Abstract
Background Interaction between wear particle debris and the cells at the implant-bone interface is an important contributory factor to periprosthetic bone loss seen in arthroplasties.
Method To investigate the effect of this particle-induced response on different stages of osteoclast maturation, polymethylmethacrylate (PMMA) particles were added to a murine osteoclastogenic bone marrow cell culture system at either day 0, day 4, or day 8 of culture, which represented PMMA particle stimulation of precursor osteoclasts, mature osteoclasts, or end-stage osteoclasts, respectively. The number of TRAP-posi-tive multinucleate cells (MNCs) and the degree of bone resorption in culture were measured
Results Treatment of precursor osteoclasts with PMMA particles resulted in a statistically significant increase in TRAP-positive MNCs that persisted for 4 days, but there was no significant increase in bone resorption. Addition of particles to mature osteoclasts resulted in a significant increase in the number of TRAP-positive MNCs that lasted for 8 days, and also a significant increase in bone resorption. Treatment of end-stage osteoclasts with PMMA particles did not result in an increased number of TRAP-positive MNCs and there was no increase in bone resorption.
Interpretation Treatment of mature osteoclasts with PMMA particles resulted in an elevated number of TRAP-positive cells. This persisted over a longer period of time than at the other stages of osteoclast development, and there was also a greater increase in bone resorption.
Osteolysis, or periprosthetic bone loss, has been implicated as a major cause of aseptic loosening seen in arthroplasties (Amstutz et al. Citation1992). Clinically, increased bone resorption and multinucleate cell (MNC) formation have been found to accompany an inflammatory reaction that occurs in the tissue surrounding the implant (Willert et al. Citation1990, Jiranek et al. Citation1993). Mature macrophages and mononuclear phagocytes involved in this inflammatory response are capable of differentiating into osteoclasts, which are in turn capable of bone resorption (Sabokbar et al. Citation1997, Haynes et al. Citation2001).
Wear particles from biomaterials have been implicated as one cause of increased osteoclastogenesis and bone resorption (Maloney et al. Citation1996, Sabokbar et al. Citation1996, Kobayashi et al. Citation1997, Haynes et al. Citation2004). Wear debris particles might cause an increased recruitment of precursor osteoclasts to the microenvironment surrounding the implant, increased differentiation of precursor osteoclasts into mature osteoclasts, activation of mature osteoclasts already present at the bone surface, and/or extended survival time of mature osteoclasts (Greenfield et al. Citation2002).
The area surrounding the prosthesis contains a mixed population of osteoclast cells: precursor osteoclasts, mature osteoclasts, and end-stage osteoclasts. We believe that each of these developmental stages of the osteoclast may contribute to the increased bone resorption and osteoclas-togenesis that occurs in response to the presence of PMMA particles. To examine these individual contributions, we quantified the in vitro effects on osteoclastogenesis and bone resorption of adding PMMA particles to each of these developmental stages of the osteoclast.
Material and methods
Cell culture
Commercially available spherical PMMA particles (Polysciences Inc., Warrington, PA) were used for all experiments. The particles were 1–10 μm in diameter (95% were < 10 μm in diameter), with a mean diameter of 4.5 μm. The particles were rinsed in ethanol 4 times, sterilized in ethanol overnight, and then rinsed 4 times with phosphate-buffered saline (PBS). They were resuspended in serum-free alpha-minimal essential medium (alpha-MEM) and stored at 20°C. For culture experiments with whole bone marrow, an optimal PMMA particle concentration represented 4.5 × 106 particles per 1.0 × 106 plated cells in 1 mL of medium.
