Abstract
Background and purpose Problems during knee extension, due to kinematic alterations, are not uncommon after total knee arthroplasty. Hinged prostheses provide higher stability than non-hinged designs and may minimize these alterations. Thus, in this in vitro study we investigated the quadriceps force required to extend the knee during an isokinetic extension cycle generating a constant extension moment after non-hinged and hinged total knee arthroplasty.
Methods Human knee specimens were tested in a kinematic knee simulator under physiological conditions, after implantation of two types of non-hinged cruciate retaining prosthesis (Gemini; Link, Germany and Interax I.S.A.; Stryker, Ireland) and a hinged prosthesis (Rotations-Knie; Link, Germany). During simulation of an extension cycle from 120° knee flexion to full extension, the change in quadriceps force to produce the constant extension moment of 31 Nm was dynamically measured using a load cell attached to the quadriceps tendon.
Results After implantation of the non-hinged pros-theses, there was no alteration in maximum quadriceps force in knee flexion compared to physiological conditions, but alteration occurred at lower flexion angle (p=0.002) and increased up to 1,257 (SD 273) N (p=0.04) in knee extension. Following implantation of the hinged prosthesis, there was no alteration in quadriceps extension force in flexion but it decreased to 690 (SD 81) N (p=0.003) in extension.
Interpretation Hinged knee prostheses restore the quadriceps lever arm in knee flexion and improve the lever arm in knee extension due to higher constraint and knee joint stability. This would offer a potential advantage for patients with weak quadriceps strength by making it easier to stabilize the knee in full extension during walking.
After a total knee arthroplasty (TKA), patients show abnormal knee function and have significantly more problems in stair climbing than control subjects (Andriacchi et al. Citation1982, Dorr Citation1988, Wimmer Citation1999). The inability to extend the knee as strongly as needed for walking, lifting, and rising from chairs and climbing stairs has been reported as one of the most commonly occurring mechanical reasons for revision of a TKA (Rand Citation1994). While the patients were able to improve upon their pre-operative extension torques by up to 50%, they did not reach the level of healthy subjects of the same age (Berman et al. Citation1991, Fuchs et al. Citation1998, Citation2004). Several authors have described a paradoxical movement of the tibiofemoral contact point from posterior to anterior during knee flexion after TKA (Lewandowski et al. Citation1997, Dennis et al. Citation1998). This would reduce the lever arm of the extensor mechanism and result in higher quadriceps muscle force required to extend the knee (Ostermeier et al. Citation2004). It has been recommended that hinged pros-theses be used in cases of severe knee instability (Barrack Citation2001, Citation2002). With this increased stability and minimized paradoxical movement due to the hinge mechanism, a mechanical improvement of the quadriceps lever arm could be postulated. We therefore compared the quadriceps forces required to extend the physiological knee at a constant extension moment with those required after implantation of non-hinged or hinged TKAs.
Methods
The experimental set-up and the test cycle used in this study were the same as previously reported by Stukenborg-Colsman et al. (Citation2002) and Ostermeier et al. (Citation2004). 12 knee specimens of approximately the same size (median age 63 (52–67) years, all male) were transected 30 cm proximally and dis-tally to the knee joint line. The skin, subcutaneous tissue, muscles, articular capsule, ligaments, and tendons were preserved. The specimens were mounted into a specially designed knee simulator in which isokinetic flexion-extension moments are simulated ( and ). The resulting arrangement gives complete freedom of movement of the joint—with the exception of flexion-extension, which is determined by the position of the swing-arm. Movement of the tibia was achieved by coordinated activation of three hydraulic cylinders, which were attached to the tendons of the specimens by special clamps: one to simulate quadriceps muscle force, one to simulate a co-contraction of the hamstrings muscles, and the third to apply an external flexion moment. The test cycle simulated an isokinetic extension cycle from 120° knee flexion to full extension. Thus, sufficient force to the quadriceps tendon was applied by the the quadriceps cylinder in a closed-loop control cycle to generate a constant extension moment of 31 Nm about the knee. The hamstrings cylinder simulated the co-contraction of the hamstrings muscles with a constant co-contractive flexion force of the hamstrings of 100 N.
