Abstract
Background Operative and nonoperative treatment of anterior cruciate ligament (ACL) injuries is often associated with a lack of proprioception and persistent muscle weakness of unknown origin.
Methods This long-term study in the cat experimentally compares both neural and muscular activity in the articular nerves of the knee (PAN and MAN), quadriceps and hamstrings, in the chronic unstable knee, and in the reconstructed knee. We also investigated changes in neuromuscular response due to the mechanical competence of the graft, comparing stable and unstable reconstructed knees.
Results We found increased periarticular muscle activity during anterior tibial translation in chronically unstable knees. Both reconstructed and non-reconstructed knees lost fast reactive activity in the articular nerves. When stability was recovered after reconstruction, the knees showed a more adjusted—although incomplete—muscular reaction.
Interpretation ACL-injured knees in the cat, with or without reconstruction, show definite abnormalities in neuromuscular reaction in the long term. Regaining stability with a competent graft in the reconstructed knee is crucial for reduction of this anomalous reaction.
Clinically, there is insufficient evidence to determine whether operative or nonoperative treatment is best for ACL injury (Linko et al. Citation2005). Although patients who do not undergo surgery may occasionally have surprisingly good function, the current recommendation for most patients with average functional requirements is surgical ACL reconstruction. Return to sports, functional outcome, and recovery time have not been found to be better after surgical reconstruction in the few trials that have compared operative and nonoperative treatment (Linko et al. Citation2005), but less knee instability was detected in patients who were treated surgically. However, better stability is not the same as a normal knee (Tsuda et al. Citation2003). Both a lack of proprioception (Bonfim et al. Citation2003) and persistent muscle weakness (Reider et al. Citation2003) have been found after operative or nonoperative treatment of ACL injuries. These considerations emphasize the difference between the normal knee and an ACL-injured knee that has undergone treatment.
Muscular impairment after ACL injury is universally accepted, but efforts to help patients regain muscular control of their knees have not been sufficiently effective (Trees et al. Citation2005). ACL-deficient knees have shown altered quadriceps control (Williams et al. Citation2004), but also persistently altered hamstrings activity after reconstruction (Tibone and Antich Citation1993)—possibly to compensate for anterior laxity. Reconstructed knees have been found to show altered coordination strategies in the lower limb, measured as relative phase dynamics (Kurz et al. Citation2005). Also, specific imbalances in the hamstrings/quadriceps ratio have been demonstrated in ACL-reconstructed knees (Hiemstra et al. Citation2004).
The origin of persistent muscular impairment after ACL injury and reconstruction is unclear. Changes in neural function and muscle physiology have been suggested (Williams et al. Citation2004), and according to clinical studies, a proprioceptive deficit remains after ACL reconstruction (Bonfim et al. Citation2003). The fact that there may be persistent neurophysiological abnormalities after ACL reconstruction even suggests that the loss of ACL mech-anoreceptors may initiate central nervous system modifications that are not compensated for by other nervous structures (Valeriani et al. Citation1999).
Animal models have shown changes in neural and muscular activity around the knee after acute total ACL section (Gomez-Barrena et al. Citation1997) and reconstruction (Gomez-Barrena et al. Citation1999), and they may be useful to determine whether or not this neural and muscular activity is modified in the long term after ACL reconstruction. The hypothesis in this study was that neural and muscular activity in the ACL reconstructed knee is similar to that in the ACL-deficient knee—and not to that in the normal, uninjured knee. To test this hypothesis, we investigated neural and muscular activity in the chronic unstable knee experimentally and compared it with reconstructed knees after acute ACL injury in the long term. We also formulated a second hypothesis—that the neural and muscular status of the reconstructed knee depends on the mechanical competence of the graft and not on the supposed proprioceptive recovery through auto-graft re-innervation. To test this second hypothesis, we performed a long-term study of the neural and muscular outcome of ACL reconstructed knees that were either stable or unstable after surgery.
