Abstract
Background and purpose A recently developed animal model of posttraumatic contractures reflects the chronic stages of the human condition. To understand the initiation of the process, we evaluated the cellular, matrix, and growth factor changes in the joint capsule in the early stages of the animal model, which would not be possible in humans.
Methods 18 skeletally mature rabbits had intraar-ticular cortical windows removed from the medial and lateral femoral condyles, and the knee joint was immobilized. The contralateral unoperated limb served as a control. Equal numbers of rabbits were killed 2,4, and 6 weeks after surgery. Myofibroblast, mRNA, and protein determinations were done with immunohistochemistry, RT-PCR, and western blot, respectively.
Results Myofibroblast numbers were statistically significantly elevated in the joint capsules of the experimental knees as compared to control knees. The mRNA and protein levels for collagen types I and III, matrix metalloproteinases 1 and 13, and transforming growth factor β1 were statistically significantly greater, and for tissue inhibitor of matrix metalloproteinases 1 significantly less, in the experimental capsules than in the control capsules.
Interpretation The experimental joint capsule changes in the acute stages of posttraumatic contractures are similar to those in the chronic stages of the process in this model. Thus, it appears that the mechanisms that attenuate the acute stages of the response to injury are circumvented, contributing to a prolonged modulation of myofibroblast numbers, matrix molecules and growth factors, and leading to joint contractures. Thus, in clinical practice, new approaches to prevention of posttraumatic contractures should be implemented as soon as possible.
The joint capsule is a key contributor to the formation of posttraumatic contractures, and surgical release to improve joint motion invariably include its removal (Timmerman and Andrews Citation1994, Aldridge III et al. Citation2004, O'Driscoll Citation2006). Changes in the human elbow joint capsule in chronic posttraumatic contractures have recently been characterized and correlated with chronic changes in a newly developed rabbit knee model of posttraumatic contractures (Hildebrand et al. Citation2004a, Citationb, Citation2005, Citation2006, Citation2007). The joint capsule abnormalities followed similar patterns in both cases. It was found that the number of myofibroblasts—a specialized fibroblast expressing the contractile smooth muscle protein α-smooth muscle actin (α-SMA) and associated with contracture and fibrosis in many organ systems—is elevated in joint capsules from posttraumatic contractures relative to similar tissues from normal joints. Growth factor mRNA levels were selectively increased in joint capsules in these chronic contractures, including the myofibroblast inducer transforming growth factor β1 (TGF-β1) and the profibrotic connective tissue growth factor (CTGF). It was also found that mRNA levels for collagen and matrix metalloproteinases (MMPs) were increased, while mRNA levels for tissue inhibitors of matrix metallopro-teinases (TIMP) were reduced in chronic posttrau-matic contractures.
Clinical observations would suggest that the contracture process begins very soon after the injury. Early motion after joint injury lessens the severity of contracture and modern internal fixation techniques have advanced this practice in the last century (Doornberg and Jupiter Citation2006). Methods to prevent heterotopic ossification have to be instituted within a few days of the injury (Rumi et al. Citation2005). We have now investigated early changes in the joint capsule of the animal model of post-traumatic contracture in an attempt to ascertain changes correlated with contracture development. The hypotheses tested were as follows: (1) that myofibroblast numbers, and mRNA and protein levels for α-SMA, collagen, growth factors, and MMPs will be increased while the mRNA and protein levels for TIMPs will be reduced in capsules of experimental joints when compared to similar tissues from the contralateral (unoperated) control joints, and (2) that these changes will occur very soon after the injury, and will remain altered throughout the first 6 weeks post-injury.