Figure 1. Schematic view of the test set-up according to Stukenborg-Colsmanetal. (Citation2002). 1. Control unit 2. Interface 3. Valve 4. Pressure-valve 5. Tibia frame 6. Femur frame 7. Cylinder (tibia) 8. Cylinder frame 9. Cylinder (quadriceps) 10. Cylinder (hamstrings) 12. Force transducer (quadriceps) 13. Force transducer (tibia) 14. Angle measurement (tibia)
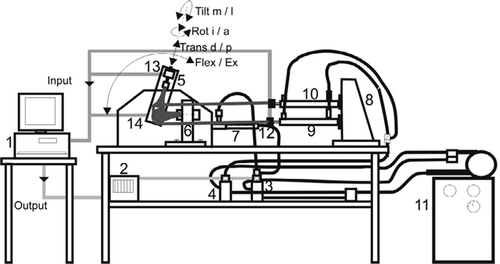
Quadriceps force was measured with a frequency of 10 Hz and an accuracy of ± 0.1 N using a load cell (Hottinger Baldwin Messtechnik GmbH, Darmstadt, Germany) attached between the tendon clamp and the quadriceps cylinder. Degree of knee flexion was measured using a custom-made voltage goniometer attached to the tibial swing arm with a frequency of 10 Hz and an accuracy of ± 0.05°.
The quadriceps forces of all specimens were first measured in the normal physiological joint, after which a non-hinged, posterior cruciate retaining TKA with no patellar resurfacing was implanted without bone cement according to the manufacturer's guidelines and by the same team of surgeons. One half of the specimens received an implantation of the Gemini prosthesis system (GEM, size 5; Link, Germany) with a rotating inlay (9 mm), and the other half received implantation of the Interax I.S.A. prosthesis system (INT, size 500; Stryker/ Howmedica, Ireland) with a mobile bearing inlay (8 mm). Then, in each knee specimen a hinged knee prosthesis (size 5, Rotations-Knie; Link, Germany) was implanted. This type of system is a constrained knee prosthesis with a tibiofemoral rotating hinge mechanism, which limits all directions of translational tibiofemoral movements but allows the joint to flex and rotate along the long tibial axis. The femoral component has an anatomical patella flange, which offers the possibility of leaving the patella unresurfaced. According to the manufacturer's guidelines, all remaining ligaments and capsular tissue structures around the knee joint—including the posterior cruciate ligament and the collateral ligaments—were resected. Although the prosthesis was originally planned to be implanted with bone cement, in this particular test set-up, the primary stability of the prosthesis was sufficient enough to be implanted without cement.
The physiological knee and each type of knee prosthesis in the same specimen were sampled 3 times. Differences in the quadriceps force between the mean values of the experimental groups were evaluated using ANOVA at a significance level of p ≤0.05.
Results
The quadriceps force curve reached a maximum value of 1,343 N at 107° of flexion in the physiological knee (Table). In the range between 60° and 10° of knee flexion, a quadriceps force of less than 900 N was required to extend the knee (). Implantation of the non-hinged and the hinged prostheses resulted in higher, but not statistically significant, maximum quadriceps forces in flexion. The values were 1,412 N (GEM, p=0.2), 1,466 N (INT, p=0.4), and 1,427 N (RO, p=0.2). The maximum quadriceps force following the non-hinged prosthesis occurred at lower angles of knee flexion (94° and 96°, p=0.002 and p=0.004) compared to physiological knee conditions, while maximum quadriceps force after implantation of the hinged prosthesis was similar to that under physiological knee conditions (106°, p=0.4). During further knee extension between 60° and 30°, the quadriceps force increased (p=0.02) compared to physiological conditions following all types of TKA (Table). In knee extension, the quadriceps force of the physiological knee increased up to 1,257 N (p=0.05) after implantation of the non-hinged prostheses whereas the quadriceps force decreased to 689 N (p=0.2) after implantation of the hinged prosthesis. Comparing non-hinged and hinged prostheses, the quadriceps force decreased (p=0.002 and p=0.05) in knee extension with the hinged prosthesis.