Material and methods
Experiments were conducted in 24 cats (weight 2.5–5 kg) that had been obtained from specialized dealers (Centro de Animales de Experimentación, Universidad de Córdoba; and Isosquimen Ltd., Barcelona). Experiments included two surgeries and one set of neural and muscular electric activity registrations. All were carried out in accordance with the European Community Council directive (86/609/EEC), and efforts were made to minimize animal suffering and the number of animals used in the experiments. Before surgery, the animals were examined by two investigators to ensure normal bilateral knee stability. A control group (group 0) of 16 knees was established from normal knees that were registered before any surgery was performed. To avoid potential influence of contralateral knee instability, only one knee per animal underwent surgery and the side chosen for the experimental knee was alternated; thus, 12 right knees and 12 left knees were operated.
In the first surgery, the animals were anesthetized with intraperitoneal pentobarbital (33 mg/ dL, 100 mg/kg). The ACL was sectioned in every animal through an anterolateral miniarthrotomy. In 10 cases, this was followed by closure of the arthrotomy, leaving the knee unstable (group I). In 8 cases (group II) this was followed by enlargement of the skin incision, harvesting of the extensor digitorum longus (EDL) tendon from its lateral femoral condyle insertion without sectioning from the musculotendinous junction, and ACL reconstruction through tibial and femoral tunnels—fixed with bone plugs and ligament sutures to femoral and tibial AO minifragment screws when needed. From these 8 reconstructed knees, 5 animals were left unstable at surgery (subgroup IIa) and 3 were stabilized (IIb). In 6 other animals (group III) ACL section was followed by skin incision enlargement, harvesting of a bone-tendon-bone (BTB) graft from the middle third of the patellar ligament, and ACL reconstruction through a transpatellar ligament approach—fixing the graft with AO mini-fragment screws as interferential screws in tibial and femoral tunnels, and secured by bone sutures to femoral and tibial screws when needed. From the 6 knees reconstructed with BTB autografts, 3 were left unstable (subgroup IIIa) and 3 were stabilized (IIIb). This translated into 10 unstable, unreconstructed (UNR) knees, group I; 8 unstable, reconstructed (UR) knees, groups IIa and IIIa; and 6 stable reconstructed (SR) knees, groups IIb and IIIb (). Unstable reconstructions were obtained when graft fixation was accomplished without previous graft tensioning. Stable reconstructions included graft tensioning prior to graft fixation.
Figure 1. Both techniques of ACL reconstruction, using the pediculated tendon of the extensor digitorum longus (a), and using the free bone-tendon-bone autograft from the central third of the patellar ligament (b).
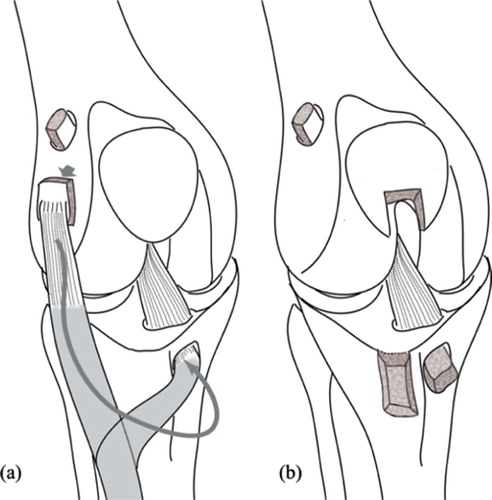
The animals were allowed free motion in a 15 m2 room over median 12 (9–18) months. After this, the animals were again anesthetized with intraperitoneal pentobarbital and underwent bilateral knee surgery to isolate medial (MAN) and posterior (PAN) articular nerves through medial and posterior approaches, as previously described (Gomez-Barrena et al. Citation1996, Citation1997, Citation1999). Stainless hook electrodes were implanted surrounding these nerves with the help of a surgical microscope. Stainless electrodes were also located in rectus anterior to record the electromyogram (EMG) in quadriceps, and in biceps femoris to record hamstring EMG. Electrical activity in articular nerves and periarticu-lar muscle EMG at the index and contralateral knees was recorded during passive flexion, extension, and anterior tibial displacement (at 90°, similar to anterior drawer, and 30° flexion, similar to Lachman maneuver), as in previous studies, from the start to the end of the movement. Extra doses of anesthetic were given when needed, to maintain a deep level of anesthesia. Nerve potentials and EMG were filtered (300–3,000 Hz), amplified, and analyzed with Spike 2 software (CED, Cambridge, UK), calculating summed peristimulus time histograms (PSTHs). PSTHs were calculated by summing the number of spike repetitions (nerve or muscle activity shown in the recordings of repeated experimental series of movements) present at a certain interval of time. The mean firing activity per movement was calculated from the start of the movement to the PSTH peak (firing activity per second). The slope of the PSTH chart (electrical activity counts against time) was used to assess the correlation of nerve and muscle activity to knee motion ().
