Abstract
Background and purpose Antibiotic-releasing bioresorbable implants are used for local treatment of bone infections, but most drug delivery systems release antibiotic for too short a time.
Methods We used pellets (0.9 × 1.0 mm) made of bioabsorbable poly(DL) lactic acid matrix, ciprofloxacin (7.3 ± 0.4 wt%), and bioactive glass microspheres of 90–125 μm (29.3 ± 0.2 wt%). The ciprofloxacin release and antibacterial activity was measured in elution tests in vitro and local tissue concentrations were measured in rabbits.
Results In elution tests in vitro, the therapeutic level (> 2 μg/mL) of ciprofloxacin was achieved within 6 h of the start of the test, and it was maintained for up to 300 days. The antibacterial activity of the antibiotic released from sterilized composites was similar to that of the unprocessed ciprofloxacin. In vivo measurements showed high local tissue concentrations (16-86 μg/g of bone tissue) for 3 months. Compared to previous experiments on two-component polymeric matrices (PLGA or PDLLA) with ciprofloxacin alone, adding bioactive glass microspheres into the composite resulted in morphological changes that facilitated fluid intrusion into the microstructure and quickened ciprofloxacin release.
Interpretation This type of composition of implant may fulfill the requirements of bone infection therapy, for sustained local release of the selected antibiotic over several months.
Treatment of deep bone infections remains challenging, with a significant relapse rate (Simpson et al. Citation2001, Cierny et al. Citation2003). As a cornerstone of treatment, the necessary prolonged intravenous antimicrobial therapy (for a minimum of 4–6 weeks) also carries inherent risks of severe systemic complications (Shuford and Steckelberg Citation2003). Thus, alternative approaches to antibiotic delivery have been developed. Local antibiotic therapy would be expected to have several advantages over the use of systemic antibiotics (Holtom and Patzakis Citation2003). Biodegradable implants that release antibiotics may be able to provide adequate local bactericidal tissue concentrations for the length of time needed to completely eradicate infection and prevent relapse. Different drug-delivery systems have been investigated (Wei et al. Citation1991, Garvin et al. Citation1994, Nie et al. Citation1995, Mader et al. Citation1997, Kanellakopou-lou et al. Citation1999, Ambrose et al. Citation2003), but most of them have failed to provide sustained release of the selected antibiotic(s) at a uniform rate for the length of time required (minimum goal of 3–6 months) (Galanakis et al. Citation1997).
We have studied polymeric composites (PLGA and PDLLA) for local delivery of ciprofloxacin (Koort et al. Citation2005, Citation2006, Mäkinen et al. Citation2005). Prolonged antibiotic release has been achieved with PDLLA implants (up to 300 days), but there was an initial period of 60 days with insufficient drug release (Koort et al. Citation2006). The antibiotic release profiles of PLGA implants were more favorable, but the therapeutic concentrations (> 2 μg/mL) were maintained only for 120 days (Mäkinen et al. Citation2005). In this paper, we describe in vitro release of ciprofloxacin from a multifunctional PDLLA composite containing bioactive glass. In addition, we report preliminary in vivo testing of ciprofloxacin release in a rabbit model.
Material and methods
Biomaterials
The implant was constructed from 3 components: polymer matrix, antibiotic, and bioactive glass. The selected bioabsorbable polymer matrix was race-mic poly(DL)-lactide (PDLLA 50:50) (Resomer R206; Boehringer Ingelheim, Ingelheim, Germany). The average molecular weight (Mw) of the polymer was 138,000 g/mol. The antibiotic was ciprofloxacin base (Jinxing Kangle Pharmaceutical Factory, Zhejiang, China). The polymer matrix material was modified by melt-mixing it with the bioactive glass microspheres. The bioactive glass is a synthetic silica-based ceramic, which is resorbed in a physiological environment. The bioactive glass used in this study was ABM 13-93 (Vivoxid, Turku, Finland). The glass was produced in the form of spheres and the particle size was in the 90–125-μm range. The osteoconductive effect of bioactive glass microspheres has been shown in vivo (Välimäki et al. Citation2005).
