (Ti) implants were used with the micromotion device. The cylindrical implants had a nominal diameter of 6.0 mm and an overall length of 10 mm, and consisted of a threaded Ti-6Al-4V alloy core with a coating of Ti-6Al-4V deposited by plasma-spray technique, resulting in a pore size of 200–1,000 μm at the substrate and the surface of the coating, respectively (Søballe et al. Citation1992, Citation1993).
Figure 1. At the first surgery, the revision protocol was initiated to engender a tissue reaction similar to that surrounding an aseptically loosened implant. Specifically, a 6.0-mm plasma-sprayed titanium implant axially pistoned 0.5 mm concentrically in a hole with a 0.75-mm gap. The implant pistons inward by engagement of the PE endcap with the tibial plateau. Following the loading episode, the internal spring pushes the implant outwards to move it back to its original position. The implants were weight-bearing in each medial femoral condyle for 8 weeks in the presence of PE particles (0.5–50 μm; 0.5 × 108particles; 85% < 12 μm). Following insertion of the implant, the surgical site was not lavaged further nor irrigated. At the second surgery 8 weeks later, the right-side implant was modified to prevent it from pistoning (i.e. the PE end plug was cut to prevent engagement with the tibia on the opposite side). The left side implant continued to piston after it was observed in a sham operation.
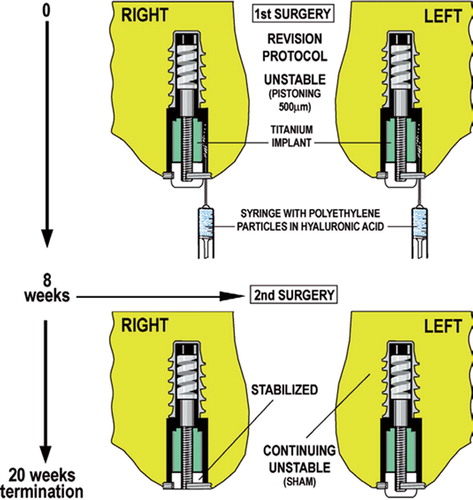
Operative procedure for insertion of micromotion device ()
Dogs were placed under general anesthesia, sterile technique was observed, and perioperative antibiotics were given (cephalexin: 1 g intravenously preoperatively, 1 g intra-operatively, and 1 g every 8 h for 1 day). Following medial arthrotomy, a guide wire was inserted into a 2.1-mm hole and spatially oriented to pass through the weight-bearing articulating surface and remain within the central portion of the condyle. A cannulated hand drill created a deep cavity (6 mm diameter by 10 mm long) and a superficial cavity (7.5 mm diameter by 20 mm long), with the most superficial 4 mm being tapped. The implant was weight bearing and pistoning in the medial femoral condyles for 8 weeks in the presence of polyethylene (PE) (0.5–50 μm; 0.5 × 108particles; 85% < 12 μm) (Bechtold Citation2001a, Citationb). The Ti implant and then the PE cap were screwed onto the distal portion of the threaded piston. The internal spring of the micro-motion device pushes the mounted Ti implant back out after it is pushed inwards during weight bearing, and this was checked intra-operatively using a custom spring gauge. Soft tissues were closed routinely, and anteroposterior radiographs were taken immediately to verify implant placement. After a postoperative recuperation period of approximately 3 days, the dogs were housed one per cage and allowed unlimited cage activity in 24 square foot runs. Their hind-limb function was assessed and noted daily, to ensure they were weight bearing their implants on a regular basis. During the 8-week period required to establish the revision setting, the dogs were allowed 2 h per day of free exercise.
Second (revision) surgery
At 8 weeks, without disturbing the fibrous interface, a second surgical procedure was performed on both knees under general anesthesia and with sterile technique. Half of the implants were stabilized by cutting the polyethylene end cap flush with the titanium centralizing ring so that it no longer protruded past the cartilage surface. This prevented relative pistoning motion, and the implants were considered “stabilized”.