Osteoclastogenic bone marrow cultures were obtained by isolating whole bone marrow cells from the tibia and femur of 4–6-week-old C57BL/6J mice (Jackson Laboratory, Bar Harbor, ME). Animals were killed using CO2. Bone marrow was harvested as described previously, with slight modification (Murrills et al. Citation1990). Briefly, the femurs and tibias of mice were aseptically removed and dissected free of adhering tissue. The bone ends were cut off with scissors and the bone marrow was flushed from the diaphysis with a syringe and 25-gauge needle, and collected in primary culture medium (alpha-MEM containing L-glutamine, nucleosides, 10% fetal bovine serum, and 1% antibiotics (penicillin and streptomycin) (all from GIBCO). Red blood cells were lysed by treatment with 1X H2O-phosphate-buffered saline (PBS) solution (GIBCO). Bone marrow cells were cultured overnight in a 25-mL cell culture flask in the presence of 5 ng/mL recombinant murine macro-phage-colony stimulating factor (M-CSF) (Pepro Tech, Rocky Hill, NJ). Non-adherent cells were collected and plated at a density of 1 × 105/well in a 96-well plate with devitalized bovine bone slices (4×4 mm2) on the bottom of the plate. After removal of non-adherent cells by rinsing in PBS, the bone slices were transferred to another 96-well plate. At this time, the cells were cultured in primary culture medium in the presence of 20 ng/mL M-CSF and 60 ng/mL recombinant murine receptor activator of nuclear factor-κβ (NF-κβ) ligand (RANKL) (Pepro Tech). The slices were then incubated at 37°C for 12 days in an atmosphere of 5% CO2. Changes of media with M-CSF and RANKL were performed every 2 days, for up to 12 days.
Particles were added to the culture at day 0, day 4, or day 8 of culture. Particles added at day 0 represented particle stimulation of precursor osteo-clasts, which were defined as mononuclear cells that did not stain positive for tartrate-resistant acid phosphatase (TRAP). Samples from addition of PMMA to precursor osteoclasts were harvested at days 4, 8, and 12, corresponding to 4 days, 8 days, and 12 days of particle stimulation.
Particles added at day 4 of culture represented particle stimulation of mature osteoclasts, which were defined as TRAP-positive cells with 3 or more visible nuclei. These cells began to appear in the control cultures at day 4. The samples from addition of PMMA to mature osteoclasts were harvested at days 8 and 12 of culture, corresponding to 4 days and 8 days of particle stimulation.
Particles added at day 8 of culture represented particle stimulation of end-stage osteoclasts. These were defined as TRAP-positive cells that had an appearance consistent with reduced cellular activity (smaller size, condensed cytoplasm, and visibly condensed nucleus). These samples were harvested at day 12, which corresponded to 4 days of particle stimulation in this group of cells. Osteoclast cultures without particles served as controls.
Tartrate-resistant acid phosphatase staining
Upon harvesting of the bone slices, the cells were fixed and TRAP stained to detect osteoclast cells in culture (Minkin Citation1982). TRAP-positive multinucle-ate cells (MNCs) were defined as those having 3 or more nuclei (Hata et al. Citation1992). The bone slices were scanned using brightfield optics on a Nikon Eclipse 800 upright microscope at a magnification of 450×, and analyzed using the Metamorph image analyzer (Molecular Devices Corporation, Downingtown, PA). For each bone slice, 5 images (each with an area of 0.182 mm2) were obtained, 1 at each corner and 1 in the center. The number of TRAP-positive cells was counted and data were expressed as the number of cells per unit area (number/mm2). 5 bone slices were used for each group at each time point (n = 5). The number of TRAP-positive MNCs present on the bone slice was used as a measure of osteoclast differentiation. All analyses was performed on coded sections by an independent observer.
Assessment of bone resorption
Bone resorption was assayed using the disaggregated osteoclast resorption assay (Boyde et al. Citation1984). After TRAP staining analysis, the cells were soaked in 1M NH4OH for 3 min to remove adherent cells. Resorption pits were visualized using a Nikon Eclipse ME600L brightfield/dark-field reflected light microscope at 200× magnification, and resorption pit area measurements were done using Bioquant Nova histomorphometry software (Bioquant Image Analysis Corp., Nashville, TN). The size of the pits was measured manually by outlining the perimeter of the pits on the bone slice. The results were expressed as the percentage of bone slice occupied by resorption pits. 5 bone slices were used for each group at each time point (n = 5). All analyses was performed on coded sections by an independent observer.
Statistics
All data are presented as mean (SD). The data were analyzed using a one-way analysis of variance (ANOVA) to identify significant differences. A Bonferroni post hoc test was used to identify significance for pairwise comparisons between treatment groups. A p-value of < 0.05 was considered significant in all analyses.