Manufacture
The implants were manufactured by melt-compounding vacuum-dried components in a small laboratory-scale mixer, thus allowing ciprofloxacin and bioactive glass to be thoroughly dispersed into the microstructure of the implants. Compounded strands of the implants were cut into pellet form. The geometry of pellets was cylindrical with an average diameter of 1.0 mm and length of 0.9 mm. The small size of the pellets was chosen to allow testing of them in an animal model. Small pellet size will also promote diffusion of antibiotic, due to the high surface-to-volume ratio. The processed pellets were dried under vacuum, packed into aluminium-foil pouches in a dry N2 atmosphere and sterilized by γ-irradiation with a nominal dose of 25 kGy (Willy Rüsch AG, Kernen-Rommelshausen, Germany).
The initial ciprofloxacin content of the gamma-sterilized pellets was measured spectrophotometri-cally. Pellets (15 mg) were dissolved in tricholo-romethane (50 mL) and absorbance values were measured using a UV spectrometer (Shimadzu UV-2501PC; Shimadzu Corporation, Japan). The initial ciprofloxacin content was expressed as the mean wt% of 10 parallel samples. The proportion of bioactive glass in the manufactured pellets was determined by burning test (ISO 1172) and expressed as the mean wt% of 5 parallel samples.
Antibiotic release in vitro
In order to determine the release characteristics of the impregnated ciprofloxacin in vitro, gamma-sterilized pellets (500 mg) were placed in 20 mL phosphate buffer solution (NaOH 0.04 mol/l and KH2PO4 0.05 mol/L, pH 7.4). 6 parallel samples were kept in an incubator-shaker at 37°C. After 6, 24, 48, and 96 h, and then every 2-3 days up to 526 days, the buffer solution was removed and replaced. The antibiotic concentration was measured spectrophotometrically and expressed in μg/mL. The value was averaged per day by dividing the concentration measured by the number of days of immersion (i.e. the number of days between changes of buffer solution).
Antibacterial activity of the antibiotic released
In parallel with measurement of ciprofloxacin concentrations, we compared the antibacterial activity of the ciprofloxacin released to the activity of unprocessed ciprofloxacin (Bayer AG, Leverkusen, Germany). The testing was performed on unsterile and gamma-sterilized specimens, as well as on samples of buffer solution from the long-term in vitro antibiotic elution studies. These analyses were done in order to rule out the possibility that exposure of the sterilization process to radiation would affect the bactericidal activity of the antibiotic, and to confirm that the antibiotic released had retained its active form throughout the process of sustained release. The minimal inhibitory concentration (MIC) testing was done using agar-dilution technique (National Committee for Clinical Laboratory Standards 2000). Briefly, inocula of 107 CFU/mL of different sandard bacterium () were placed on Mueller-Hinton II agar plates (Becton Dickinson Microbiology Systems, Cockeysville, MD) with a Denley Multipoint Inoculator (Denley Instruments Ltd., Billingshurst, UK) delivering final inocula of 1 μL (104 CFU/spot). The plates, which contained doubling dilutions of either processed or unprocessed ciprofloxacin, were incubated for 20 h at 35°C in air.
Antimicrobial activity of ciprofloxacin released from the composite as a function of release time in vitro. The efficiency of unprocessed ciprofloxacin (control) is also shown. The values represent the lowest measured concentration (MIC) prohibiting bacterial growth of 5 standard ATCC strains
Morphology of degradation of the biomaterial
Time-related changes in the surface structure of pellets subjected to antibiotic release in vitro were examined by scanning electron microscopy (SEM). The compounded 25-mm-long strands of the composite were kept in phosphate buffer and the sampling (one specimen per time point) was done at intervals of 7 days until 4 weeks, at intervals of 14 days from 5 to 20 weeks, and at intervals of 28 days from 21 to 52 weeks. The hydrolysis samples were vacuum-dried and gold-plated before SEM analysis (JEOL T100; JEOL Ltd., Tokyo, Japan). Changes in surface structure were evaluated visually and no quantitative analysis was done.
Antibiotic release in vivo
A preliminary animal experiment was performed, only to verify the release of ciprofloxacin from the composite in vivo and we had no intention of providing definite data for statistical analysis of the release profile.