Figure 2. Neurophysiological data for analysis (summed PSTH/bin). Slope of the mean activity (electrical activity counts against time) is obtained on PSTH/bin charts.
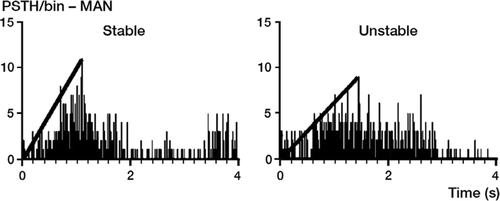
The Mann-Whitney test was used to compare mean firing activity and slope values among groups by pairs. Significance was considered when p <0.0125, after Bonferroni adjustment for multiple comparison. To evaluate the separate effects of graft type and graft competence (knee stability after the reconstruction), a two-way ANOVA was used for mean values of activity and slope, with a significance level of 0.05 and with one degree of freedom, as the interaction term was not statistically significant.
Results
The overall comparison of neuromuscular activity showed that in the long term, reactive activity during flexion and extension showed similar values, independently of whether the ACL was present, absent, or substituted by a stable or unstable graft (). However, anterior tibial translation (ATT) gave less reactivity in MAN, and most importantly, there were marked increases in muscle activity after ACL injury whether or not the knees were reconstructed. This occurred both at the quadriceps and hamstring, during anterior drawer maneuvers (ATT at 90° flexion), but more clearly during Lachman-like maneuvers (ATT at 30° flexion). Differences found in quadriceps activity between UR (unstable reconstructed) and UNR (unstable non-reconstructed) knees implied that increases in quadriceps activity were related to the non-reconstructed status, while increases in hamstring activity were related to the persistent instability.
Figure 3. Charts showing mean ± SD firing activity (PSTH, units/bin) for MAN, PAN, quadriceps and hamstrings, during anterior tibial translation at 90° and 30° knee flexion, flexion, and extension. Comparisons by Mann-Whitney test after Bonferroni adjustment are included (*p <0.0125).
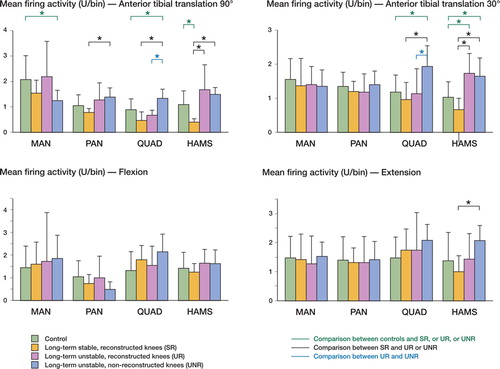
Overall analysis of the slope showed that there was a decrease in this variable with persistent instability (). As the slope relates to amplitude of the response over time, its decrease indicates a poor relationship of neuromuscular activity and knee motion with instability. However, during either knee flexion or anterior drawer, the most clear decrease in slope was found for chronic UNR knees, while during knee extension or the Lachman-like maneuver, the decrease in slope affected unstable knees with and without reconstruction to similar extents.
Figure 4. Charts showing mean ± SD slope (adimensional variable) for MAN, PAN, quadriceps and hamstrings, during anterior tibial translation at 909 and 309 knee flexion, flexion, and extension. Comparisons by Mann-Whitney test after Bonferroni adjustment are included (*p <0.0125).