Results
No addition of PMMA particles
At day 0 in the control group, all cells in the culture were mononuclear and negative for TRAP. At day 4, TRAP-positive mononuclear cells began to fuse to form TRAP-positive multinucleate cells (MNCs), and on average there were 21(1.6) TRAP-positive MNCs/mm2 in the control. At days 8 and 12, the number of TRAP-positive MNCs decreased (8.5 (5.6) TRAP-positive MNCs/mm2 and 6.3 (2.3) TRAP-positive MNCs/mm2, respectively), and some of the cells appeared to be smaller in size with no visible nucleus ().
Figure 1. Number of TRAP-positive MNCs in culture. Particles were added to precursor osteoclasts, mature osteo-clasts, or end-stage osteoclasts, and a corresponding culture system without PMMA particles served as a control. The results are expressed as the number of TRAP-positive MNCs per mm2 ± SD. The particle-stimulated mature osteoclasts had an increased number of TRAP-positive MNCs at days 8 and 12 compared to the control group, the particle-stimulated precursor osteoclasts, and particle-stimulated end-stage osteoclasts at the same time points. (Asterisk denotes significance at the p < 0.05 level).
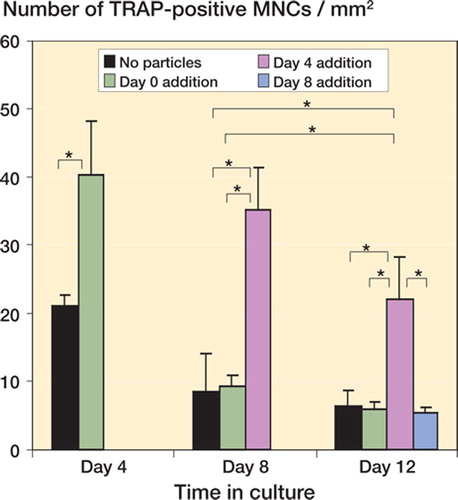
In the controls, lacunar bone resorption began at day 4, at which time 7.2 (2.0) percent of the bone surface was covered by resorption pits. The percentage of bone surface occupied by resorption pits increased at day 8 and day 12 to 17% (2.1) and 21% (5.4), respectively ().
Figure 2. Percentage of resorbed bone surface. Particles were added to precursor osteoclasts, mature osteoclasts, or end-stage osteoclasts while one group with no particles added served as a control. The results are expressed as percentage of the bone slice surface occupied by resorp-tion pits ± SD. The particle-stimulated mature osteoclasts had a significantly higher percentage of the bone surface occupied by resorption pits at day 8 and day 12, compared to all other groups. (Asterisk denotes significance at the p < 0.05 level).
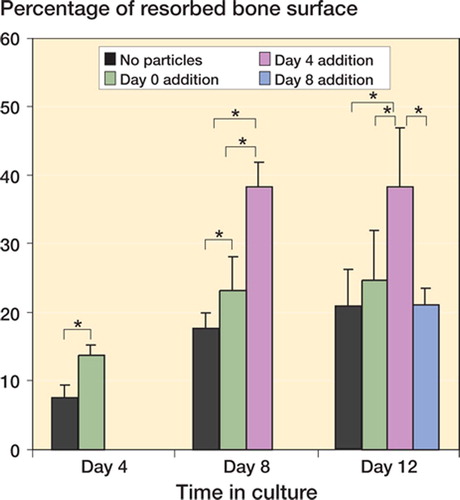
Stimulation of precursor osteoclast cells with PMMA particles
In the particle-stimulated precursor osteoclasts at day 4, there were 40 (8.0) TRAP-positive MNCs/ mm2 as compared to 21.0 (1.5) in the control (p < 0.001) ( and ). At days 8 and 12, there were 9.1 (1.6) and 6.0 (1.0) TRAP-positive MNCs/mm2, respectively, in the particle-stimulated precursor osteoclasts, compared to 8.5 (5.6) and 6.3 (2.3) TRAP-positive MNCs/mm2, respectively, in the control group () (p = 0.82, p = 0.80, respectively).