Figure 3. Quadriceps forces required to generate an extension moment of 31 Nm under physiological knee conditions, after implantation of non-hinged knee prosthesis (Gemini (GEM; Link, Germany) or Interax I.S.A. (INT;Stryker/Howmedica, Ireland)), or a hinged knee prosthesis (RO, Rotations-Knie; Link, Germany) from 120° knee flexion to full extension. Mean values of 3 repetitions. (Bars represent SD).
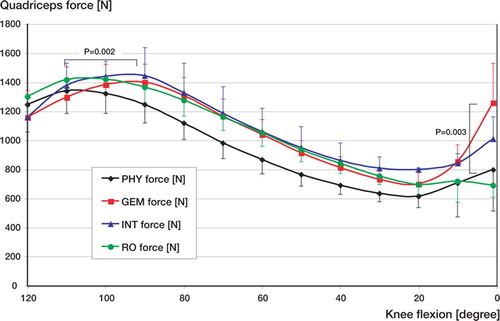
Quadriceps force to generate an extension moment of 31 Nm under physiologic knee conditions, after implantation of non-hinged knee prosthesis (GEM and INT) and a hinged knee prosthesis (RO): Mean values of 3 repetitions at specific knee flexion angle, with standard deviation (SD), percentage of forces of physiologic knee conditions, significance compared to physiologic knee conditions (p1) and between the types of knee prostheses (p2 and p3)
Discussion
We measured the dynamic changes in the quadriceps muscle force required to extend the knee with a constant extension moment of 31 Nm. Generally, the lever arm changes during extension of the knee because of the translating tibiofemoral and patel-lofemoral contact points, which results in a changing quadriceps force during extension (Nisell and Ekholm Citation1985, Ostermeier et al. Citation2004).
This in vitro test only simulated one constant moment during the whole extension cycle, which contrasts with the varying peak extension moments over an isokinetic extension cycle in vivo (Berman et al. Citation1991, Fuchs et al. Citation1998, Citation2004). Thus, the quantitative results of our study should not be translated directly to in vivo conditions. Nevertheless, the qualitative changes that we found illustrate the mechanical effect after implantation of the various knee prosthesis systems both in vitro and in vivo.
The lowest quadriceps forces under physiological knee conditions were observed between 60° and 10° of knee flexion, which is similar to the findings of Andriacchi (Citation1988) and Nisell and Eckholm (Citation1985). Thus, the force of the quadriceps muscle is at its minimum most of the time during daily activity (Andriacchi Citation1988). All prosthetic systems resulted in statistically significantly higher quadriceps forces between 30° and 60° of knee flexion, which may be associated with a potential loss of the physiological lever arm due to insufficient restoration of the patellofemoral joint after TKA (Petersilge et al. Citation1994). In addition, the increase in quadriceps load depended on the type of prosthesis. After implantation of the cruciate retaining non-hinged prosthesis type, a significantly higher quadriceps force was necessary to generate the same amount of extension moment compared to the conditions after implantation of the hinged prosthesis. In addition, maximum quadriceps load following implantation of the non-hinged prosthesis occurred at a significantly lower knee flexion angle. As the hinged prosthetic system in particular limits translation, and the axis of knee flexion is fixed due to the hinge mechanism, the lever arm of the quadriceps muscle is improved and increased continuously during knee extension. Following implantation of the non-hinged prosthesis, paradoxical movement of the tibiofemoral contact point may occur and reduce the quadriceps lever arm (Ostermeier et al. Citation2004). Furthermore, following the guidelines of the manufacturer, all ligamentous structures were resected during implantation of the hinged prosthesis, which could have an additional effect on reducing the extending quadriceps force required. Similar to a soft-tissue release, resection of the collateral ligament could minimize flexion moments on the knee joint in cases of extension lag. Transferring these in vitro findings to conditions in vivo, non-hinged prostheses demand adequate quadriceps muscle strength, as it reduces the quadriceps lever arm and therefore increases quadriceps force during knee extension at the same level of extension moment. In contrast, hinged prostheses require significantly lower quadriceps forces at a significantly higher range of knee motion. Thus, our findings suggest that patients who require sufficient joint stability and have low quadriceps force would benefit from having a hinged TKA.
