Abstract
Background Platelets contain many kinds of growth factors with the ability to accelerate angiogenesis. We analyzed whether a single injection of platelet-rich plasma (PRP) would accelerate surgical angiogenesis in necrotic bone implanted with vascular tissue.
Methods We used 24 Japanese White rabbits. PRP was refined from autologous blood by separation twice with centrifugation. A removed iliac bone was frozen in liquid nitrogen to ensure complete cellular necrosis. A narrow hole was made in the bone and the saphenous vascular bundle was passed through the hole. The bone was wrapped after injection of either 1 mL (1) PRP, or (2) saline solution into the hole, and was placed subcu-taneously in the thigh. In both groups, angiogenesis was compared 1 week and 2 weeks after surgery.
Result Angiogenesis was observed along the implanted vascular bundle in both groups. At 1 and 2 weeks after surgery, both the vessel density and the average length of newly formed vessels of the experimental group were significantly greater than in the control group. Both the vessel density and the length were greater after 2 weeks than after 1 week.
Interpretation A single injection of PRP accelerates surgical angiogenesis in vascular-implanted necrotic bone.
Avascular necrosis of bone commonly leads to bone collapse and arthritis. The optimal treatment would be rapid correction of the avascular condition before joint collapse occurs. Vascularized bone grafting or vascular bundle implantation is often performed to induce bone regeneration. Vascularized bone grafting has proved to be promising (Haw et al. Citation1978, Weiland et al. Citation1984, Sunagawa et al. Citation2000), but the rate of revascularization and subsequent bone remodeling is slow. Vascular bundle implantation induces angiogenesis in ischemic or necrotic areas of bone (Hori et al. Citation1979, Tamai et al. Citation1993). However, revascularization is delayed and unreliable. Recently, some reports have shown that administration of a growth factor, such as vascular endothelial growth factor (VEGF) or fibroblast growth factor-2 (FGF-2), can accelerate angiogenesis in necrotic bone when combined experimentally with vascular bundle implantation (Nakamae et al. Citation2004, Suzuki et al. Citation2004).
Platelet-rich plasma (PRP) is obtained from autologous blood, and thus requires no special considerations concerning antibody formation or infection risk; it also contains large amounts of growth factors.
We evaluated the possibility of accelerating angiogenesis in necrotic bone in a rabbit model using a combination of vascular bundle implantation and a single injection of PRP.
Animals and methods
Animals and experimental design ()
We used adult male Japanese White rabbits weighing from 3.0 to 3.5 kg. All animals were housed individually and fed a commercial diet. They were treated in accordance with the stipulations of the Experimental Ethics Committee for Experimental Animals at Hiroshima University and the protocol was approved by the institutional Committee for Animal Care and Use.
Figure 1. The experimental procedure. Blood was drawn off from each rabbit and anticoagulant was added. PRP was separated by a two-step centrifugation procedure. The necrotic bone was wrapped with a silicon sheet after injection of 1 ml of PRP into the hole, and it was then placed subcutaneously in the thigh.
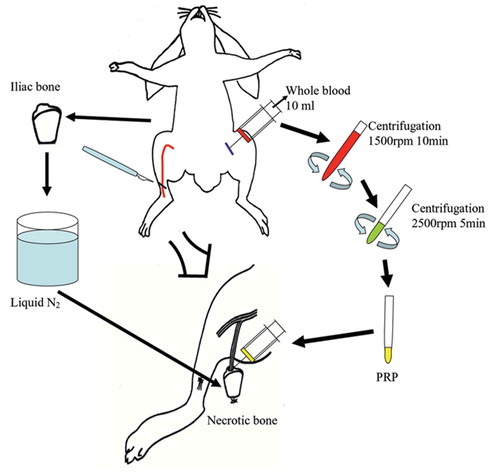
The rabbits were divided randomly into experimental and control groups with 12 animals in each group. Necrotic bone with a vascular bundle inserted was implanted subcutaneously, without (control group) or with (experimental group) the addition of PRP. Formation of new vasculature was assessed 1 and 2 weeks after surgery.