Figure 3. Light microscope image of TRAP-positive mononuclear and multinucleate cells at (A) day 4 in the control group, and (B) day 4 in the particle-stimulated precursor osteoclasts (450× magnification).
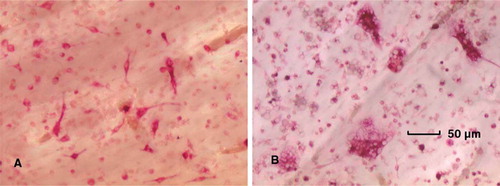
The particle-stimulated precursor osteoclasts had 14% (1.3), 23% (4.9), and 25% (7.2) of the bone surface covered by resorption pits at days 4, 8, and 12, respectively, as compared to 7.2% (2.0), 17% (2.1) and 21% (5.4), respectively, in the controls at the same time points (p < 0.001, p < 0.05, p = 0.3, respectively) ( and ).
Figure 4. Reflectance microscope image of bovine bone slices. Bone resorption pits appeared as a dark shade on the gray scale. Images were taken (A) at day 12 in the control group, (B) at day 12 in the particle-stimulated precursor osteoclasts, (C) at day 12 in the particle-stimulated mature osteoclasts, and (D) at day 12 in the particle-stimulated end-stage osteoclasts (200× magnification).
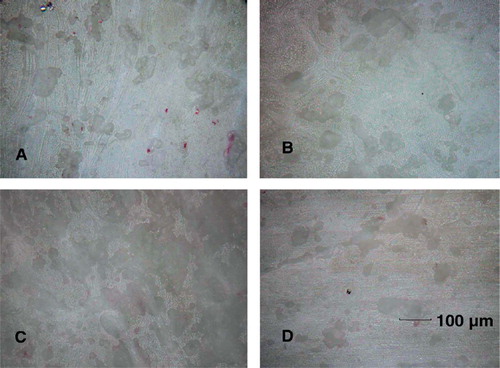
Stimulation of mature osteoclast cells with PMMA particles
In the particle-stimulated mature osteoclasts at day 8, there were 35 (6.0) TRAP-positive MNCs/mm2, which was significantly greater than for the control (8.5 (5.6) TRAP-positive MNCs/mm2) and for the particle-stimulated precursor osteoclasts (9.1 (1.6) TRAP-positive MNCs/mm2) (p < 0.001 and p < 0.001, respectively) ( and ). At day 12, there were 22 (6.3) TRAP-positive MNCs/ mm2 in the particle-stimulated mature osteoclasts, which was greater than for the control (6.3 (2.3) TRAP-positive MNCs/mm2), for the particle-stimulated precursor osteoclasts (6.0 (1.0) TRAP-positive MNCs/mm2), and for the particle-stimulated end-stage osteoclasts (5.3 (0.9) TRAP-positive MNCs/mm2) (p < 0.001, p < 0.001, and p < 0.001, respectively). In addition, the number of TRAP-positive MNCs in the particle-stimulated mature osteoclasts at day 12 was greater than in the control, and greater than in the particle-stimulated precursor osteoclasts at day 8 (p = 0.001 and p = 0.002, respectively) ().
Figure 5. Light microscope image of TRAP-positive mononuclear and multinucleate cells in (A) the control group at day 8, (B) the particle-stimulated precursor osteoclasts at day 8, and (C) the particle-stimulated mature osteoclasts at day 8 (450× magnification).
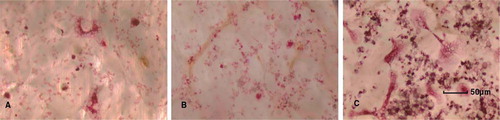
At day 8, the particle-stimulated mature osteoclasts had 38.% (3.5) of the bone surface occupied by resorption pits, which was greater than in the control (17.% (2.1)) and in the particle-stimulated precursor osteoclasts (23.% (4.9)) (p < 0.001 and p = 0.02, respectively) (). At day 12, the particle-stimulated mature osteoclasts had 38.% (SD 8.6) of the bone surface occupied by resorption pits, which was greater than for the control group (21% (5.4)), for the particle-stimulated precursor osteoclasts (25% (SD 7.2)), and for the end-stage osteoclasts (21% (2.4)) (p < 0.001, p = 0.004, and p = 0.008, respectively) ( and ).
