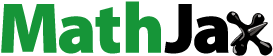
Abstract
Zinc oxide (ZnO) nanoparticles, due to their well-defined physico-chemical and antimicrobial attributes have been presented lately as favourable theranostic nanoplatforms for drug delivery. We hypothesized that surface modification of ZnO nanoparticle with activated polyethylene glycol and folic acid might be of great importance in targeted drug delivery in nasopharyngeal carcinoma since carcinoma cells possess folate receptors absent in normal cells. Therefore in this study, we have prepared and characterized activated PEG and folate conjugated dual drug loaded ZnO nanoparticles for increased cellular uptake efficiency in nasopharyngeal cancer cell lines. We synthesized the ZnO nanoparticles using sol-gel technique, conjugated them with activated PEG and folate and loaded them with two conventional anti-cancer drugs, namely cisplatin and docetaxel. The nanoparticle formulations were characterized by electron microscopy, stability studies, in-vitro release studies(acidic and normal pH), encapsulation & loading efficiency studies, FTIR and cytotoxicity studies. Additionally, cellline studies were done to assess cellular uptake efficiency using HNE-1 and CNE-2 cells. The dual drug loaded, folate & PEG activated ZnO nanoparticle were found to be extremely small size with high zeta potential & polydispersity index and high entrapment efficiency (82%) too. The nanoparticle formulations had stability and did not aggregate when kept in dispersed form for a long time. Moreover, they were found to be cytocompatible with negligible side-effects when incubated with normal cells. They also exhibited higher cellular uptake efficiencies when compared with free form. Therefore, it may safely concluded that combinatorial drug loaded, folate & PEG activated ZnO nanoparticle may result in better uptake of chemotherapeutic drugs with lower toxicity to normal cells.
1. Introduction
Nanobiomedicine with its advances has found its potential in various fields of medicine, therapeutics and theranostics [Citation1]. More recently, nanobiomedicine has yielded several novel breakthroughs in chemotherapy, hyperthermia and radiotherapy which may lead to clinical transformations too [Citation2–6]. The advent of these nanoplatforms that may lead to amalgamation of therapeutics and diagnostics may revolutionize cancer therapeutics and management [Citation7, Citation8]. Amongst the whole area of nanobiomedical applications, smart and targeted nanoparticles hold an important place for its unique physico-chemical parameters. These nanocarriers exhibit targeted delivery of drug and minor cytotoxic effects on other cells which renders them extremely useful for the cause [Citation9].
Nasopharyngeal carcinoma is one of the deadliest maladies to affect the population of east, Southeast Asia as well as north & West Africa [Citation10, Citation11]. The mortality occurs due to recurrence and quick metastasis. The treatment regime includes radiotherapy followed by cisplatin-loaded chemotherapy in case of advanced metastasis [Citation12]. Surgery is generally avoided because of its sensitive area of occurrence [Citation10, Citation13]. However, the poor survival rate of nasopharyngeal carcinoma is an indication that there is a need to develop novel treatment therapies. Moreover, the problem with radiotherapy or drug based chemotherapy lies in the cytotoxic side effects that affect the normal functioning of the system. Hence, nanotherapeutics tool loaded with drug for restricted drugs delivery to the place of occurrence of cancer may be a better option which has been clearly elucidated by many studies. The drugs when reduced to nanosized demesne have entirely different unique properties which do not reflect in their bulk size [Citation14]. One of the main reasons of the quick increase in efficacy of the nanoplatforms is reduction in cytotoxicity.
It was also observed that folate (folic acid) conjugated nanoparticles have been extensively studied as cancer conjugation moieties [Citation15]. It has been found that folate has been used as a conjugation mediator to improve the cytotoxicity of nanoparticles towards cancerous cells [Citation16]. It has also been seen that folate receptors which have an extremely high degree of affinity towards folate moieties are overexpressed in malignant cells which are not so prevalent in normal cells [Citation17, Citation18]. This overexpression of folate receptors on the cell membrane may be exploited to target the cancerous cells.