PRP preparation
10 mL of autologous whole blood was drawn from each rabbit and supplemented with 1 mL 3.8% citrate phosphate dextrose (CPD) to prevent coagulation. The blood was centrifuged at 1,500 rpm (420 x g) for 10 min to separate the plasma containing platelets from red cells, and then centrifuged for an additional 5 min at 2,500 rpm (1,160 x g) to separate 1mL PRP from the platelet-poor plasma (PPP).
Surgical procedure
Animals were anesthetized using a combination of intravenous sodium pentobarbital at 20 mg/kg body weight and intramuscular ketamine at 50 mg/kg body weight. The right iliac crest was then exposed and a 2 × 2-cm segment was removed to create a nonvascularized bone graft. To ensure complete cellular necrosis, the bone was frozen for 5 min in liquid nitrogen (Malawer et al. Citation1988, Sunagawa et al. Citation2000). Meanwhile, the saphenous artery and its venae comitantes were exposed in the medial aspect of the right thigh and mobilized as a vascular bundle. A hole larger than the diameter of the saphenous bundle was then made in the bone graft and the bundle was passed through this hole. The bone was wrapped with a 0.3-mm-thick silicon sheet to prevent direct revascularization from the adjacent soft tissue and 1 mL PRP (experimental group) or 1 mL saline solution (control group) was then injected into the hole. The whole graft was then implanted subcutaneously in the thigh.
Microangiography
In both groups, quantitative evaluation of neovas-cularization was carried out 1 and 2 weeks after surgery. Latex contrast medium was infused by manual pressure through the femoral artery to analyze neovascularization, and the bone was decalcified by a modification of the Spalteholz bone-clearing technique.
Measurement of vessel density and length
An angiographic score was calculated as the ratio of circles crossed by opacified arteries divided by the total number of circles in each specimen, which reflects the vascular density of the specimen. The length of the newly formed vessels was measured at 10 points perpendicular to the implanted vascular bundle, at 2-mm intervals.
Statistics
The Mann-Whitney U test was used for statistical evaluation. P-values less than 0.05 were considered to be significant.
Results
We observed neovascularization along the implanted vascular bundle in both the experimental and the control groups. At 1 week after surgery, invasion of newly formed vessels into the necrotic bone was clearly recognizable in both groups (). 2 weeks after surgery, neovascularization had increased in both groups ().
Figure 2. Microangiography 1 week after surgery; newly formed vessels could be seen along the implanted vascular bundle in the control group (left panel) and in the experimental group (right panel).
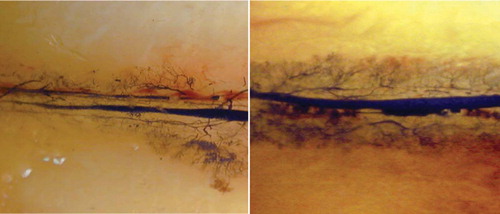
Figure 3. Microangiography 2 weeks after surgery; newly formed vessels could be seen along the implanted vascular bundle in the control group (left panel) and in the experimental group (right panel).
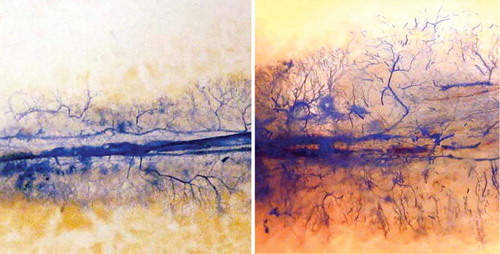
1 week after surgery, the mean angiographic score of the experimental group was higher than that of the control group (). This difference was maintained at 2 weeks.
The mean length of newly formed vessels at 1 and 2 weeks after surgery was significantly higher in the experimental group than in the control group ().
Figure 4. Mean angiographic score at 1 and 2 weeks after surgery. 1 week after surgery, that of the experimental group was 0.31 (SE 0.039), higher than that of the control group (0.18 (SE 0.039)) (p = 0.05). This difference was maintained at 2 weeks, when the mean angiographic score of the experimental group was 0.54 (SE 0.033) and that of the control group was 0.34 (SE 0.063) (p = 0.02).