Stimulation of end-stage osteoclast cells with PMMA particles
In the particle-stimulated end-stage osteoclasts at day 12, there were 5.3 (0.9) TRAP-positive MNCs/ mm2, which was not statistically significantly different from the control (6.3 (2.3)) ().
At day 12, the particle-stimulated end-stage osteoclasts had 21% (2.4) of the bone surface occupied by resorption pits. This was not statistically significantly different from the control (21% (5.4)) ( and ).
Discussion
A correlation has been found in clinical studies between the presence of wear particles, multinucleate cells, and increased bone resorption in patients who experience aseptic failure of arthroplasties (Wang et al. Citation1997, Haynes et al. Citation2001). Studies that have attempted to examine this relationship in vitro have mainly focused on the role of precursor osteoclast differentiation in response to debris resulting from wear particles. One study by Sabokbar et al. (Citation1996) found that PMMA particles added to a mouse monocyte cell culture system at day 0 resulted in an increase in both the number of TRAP-positive MNCs after 7 days of culture and in bone resorption after 14 days of culture. Other studies have also suggested that wear particles increase the degree of differentiation of precursor osteoclasts into mature osteoclasts, which leads to an increase in bone resorption in the culture (Pandey et al. Citation1996, Quinn et al. Citation1996, Sabokbar et al. Citation1997, Bi et al. Citation2001).
In contrast to these past studies, in which the responses of the different stages of osteoclast development were not differentiated from one another, we attempted to isolate the effects of PMMA particles on precursor osteoclasts, mature osteoclasts, and end-stage osteoclasts. Particles added in the presence of mature osteoclasts caused an increase in osteoclastogenesis that persisted over a longer time period than in the particle-stimulated precursor osteoclast culture. Also, of all the stages of particle-stimulated osteoclast development, addition of particles to mature osteoclasts resulted in the greatest increase in bone resorption.
In this work, all osteoclast cell cultures were treated with receptor activator of NF-κβ ligand (RANKL). In precursor and mature osteoclasts, the interaction between RANKL and its receptor, receptor activator of NF-κβ (RANK), is a primary regulator of osteoclast differentiation and activity (Lacey et al. Citation1998, Burgess et al. Citation1999, Hsu et al. Citation1999, Clohisy et al. Citation2003, Boyce et al. Citation2005). Previously published experiments in vivo and in vitro have illustrated the importance of the RANK/RANKL interaction in mediating the osteoclas-togenic and bone resorption response resulting from particle stimulation of precursor and mature osteoclasts (Haynes et al. Citation2001, Mandelin et al. Citation2003, Wei et al. Citation2005). One study by Clohisy et al. (Citation2003) demonstrated that PMMA particles induce osteoclastogensis partly through a tumor necrosis factor-α (TNF-α)- and RANKL-dependent mechanism. In a subsequent study, the use of a blockade of transcription factor NF-κβ was found to block mature osteoclast formation due to PMMA particle stimulation of precursor osteoclasts, suggesting that this transcription factor is important in RANKL- and TNF-α-mediated osteoclastogenesis (Clohisy et al. Citation2004).
Both precursor osteoclasts and mature osteoclasts have been shown to have the capacity to phagocytose PMMA particles (Murray and Rush-ton Citation1990, Wang et al. Citation1997), and this is consistent with our observations. PMMA-mediated stimulation of precursor osteoclasts resulted in an increase in osteoclastogenesis, which is consistent with the findings of previous studies (Sabokbar Citation1996, Clohisy et al. Citation2002). The effects of addition of PMMA particles on osteoclastogenesis were restricted to the first 4 days in culture, indicating that the presence of particles did not lengthen the time that the resulting osteoclasts spent on the bone surface. In addition, no significant increase in bone resorption occurred in the particle-stimulated precursor osteoclasts compared to the control group, suggesting that these newly formed osteoclasts were not able to increase the amount of bone resorption above that of the control. In contrast, addition of PMMA particles to the mature osteoclasts resulted in an increase in the number of osteoclasts, which persisted for 8 days in culture. This increase in osteoclastogenesis was accompanied by an increase in bone resorption relative to the control and to precursor osteoclasts.