Animals and methods
Institutional animal care committee approval was obtained from the University of Calgary Animal Care Committee (October 2003, registration number M01042). 18 skeletally mature New Zealand White female rabbits (5.4 ± 0.5 kg) were obtained from the same source as used in previous studies (Reimans Furrier, St. Agatha, ON, Canada). All rabbits had the following surgical procedure under general anesthesia, as previously described (Hildebrand et al. Citation2004a). Briefly, incisions over the lateral thigh and anterior aspect of the mid-tibia were made in the right hind limb. Medial and lateral parapatellar arthrotomies were made through the thigh incision and 5-mm2 cortical windows were removed from the non-articular cartilage portion of the medial and lateral femoral condyles, preserving the collateral ligament insertions. Bleeding from the condyles resulted in hemarthrosis, simulating a stable intraarticular fracture (Uusitalo et al. Citation2001). The knee joint was then immobilized with a 1.6-mm diameter Kirsch-ner wire drilled through the tibia, passed posterior (extraarticular) to the knee joint and bent around the femur with the knee at 150° of flexion. All skin incisions were closed with 3–0 Ethilon. The rabbits were allowed free cage activity (0.1 m3) following all operations. The left knees were never manipulated surgically and served as controls.
Equal numbers of rabbits were killed with an overdose (1.5 mL) of pentobarbital at 2, 4, or 6 weeks after the procedure. The posterior joint capsule was isolated immediately by dissecting the gastrocnemius muscle from distal to proximal to the fibellae in the heads of the gastrocnemii. The medial collateral and lateral collateral ligaments were identified and then the tibial insertion of the joint capsule was identified. The posterior loose connective tissue and popliteal neurovascular structures were dissected away from the capsule. The capsule was then removed, going from the fibellae proximally, the tibia distally, and collateral ligaments medially and laterally. The capsule was placed in a cryovial and frozen in liquid nitrogen. For the 4-week group, approximately one-third of the capsule was placed in Optimal Cutting Temperature (OCT) embedding material (Sakura Finetek, Torrance, CA) for subsequent immunohistochemi-cal examination. All tissues were stored at −80°C.
The immunohistochemistry studies followed protocols established in our laboratory (Hildebrand et al. Citation2004a, Citationb). A double-labeling protocol was used to differentiate myofibroblasts from blood vessels. 8-μm frozen sections of the samples were cut and pretreated with hyaluroni-dase. Endogenous peroxidases were quenched using 0.3% hydrogen peroxide in methanol, followed by application of the blocking agent (10% normal goat serum in PBS). The monoclonal anti-α-SMA antibody (clone asm-1; Röche Molecular Biochemicals, Laval, QC, Canada) was applied at 5 μ/mL for 60 min, followed by the secondary antibody, horseradish peroxidase-conjugated sheep anti-mouse IgG (Röche) (diluted 1:50), for 60 min. The diaminobenzidine (DAB)/peroxide substrate (Röche) was applied for 13 min. Subsequently, the same sections had primary antibodies to laminin (chicken anti-laminin; GeneTex, San Antonio, TX) applied at a dilution of 1:50 overnight. This was followed by application of secondary antibody, a goat anti-chicken antibody conjugated with Alexa Fluor 488 (Molecular Probes, Eugene, OR) at a dilution of 1:1,000, for 60 min. For all secondary antibody reactions, the slides were kept in the dark. The cellular nuclear stain, 4′-6-diamidine-2-phenyl indole (DAPI) (Vector Laboratories, Burlington, ON, Canada) was applied, followed by coverslips. Appropriate controls were included in all experimental series. For positive controls regarding α-SMA and laminin, blood vessels in capsular tissue were examined. Negative controls included substituting primary antibodies with 3% bovine serum albumin. The sections were viewed under a light microscope (Axioskop 2 plus; Zeiss, Toronto, ON, Canada). Images at 200x were captured from 3 randomly selected areas in each of 6 sections for each specimen using a digital camera (Zeiss Axiocam). Image-Pro Plus software (Media Cybernetics, Silver Spring, MD) was used to analyze cell numbers. Cells with nuclei expressing α-SMA but not expressing laminin were counted as myofibroblasts. Total cell counts were also obtained. The data were collected as absolute numbers of myofibroblasts and also expressed as a ratio of myofibroblast cells to total cells.
Quantification of mRNA was done following methods described previously (Hildebrand et al. Citation2004b, Citation2006). The TRIspin method was used for isolation of total RNA (Reno et al. Citation1996) and the yield was determined fluorometrically.