The contralateral implant remained unstable with a sham operation that opened the joint, examined the implant, and verified pistoning (the so-called “unstable” implant).
Experimental design
This revision protocol was initiated in 32 implants (16 dogs). The dogs were skeletally mature female hound dogs (between 50 and 96 weeks of age) with a mean weight of 19 kg (16–21 kg). There were no statistically significant differences in weight and age between treated and untreated groups (p = 0.28).
After 10 weeks of alendronate administration, the dogs were killed and necropsy was conducted. The knee joints were removed en bloc, placed in airtight plastic bags, and frozen at –20°C for later processing.
Alendronate dosage
2 weeks after the second surgery, a bisphosphonate (alendronate, 0.5 mg/kg/day orally; Merck) was given orally by capsule to half of the dogs (randomly chosen). The other dogs received nothing. To ensure absorption, all dogs were kept from food for 1 h after administering the alendronate to the treatment group.
Specimen preparation
The implant and surrounding bone was cut into two pieces perpendicular to the long axis of the implant using a water-cooled diamond band saw and predrilled alignment cannula.
The tissue response was evaluated in blind fashion. Mechanical interface fixation was determined by static axial pushout tests. Histomorphometric measures of bone area and its distribution represented the detailed localized tissue response. 1 transverse block was used for pushout testing and it was stored frozen at –20°C. The other block was used for histomorphometry, and its preparation is described below.
Mechanical properties (interfacial shear strength, stiffness, energy)
The distal transverse section was kept frozen at –20°C, and was thawed prior to pushout testing. The specimens were placed on the metal platform of a universal test machine (Model 4302, Instron, UK), with a central circular opening supporting the bone to within 500 μm of the interface. A metal rod was placed in the upper holding device for the axial pushout test of the implant from the surrounding tissues. A displacement rate of 5mm/min was used for all tests and load-deformation curves were obtained. Ultimate shear strength and apparent shear stiffness were estimated from the loaddisplacement curves (Søballe et al. Citation1992, Citation1993).
Quantitative histomorphometric analysis
The specimens were fixed in 70% ethanol, dehydrated in graded ethanol (70–99%), embedded in methylmethacrylate, and cut (EXACT Apparatebau GmbH & Co., Norderstedt, Germany) to a thickness of 50 μm. The ground sections were stained with basic fuchsin and counterstained with light green. To verify that the polyethylene particles were retained in the peri-implant space, polarization microscopy was used.
The procedure was performed blind and in random order with a microscope feeding an image to a computer via a video camera using a standard digitizing method (Bioquant software; R&M Biometrics, Nashville, TN). Histomorphometric measurements gave the amount of bone in contact with the implant, as well as the amount of bone located in 2 zones. Zone 1 was defined as extending from the implant to 50% of the drill gap, and zone 2 was defined as extending from 50% of the gap to 95% of the radius of the original drill gap. 3–4 transverse sections from the same implant were sampled.
Statistics
Repeated measures considered the two different stability conditions (stable/unstable) within a single animal. This was necessary since animals were treated systemically with the alendronate (or control) and hence both right and left sides were treated with the same alendronate condition.
Calculation of sample size was based on the following equation: n1 = n2 = 2(t2α + tβ)2× SD2/ D2. Error of the first kind (2α) was selected to be 5%. Based on our previous studies, an SD of 50% was chosen for both mechanical and histological data. The minimal clinically relevant difference (D = MIREDIF) not to be overlooked between groups was selected to be 80%. Error of the second kind (β)—the risk of concluding that two effects are identical if in fact the difference is below the MIREDIF (false negative result)—was chosen to be 20%. Based on these assumptions, and to account for loss of a specimen, 8 implants per group were included.
Results
All dogs completed the study. No clinical signs of infection were seen at the time of necropsy or dissection for any of the implants. All bacterial cultures taken at the same time were negative. The mechanical pushout data were lost for 1 animal in each group (“+ alendronate” and “– alendronate” groups). Histomorphometric data were lost for 1 dog in the “– alendronate” group.