We thank ENDO-Stiftung e.V. and Waldemar Link GmbH & Co. KG, Hamburg, Germany, for their financial support of this study. The sponsors did not participate in the design of the study, in the evaluation of the results, or in the writing of the article.
Contributions of authors
SO performed the test set-up, observed the test cycles, and did the biomechanical and statistical analysis. He also wrote the draft manuscript and revisions. CF initiated the study and performed implantation of the prosthetic systems. SF prepared the knee specimens and performed the test cycles. CH helped with the biomechanical and statistical analyses and revised the manuscript. CSC designed the test set-up and helped with the clinical background.
- Andriacchi T P. Biomechanics and gait analysis in total knee arthroplasty. Orthop Rev 1988; 17: 470–3
- Andriacchi T P, Galante J O, Fermier R W. The influence of total knee-replacement design on walking and stair-climbing. J Bone Joint Surg (Am) 1982; 64: 1328–35
- Barrack R L. Evolution of the rotating hinge for complex total knee arthroplasty. Clin Orthop 2001, 392: 292–9
- Barrack R L. Rise of the rotating hinge in revision total knee arthroplasty. Orthopedics 2002; 25: 1020–58
- Berman A T, Bosacco S J, Israelite C. Evaluation of total knee arthroplasty using isokinetic testing. Clin Orthop 1991, 271: 106–13
- Dennis D A, Komistek R D, Colwell C E, Ranawat C, Scott R D, Thornhill T S, Lapp T S. In vivo anteroposterior femorotibial translation of total knee arthroplasty: a mul-ticenter analysis. Clin Orthop 1998, 356: 47–57
- Dorr L D. Functional comparison of posterior of posterior cruciate retaining versus cruciate sacrificed total knee arthroplasty. Clin Orthop 1988, 236: 36–41
- Fuchs S, Tibesku C O, Jerosch J. Isokinetic measurements in total knee arthroplasty patients compared to healthy volunteers. Nice, 8th Congress of the European Society of Sports Traumatology, Knee Surgery and Arthrocospy
- Fuchs S, Tibesku C O, Genkinger M, Volmer M, Laass H, Rosenbaum D. Clinical and functional comparison of bicondylar sledge prostheses retaining all ligaments and constrained total knee replacement. Clin Biomech (Bristol, Avon) 2004; 19: 263–9
- Lewandowski P J, Askew M J, Lin D F, Hurst F W, Melby A. Kinematics of posterior cruciate ligament-retaining and -sacrificing mobile bearing total knee Aarthroplasties. J Arthroplasty 1997; 12: 777–84
- Nisell R, Ekholm J. Mechanics of the knee. Acta Orthop Scand (Suppl 216) 1985; 1–42
- Ostermeier S, Hurschler C, Stukenborg-Colsman C. Quadriceps function after TKA-an in vitro study in a knee kinematic simulator. Clin Biomech (Bristol, Avon) 2004; 19: 270–6
- Petersilge W J, Oishi C S, Kaufman K R, Irby S E, Colwell C W, Jr. The effect of trochlear design on patellofemoral shear and compressive forces in total knee arthroplasty. Clin Orthop 1994; 124–30
- Rand J A. Current concepts review: The patellofemoral joint in total knee arhtroplasty. J Bone Joint Surg (Am) 1994; 76: 612–20
- Stukenborg-Colsman C, Ostermeier S, Hurschler C, Wirth C J. Tibiofemoral contact stress after total knee arthroplasty: comparison of fixed and mobile-bearing inlay designs. Acta Orthop Scand 2002; 73: 638–46
- Wimmer M A. Wear of the polyethylene component created by rolling motion of the artificial knee joint. Aachen, Shaker 1999