Semiquantitative reverse transcriptase-PCR (RT-PCR) was performed on all mRNA samples simultaneously to minimize variability in the results. Reverse transcription was carried out using aliquots containing 1 μg of total RNA in the first step of the RT-PCR kit (Stratagene, La Jolla, CA). PCR was performed using the Life Technologies kit (Life Technologies, Gaithersburg, MD). Rabbit-specific primers were used, as published previously (Hildebrand et al. Citation2006, Citation2007). Primer sets were optimized for PCR cycles, and linear amplification curves were plotted. The optimal number of cycles was selected to be in the linear part of the amplification curve. All controls in which the RT stage was omitted were negative, indicating that no genomic DNA was detectable in the RNA samples. All samples used in this study were reverse transcribed at least twice and all PCR experiments were done at least twice, with similar results each time. PCR products were separated by electrophoresis using a 2% agarose gel and ethidium bromide, and the sizes compared with a standard 1-kb DNA ladder (Life Technologies). Images were captured using the Gel-Doc image analysis system (Bio-Rad, Mississauga, ON, Canada). Relative band intensities were quantified by densitometric scanning of negatives using the Masterscan Interpretive Densi-tometer (CSPI, Billerica, MA). The mRNA levels of all molecules were normalized to the mRNA levels of the housekeeping gene glyceraldehyde-3-phosphate dehydrogenase (GAPDH), which did not change during the course of the study.
The protein values were obtained using western blot analysis as previously described (Hildebrand et al. Citation2004b). The frozen posterior capsule tissue was powdered. 1 mL of sample buffer was placed in the dismembrator vessel while the powder was still frozen. After thawing, the slurry was centrifuged and the supernatant fraction was removed and concentrated. Total protein content of the samples was determined using a Bio-Rad protein assay. The protein sample was boiled in sample buffer and 2.5μg protein per lane was run on a 12.5% polyacrylamide precast gel (Owl Scientific, Portsmouth, NH). Fractionated proteins were transferred to nitrocellulose paper overnight (without current) and nonspecific binding sites were blocked using 5% skimmed milk in Tris-buffered saline (TBS). Primary antibodies diluted to 2.5 μg/mL in TBS were added, followed by incubation with sheep anti-mouse IgG conjugated with horseradish peroxidase (Table). Peroxidase activity was revealed with enhanced chemilu-minescent assay detection reagents (Amersham Pharmacia Biotech, Baie d'Urfe, QC, Canada). An image of the peroxidase reaction was captured, and protein density was calculated using Quantity One Software (Bio-Rad). The specific antibodies used all reacted with rabbit tissue ().
Primary antibodies used in western blot
Statistics
Data are presented as mean values with SD in parentheses. For statistical analysis, we used a paired t-test to evaluate differences between experimental and control knees at each time period. An ANOVA with posthoc Tukey test was used to examine differences between experimental groups over time. Values of p less than or equal to 0.05 were considered to be statistically significant.
Results
The rabbits had lost about 5–10% of their starting weight at the time of killing. All K-wires were intact at the time of killing. There were no fractures. Loss of motion was not evident in the 2-week group of experimental knees, but qualitatively limitation of motion was evident in the 6-week experimental knees when preparing the limbs for further studies. The mRNA and protein levels of the housekeeping gene GAPDH showed no statistically significant differences between the experimental and contra-lateral control knees in all groups (0.31 ≤ p ≤ 0.99, data not shown).
Myofibroblast counts were elevated in the capsules of the experimental joints as compared to the contralateral capsules (p < 0.001). The average number of myofibroblasts was 135 (20) in the capsules of the experimental knees of the 4-week group, and 28 (5) in the capsules of the control knees. The proportion of total cells that were myofibroblasts was elevated in the experimental capsules (47% (2%)) when compared to the control capsules (9% (1%)) (p < 0.001). There were no significant differences in the number of total cells between the experimental groups (294 (47)) and the control groups (308 (40)). The myofibroblast marker α-SMA had elevated mRNA levels () (0.001 ≤ p < 0.0001) and protein levels () (0.02 ≤ p < 0.002) in the experimental capsules as compared to the contralateral control capsules. The mRNA and protein levels were significantly elevated by 2 weeks after surgery, and remained elevated for the duration of the study (). There was no effect of time on the mRNA levels in the experimental group, while the experimental 4- and 6-week protein levels were greater than the experimental 2-week values (p = 0.05 and p = 0.006, respectively).