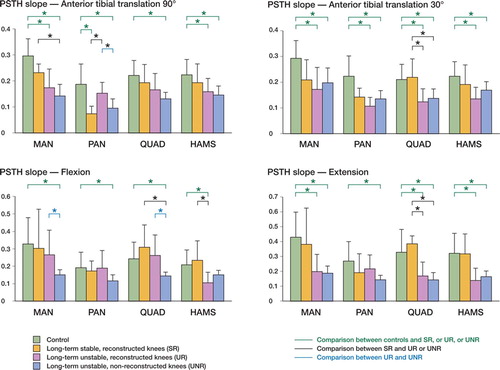
When we systematically compared the control knees (group 0, n=16) with the long-term injured knees without reconstruction (group I, UNR, n=10), we observed in the latter a significantly increased muscular activity in quadriceps during flexion and ATT both at 90° and 30° knee flexion, and in hamstrings during ATT at 30° knee flexion (). The reduced PSTH slope was almost universal in both muscles in the chronic UNR knee (). Neural activity at MAN was also reduced during anterior drawer, while neural activity in MAN and PAN was similar to controls whether in flexion-extension or in Lachman-like maneuvers in the long-term injury—with or without reconstruction. However, the slope was significantly reduced in all manoeuvres for both MAN and PAN (p <0.01).
When reconstructed knees (groups II and III, SR + UR, n=14) were compared to non-reconstructed knees (n=10), a major decrease in quadriceps activity was observed after reconstruction during ATT at 30° and 90° knee flexion (p <0.01). The slope showed a corresponding increase with reconstruction, indicating a better relationship of quadriceps activity with motion during flexion (p<0.01).
Stable reconstructed knees (groups IIa and IIIa, SR, n=6) showed activity values closer to control knees, except for a decrease in hamstrings activity during the drawer-like maneuver. The muscular adaptation of the signal to motion, as measured by the PSTH slope, was similar to that of controls in these stable knees, except in the neural signaling through the posterior articular nerve, where it was reduced ( and ).
Unstable reconstructed knees (groups IIb and IIIb, UR, n=8) showed increased muscular activity during ATT, particularly the hamstrings activity during the Lachman-like maneuver, as compared to controls. This was also found during the anterior drawer (both hamstrings and quadriceps) and extension (hamstrings), when comparing UR knees to SR knees. The adaptation to motion was also significantly different to that of controls in both quadriceps and hamstrings during ATT at 30° knee flexion, and during extension. When comparing muscle activity in UR and SR knees, hamstrings adaptation to motion was significantly reduced during flexion and extension, while quadriceps adaptation was poor during ATT at 30° of knee flexion and during extension, but was maintained during flexion and anterior drawer. Most striking were the similarities in activity and slope between UR and UNR knees during ATT at 30° and 90°, and during extension ( and ).
To determine whether differences in reconstructed knees were mostly due to the graft type or to the recovery of knee stability, two-way ANOVA was used and showed no significant differences between means of activity or slope, indicating a powerful effect of one variable. Comparing BTB and EDL tendon grafts, the fact that there were no significant differences between means indicated that the graft type was not statistically loading the relationship. Comparison of stable and unstable grafts () showed increased activity in hamstrings from UR knees during ATT at 30° (p=0.003) and at 90° (p=0.02), while there was increased slope in quadriceps from SR knees during ATT at 30° (p=0.01) and extension (p=0.001), but also in hamstrings from SR during flexion (p=0.03) and extension (p=0.02). As shown in , graft stability loaded the differences in activity (decrease towards normal) and slope (increase towards normal) with both graft types.
Figure 5. Mean firing activity and PSTH slope in hamstrings recordings at ATT 30° flexion and during extension, to observe the contribution of graft type and stability, after two-way ANOVA analysis.