Therefore, we have prepared and characterized a novel metallic (ZnO) nanoparticle which is decorated with a combination of drugs, is folate-conjugated and PEG-functionalized. Surface alteration of the nanoparticle was performed by PEGylation to reduce the inherent toxicity of the ZnO nanoparticle so that it has minimum cytotoxic effect on the normal cells. ZnO nanoparticles are known significantly reduce the respiratory activity of nasopharyngeal epithelial carcinoma cells and also as drug delivery vehicles for anti-cancer activity [Citation19, Citation20]. The distinctive electrostatic property of ZnO nanoparticles enables surface modification by proton transfer at acidic pH (tumor microenvironment). This encourages interaction with tumor cells [Citation21]. These are also relevant for surface modifications like coating. The drugs loaded in the ZnO nanoparticles were cisplatin and docetaxel which have been applied for nasopharyngeal carcinoma treatment chemotherapy with encouraging results [Citation22]. The taxenes group of chemotherapeutic drugs have exhibited considerable anti-malignant activity in head, stem and nasopharyngeal cancers [Citation23–26]. Docetaxel was found to be less neurotoxic than paclitaxel and hence this was used for the current study. A successful combination of potent anticancer drugs when applied loaded within a nanoparticle, targeted specifically to the cancerous tissue may yield much better results than normal adjuvant chemotherapy. The primary objective of the study was to prepare folate-conjugated, PEG-functionalized drug loaded ZnO nanoparticle which would be targeted to the specific site and would elicit cellular response in curing nasopharyngeal cancer. Another objective was to reduce the cytotoxicity of the nanoparticle so that it may cause minimal damage to other surrounding tissues. Along with the preparation of the nanoparticle, complete characterization of the nanoparticle was also carried out using particle size distribution, scanning electron microscopy, transmission electron microscopy, FTIR, in-vitro release studies, cytotoxicity studies, encapsulation and loading efficiencies, stability of the nanoparticles and finally the in-vivo studies.
2. Materials and methods
2.1. Materials
Cisplatin and docetaxel were procured from Chengdu Mansite Pharmaceutical Co., Ltd. from Sichuan, China. Zincacetate dehydrate [Zn (CH3COOH)2. 2H2O], methanol, ethanol, polyethylene glycol (PEG) were procured Sigma Aldrich, China. All other chemicals used for the study were purchased from Sigma Aldrich (MO, USA) unless otherwise mentioned. Merck supplied sodium borohydride (NaBH4, 98%), citric acid, lysine, N,N′-dicyclohexylcarbodiimde (DCC), and dimethylsulphoxide (DMSO) required in the current study. Folic acid was procured from Fluka.
2.2. Cell culture
Shanghai LeiDi biotechnology Co. Ltd. located in Shanghai, China very kindly donated human nasopharynx carcinoma cells (HNE-1). RPMI medium 1640 procured from Gibco, Waltham, MA was used to culture the HNE-1 cells augmented with 10% FBS also procured from Gibco, Waltham, MA. The entire procedure was carried out at 37 °C in a humidified incubator having 5% CO2.
2.3. Preparation of the PEGylated ZnO nanoparticles (PEG-ZnO)
The procedure was adapted from [Citation27] with relevant modifications. Preparation of PEG encapsulated ZnO nanoparticles was carried out using zincacetate dehydrate [Zn(CH3COOH)2.2H2O] as the precursor in our study. First the PEG was activated by using citric acid from a reported research [Citation28]. Initially, 5 g of PEG (MW: 1500) was supplemented with 2.5 g of citrate (PEG/citrate ratio being 2:1). Thereafter, the prepared solution was heated to 110 °C for 15 min followed by 30 min incubation at130 °C. The formed compound was a high viscous liquid with the following formula, PEG:(COOH)3-PEG-(COOH)3.