The animal model used (intramedullary defect in the proximal tibia filled with pellets), as described in our previous experiments (Mäkinen et al. Citation2005, Koort et al. Citation2006), was originally designed to simulate clinical dead space management of a contained bone defect following radical debridement of localized osteomyelitis. Adult male New Zealand White rabbits (Harlan, Horst, the Netherlands) with an average weight of 2.80 (2.40–3.30) kg were used. The animals received a single dose of benzylpeni-cillin as a standard prophylactic antibiotic (500,000 IU intramuscularly) and were premedicated with a subcutaneous injection of 1 mg/kg atropine. Surgical anesthesia was induced by a subcutaneous injection of 0.3 mL/kg fentanyl citrate-fluanisone, which was supplemented if necessary. Using sterile surgical conditions, a full-thickness cortical bone window (approximately 6 mm × 2.7 mm) was drilled under saline cooling into the proximal medial metaphysis of the right tibia. The size of the cortical defect was determined by the diameter of the drill bit (2.7 mm). Local bone marrow was removed with saline lavage and the defect of the medullary cavity created was impacted with 160 mg of the composite. Based on pilot studies, this was the feasible amount of composite that could be impacted locally into the medullary canal through the cortical bone window. After implantation, periosteum and fascial layers were closed over the defect. The skin wound was closed in layers. At the end of surgery, 0.1 mg/kg of naloxone was injected intramuscularly to reverse the anesthesia. We did not limit functional activity after surgery, and the animals received standard postoperative pain medication (buprenorphine) for 3 days.
We used 4 animals to determine the concentration of ciprofloxacin released in bone tissue as a function of implantation time. One animal was killed at each time point (2, 4, 6, and 12 weeks). In addition, 1 animal was used to monitor systemic antibiotic exposure by determination of ciprofloxacin concentration in the peripheral blood. From this rabbit, 10-ml arterial blood samples were taken from the ear artery at 6 h, 24 h, and thereafter once a week for 8 weeks. The animal was killed at 24 weeks, and bone tissue specimens were harvested for measurement of ciprofloxacin concentration.
Determination of ciprofloxacin levels in serum and bone
We used a high-performance liquid chromato-graphic (HPLC) method with fluorescence detection (FLD) for determination of serum ciprofloxacin concentrations (CRST Bioanalytics, Turku, Finland). Ofloxacin was used as the internal standard.
The frozen serum samples were thawed in a refrigerator and prepared for analysis by protein precipitation with perchloric acid. HPLC separations were performed with a Nova-Pak C18 150 × 3.9 mm i.d. column coupled to an integrated Nova-Pak C18 guard column (Waters Co., Milford, MA). The analyses were carried out on an HPLC system consisting of a Waters 2690 Separations Module and an Applied Biosystems 980 programmable fluorescence detector. The concentration of ciprofloxacin measured in serum was expressed in ng/mL.
We measured bone tissue concentrations of ciprofloxacin at two locations of the tibia. The first tissue sample was taken from the proximal tibial metaphysis containing trabecular and cortical bone. This area was a few millimeters proximal to the implantation site of the composite. The second tissue sample was taken starting 5 mm distally from the distal edge of the defect and was comprised of mostly cortical bone. The bone specimens were cut with a high-speed diamond-blade saw, cleaned of any composite material, and pulverized with a homogenizer (Mikro-Dismembrator S; B. Brown, Melsungen, Germany). Ciprofloxacin was extracted from 100–400 mg of the fine-ground bone with a solution containing methanol and buffer. HPLC-FLD analysis was carried out using a Waters 2695 separations module and Waters 2475 multi λ-fluorescence detector. The ciprofloxacin concentrations measured were expressed per weight of bone tissue (μg/g).
Ethical considerations
The Ethical Committee of the University of Turku and the Provincial State Office of Western Finland approved the study protocol (Reg. # 928/99, issued September 1999) and the study was carried out in accordance with the guidelines of the local Animal Welfare Committee.
Results
Ciprofloxacin release in vitro
The initial ciprofloxacin and bioactive glass content of the pellets was 7.3 (SD 0.4) wt% (n = 10) and 29.3 (SD 0.2) wt% (n = 5), respectively. The therapeutic level of ciprofloxacin (> 2 μg/mL) was reached from the very beginning of the in vitro immersion test, and it was maintained up to 300 days (). After the initial burst release during the first 6 h, the concentrations remained below the potentially detrimental level for osteoblastic cells (20 μg/mL) (Miclau et al. Citation1998). During the sustained long-term release, the highest concentration of ciprofloxacin released per day (19 μg/mL) was measured on the day 46. All the ciprofloxacin loaded had been released from the pellets by 526 days ().