Mechanical shear properties of the interface ()
Motion effect. Strength was lower for implants that remained unstable (i.e. showing movement) than for implants that were stable (showing no movement) (p = 0.05), independently of alendronate treatment. Similarly, stiffness and energy were lower for implants that remained unstable, independently of alendronate treatment, but this was not statistically significant (p = 0.1 for both).
Figure 2. Implant shear pushout test. Bars represent stable and unstable implants with and without the bisphosphonate alendronate (+ BP and – BP).
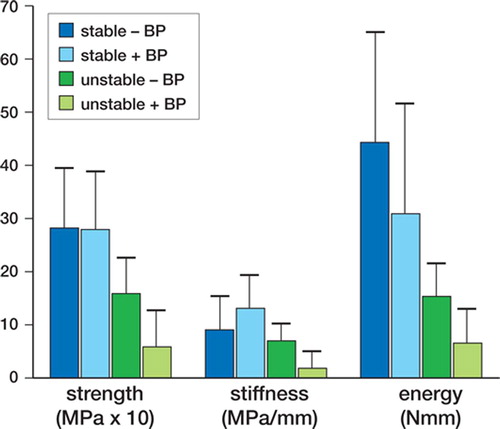
Alendronate effect. There was no significant difference in strength, shear stiffness, or energy between the “+ alendronate” and “– alendronate” groups, either for implants that were stable or for those that remained unstable.
Interaction between motion and alendronate status. There were no interaction effects between motion and alendronate status for any of the mechanical parameters.
Histomorphometry ()
Motion and alendronate effects. There were no statistically significant differences in histomorphometric measures due to stability or alendronate effects (stability effect: % contact, p = 0.2; % of bone in zone 1, p = 0.1; and % of bone in zone 2, p = 0.3; alendronate effect: p < 0.5)
Figure 3. Mean and standard error for percentage of bone in contact with implant (bone ongrowth), percentage of bone in zone 1 (half of gap closest to the implant surface) and percentage of bone in zone 2 (half of gap closest to the original drill hole), for stable and unstable implants with and without the bisphosphonate alendronate (+ BP and – BP).
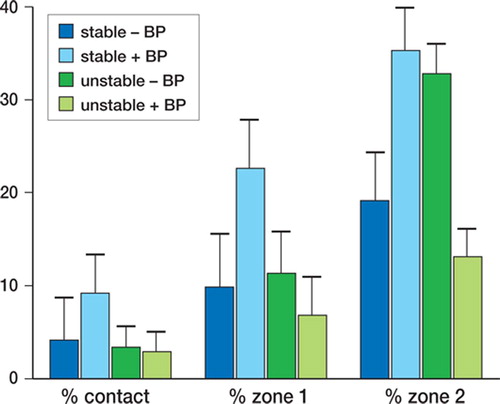
Interaction between motion and alendronate status. There was a statistically significant interaction effect between stability and alendronate status, with the % of bone in zone 2 (p = 0.001). For stable implants, the proportion of bone in zone 2 decreased with alendronate. Without alendronate, there was 35.% bone in zone 2 (SE 4.7) and the corresponding value with alendronate was 19.% (SE 5.1). Conversely, for unstable implants the amount of bone in zone 2 increased with alendronate. Without alendronate, the proportion of bone in zone 2 was 13.% (SE 3.0), and with alendronate it was 33.% (SE 3.2). For bone in contact with the implant and bone in zone 1, when implants were stabilized there was a tendency for the histomorphometric measures to indicate more bone in the alendronate group, but this was not statistically significant. Conversely, when implants remained unstable there was a tendency for the histomorphometric measures to indicate less bone in the alendronate group, but this was not statistically significant.