Figure 1. Levels of α-SMA mRNA and protein in joint capsules. (α-SMA is a myofibroblast marker). The values are normalized to GAPDH mRNA and protein.
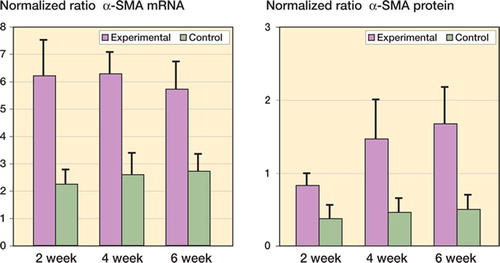
Figure 2. Levels of collagen mRNA and protein in joint capsules. The values are normalized to GAPDH mRNA and protein.
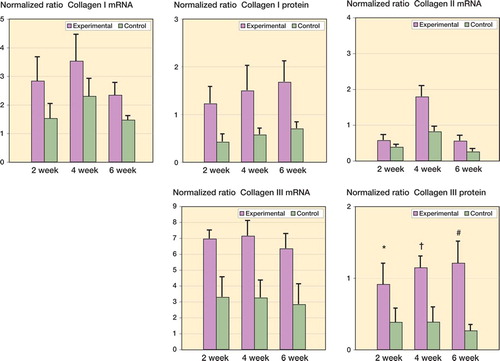
Collagen mRNA and protein levels were elevated in the experimental capsules as compared to contralateral controls (). The mRNA levels for collagen I, II, and III were elevated by 2 weeks after surgery and remained elevated for the duration of the study () (0.02 ≤ p < 0.001). Protein levels of collagen I and III showed a similar pattern () (0.02 ≤ p < 0.001). For the effect of time, the experimental 4-week mRNA levels for collagen I were greater than the experimental 6-week values (p = 0.03). The experimental 4- week mRNA levels for collagen type II were greater than for all other experimental groups (p < 0.001). There was no time effect for collagen type III mRNA levels. For collagen I and III protein, there was also no effect of time between the experimental groups.
The mRNA and protein levels for MMP-1 and MMP-13 were significantly elevated in the experimental capsules as compared to contralateral controls (). It was only in the 4-week group that MMP-1 mRNA levels were elevated in the experimental capsules when compared to the contralateral capsules () (p = 0.04). The MMP-13 mRNA levels were elevated 2 weeks after surgery, and remained elevated for the duration of the study () (0.02 ≤ p ≤ 0.002). Protein levels of MMP-1 and MMP-13 were elevated by 2 weeks and remained elevated until 6 weeks in the experimental joint capsules () (0.008 ≤ p < 0.0001). Regarding the effect of time on the experimental capsules, there were no statistically significant differences in mRNA levels for either MMP between groups. However, when protein levels in the experimental capsules were considered, MMP-1 levels in the 4-week group were significantly greater than the corresponding values for the 2-week group (p = 0.02), while the levels of MMP-13 protein were significantly greater in the 6-week group than in the 4-week group () (p = 0.02).
Figure 3. Levels of MMP mRNA and protein in joint capsules. The values are normalized to GAPDH mRNA and protein.