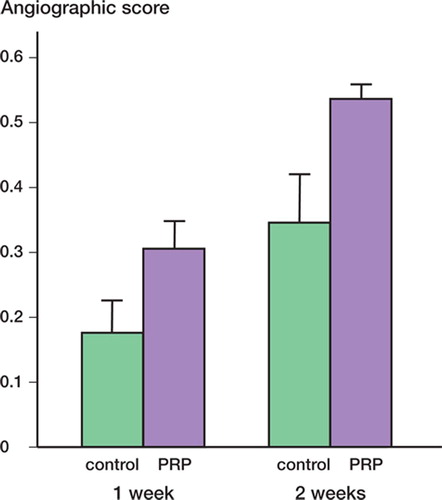
Figure 5. Mean length of newly formed vessels at 1 and 2 weeks after surgery. 1 week after surgery, the length of newly formed vessels in the experimental group (2.07 mm (SE 0.65)) was significantly greater than in the control group (0.98 (SE 0.32)) (p = 0.03). At 2 weeks, the mean vessel length in the experimental group was 3.3 (SE 0.16) and it was 2.3 (SE 0.3) in the control group (p = 0.01).
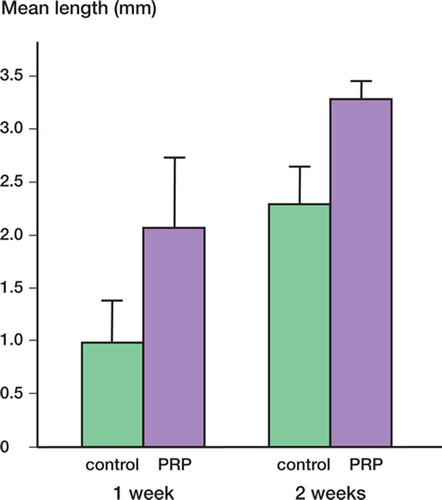
Discussion
We found that PRP combined with vascular bundle implantation accelerates angiogenesis in necrotic bone. Blood platelets contain numerous substances that are capable of affecting angiogenesis, such as VEGF, FGF-2, PDGF, IGF-I, thrombospondin, endostatain, and PF-4 (Bennett et al. Citation2003). Such growth factors released from the platelets mainly promote angiogenesis. Nakamae et al. (Citation2004) reported an acceleration of angiogenesis using a single injection of FGF-2 in the same experimental model as ours. VEGF has been shown to promote angiogenesis and healing of chronic wounds (Brill et al. Citation2004), and to aid in endochondral ossification (Maes et al. Citation2002). Suzuki et al. (Citation2004) reported that local delivery of VEGF for 3 days after surgery significantly improved surgical angiogenesis in necrotic bone during the first postoperative week. Although these reports have demonstrated that each growth factor individually upregulates angiogenesis, Yancoupoulos et al. (Citation2000) reported that individual growth factors such as VEGF and bFGF induce the formation of immature leaky vessels and lead to inflammation, which results in pronounced tissue swelling and edema. It is now well established that angiogenesis is orchestrated by a variety of activators that sequentially coordinate the complex series of events involved in new vessel growth. Brill et al. (Citation2004) reported that the combined effects of FGF-2 and VEGF appear to be more crucial for the entire process of vessel formation than a single administration of FGF-2 or VEGF alone. Since PRP contains these growth factors, it is reasonable to expect that PRP would promote more clinically applicable angiogenesis in necrotic bone. With regard to blood flow, we did not examine this in our study. However, using the same model, Suzuki et al. (Citation2004) included administration of VEGF and showed that blood flow parallels vascular density. Since our study using PRP seems to have resulted in the formation of more mature vessels, we would expect similar results.
Lynch et al. (Citation1989) administered PDGF, IGF-1, EGF, and FGF in various combinations to porcine skin wounds, to determine their effect on the wound-healing process. The synergy of PDGF and IGF-I was optimal at a ratio of 2:1 by weight, and this combination was superior to PDGF at 500 ng given alone. These results suggested that multiple growth factors formed a network, resulting in a synergistic effect. It is also more rational to expect synergy between these growth factors than to expect the same level of effect from any particular one.
Various growth factors are being developed for clinical application. As these cytokines are obtained allogeneically, their clinical use requires caution. In contrast, PRP prepared from autologous blood requires no special considerations concerning antibody formation, the risk of infection or prior diseases by agents such as HIV, hepatitis viruses, or prions, and contains large amounts of growth factors. In these respects, it is suitable as a source of growth factors for clinical use.