Discussion
Clinically, alendronate treatment has been proposed as a means of delaying or avoiding revision surgery for implants that are at risk of failure due to loosening (Schanbhag et al. Citation1997, Wise et al. Citation2005). Our study used a model of the revision implant setting, which allowed the potentially confounding effect of relative implant motion to be controlled for. Specifically, we studied the separate and combined effects of alendronate and relative implant stability. We found that the most effective strategy for improving the mechanical strength of the interface in the revision setting was to stabilize the implant. This is consistent with the commonly accepted necessary role of a stable environment in fostering bone growth, both for joint replacement implants and for fracture fixation. Regarding the effect of alendronate, the results were mixed. When implants remained unstable, alendronate treatment did not improve any measure of implant fixation significantly. When implants were stable, alendronate treatment was associated with histomorphometrically more bone in the outer zone of the original gap (adjacent to the sclerotic bone rim that formed during the process of loosening).
Our data must be interpreted within the context and limitations of our protocol and model, i.e. using our specific revision protocol, and after 10 weeks of relatively high-dose alendronate (oral 0.5 mg/ kg/day). These limitations are discussed below.
We experimentally reproduced localized conditions of a revision setting using our weightbearing revision implant model (Bechtold et al. Citation2001a, Citationb, Søballe et al. Citation2003, Citation2004, Vestermark et al. Citation2004). This established revision protocol produces a controlled periprosthetic tissue reaction in the canine knee. This reaction consists of dense layers of fibrous tissue with synovial-like lining cells, macrophages with ingested particulate polyethylene (PE), elevated inflammatory cytokines, and a sclerotic bone rim (neocortex) that forms at the original bone border. Using this experimental model, one has intraarticular access to the implant interface, which allows pumping of joint fluid along the interface. Also, as for loosened implants in humans, compared to primary implants there is increased joint fluid and a thickened joint capsule. While revision joint replacements are clearly complex and present with varying degrees of bone loss, this model represents the sclerotic region adjacent to cancellous bone. This region is critical for secure overall fixation of revision implants. Here, we have used the model to determine whether the poor mechanical interface inherent in the revision setting can be rescued with systemic bisphosphonate administration, and to investigate the nature of the confounding effect of an unstable implant bone interface.
To create stabilized or continuously moving implants, we used two forms of the polyethylene endcap that protrudes into the knee joint. To stop articulation and hence stabilize the implant, the endcap was shaved flush with the joint surface during an operative procedure at 8 weeks. The continuous motion group had no alteration of the endcap during a sham operation at the same time point. It should be noted that in this model stabilization of the implant without removing it (i.e. when preserving the fibrous interface) necessarily converted an unstable, weight-bearing implant into a stable, non-weight-bearing implant. Communication of joint fluid was maintained in both groups, however, and the femoral condyles surrounding the implant continued to be weight bearing through articulation with the tibial plateau.
Clearly, human implants present for revision with a wide range of bone loss and structural support issues. Our intention is not to present the entire range of factors that can affect the fixation of revision implants. Rather, our revision model focuses on the interface between a revision implant and the surrounding proximal cancellous bone. Our aim is to improve revision implant fixation in the devitalized sclerotic shell (neocortex) with dense fibrous tissue that forms in the cancellous region (Kold et al. Citation2005). Secure fixation at this site is critical for long-term fixation of an implant.
The dosage and administration time of bisphosphonate can affect bone response (Chappard et al. Citation1991, Garcia-Moreno et al. Citation1998, Eberhardt et al. Citation2005). The effect of the alendronate time protocol and dosage used in our study has been shown previously to have a positive affect on the micro-CT and 3-dimensional mechanical properties of their lumbar vertebrae, humeral head, and greater tuberosity, in the very same dogs as used in this present study (Hu et al. Citation2002). Also, a separate study investigating a different implant setting has been conducted using the same dogs. Fixation of primary press-fit implants was improved in the animals exposed to alendronate, whereas primary fixation was not improved for implants surrounded by a gap (Jensen et al. Citation2007). From these companion studies, we consider that our time course and dosage was sufficient to have influenced bony response at the implant interface in this particular animal model.