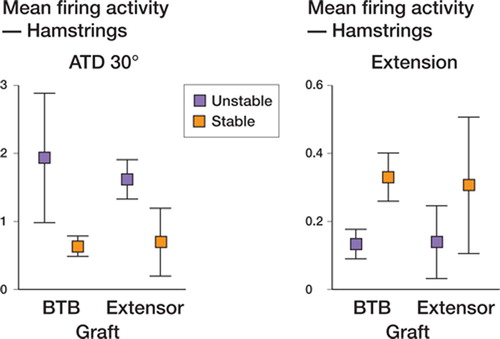
Effect of graft and/or stability on mean firing activity and PSTH slope in reconstructed knee. Two-way ANOVA for mean values of activity and slope in reconstructed knees. P-values are given when p <0.05
Discussion
Different techniques are available for reconstruction of the injured ACL (Sherman and Banffy Citation2004). Using the cat, we made use of two experimental techniques with autografts from different locations, to incorporate the variable of free vs. pediculated graft. We found no neuromuscular differences related to these types of graft, if graft competence was isolated as a variable. Real differences appeared when stability was not achieved. This result supports the idea that the main effect of ACL reconstruction is based on mechanical stability rather than on biological recovery of the graft, which would be enhanced in the pediculated graft in our model, compared to the free graft. This reasoning is in line with clinical findings of equivalent outcomes with BTB and 4-strand tendons with equivalent mechanical performance (Aglietti et al. Citation2004). Also, this result allowed us to jointly analyze the groups IIa and IIIa (SR knees) and IIb and IIIb (UR knees), as differences were not related to the type of graft but to the stability achieved.
When the normal knee was compared to the chronically unstable (UNR) knee, differences compared to our previous data on acute ACL section experiments (Gómez-Barrena et al. Citation1997) were seen in the long term. Unstable knees lose the relationship between motion and neural activity (which is reduced in the long term) and also the relationship between motion and muscular activity (still increased in the long-term to oppose anterior tibial translation). This could be interpreted as a long-term effect of the lack of proprioceptive information (registered in articular nerves) that no longer guides any muscular reaction. However, muscular reaction is maintained (with persistently increased activity)—albeit inefficiently, with no relation to motion (reduced slope). The decrease in the slope as a measure of the activity amplitude with time has been supported by other signal changes with time, such as in-ligament deformation measured by gauges (Bonsfills et al. Citation2007). As a relative magnitude, it avoids the potential errors of latency, where the starting point of the movement may be difficult to determine. These findings support pathophysi-ological interpretations suggested by other authors from clinical data, including lack of proprioception and inefficient muscular reaction (Snyder-Mackler et al. Citation1994, Williams et al. Citation2004).
Although stable (SR) knees permit a better muscular response than chronically unstable ones (UNR), there is insufficient proprioceptive output from the knee—both in stable and unstable knees, with and without reconstruction—to obtain a normal response to stimuli. The long-term adaptation is not successful, neither in stable, reconstructed knees nor in chronic unstable knees, after failed reconstruction or with no reconstruction. This is in accordance with clinical data from subjective and quantitative functional assessment (Fremerey et al. Citation2000, Bonfim et al. Citation2003) of long-term follow-up in ACL injured knees (Maletius andMessner Citation1999).
Regarding the role of mechanical stabilization, we found that neuromuscular reaction in stable knees was close to that of the controls, and this would support reconstruction to regain knee stability as being the best option to improve the injured knee towards normal. This is today's clinical standard, and this can also be supported from the neuromuscular point of view, and also by our data. These SR knees are, however, different from normal in the long term, as neural perception is reduced (in the PAN, the articular nerve collecting information fromtheACL—as shown in retrograde axonal transport from the ACL) (Gomez-Barrena et al. Citation1996). The fact that this hamstrings activity is raised in unstable reconstructed or non-reconstructed knees suggests that other sources of information (e.g. medial meniscus) initiate the response in unstable knees, but not after stable reconstruction, where this activity becomes reduced. Thus, mechanical stability is important but in our experimental setting neuromuscular deficit from the injured ACL remains, which is in accordance with the results of clinical studies (Fremerey et al. Citation2000).