In the present work, osteoclastogenesis was stimulated in the control group in the absence of support cells, by administration of soluble RANKL to the culture medium. The addition of PMMA particles to both mature osteoclasts and precursor osteoclasts further enhanced the osteoclastogenic response above the levels seen due to RANKL administration in the control group. Ingestion of wear particles by monocytes/precursor osteoclasts has been shown to increase the expression of cyto-kines and growth factors such as RANKL, TNF-α, interleukin-6 (IL-6), and IL-1β, which can stimulate osteoclastogenesis (Blaine et al. Citation1996, Merkel et al. Citation1999, Haynes et al. Citation2001, Hirashima et al. Citation2001, Clohisy et al. Citation2003, Wei et al. Citation2005). A previous study has shown that TNF-α in particular is capable of potently enhancing RANKL-medi-ated osteoclastogenesis (O'Gradaigh et al. Citation2004). In addition, stimulatory factors such as RANKL and TNF-α have been shown to increase the survival and the bone resorption activity of mature osteoclasts (Kudo et al. Citation2002, Shiotani et al. Citation2002, Glantschnig et al. Citation2003, Wu et al. Citation2005). Different responses of the precursor osteoclasts, mature osteoclasts, and end-stage osteoclasts to these inflammatory mediators might explain our results.
Precursor osteoclasts do not have a fully developed bone resorption function after treatment with PMMA particles, and they may not be able to respond to inflammatory mediators over a short time span by increasing their bone resorption capacity. In contrast, mature osteoclasts have the capacity for bone resorption before treatment with PMMA particles—and as a result they could respond to inflammatory mediators produced in the presence of PMMA particles by increasing their bone resorption function. In addition, PMMA-stimulated mature osteoclasts remained on the bone surface longer than cells at other stages of osteoclast development, which may also have contributed to their increased bone resorption. This response to treatment with PMMA particles was limited in its time span, however, because the loss of bone resorption function and osteoclastogenic ability could not be rescued in end-stage osteoclasts. It is possible that our findings after treatment of cultures with PMMA particles may have been the result of differences in the cellular pathways that are activated in response to inflammatory mediator production that results upon exposure to the PMMA particles, and this possibility will be investigated in future studies.
In conclusion, our findings suggest that the results of stimulation with PMMA particles may be closely related to the cellular activity levels at the different stages of osteoclast development. The response to PMMA particles was the strongest in mature osteoclasts: there was an elevated number of TRAP-positive cells—which persisted until 8 days after particle addition—and increased bone resorption. In contrast, PMMA particles exposed to precursor osteoclasts alone showed a similar increase in cell number, but this only persisted for 4 days and resulted in no significant increases in bone resorption. In end-stage osteoclast cultures, the reduced numbers of cells and loss of resorption activity could not be rescued by exposure to PMMA particles.
Financial support for this project was provided by the National Institutes of Health (AR48337 (NPC)). We used the facilities of the HSS Core Center for Skeletal Integrity (NIH AR46121). This investigation was conducted in a facility that was constructed with support from the Research Facilities Improvement Program (grant no.C06-RR12538-01) from the National Center for Research Resources, National Institutes of Health.
Contributions of authors
HZ, XY, YS, NC, and MB designed the study. HZ, BR, and XY gathered and analyzed the data, and did the statistical analysis. HZ, BR, XY, NC, MB checked the accuracy of the analyses. HZ and BR wrote the manuscript.