Since absorption of alendronate has been reported to be between 15% and 20% higher in dogs than in humans (Michael et al. Citation1972), the available dose of alendronate in this study is estimated to have been closer to 3 times the usual clinical dose. In an implant instability study in rats, Astrand and Aspenberg (Citation2002) found that alendronate reduced bone resorption only at high doses. Eberhardt et al. (Citation2005) reported similar findings with ibandronate in rats. While not directly comparable to this study, along with the micro-CT and press-fit studies these findings support the use of a higher dose of alendronate in this study for the purpose of improving implant fixation.
Bisphosphonate administration has been studied in several animal models of impaired implant fixation. In a canine model, Shanbhag et al. (Citation1997) showed that daily use of 5 mg oral alendronate inhibited bone lysis in a particle-induced uncemented femoral implant failure study. In a canine particle-induced total hip failure model, Wise et al. (Citation2005) reported improved material properties of cortical bone with two doses of zoledronate. In a rat model of a 1.3-mm diameter polyethylene implant with press fit into the tibia, intra articular injection of polyethylene particles caused substantial bone loss (Millett et al. Citation2002). Following intravenous administration of alendronate (miniosmotic pump; 0.01 mg/kg/day), bone volume was similar to counterparts not exposed to particles. While these results are encouraging, it should be noted that the experimental protocols did not allow mechanical stability or weight bearing to be controlled (Mouzin Citation2001).
A nonoperative treatment that could prevent or delay the need for surgical intervention would be preferred by the patient and would reduce healthcare costs. While bisphosphonate is a candidate nonoperative treatment, when controlling for implant motion, we were unable to confirm the findings of an improved bone implant interface following bisphosphonate administration (Bhandari et al. Citation2005). Mechanical fixation and histomorphometric bone distribution improved with alendronate only after stabilization of our implants. Unfortunately, stabilization of an implant clinically requires operative intervention (such as removal of the loose device and replacement with a new implant, or addition of interlocking screws).
While other scenarios for experimental investigation of revision implants have been designed to address other specific questions (Shanbhag et al. Citation1997, Aspenberg and Van der Vis Citation1998, Millett et al. Citation2002, Astrand et al. Citation2003, Wise et al. Citation2005), our protocol was designed to either permit or prohibit relative implant motion. This allowed the potentially confounding variable of relative implant motion to be identified in a weight-bearing model exposed to joint fluid along the implant interface.
In summary, with our model using a controlled motion device, stabilization of the revision implant interface was more effective at improving fixation (shear strength) than the administration of alendronate. The bone near the sclerotic shell of the revision cavity was increased with alendronate when the implant was stable, but was reduced with alendronate when the implant was unstable. As expected, the fibrous membrane remained under unstable conditions despite administration of alendronate. The results of this study suggest that it may be difficult for alendronate administration alone to rescue implants that are already loose. In implants that have not progressed to loosening, alendronate may be capable of increasing bone density at the border with the sclerotic shell, but the effect of this bone in delaying eventual loosening—or whether it could have the negative effect of isolating the prosthesis more completely, physically and biologi-cally—is not known. Further clinical studies that investigate such questions are needed.
This work was funded by grants from the NIH (AR4205), the Arthritis Foundation, and Minnesota Supercomputing Institute. Biomet provided the implants, and Merck provided alendronate and a Medical School Grant to JEB. The assistance of Dr. Robert E. Sherman with statistics is gratefully acknowledged.
In memory of Professor Flemming Melsen (1938–2005), and in grateful appreciation of his generous and invaluable advice and his keen insight into the histological and histomorphometric evaluation of the bone-implant interface over more than two decades of collaboration with our research group.
Contributions of authors
KS: study design, experimental surgeries, data analysis and interpretation, and manuscript preparation. XC: study design, assistance with experimental surgeries, and administration of bisphosphonate. TJ: study design, data analysis and interpretation, and manuscript preparation. LK: study design, histomorphometric analysis, and manuscript preparation. JB: study design, assistance with experimental surgeries, data analysis and interpretation, and manuscript preparation.