Antimicrobial activity
Based on in vitro testing against 5 ATCC bone pathogens, the ciprofloxacin had remained active throughout the manufacturing process (Table). The activity of the antibiotic released showed no diminution during the follow-up period of 436 days. The antimicrobial activity of the released antibiotic against different pathogens was similar to that of the unprocessed ciprofloxacin. Gamma sterilization had no adverse effect on the antimicrobial activity. The MICs of ciprofloxacin released from unsterile and sterilized composites against the 5 ATCC strains were similar to those of the control (unprocessed) ciprofloxacin.
Morphology of degraded composite
The cut surface of the composite at the start-up after compounding and during the hydrolysis test was examined under SEM. The addition of bioactive glass microspheres introduced a porous morphology of melt-compounded strands (). The adhesion between the bioabsorbable matrix polymer and bioactive glass was not firm, which resulted in pore space around the bioactive glass particles. This facilitated the penetration of phosphate buffer solution into the microstructure of the pellets. The microstructure of the composite changed during the in vitro elution test (). More pores developed in the matrix as a result of hydrolysis of the matrix, fluid penetration, and simultaneous dissolution of ciprofloxacin.
Figure 2. SEM micrograph (100× magnification) of a composite microcylinder (pellet) at the start-up after melt-compounding. The PDLLA matrix mixed with bioactive glass microspheres showed microcavities and pores, which facilitated initial intrusion of fluid into the microstructure.
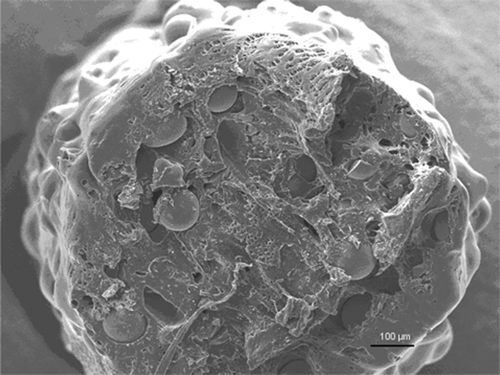
Figure 3. SEM micrographs (500× magnification) of composite microcylinders as a function of immersion time in phosphate buffer. Consecutive representative specimens from two time points: immediately after processing (A) and at day 49 of the immersion test (B). The cut surfaces of the implants, examined under SEM, showed that the PDLLA matrix developed microporosity during degradation and release of ciprofloxacin.
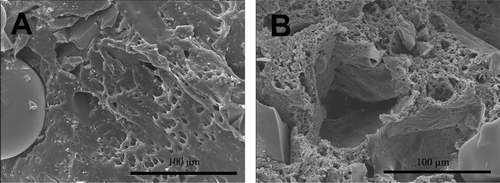
Ciprofloxacin concentrations in vivo
High ciprofloxacin concentrations were measured in the bone tissue samples harvested from the rabbit proximal tibia. The concentrations of ciprofloxacin measured per gram of bone tissue (one animal per time point) were 48 μg/g at 2 weeks, 86 μg/g at 4 weeks, 16 μg/g at 6 weeks, 45 μg/g at 12 weeks, and 0.48 μg/g at 24 weeks. Under similar conditions, a single therapeutic dose (10 mg/kg) of intravenous ciprofloxacin will result in a bone tissue concentration of 0.8 μg/g in the rabbit proximal tibia 120 min after infusion (Koort et al. Citation2005). The cortical bone specimens harvested more distally from the implantation site showed lower concentrations of ciprofloxacin. The concentrations of ciprofloxacin measured per gram of bone tissue were 1.7 μg/g at 2 weeks, 0.78 μg/g at 4 weeks, 2.1 μg/g at 6 weeks, 3.0 μg/g at 12 weeks, and 0.1 μg/g at 24 weeks. The serum concentration of ciprofloxacin remained undetectable and below the resolution of the testing method (< 5.0 ng/mL) in the animal followed for 24 weeks.
Discussion
We aimed at obtaining a sustained release of ciprofloxacin from multifunctional PDLLA composite by modifying it through addition of bioactive glass microspheres. Adding microspheres into the structure enhanced hydrolysis of the matrix, fluid penetration, and simultaneous dissolution of ciprofloxacin. As a result, the antibiotic was released from the composite at therapeutic levels and in a controlled manner for up to 300 days in vitro. In vivo, the delivery system produced high local bone concentrations for a minimum time period of 3 months. The antimicrobial activity of the ciprofloxacin released did not appear to be adversely affected by the extended time periods involved.