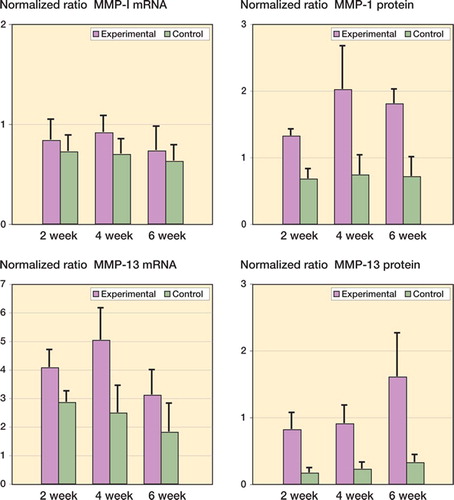
The TIMP mRNA and protein levels were statistically significantly reduced in the experimental capsules compared to contralateral controls (). The mRNA and protein values were significantly less on the experimental side than in contralateral controls by 2 weeks after surgery and remained lower until 6 weeks (0.04 ≤ p < 0.001). Regarding the effect of time on the experimental capsules, there were no statistically significant differences in the mRNA values for any TIMP variants assessed (). However, when considering protein levels in the experimental capsules, the values for the 6-week TIMP-1 group were significantly greater than for the 2-week and 4-week groups () (0.003 ≤ p ≤ 0.007).
Figure 4. Levels of TIMP mRNA and protein in joint capsules. The values are normalized to GAPDH mRNA and protein.
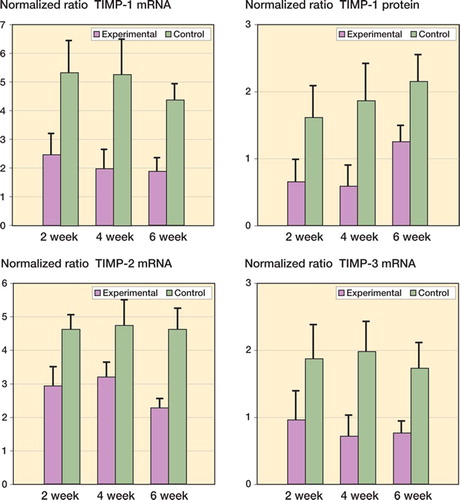
Significant increases in mRNA and protein levels were seen for the growth factor TGF-β1 (). The mRNA and protein levels of this growth factor in experimental joint capsules were greater than their contralateral control values in all groups by 2 weeks after surgery, and remained elevated until 6 weeks () (0.02 ≤ p ≤ 0.001). There were no significant differences in the mRNA and protein levels of TGF-β1 over time in the experimental capsules. The mRNA levels for the ED-A domain of fibronectin and CTGF were not statistically significantly different in the experimental capsules from those in the contralateral control capsules (). There was no effect of time on CTGF mRNA levels in the contracture capsules. The mRNA levels for the ED-A domain of fibronectin in the contracture capsules were greater in the 2-week group than in the 4- and 6-week groups () (p < 0.001). Protein levels were not measured for CTGF or for the ED-A domain of fibronectin.
Discussion
From comparison of contracture knees and control knees, it is clear that changes occur within the joint capsule very early in this animal model of posttrau-matic contractures. The mRNA and protein levels for a number of molecules were significantly altered within 2 weeks of injury. Myofibroblast numbers, and percentage of myofibroblasts relative to total number of cells, were also significantly increased at 4 weeks post-injury. The changes in mRNA and/ or protein levels with increased amounts of collagen I, II, and III, MMP-1 and MMP-13, and also TGF-β1, and decreases in TIMP-1, -2, and -3, persist for at least 6 weeks after injury. In a few cases, there were some significant time-related effects in the contracture capsule. Our findings regarding the changes in these molecules in the joint capsule during the acute stages are similar to the changes observed in the chronic stages of the rabbit knee model of posttraumatic contractures, and to those in chronic human elbow contractures published previously by our laboratory (Hildebrand et al. Citation2004a, Citationb, Citation2005, Citation2006, Citation2007).