References
- Aspenberg P, Herbertsson P. Periprosthetic bone resorption. Particles vs. movement. J Bone Joint Surg (Br) 1996; 78(4)641–6
- Aspenberg P, Van der Vis H. Migration, particles and fluid pressure A discussion of causes of prosthetic loosening. Clin Orthop 1998; 352: 75–80
- Aspenberg P, Goodman S, Toksvig-Larson S, Ryd L, Albrektsson T. Intermittent micromotion inhibits bone ingrowth. Titanium implants in rabbits. Acta Orthop Scand 1992; 63(2)141–5
- Astrand J, Aspenberg P. Alendronate did not inhibit instability-induced bone resorption A study in rats. Acta Orthop Scand 1999; 70(1)67–70
- Astrand J, Aspenberg P. Reduction of instability-induced bone resorption using bisphosphonates High doses are needed in rats. Acta Orthop Scand 2002; 73(1)24–30
- Astrand J, Skripitz R, Skoglund B, Aspenberg P. A rat model for testing pharmacologic treatment of pressure-related bone loss. Clin Orthop 2003; 409: 296–305
- Astrand J, Aspenberg P. Topical, single dose bisphosphonate treatment reduced bone resorption in a rat model for prosthetic loosening. J Orthop Res 2004; 22(2)244–9
- Bauer D C, Garnero P, Hochberg M C, Santora A, Delmas P, Ewing S K, Black D M. for the Fracture Intervention Research Group Pretreatment levels of bone turnover and the antifracture efficacy of alendronate: the fracture intervention trial. J Bone Miner Res 2006; 21(2)292–9
- Bechtold J E, Soballe K, Kubic V, Gustilo R. A controlled experimental model of revision implants Part I. Acta Orthop Scand 2001a; 72(6)642–9
- Bechtold J E, Mouzin O, Kidder L, Soballe K. A controlled experimental model of revision implants Part II. Acta Orthop Scand 2001b; 72(6)650–6
- Bechtold J E, Soballe K, Overgaard S, Kubic V, Lewis J. Bone ingrowth in the presence of particulate polyethylene, synergy between interface motion and periprosthetic tissue response. J Bone Joint Surg (Br) 2002; 84(6)915
- Bhandari M, Bajammal S, Guyatt G H, Griffith L, Busse J W, Schunemann H, Einhorn T A. Effect of bisphosphonates on periprosthetic bone mineral density after total joint arthroplasty. A meta-analysis J Bone Joint Surg (Am) 2005; 87(2)293–301
- Black D M, Thompson D E, Bauer D C, Ensrud K, Musliner T, Hochberg M C, Nevitt M C, Surywanshi S, Cummings S R. The FIT Research Group Fracture Intervention Trial. The FIT Research Group Fracture risk reduction with alendronate in women with ostheoporosis: the fracture intervention trial. J Clin Endocrinol Metab 2000; 85(11)4118–24, Erratum in J Clin Endocrinol Metab 2001; 86 2 938
- Cecchini M G, Felix R, Fleisch H, Cooper P H. Effect of bisphosphonates on proliferation and viability of mouse bone marrow ferived macrophages. J Bone Miner Res 1987; 2(2)135–42
- Chappard D, Petitjean M, Alexandre C, Vico L, Minaire P, Riffat G. Cortical osteoclasts are less sensitive to etidronate than trabecular osteoclasts. J Bone Miner Res 1991; 7: 673–80
- Dohmae Y, Bechtold J E, Sherman R E, Puno R M, Gustilo R B. Reduction in cement-bone interface shear strength between primary and revision arthroplasty. Clin Orthop 1988; 236: 214–20
- Eberhardt C, Schwarz M, Kurth A H. High dosage treatment of nitrogen-containing bisphosphonate ibandronate is required for osseointegration of cementless metal implants. J Orthop Sci 2005; 10(6)622–6
- Fleisch H. Development of bisphosphonates. Breast Cancer Res 2002; 4(1)30–4