Following this procedure of activation of PEG, PEG-ZnO nanoparticles were prepared subsequently by the sol–gel technique. 5.5 g of Zn(CH3COOH)2.2H2O and PEG were mixed in 350 ml of ethanol in a 10:1 (w/w) ratio of Zn: PEG respectively. Magnetic stirring of the solution was done for one day completely at 80 °C till the appearance of a transparent mixture. The hence obtained mixture was kept undisturbed till a gel was acquired from this mixture. Methanol wash was given to the gel for 3–4 times ensuring proper cleaning of the gel. After cleansing, calcination of the gel was done in a furnace at 150 °C for a time period of 2 h.
2.4. Preparation of DUAL-FOL-PEG-ZnO
Folic acid like PEG had to be activated for adhering to the nanoparticle surface. This is due to weak reactivity of carboxylic groups in PEG. The activation of the carboxyl group of folic acid was performed as referred by an earlier study with minor modifications [Citation29]. In the beginning, 5 mL of dimethyl sulphoxide (DMSO) was used for dissolving 4.5 mg of folic acid. Next, in a ratio of folic acid/DCC 1:1, DCC was supplemented to the solution and continuous stirring was carried out for 2 h within a nitrogen atmosphere. Finally, PEG-ZnOs were added with simultaneous stirring for 2 h within nitrogen atmosphere. Thereafter, modified FOL-PEG-ZnO nanoparticles (FOL-PEG-ZnO) were finally twice washed with water followed by freeze-drying.
The anticancer drug docetaxel and cisplatin (2.5 mg each) was thawed at pH 7in 2.5 mL of tris(hydroxymethyl)aminomethane buffer solution ensuring that the investigational state was in close proximity to physiological pH. 25 mg of FOL-PEG-ZnO was variegated with 2.5 mL of mixture of drugs with the concentration of the drugs being1 mg/mL−1at pH 7and continuously mixed at room temperature. The formed mixture was stirred in a rotary shaker for complete 1 day at 37 °C and freeze-dried for next 2 days to finally prepare DUAL-FOL-PEG-ZnO. The single drug loaded nanoparticles (CIS-FOL-PEG-ZnO and DOC-FOL-PEG-ZnO) were also prepared by similar procedure involving loading of single drug in the buffer solution.
2.5. Morphological analysis of DUAL-FOL-PEG-ZnO
The morphology of the ZnO nanoparticles, DOC-FOL-PEG-ZnO, CIS-FOL-PEG-ZnO and DUAL-FOL-PEG-ZnO were observed by electron microscopy, both scanning and transmission. The nanoparticles were dispersed in water and coated with gold on aluminium stub aided with double sided carbon tape following a drop method. Gold sputter coating unit was employed to coat the respective nanoparticles for 10 s at 10 Pa vacuum. Distinctive acceleration potential used was 30 kV and the image was shot at the selected magnification.
Transmission electron microscopy of the ZnO nanoparticles, DOC-FOL-PEG-ZnO, CIS-FOL-PEG-ZnO and DUAL-FOL-PEG-ZnO was further done with high resolution JEM-2010HR microscope strengthened with twin lens, power being 80 kV and the electron source being LaB6. One drop of nanoparticle was placed judiciously on a 300 mesh copper grid coated with carbon with the excess being soaked up with an absorbent paper. 1% sodium phosphotungstate solution was utilized for staining the nanoparticles and they were viewed at magnification up to 1,000,000 X. The nanoparticles were air-dried, incubated for 12 h following which the TEM images were observed. The procedure was adapted with few changes from [Citation30].
2.6. Particle size and polydispersity index (PDI)
To calculate the mean diameter and size distribution (PDI) of ZnO nanoparticles, DOC-FOL-PEG-ZnO, CIS-FOL-PEG-ZnO and DUAL-FOL-PEG-ZnO, Delsa Nano CZetasizer was utilized. The readings were taken at room temperature. Hitherto freeze-dried nanoparticles were weighed and subsequently dispersed in Milli-Q water for dilution as well as attaining an appropriate scattering intensity. Each sample was measured with readings being taken thrice. The experiment was implemented at 25˚C. The ζ potential values were deliberated as the mean of three measurements of the values of electrophoretic mobility.