We compared unstable, reconstructed knees to chronically unstable ones without reconstruction to clarify the role of conserved or newly grown neural endings in the graft. Some studies (Goertzen et al. Citation1992, Wada et al. Citation1997, Georgoulis et al. Citation2001, Adachi et al. Citation2002) have claimed that surgery helps recovery of the proprioceptive apparatus in the ACL-reconstructed knee, from morphological findings. In line with this possibility, we did not resect the injured ACL remnants and we included reconstructions with pediculated grafts. However, we did not find a definite difference in muscular or neural activity between unstable reconstructed and unstable non-reconstructed knees. Although the quadriceps activity is lower in the UR, the hamstrings hyperactivity (a clear effect of long-term compensation of instability in the ACL injured knee) is present in both. Differences in the slope that might suggest a regained proprioception in the UR compared to the UNR when the knee is tested in flexion and anterior drawer are not supported in extension or Lachman, and one would expect a similar recovery if this was related to a biological recovery of neural endings. Other reasons for this partial recovery may relate to learning neuromuscular processes that are as yet unknown. Then, neuromuscular data do not support the functional recovery of neural endings.
One must bear in mind that, apart from reconstruction and free motion, no other intervention was practiced in our experiments, and the role of specific nonoperative treatments such as rehabilitation programs and muscular stimulation were not evaluated in our experimental study. However, the conclusions on the biological and mechanical bases of neuromuscular reaction in the long term after ACL injury stand, given that the postoperative management was the same in all our series.
In conclusion, ACL injured knees, with or without reconstruction, display abnormalities in neu-romuscular reaction in the long term, including increased neural and muscular activity with instability reduced after stabilization. Non-reconstructed knees sustained quadriceps activity increases, but also hamstrings activity increases that were also present in unstable knees with persistent laxity after reconstruction. A poor neuromuscular adaptation to motion, as seen in the slope values, was generalized after ACL injury in the long term, either in reconstructed or non-reconstructed knees. Recovered stability, rather than the presence of a graft, facilitated a behavior that was somewhat closer to that of controls. In this sense, the recovery of knee stability with a competent graft showed a less anomalous neuromuscular reaction after ACL injury in the long term.
This work was supported by FIS (Fondo de Investigaciones Sanitarias; Ministry of Health, Spain) research grant FIS 01/0371.
Contributions of authors
EGB: design, experimental work and set-up, and writing of the manuscript. NB, JGM, and RBM: experimental work and set-up. AF: experimental work. ANM: neurophysiology and editing of manuscript.
- Adachi N, Ochi M, Uchio Y, Iwasa J, Ryoke K, Kuriwaka M. Mechanoreceptors in the anterior cruciate ligament contribute to the joint position sense. Acta Orthop Scand 2002; 73(3)330–4
- Aglietti P, Giron F, Buzzi R, Biddau F, Sasso F. Anterior cruciate ligament reconstruction: bone-patellar tendon-bone compared with double semitendinosus and gracilis tendon grafts. A prospective, randomized clinical trial. J Bone Joint Surg (Am) 2004; 86(10)2143–55
- Bonfim T R, Jansen Paccola C A, Barela J A. Propriocep-tive and behavior impairments in individuals with anterior cruciate ligament reconstructed knees. Arch Phys Med Rehabil 2003; 84(8)1217–23
- Bonsfills N, Raygoza J J, Boemo E, Garrido J, Nunez A, Gomez-Barrena E. Proprioception in the ACL-ruptured knee: The contribution of the medial collateral ligament and patellar ligament. An in vivo experimental study in the cat. Knee 2007; 14(1)39–45