- Garcia-Moreno C, Serrano S, Nacher M, Farre M, Diez A, Marinoso M L, Carbonell J, Mellibovsky L, Nogues X, Ballester J, Aubia J. Effect of alendronate on cultured normal human osteoblasts. Bone 1998; 22: 233–9
- Hu J H, Ding Ming, Soballe K, Bechtold J E, Danielson C C, Day J S, Hvid I. Effects of short-term alendronate treatment on the three-fimensional microstructural, physical and mechanical properties of dog trabecular bone. Bone 2002; 31(5)591–7
- Jensen T B, Bechtold J E, Chen X, Soballe K. Systemic alendronate treatment improves fixation of press-fit implants. A canine study using non-loaded implants. J Orthop Res 2007; 22, [Epub ahead of print] PMID: 17318896
- Jeppsson C, Astrand J, Tagil M, Aspenberg P. A combination of bisphosphonate and BMP additives in impacted bone allografts. Acta Orthop Scand 2003; 74(4)483–9
- Kold S, Bechtold J E, Mouzin O, Elmengaard B, Chen X, Soballe K. Fixation of revision implants is improved by a surgical technique to crack the sclerotic bone rim. Clin Orthop 2005, 432160-6
- Michael W R, King W R, Wakim J M. Metabolism of disodium ethane-1-hydroxy-1, 1-diphosphinate (disodium etidronate) in the rat, rabbit, dog and monkey. Toxicol Appl Pharmacol 1972; 21: 503–15
- Millett P J, Allen M J, Bostrom M P. Effects of alendronate on particle-induced osteolysis in a rat model. J Bone Joint Surg (Am) 2002; 84: 236–49
- Mochida Y, Bauer T W, Akisue T, Brown P R. Alendronate does not inhibit early bone apposition to hydroxyapatitecoated total joint implants A preliminary study. J Bone Joint Surg (Am) 2002; 84: 226–35
- Mouzin O, Bechtold J E, Søballe K. Loading improves anchorage of hydroxyapatite implant more than titanium implants. J Biomed Mat Res 2001; 58(1)61–8
- Rodan G A. Bone mass homeostasis and bisphosphonate action. Bone 1997; 20(1)1–4
- Shanbhag A S, Hasselman C T, Rubash H E. Inhibition of wear debris mediated osteolysis in a canine total hip arthroplasty model. Clin Orthop 1997; 344: 33–43
- Soballe K, Hansen E S, B-Rasmussen H, Bunger C. Tissueingrowth into titanium and hydroxyapatite coated implants during stable and unstable mechanical conditions. J Orthop Res 1992; 10: 285–99
- Soballe K, B-Rasmussen H, Hansen E S, Bunger C. Hydroxyapatite coating converts fibrous tissue to bone around loaded implants. J Bone Joint Surg (Br) 1993; 75(2)270–8
- Soballe K, Mouzin O, Kidder L, Overgaard S, Bechtold J E. The effects of hydroxyapatite coating and bone allograft on fixation of loaded experimental primary and revision implants. Acta Orthop Scand 2003; 74(3)239–47
- Soballe K, Jensen T B, Mouzin O, Kidder L, Bechtold J E. Differential effect of a bone morphogenetic protein-7 (OP-1) on primary and revision loaded, stable implants with allograft. J Biomed Mater Res A. 2004; 15(71)4, 569–76
- Tucci J R, Tonino R P, Emkey R D, Peverly C A, Kher U, Santora A C. Effect of three years of oral alendronate treatment in postmenopausal women with osteoporosis. Am J Med 1996; 101(5)488–501
- Vestermark Toft M, Bechtold J E, Swider P, Soballe K. Mechanical interface conditions affect morphology and cellular activity of sclerotic bone rims forming around experimental loaded implants. J Orthop Res 2004; 22(3)647–52
- Wise L M, Waldman S D, Kasra M, Cheung R, Binnington A, Kandel R A, White L M, Grynpas M D. Effect of zoledronate on bone quality in the treatment of aseptic loosening of hip arthroplasty in the dog. Calcif Tissue Int 2005; 77(6)367–75