2.7. FTIR measurements
FTIR spectroscopy was implemented at 37 °C to detect folic acid and zinc in the produced DOC-FOL-PEG-ZnO, CIS-FOL-PEG-ZnO and DUAL-FOL-PEG-ZnO, by means of a NICOLET 200SXV Infrared Spectrophotometer procured from Rhinelander, WI. The nanoparticles were loaded on KBr discs. At room temperature, scanning was carried outranging from 4000 to 1000 cm−1.
2.8. Determination of encapsulation efficiency (EE%) and loading efficiency (LE%) of the nanoparticles
The procedure was adapted from few studies reported [Citation31, Citation32] with changes to suit our experiments. The nanoparticle samples were centrifuged at 10,000 rpm (High Speed Centrifuge, TGL-16B, Shanghai Anting Scientific Instrument Factory, Shanghai, China) for 15 min which may lead to the separation of free cisplatin and docetaxel from nanoparticle formulations. The supernatant was strained with a 0.45-μm filter.
Investigation of free drugs in the filtered supernatant was performed with an HPLC system (Agilent, US) using the following parameters: a TCC 300 C18 column (250 mm × 4.6 mm, 5 μm), a identifying wavelength of 425 nm and a mobile phase of 7.5:7.5:85, v/v of methanol/acetonitrile/0.4% aqueous phosphoric acid and the flow rate of 1 mL/min. Measurement of the total drug loaded was carried out after the DOC-FOL-PEG-ZnO, CIS-FOL-PEG-ZnO and DUAL-FOL-PEG-ZnO were completely dissolved in ethanol. The dual and individual drug encapsulation efficiency (EE) along with loading efficiency (LE) was the derivative of the succeeding formulae:
(1)
(1)
(2)
(2)
The formulas were used to determine the EE and LE % of the various formulations of the nanoparticles.
2.9. Stability studies
The stability of DOC-FOL-PEG-ZnO, CIS-FOL-PEG-ZnO and DUAL-FOL-PEG-ZnO was tested at 37 °C.10 mg of nanoparticle formulations were dispersed by sonication in 50 ml of Milli-Q water. Visual observation of settling of nanoparticles were judiciously made followed by measurement of size distribution, polydispersity index and zeta potential of the nanoparticles at regular time interval of 1,02,030 days using the Delsa Nano C Zetasizer to note the aggregation of nanoparticles.
2.10. Kinetics of in-vitro drug release
Here in our study, we adopted a slightly different kind of approach in this particular study and were influenced by the study by Asadishad et al [Citation9]. We knew that because of the PEG coating the drug release from the nanoparticle formulations will be slow and sustained. Hence, to define the kinetics and response of drug release, the DOC-FOL-PEG-ZnO, CIS-FOL-PEG-ZnO and DUAL-FOL-PEG-ZnO was made with varying molar ratios (2:1, 1:1, and 1:2) of ZnO/PEG with activated-PEG concentrations of 0.25, 0.5 and 1 mM, correspondingly and was vacuum-packed in porous dialysis membranes. The drugs were released into 50 mL of Milli-Q water at 37 °C. The released drugs along with the medium were removed at various time intervals with immediate renewal of the media. The fraction of drugs released was directly calculated from the absorbance at 254 nm aided by a UV-Visspectrophotometer (UV-9200).The entire in-vitro release of drugs was carried out in two different pH of 4.5 and 7.4 corresponding to tumor microenvironment and normal physiological condition respectively.
2.11. In-vitro cytotoxicity
2.11.1. Cell culture
For the in-vitro cytotoxicity studies, we chose CNE-2 cells which were folate receptor negative cells. The cells were retained in RPMI1640 medium (without folic acid) with 10% fetalbovine serum (FBS) at 37 °C in an atmosphere of humidified 5% CO2. In the logarithmic growth phase, the cells incubated and seeded on a 96well plate at 5 × 104 cells/mL cell density.