In the current study on the acute stages in our animal model of posttraumatic contractures, we did not measure the joint contractures mechanically. However, following 8 weeks of immobilization plus injury, contractures can be readily measured mechanically, and these do not resolve in the model described in the current study (Hildebrand et al. Citation2004a). Preliminary work in our laboratory to develop the animal model and to evaluate precise methods for measurement of contracture showed that contractures can be detected as early as 5 weeks post-injury (Hildebrand et al. Citation2003). Other investigators who immobilized normal rat or rabbit knees reported that contractures could be detected by 2 weeks following immobilization (Finsterbush and Friedman Citation1973, Langenskiöld et al. Citation1979, Trudel and Uhthoff Citation2000). Thus, while it would seem reasonable that contractures would have been detected with biomechanical measurements of the knees in the current study, it would be prudent to refer to the experimental joints as joints at risk of developing contractures. Qualitatively, however, we did detect motion loss in the 6-week experimental knees while preparing the limbs for subsequent studies at the time of killing. Finally, myofibroblast numbers were measured in the 4-week group only. However, in the current study the numbers in the contracture and control capsules are very similar to the myofibroblast numbers reported for the 8-week and 32-week remobilized groups previously, indicating that there is little change over time (Hildebrand et al. Citation2004a).
Early changes in the joint capsule and other joint soft tissues have been observed by several researchers using animal models (Akeson et al. Citation1974,Videman et al. Citation1976, Trudel et al. Citation2000, Matsumoto et al. Citation2002). It was found that incorporation of radioactive sulfate into glycosaminoglycans (GAGs) in the adherent ligaments of the capsule occurred within 4 days of immobilization of uninjured knees in skeletally mature rabbits. The levels of radioactive isotope increased over the 40 days of immobilization (Videman et al. Citation1976). Another group of investigators found that the hexosamine content (a constituent of GAGs) of the periarticu-lar soft tissues decreased along with water content in the immobilized, uninjured skeletally mature rabbit knee from 1 to 6 weeks after immobilization (Akeson et al. Citation1974). In a series of experiments in which an uninjured rat knee was immobilized, changes in several parameters—including reduced synovial intimal length and collagen III levels, with increased collagen I levels—were evident in the joint capsule by 2 and 4 weeks of immobilization (Trudel et al. Citation2000, Matsumoto et al. Citation2002). Clinical experience recognizes the importance of instituting motion within days of injury, to lessen the chance of posttraumatic contractures and to institute nonsteroidal anti-inflammatory therapy or radiation treatment perioperatively, in order to prevent recurrence of heterotopic ossification following surgical releases (Rumi et al. Citation2005, Cohen Citation2006, Doornberg and Jupiter Citation2006). These clinical experiences support the early changes in the joint capsule reported in the current study.
The pattern of early changes in levels of myo-fibroblasts, matrix molecules, and enzymes and growth factors in the joint capsules of injured and immobilized rabbit knees is similar to what has been observed in chronic contractures of the human elbow joint and in the chronic stages of the present animal model (Hildebrand et al. Citation2004a, Citationb, Citation2005, Citation2006, Citation2007). It appears that the contracture process is turned on early during the acute phase and that it does not subsequently revert to baseline conditions in more chronic stages, as has been described in a healing ligament (Frank et al. Citation1983). It is of paramount importance that we should gain an understanding of the factors that contribute to the transition from an acute phase to a chronic stage in contracture formation, instead of reverting to the baseline condition. The mechanisms underlying this phenomenon cannot be determined from the present study, but certain observations suggest lines of investigation for future work.
To understand the factors that cause acute changes in the chronic phase, one must probably consider the model as a whole. Joint injury (intraarticular fracture with subsequent hemarthrosis) in combination with immobilization is required. Several authors have produced contractures in rabbit or rat knee joints and different forms of immobilization have been used (Langenskiöld et al. Citation1979, Akeson et al. Citation1980, Akai et al. Citation1993, Trudel et al. Citation2000). While different methods were used, in all cases some form of immobilization was used—which points to its importance. Immobilization alone is not enough, however, as it appears from the literature that injury is an important factor in developing posttraumatic contractures in animal models. In a mechanical and chemical evaluation of the effect of remobilizing previously uninjured and immobilized rabbit knees, the changes observed after 9 weeks of immobilization were reversed by 9 weeks of remobilization (Akeson et al. Citation1977). Specifically, the increased stiffness of the joint (the torque required to move the joint through a specified range of motion) resolved and the reduced hexosamine and water content returned to control values. In an evaluation of uninjured shoulders of dogs immobilized for 12 weeks and then remobilized for 12 weeks, the joint stiffness, synovial hyperplasia, and changes in collagen type I and III measured after 12 weeks of immobilization reverted back to normal after 12 weeks of remobilization (Schollmeier et al. Citation1996). In the animal model used in the current study, our previous work has shown persistent increases in myofibroblast numbers, and MMP, collagen, and TGF-α1 mRNA levels, and decreases in TIMP mRNA levels in the setting of permanent loss of motion, or contractures, with remobilization that is 4 times longer than the immobilization time (32 weeks and 8 weeks, respectively) (Hildebrand et al. Citation2004a, Citation2004b, Citation2005, Citation2006, Citation2007). The major difference between the animal model used in this study, and the rabbit knee model and canine shoulder model mentioned above is the intraarticular fracture with the accompanying hemarthrosis. The components of the injury that may be important for the process include blood, exposure to elements of bone marrow, or both.