As observed previously with PDLLA composites (Koort et al. Citation2006), the release profile of antibiotic in vitro showed two major peaks: an initial burst and a second peak after 50 days. The second peak appeared during the time that the matrix developed microporous structure, as observed from SEM images. This microporous transformation is caused by gradual hydrolytic breakdown of the polymeric matrix as a result of cleavage of ester linkages.
Although currently relevant, the role of quino-lones in the treatment of deep bone infections is still unclear. It is evident that prolonged systemic treatment with quinolones carries an increased risk of development of resistant strains, but to date, there have been no reports on whether this holds true for local antibiotic treatment. During the past decade, multidrug resistance has become common in both S. aureus and S. epidermidis strains (Giannoudis et al. Citation2005). Overall, the development and spread of resistant strains in orthopedic units (Kotilainen et al. Citation1995) is a complex issue, with many still unknown factors involved. Introduction of any new antimicrobial treatment modality requires careful safety studies. Methicillin-resistant strains frequently require combination therapy with two different antibiotics. Fortunately, the use of local drug delivery systems is amenable to and even ideal for this type of targeted long-lasting infection therapy. Our experience in the development of a drug-delivery system for release of one antibiotic suggests that the task will not be easy. The thermostability of different antibiotics may limit their incorporation with common polymers. Without doubt, it will be challenging but not impossible to find a suitable form of composite for release of 2 antibiotics at a uniform rate and at therapeutic concentrations for at least 3 months.
Using the same rabbit model and the highly sensitive HPLC method of analysis (Mäkinen et al. Citation2005, Koort et al. Citation2006), we have already shown that systemic exposure is rare and occurs only momentarily (mainly during the initial burst release) in local therapy with biodegradable PLGA or PDLLA antibiotic-releasing implants. Thus, it is unlikely that the therapy would result in prolonged systemic exposure to antibiotics at subinhibitory levels, which might allow mutational resistance to occur. The main purpose of bioactive glass micro-spheres is to provide the implant with osteocon-ductive properties. In the current study, we did not explore the osteoconductivity of the composite or the rate of dissolution of bioactive glass, but our previous studies have suggested that bioactive glass microspheres can provide the implant with an independent osteoconductive property (Koort et al. Citation2005, Mäkinen et al. Citation2005). Another important, although still incompletely characterized feature might be the bacteriostatic activity of bioactive glass in vitro and in vivo (Stoor et al. Citation2001).
The composite as used here seems to fulfill the basic requirements for a suitable drug-delivery system for long-lasting local therapy of bone infections. As compared to previous drug-delivery systems, the present composite seems to provide extended release of the antibiotic (for up to several months) and an osteoconductive agent is also included as part of the polymeric composite. This type of composite may allow debridement and filling of an osteomyelitic focus in a single operation. The composite might also be used in prevention of infection in contaminated open fractures with bone loss, without the need for secondary bone grafting. Definitive in vivo studies are needed to assess the safety and efficacy of this treatment modality.
JKK and MV are Ph.D. students at the Finnish National Graduate School for Musculoskeletal Diseases and Bioma-terials (TBGS). The work was financially supported by the National Agency for Innovation and Technology (TEKES # 40176/02), Finland.
Contributions of authors
JKK and TJM designed the experiment, performed the animal surgeries, and wrote the manuscript. MV, ES, and PT manufactured the biomaterial and performed the in vitro elution tests. JJ performed the microbiological analyses. HTA also designed the experiment and wrote the manuscript.