- Amstutz H C, Campbell P, Kossovsky N, Clarke I C. Mechanism and clinical significance of wear debris-induced osteolysis. Clin Orthop 1992, 276: 7–18
- Bi Y, Van de Motter R R, Ragab A A, Goldberg V, Anderson J M, Greenfield E M. Titanium particles stimulate bone resorption by inducing differentiation of murine osteoclasts. J Bone Joint Surg (Am) 2001; 83: 501–8
- Blaine T A, Rosier R N, Puzas J E, Looney R J, Reynolds P R, Reynolds S D, et al. Increased levels of tumor necrosis factor-alpha and interleukin-6 protein and messenger RNA in human peripheral blood monocytes due to titanium particles. J Bone Joint Surg (Am) 1996; 78: 1181–92
- Boyce B F, Yamashita T, Yao Z, Zhang Q, Li F, Xing L. Roles for NF-kappaB and c-Fos in osteoclasts. J Bone Miner Metab (Suppl 23) 2005; 11–5
- Boyde A, Ali N N, Jones S J. Resorption of dentine by isolated osteoclasts in vitro. Br Dent J 1984; 156: 216–20
- Burgess T L, Qian Y, Kaufman S, Ring B D, Van G, Capparelli C, et al. The ligand for osteoprotegerin (OPGL) directly activates mature osteoclasts. J Cell Biol 1999; 145: 527–38
- Clohisy J C, Teitelbaum S, Chen S, Erdmann J M, Abu-Amer Y. Tumor necrosis factor-alpha mediates polymethylmethacrylate particle-induced NF-kappaB activation in osteoclast precursor cells. J Orthop Res 2002; 20: 174–81
- Clohisy J C, Frazier E, Hirayama T, Abu-Amer Y. RANKL is an essential cytokine mediator of poly methylmethacrylate particle-induced osteoclastogenesis. J Orthop Res 2003; 21: 202–12
- Clohisy J C, Hirayama T, Frazier E, Han S K, Abu-Amer Y. NF-kB signaling blockade abolishes implant particle-induced osteoclastogenesis. J Orthop Res 2004; 21: 202–12
- Glantschnig H, Fisher J E, Wesolowski G, Rodan G A, Reszka A A. M-CSF, TNFalpha and RANK ligand promote osteoclast survival by signaling through mTOR/S6 kinase. Cell Death Diff 2003; 10: 1165–77
- Greenfield E M, Bi Y, Ragab A A, Goldberg V M, Van de Motter R R. The role of osteoclast differentiation in aseptic loosening. J Orthop Res 2002; 1–8
- Hata K, Kukita T, Akamine A, Kukita A, Kurisu K. Trypsinized osteoclast-like multinucleated cells formed in rat bone marrow cultures efficiently form resorption lacunae on dentine. Bone 1992; 13: 139–46
- Haynes D R, Crotti T N, Potter A E, Loric M, Atkins G J, Howie D W, Findlay D M. The osteoclastogenic molecules RANKL and RANK are associated with periprosthetic osteolysis. J Bone Joint Surg (Br) 2001; 83: 902–11
- Haynes D R, Crotti T N, Zreiqat H. Regulation of osteo-clast activity in peri-implant tissues. Biomaterials 2004; 25: 4877–85
- Hirashima Y, Ishiguro N, Kondo S, Iwata H. Osteoclast induction from bone marrow cells is due to pro-inflammatory mediators from macrophages exposed to polyethylene particles: A possible mechanism of osteolysis in failed THA. J Biomed Mater Res 2001; 56: 177–83
- Hsu H, Lacey D L, Dunstan C R, Solovyev I, Colombero A, Timms E, et al. Tumor necrosis factor receptor family member RANK mediates osteoclast differentiation and activation induced by osteoprotegerin ligand. Proc Natl Acad Sci U S A 1999; 96: 3540–5
- Jiranek W A, Machado M, Jasty M, Jevsevar D, Wolfe H J, Goldring S R, et al. Production of cytokines around loosened cemented acetabular components. J Bone Joint Surg (Am) 1993; 75: 863–79
- Kobayashi A, Freeman M A R, Bonfield W, Kadoya Y, Yamac T, Al–Saffar N, et al. Number of polyethylene particles and osteolysis in total joint replacements. J Bone Joint Surg (Br) 1997; 79: 844–8