The current study has certain limitations regarding the mRNA methods used. We used a semiquan-titative method of mRNA analysis. This has been well-established in the author's laboratories and gives consistent results. Recent studies comparing results obtained from the semiquantitative method described in the present study with real-time PCR for certain of these molecules have shown similar results (Kydd, Lui, Reno and Hart, unpublished observations). However, as yet we do not have a full complement of validated reagents for the realtime approach. In the current study, the protein levels measured reflected the mRNA levels measured, which supports the validity of the mRNA results we obtained with the semi-quantitative methodology.
In summary, the process leading to posttrau-matic contracture formation appears to be turned on very early in the capsule, and specific factors that keep it in a chronic state of turnover must be activated—thus preventing a return to baseline properties. The rabbit model will continue to facilitate studies on the acute stages of the post-traumatic contracture process, studies that are not possible in humans. The various components of the model (injury, immobilization, timing, remobilization, shams) can be isolated and evaluated for their effects on biomechanical measurements of joint motion and cellular, matrix, and growth factor changes. The animal model can also be used to modify specific influences (growth factors, myofibroblast regulation) in a mechanistic approach. This study supports the current clinical practice of empirically using early motion to reduce the incidence and/or severity of posttrau-matic contractures. It also suggests that any other methods to prevent contracture formation should be instituted as early as possible.
Funding for the study was received from the Canadian Institutes of Health Research (KAH), the Health Research Foundation (KAH), the Alberta Heritage Foundation for Medical Research (KAH), and the Calgary Foundation Grace Glaum Professorship (DAH).
Contributions of authors
KAH, DAH: study design. KAH, MZ, NMG, CW: experiments. KAH, DAH, MZ, NMG: data analysis. KAH, DAH: writing of manuscript.
- Akai M, Shirasaki Y, Tateishi T. Viscoelastic properties of stiff joints: A new approach in analyzing joint contracture. Biomed Mater Eng 1993; 3: 67–73
- Akeson W, Woo S L-Y, Amiel D, Matthews J V. Biomechanical and biochemical changes in the periarticular connective tissue during contracture development in the immobilized rabbit knee. Connect Tissue Res 1974; 2: 315–23
- Akeson W, Woo S L-Y, Amiel D, Doty D. Rapid recovery from contracture in rabbit hindlimb: a correlative biomechanical and biochemical study. Clin Orthop 1977, 122: 359–65
- Akeson W, Amiel D, Woo S L-Y. Immobility effects on synovial joints the pathomechanics of joint contracture. Biorheology 1980; 17: 95–110
- Aldridge J M, III, Atkins T A, Gunneson E E, Urbaniak J R. Anterior release of the elbow for extension loss. J Bone Joint Surg (Am) 2004; 86: 1955–60
- Cohen M S. Heterotopic ossification of the elbow. The Stiff Elbow, J B Jupiter. Am Academy of Orthopaedic Surgeons, Rosemont, IL 2006; 31–40, 1st
- Doornberg J B, Jupiter J B. The posttraumatic stiff elbow: A historical perspective of treatment. The Stiff Elbow, J B Jupiter. American Academy of Orthopaedic Surgeons, Rosemont, IL 2006; 1–7, 1st