2.11.2. Cytotoxicity assay
After the cells were incubated in the medium for 24 h, ZnO nanoparticles, PBS (control) free cisplatin and docetaxel were added to the cell culture at a dose of 2.5 µg/ml [Citation9]. The drug concentration of the DUAL-FOL-PEG-ZnO, DOC-FOL-PEG-ZnO, CIS-FOL-PEG-ZnO nanoparticles were adjusted to the free drug concentration which was 2.5 µg/ml. After 6 hours durations, removal of the medium was carried out followed by washing of the cells thrice with PBS. 10 µl of MTT dye (5 mg/mL) was supplemented in each well. The cultures were gestated with MTT dye for 4 h at 37 °C. For measurement of absorbance at 450 nm, a microplate reader was used. The procedure was adapted with suitable modifications from [Citation33].
IC50 is the value of concentration of each nanoformulation which inhibited 50% cell growth which was calculated with following formulae:
(3)
(3)
The measurements were done thrice.
2.11.3. Live/dead cell assay for cytotoxicity
Drug-treated cells from MTT assay were incubated for 24 h. There was a collection of all detached and adherent cells which were then for 10 min centrifuged at 1500 rpm. The supernatant was thrown away. Cells were washed thrice with normal saline (NS). At the required density of105 cells per mL the cells were removed, dyed with propidium iodide/acridine orange and viewed with fluorescent microscope.
2.12. Cellular uptake of the nanoparticles
The procedure was adapted from [Citation34] with suitable changes to suit our study. 5 × 104 cells/mL concentrations of HNE-1 and CNE-2 were harvested in 100 mm tissue culture plates. Incubation of cells was done at room temperature for 48 h with free cisplatin and docetaxel DUAL-FOL-PEG-ZnO, DOC-FOL-PEG-ZnO, CIS-FOL-PEG-ZnO nanoparticles at the IC50 concentration measured earlier. After incubation the cells washed with PBS for two times and trypsinized followed by resuspension in PBS and finally totalled. The quantities of drug accumulated in the cells were determined with an inductively coupled plasma mass spectrometer (ICP-MS, ELAN DRC-II, PerkinElmer). Concisely, treatment of the cell suspensions were carried out with 23% of nitric acid followed by 8% H2O2 and for one hour the cell suspension was wet autoclaved. The cell suspension was then introduced in the ICP-MS for quantification of drug uptaken by the cells.
3. Results and discussion
3.1. Preparation of the nanoparticles
Based on the experimental study in the preparation of ZnO nanoparticles, a chain of chemical reactions have occurred here. There was thorough hydrolysis of Zn(CH3COOH)2.2H2O in the ethanolic solution that resulted in the ZnO colloid which again was reacted with activated PEG to finally form the PEG-ZnO nanoparticle complex. Due to the heating procedure, intermediate zinc hydroxide acetate is transformed to ZnO on continuous stirring. Sodium acetate which is soluble in water was removed easily. Thus, high purity ZnO nanopowder may be obtained from sol-gel technique successfully.
DOC-FOL-PEG-ZnO, CIS-FOL-PEG-ZnO and DUAL-FOL-PEG-ZnO were also prepared successfully as would be seen in morphological analysis.
3.2. Morphological analysis of ZnO nanoparticles, DOC-FOL-PEG-ZnO, CIS-FOL-PEG-ZnO and DUAL-FOL-PEG-ZnO
TEM images were presented in . The ZnO nanoparticles exhibited in irregular symmetry whereas DOC-FOL-PEG-ZnO, CIS-FOL-PEG-ZnO and DUAL-FOL-PEG-ZnO show a hexagonal or near hexagonal symmetry. It was clearly noted that the DUAL-FOL-PEG-ZnO has a bigger size than the DOC-FOL-PEG-ZnO or CIS-FOL-PEG-ZnO. For the purpose of obtaining a better view of the nanoparticles, over 400 nanoparticles were scanned continuously. It was noted that ZnO nanoparticles did not have a clear defining edge which was clearly visible in other nanoparticles.