Angiogenesis is the formation of new blood vessels by a process involving proliferation and cytotaxis of vascular endothelial cells; it is controlled by growth factors and is under the influence of the vascular structure (Folkman and Klagsbrun Citation1987). It plays a critical role in the osteogenic process. The contribution of vascularization to normal osteogenesis was thoroughly studied by Trueta; this is described in his classic histological studies of the femoral head Trueta (Citation1963). Early studies on revascularization of necrotic bone concentrated on direct implantation of blood vessels into an avascular graft of autogenous bone. Hori et al. (Citation1979) first demonstrated the ability of an implanted vascular bundle to induce proliferation of blood vessels into the necrotic bone. Tamai et al. (Citation1993) reported clinical cases of Kienbock's disease treated by vascular bundle implantation. They showed that vascular bundle implantation was a useful procedure in treating the initial stage of Kienbock's disease. However, none of these studies attempted to measure the rate or extent of angiogenesis quantitatively.
The regeneration of necrotic bone requires the presence of existing vessels, a suitable scaffold, and appropriate cytokines. Our results demonstrate that combining vascular bundle implantation with a single injection of PRP into the necrotic bone accelerates angiogenesis in the bone.
Contributions of authors
KY performed much of the study and wrote most of the article. TS and MO designed the study. OI and TS conducted the study. OS and AN helped in performance of the study. OI and MO helped in writing, and reviewed the article.
- Bennett S P, Griffiths G D, Schor A M, Leese G P, Schor S L. Growth factors in the treatment of diabetic foot ulcer. Br J Surg 2003; 90: 133–46
- Brill A, Elinav H, Varon D. Differencial role of platelet granular mediators in angiogenesis. Cardiovasc Res 2004; 63: 226–35
- Folkman J, Klagsbrun M. Angiogenic factors. Science 1987; 235: 442–7
- Haw C S, O'Brien B M, Kurata T. The microsurgical revascularization of resected segments of tibia in the dog. J Bone Joint Surg (Br) 1978; 60: 266–9
- Hori Y, Tamai S, Okuda H, Sakamato H, Takita T, Masuhara K. Blood vessl transplantation to bone. J Hand Surg (Am) 1979; 4(1)23–33
- Lynch S E, Colvin R B, Antoniades H N. Growth Factors in wound healing. J Clin Invest 1989; 84(2)640–6
- Maes C, Carmeliet P, Moermans K, Stockmans I, Smet N, Collen D, Bouillon R, Carmeliet G. Impaired angiogenesis and endochondral bone formation in mice lacking the vascular endothelial growth factor isoforms VEGF164 and VEGF188. Mech Dev 2002; 111(1–2)61–73
- Malawer M M, Marks M R, McChensney D. The effect of cryosurgery and polymethylmethaclylate in dogs with experimental bone defects comparable to tumor defect. Clin Orthop 1988, 226: 299–310
- Nakamae A, Sunagawa T, Ishida O. Acceleration of surgical angiogenesis in necrotic bone with a single injection of fibroblast growth factor-2 (FGF-2). J Orthop Res 2004; 22: 509–13
- Sunagawa T, Bishop A T, Muramatsu K. Role of conventional and vascularized bone graft in scaphoid nonunion with avascular necrosis: A canine experimental study. J Hand Surg 2000; 25A: 849–59
- Suzuki O, Bishop A T, Sunagawa T, Katsube K, Friedrich P F. VEGF Promoted surgical angiogenesis in necrotic bone. Microsurgery 2004; 24: 85–91
- Tamai S, Yajima H, Ono H. Revascularization procedures in the treatment of Kienbock's disease. Hand Clin 1993; 9: 455–66
- Trueta J. The role of the vessels in osteogenesis. J Bone Joint Surg (Br) 1963; 45: 402–18
- Weiland A J, Phillips T W, Randolph M A. Bone graft: a radiologic, histologic, and biomechanical model comparing autograft, allograft, and free vascularized bone graft. Plast Reconst Surg 1984; 74: 368–79
- Yancopoulos G D, Davis S, Nicholas W G. Vascular-specific growth factors and blood vessel formation. Nature 2000; 407: 242–8