- Finsterbush A, Friedman B. Early changes in immobilized rabbits knee joints: A light and electron microscopic study. Clin Orthop 1973, 92: 305–19
- Frank C B, Amiel D, Akeson W H. Healing of the medial collateral ligament of the knee. Acta Orthop Scand 1983; 54: 917–23
- Hildebrand K A, Holmberg M, Shrive N G. A new method to measure post-traumatic joint contractures in the rabbit knee. J Biomech Eng 2003; 125: 887–92
- Hildebrand K A, Sutherland C, Zhang M. Rabbit knee model of post-traumatic joint contractures: the long-term natural history of motion loss and myofibroblasts. J Orthop Res 2004a; 22: 313–20
- Hildebrand K A, Zhang M, van Snellenberg W, King G J W, Hart D A. Myofibroblast numbers are elevated in human elbow joint capsules following trauma. Clin Orthop 2004b, 419: 189–97
- Hildebrand K A, Zhang M, Hart D A. High rate of joint capsule matrix turnover in chronic human elbow contractures. Clin Orthop 2005, 439: 228–34
- Hildebrand K A, Zhang M, Hart D A. Joint Capsule Matrix Turnover in a Rabbit Model of Chronic Joint Contractures: Correlation with Human Contractures. J Orthop Res 2006; 24: 1036–43
- Hildebrand K A, Zhang M, Hart D A. Myofibroblast upregu-lators are elevated in joint capsules in post traumatic contractures. Clin Orthop 2007, 456: 85–91
- Langenskiöld A, Michelsson J-E, Videman T. Osteoarthritis of the knee in the rabbit produced by immobilization. Acta Orthop Scand 1979; 50: 1–14
- Matsumoto F, Trudel G, Uhthoff H K. High collagen type I and low collagen type III levels in knee joint contracture: An immunohistochemical study with histological correlate. Acta Orthop Scand 2002; 73: 335–43
- O'Driscoll S W. Clinical assessment and open and arthroscopic surgical treatment of the stiff elbow. The Stiff Elbow, J B Jupiter. American Academy of Orthopaedic Surgeons, Rosemont, IL 2006; 9–19, 1st
- Reno C, Marchuk L, Sciore P, Frank C B, Hart D A. Rapid isolation of total RNA from small samples of hypocel-lular, dense connective tissues. Biotechniques 1996; 22: 1082–6
- Rumi M N, Deol G S, Bergandi J A, Singapuri K P, Pellegrini V D, Jr. Optimal Timing of Preoperative Radiation for Prophylaxis Against Heterotopic Ossification. A Rabbit Hip Model. J Bone Joint Surg (Am) 2005; 87: 366–73
- Schollmeier G, Sarkar K, Fukuhara K, Uhthoff H. Structural and functional changes in the canine shoulder after cessation of immobilization. Clin Orthop 1996, 323: 310–5
- Timmerman L, Andrews J. Arthroscopic treatment of post-traumatic elbow pain and stiffness. Am J Sports Med 1994; 22: 230–5
- Trudel G, Uhthoff H. Contractures secondary to immobility: Is the restriction articular or muscular? An experimental longitudinal study in the rat knee. Arch Phys Med Rehabil 2000; 81: 6–13
- Trudel G, Seki M, Uhthoff H K. Synovial adhesions are more important than pannus proliferation in the patho-genesis of knee joint contracture after immobilization: An experimental investigation in the rat. J Rheumatol 2000; 27: 351–7
- Uusitalo H, Rantakokko J, Ahonen M, Jamsa T, Tuukkanen J, KaHari V, Vuorio E, Aro H T. A metaphyseal defect model of the femur for studies of murine bone healing. Bone 2001; 28: 423–9
- Videman T, Michelsson J-E, Rauhamäki R, Langenskiöld A. Changes in 35S-Sulphate uptake in different tissues in the knee and hip regions of rabbits during immobilization, remobilization and the development of osteoarthritis. Acta Orthop Scand 1976; 47: 290–8