Figure 1. Morphological analysis of nanoparticles through TEM 1A. ZnO nanoparticles; 1B. PEG encapsulated ZnO nanoparticles; 1C. DOC-FOL-PEG-ZnO nanoparticles; 1D. CIS-FOL-PEG-ZnO; 1E. DUAL-FOL-PEG-ZnO nanoparticles.

The SEM images in pointed towards an interesting observation. The occurrence of big sizes of ZnO nanoparticles in may be due to the amassing or coinciding of smaller nanoparticles. However, small sized monodispersed nanoparticles evident in correspond to PEG encapsulated ZnO nanoparticles. Therefore, it may be inferred that the addition of activated PEG led to significant changes in the shape, structure, physical properties of the ZnO nanoparticles. However, addition of individual or dual drug caused no significant difference in the structure or morphology of the nanoparticles other than increased size.
Figure 2. SEM Images of 2A. ZnO nanoparticles; 2B. PEG encapsulated ZnO nanoparticles; 2C. DOC-FOL-PEG-ZnO nanoparticles; 2D. CIS-FOL-PEG-ZnO; 2E. DUAL-FOL-PEG-ZnO nanoparticles.

The sizes of the nanoparticles were depicted in along with the zeta potential and PDI. The size of the DUAL-FOL-PEG-ZnO was the largest with the mean diameter and S.D. ranging from 75.45 ± 1.5 nm. The sizes of DOC-FOL-PEG-ZnO and CIS-FOL-PEG-ZnO are in the range of 61.23 ± 1.2 nm and 62.13 ± 1.6 nm respectively. The size of the ZnO nanoparticles ranged from 42.34 ± 2.2 nm. The increase in the size of nanoparticles upon drug loading was a clear indication of the successful drug trapping within the nanoparticles. The zeta potential values indicated a major force in developing the stability or dispersion of the nanoparticles. It was evident from the observed values that zeta potential of ZnO nanoparticles was much lower than others which caused a change in the dispersion of the nanoparticles. The point that ZnO nanoparticles have neutral hydroxyl groups chemisorbed on their surface lends it a positive value. However the drug loaded nanoparticles have higher positive values because of the basicity of drugs which imparts a positive charge to the nanoparticles. However the PEG-ZnO has lower positive charge which may be due to the coating of PEG over the nanoparticle. The agglomeration of ZnO observed in the SEM and the uniformly dispersed PEG-ZnO supported the zeta potential & PDI readings.
Table 1. Size distribution, zeta potential and PDI of the nanoparticle formulations.
3.3. FTIR measurement
represented the typical FTIR spectrum of ZnO, PEG-ZnO, DOC-FOL-PEG-ZnO, CIS-FOL-PEG-ZnO and DUAL-FOL-PEG-ZnO. Conjugation of PEG to ZnO and folate to PEG-ZnO was confirmed by the FTIR spectra. Absorption band for C–O–C ether stretching vibration was seen at 1192 cm−1of recurrent –OCH2CH2 components of PEG block. Asymmetric stretching vibration of -NH2 in folate corresponds to the band at 1459 cm−1 and C = O stretching in carboxyl acids was seen at 1700 cm−1. Combination of hydroxyl (OH) stretching results in absorption bands at 3423 cm−1.
3.4. Encapsulation efficiency (EE %) and loading efficiency (LE %) of the nanoparticles
The encapsulation and the loading efficiencies are noted in . The encapsulation efficiencies of DOC-FOL-PEG-ZnO, CIS-FOL-PEG-ZnO and DUAL-FOL-PEG-ZnO were 78.25%±0.41, 80.15% ± 1.21 and 84.25%± 2.12 respectively. There wasn’t any noteworthy difference between the encapsulation of drug(s) in the three formulations and the high EE % was perhaps due to the coating of PEG over the nanoparticles. The coating probably prevented any leaching of drugs from the nanoparticles. The drug loading efficiency of DOC-FOL-PEG-ZnO, CIS-FOL-PEG-ZnO and DUAL-FOL-PEG-ZnO was 43.5%, 41.45% and 47.14% respectively. From the studies, it was evident that there was essentially no noteworthy difference between the encapsulation and loading efficiencies of DOC-FOL-PEG-ZnO, CIS-FOL-PEG-ZnO and DUAL-FOL-PEG-ZnO. The high percentages of EE and LE indicated towards a stable formulation.