- Kudo O, Sabokbar A, Pocock A, Itonaga I, Athanasou N A. Isolation of human osteoclasts formed in vitro: hormonal effects on the bone-resorbing activity of human osteoclasts. Calcif Tissue Int 2002; 71: 539–46
- Lacey D L, Timms E, Tan H L, Kelley M J, Dunstan C R, Burgess T, et al. Osteoprotegerin ligand is a cytokine that regulates osteoclast differentiation and activation. Cell 1998; 93: 165–76
- Maloney W J, James R E, Lane Smith R. Human macrophage response to retrieved titanium alloy particles in vitro. Clin Orthop 1996, 322: 268–78
- Mandelin J, Li T F, Liljestrom M, Kroon M E, Hanemaaijer R, Santavirta S, et al. Imbalance of RANKL/RANK/OPG system in interface tissue in loosening of total hip replacement. J Bone Joint Surg (Br) 2003; 85: 1196–201
- Merkel K D, Erdmann J M, McHugh K P, Abu–Amer Y, Ross F P, Teitelbaum S L. Tumor necrosis factor-alpha mediates orthopedic implant osteolysis. Am J Pathol 1999; 154: 203–10
- Minkin C. Bone acid phosphatase: tartrate-resistant acid phosphatase as a marker of osteoclast function. Calcif Tissue Int 1982; 34: 285–90
- Murray D W, Rushton N. Macrophages stimulate bone resorption when they phagocytose particles. J Bone Joint Surg (Br) 1990; 72: 988–92
- Murrills R J, Stein L S, Fey C P, Dempster D W. The effects of parathyroid hormone (PTH) and PTH-related peptide on osteoclast resorption of bone slices in vitro: an analysis of pit size and resorption focus. Endocrinology 1990; 127: 2648–53
- O'Gradaigh D, Ireland D, Bord S, Compston J E. Joint erosion in rheumatoid arthritis: interactions between tumor necrosis factor-alpha, interleukin 1, and receptor activator of nuclear factor kB ligand regulate osteoclasts. Ann Rheum Dis 2004; 63: 354–9
- Pandey R, Quinn J, Joyner C, Murray D W, Triggitt J T, Athanasou N A. Arthroplasty implant biomaterial particle associated macrophages differentiate into lacunar bone resorbing cells. Ann Rheum Dis 1996; 55: 388–95
- Quinn J M W, Sabokbar A, Athanasou N A. Cells of the mononuclear phagocyte series differentiate into osteo-clastic lacunar bone resorbing cells. J Pathol 1996; 179: 106–11
- Sabokbar A, Fujikawa Y, Brett J, Murray D W, Athanasou N A. Increased osteoclast differentiation by PMMA particle-associated macrophages. Acta Orthop Scand 1996; 67: 593–8
- Sabokbar A, Fujikawa Y, Neale S, Murray D W, Athanasou N A. Human arthroplasty derived macrophages differentiate into osteoclastic bone resorbing cells. Ann Rhem Dis 1997; 56: 414–20
- Shiotani A, Takami M, Itoh K, Shibasaki Y, Sasaki T. Regulation of osteoclast differentiation and function by receptor activator of NF-kB ligand and osteoprotegerin. Anat Rec 2002; 268: 137–46
- Wang W, Ferguson D J P, Quinn J M W, Simpson A H R W, Athanasou N A. Osteoclasts are capable of particle phagocytosis and bone resorption. J Pathol 1997; 182: 92–8
- Wei S, Kitaura H, Zhou P, Ross F P, Teitelbaum S L. IL-1 mediates TNF-induced osteoclastogenesis. J Clin Invest 2005; 115: 282–90
- Wei X, Zhang X, Zuscik M J, Drissi M H, Schwarz E M, O'Keefe R J. Fibroblasts express RANKL and support osteoclastogenesis in a COX-2-dependent manner after stimulation with titanium particles. J Bone Miner Res 2005; 20: 1136–48
- Willert H G, Bertram H, Buchhorn G H. Osteolysis in alloar-throplasty. Clin Orthop 1990, 58: 108–21
- Wu X, Pan G, McKenna M A, Zayzafoon M, Xiong W C, McDonald J M. RANKL regulates Fas expression and Fas-mediated apoptosis in osteoclasts. J Bone Miner Res 2005; 20: 107–16