- Fremerey R W, Lobenhoffer P, Zeichen J, Skutek M, Bosch U, Tscherne H. Proprioception after rehabilitation and reconstruction in knees with deficiency of the anterior cruciate ligament: a prospective, longitudinal study. J Bone Joint Surg (Br) 2000; 82(6)801–6
- Georgoulis A D, Pappa L, Moebius U, Malamou-Mitsi V, Pappa S, Papageorgiou C O, Agnantis N J, Soucacos P N. The presence of proprioceptive mechanoreceptors in the remnants of the ruptured ACL as a possible source of re-innervation of the ACL autograft. Knee Surg Sports Traumatol Arthrosc 2001; 9(6)364–8
- Goertzen M, Gruber J, Dellmann A, Clahsen H, Schulitz K P. Neurohistological findings after experimental anterior cruciate ligament allograft transplantation. Arch Orthop Trauma Surg 1992; 11(2)126–9
- Gomez-Barrena E, Martinez-Moreno E, Munuera L. Seg-mental sensory innervation of the anterior cruciate ligament and the patellar tendon of the cat's knee. Acta Orthop Scand 1996; 67(6)545–52
- Gómez-Barrena E, Núñez A, Martínez-Moreno E, Valls J, Munuera L. Neural and muscular electric activity in the cat's knee. Acta Orthop Scand 1997; 68(2)149–55
- Gomez-Barrena E, Nunez A, Ballesteros R, Martinez-Moreno E, Munuera L. Anterior cruciate ligament reconstruction affects proprioception in the cat's knee. Acta Orthop Scand 1999; 70(2)185–93
- Hiemstra L A, Webber S, MacDonald P B, Kriellaars D J. Hamstring and Quadriceps Strength Balance in Normal and Hamstring Anterior Cruciate Ligament-Reconstructed Subjects. Clin J Sport Med 2004; 14(5)274–80
- Kurz M J, Stergiou N, Buzzi U H, Georgoulis A D. The effect of anterior cruciate ligament reconstruction on lower extremity relative phase dynamics during walking and running. Knee Surg Sports Traumatol Arthrosc 2005; 13(2)107–15
- Linko E, Harilainen A, Malmivaara A, Seitsalo S. Surgical versus conservative interventions for anterior cruciate ligament ruptures in adults. Cochrane Database Syst Rev 2005; 18(2), CD001356
- Maletius W, Messner K. Eighteen- to twenty-four-year follow-up after complete rupture of the anterior cruciate ligament. Am J Sports Med 1999; 27(6)711–7
- Reider B, Arcand M A, Diehl L H, Mroczek K, Abulencia A, Stroud C C, Palm M, Gilbertson J, Staszak P. Proprioception of the knee before and after anterior cruciate ligament reconstruction. Arthroscopy 2003; 19(1)2–12
- Sherman O H, Banffy M B. Anterior cruciate ligament reconstruction: which graft is best?. Arthroscopy 2004; 20(9)974–80
- Snyder-Mackler L, De Luca P F, Williams P R, Eastlack M E, Bartolozzi A R. Reflex inhibition of the quadriceps femoris muscle after injury or reconstruction of the anterior cruciate ligament. J Bone Joint Surg (Am) 1994; 76(4)555–60
- Tibone J E, Antich T J. Electromyographic analysis of the anterior cruciate ligament-deficient knee. Clin Orthop 1993, 288: 35–9
- Trees A, Howe T, Dixon J, White L, Trees A. Exercise for treating isolated anterior cruciate ligament injuries in adults. Cochrane Database Syst Rev 2005; 19(4), CD005316
- Tsuda E, Ishibashi Y, Okamura Y, Toh S. Restoration of anterior cruciate ligament-hamstring reflex arc after anterior cruciate ligament reconstruction. Knee Surg Sports Traumatol Arthrosc 2003; 11(2)63–7
- Valeriani M, Restuccia D, Di Lazzaro V, Franceschi F, Fabbriciani C, Tonali P. Clinical and neurophysiologi-cal abnormalities before and after reconstruction of the anterior cruciate ligament of the knee. Acta Neurol Scand 1999; 99(5)303–7
- Wada Y, Takahashi T, Michinaka Y, Morisawa Y, Yamamoto H. Mechanoreceptors of patellar tendon used for ACL reconstruction. Rabbit experiments. Acta Orthop Scand 1997; 68(6)559–62
- Williams G N, Barrance P J, Snyder-Mackler L, Buchanan T S. Altered quadriceps control in people with anterior cruciate ligament deficiency. Med Sci Sports Exerc 2004; 36(7)1089–97