- Ambrose C G, Gogola G R, Clyburn T A, Raymond A K, Peng A S, Mikos A G. Antibiotic microspheres: preliminary testing for potential treatment of osteomyelitis. Clin Orthop 2003, 415: 279–85
- Cierny G III, Mader J T, Penninck J J. A clinical staging system for adult osteomyelitis. Clin Orthop 2003, 414: 7–24
- Galanakis N, Giamarellou H, Moussas T, Dounis E. Chronic osteomyelitis caused by multi-resistant Gram-negative bacteria: evaluation of treatment with newer quinolones after prolonged follow-up. J Antimicrob Chemother 1997; 39(2)241–6
- Garvin K L, Miyano J A, Robinson D, Giger D, Novak J, Radio S. Polylactide/polyglycolide antibiotic implants in the treatment of osteomyelitis. J Bone Joint Surg (Am) 1994; 76(10)1500–6
- Giannoudis P V, Parker J, Wilcox M H. Methicillin-resistant Staphylococcus aureus in trauma and orthopaedic practice. J Bone Joint Surg (Br) 2005; 87(6)749–54
- Holtom P D, Patzakis M J. Newer methods of antimicrobial delivery for bone and joint infections. AAOS Instructional Course Lectures 2003; 52: 745–9
- Kanellakopoulou K, Kolia M, Anastassiadis A, Korakis T, Giamarellous-Bourboulis E J, Andreopoulos A, Dounis E, Giamarellou H. Lactic acid polymers as biodegradable carriers of fluoroquinolones: An in vitro study. Antimicrob Agents Chemother 1999; 43(3)714–6
- Koort J K, Mäkinen T J, Suokas E, Veiranto M, Jalava J, Knuuti J, Törmälä P, Aro H T. Efficacy of ciprofloxacin releasing bioabsorbable osteoconductive bone defect filler for treatment of experimental osteomyelitis due to Staphylococcus aureus. Antimicrob Agents Chemother 2005; 49(4)1502–8
- Koort J K, Suokas E, Veiranto M, Mäkinen T J, Jalava J, Törmälä P, Aro H T. In vitro and in vivo testing of bioabsorbable antibiotic containing bone filler for osteomyelitis treatment. J Biomed Mater Res A 2006; 78(1)532–40
- Kotilainen P, Huovinen S, Jarvinen H, Aro H, Huovinen P. Epidemiology of the colonization of inpatients and outpatients with ciprofloxacin-resistant coagulase-negative staphylococci. Clin Infect Dis 1995; 21(3)685–7
- Mader J T, Calhoun J, Cobos J. In vitro evaluation of antibiotic diffusion from antibiotic-impregnated biodegradable beads and polymethylmetacrylate beads. Antimicrob Agents Chemother 1997; 41(2)415–8
- Miclau T, Edin M L, Lester G E, Lindsey R W, Dahners L E. Effect of ciprofloxacin on the proliferation of osteoblast-like MG-63 human osteosarcoma cells in vitro. J Orthop Res 1998; 16(4)509–12
- Mäkinen T J, Veiranto M, Lankinen P, Moritz N, Jalava J, Törmälä P, Aro H T. In vitro and in vivo release of ciprofloxacin from osteoconductive bone defect filler. J Antimicrob Chemother 2005; 56(6)1063–8
- National Committee for Clinical Laboratory Standards. Methods for Dilution Antimicrobial Susceptibility Tests for Bacteria that Grow Aerobically- Fifth Edition: Approved Standard M7-A5. NCCLS. Wayne, PAUSA 2000
- Nie L, Nicolau D P, Nightingale C H, Browner B D, Quintiliani R. In vitro elution of ofloxacin from a bioabsorbable polymer. Acta Orthop Scand 1995; 66(4)365–8
- Shuford J A, Steckelberg J M. Role of oral antimicrobial therapy in the management of osteomyelitis. Curr Opin Infect Dis 2003; 16(6)515–9
- Simpson A H, Deakin M, Latham J M. Chronic osteomyelitis. The effect of the extent of surgical resection on infection-free survival. J Bone Joint Surg (Br) 2001; 83(3)403–7
- Stoor P, Söderling E, Grénman R. Bioactive glass S53P4 in repair of septal perforations and its interactions with the respiratory infection-associated microorganisms Hae-mophilus influenzae and Streptococcus pneumoniae. J Biomed Mater Res 2001; 58(1)113–20
- Välimäki V V, Yrjans J J, Vuorio E I, Aro H T. Molecular biological evaluation of bioactive glass microspheres and adjunct bone morphogenetic protein 2 gene transfer in the enhancement of new bone formation. Tissue Eng 2005; 11(3-4)387–94
- Wei G, Kotoura Y, Oka M, Yamamuro T, Wada R, Hyon S-H, Ikada Y. A bioabsorbable delivery system for antibiotic treatment of osteomyelitis. J Bone Joint Surg (Br) 1991; 73(2)246–52