Table 2. Encapsulation efficiency (EE %) and loading efficiency (LE %) of the nanoparticle formulation.
3.5. Stability studies
For inspection of the stability of the DOC-FOL-PEG-ZnO, CIS-FOL-PEG-ZnO and DUAL-FOL-PEG-ZnO, measurements of differences in size, PDI, zeta potential was carried out at regular time intervals of 10, 20 and 30 days of storage at room temperature. The shelf life or the stability of DOC-FOL-PEG-ZnO, CIS-FOL-PEG-ZnO and DUAL-FOL-PEG-ZnO was observed through this study. The values obtained are depicted in . There is a minor decrease in size of the ZnO & PEG-ZnO nanoparticles and slight increase in sizes of DOC-FOL-PEG-ZnO, CIS-FOL-PEG-ZnO and DUAL-FOL-PEG-ZnO. This was also followed by decrease in PDI and zeta potential values. This may accounted by the fact that during storage, the activated PEG on the surface may have arranged itself neatly and densely, thereby reducing the size and zeta potential in ZnO and PEG-ZnO nanoparticles. The increased size may have been a result of PEG and folate rearranging themselves on the surface. The exact reason of reduced zeta potential values is still unclear but since the zeta potential values remain positive, we assumed that there is no change in the stability of the nanoparticle formulations. From the results in the table it is clear that there is no noteworthy change in the sizes, PDI and zeta potential values of the DOC-FOL-PEG-ZnO, CIS-FOL-PEG-ZnO and DUAL-FOL-PEG-ZnO and the formulations are thermodynamically most stable. Any possibility of anti-microbial effect on the nanoparticle formulations may be negated since it has been reported in previous researches that nanoparticles of ZnO exhibit great anti-microbial properties than their microscale counterparts [Citation35].
Table 3A. Size, zeta potential and PDI of DOC-FOL-PEG-ZnO after 10, 20 & 30 days during stability studies.
Table 3B. Size, zeta potential and PDI of CIS-FOL-PEG-ZnO after 10, 20 & 30 days during stability studies.
Table 3C. Size, zeta potential and PDI of DUAL-FOL-PEG-ZnO after 10, 20 & 30 days during stability studies.
3.6. Kinetics of in-vitro drug release
The in-vitro drug release behaviour of the nanoparticle formulations are depicted in . The pattern of in-vitro drug release was unclear in DUAL-FOL-PEG-ZnO, DOC-FOL-PEG-ZnO and CIS-FOL-PEG-ZnO formulations. As anticipated, in pH 7.4 () there wasn’t a noteworthy difference in the in-vitro release of drug from the nanoparticle formulations prepared. It was seen that about 30% of drug was released from the nanoparticle in the first 15 h in case of DUAL-FOL-PEG-ZnO and in case of DOC-FOL-PEG-ZnO and CIS-FOL-PEG-ZnO; the % of drug released was 40% and 35% respectively in case of the molar ratio of PEG: ZnO (2:1, w/w) and concentration of activated PEG was 0.5 mM. This clearly reflected that the drugs were embedded in the nanoparticle and not adsorbed on the surface along with PEG forming a protective layer. The surface adsorption of the drugs leads to a burst release of drug which may lead to frequent renewal of drugs which is the drawback in maximum cases of drug delivery systems. In contrast in the current formulation, the DOC-FOL-PEG-ZnO, CIS-FOL-PEG-ZnO and DUAL-FOL-PEG-ZnO showed a much slower cumulative release. Approximately, in 92 h about 95% of the drug was released from the nanoparticle formulation. In case of other molar ratios of PEG: ZnO (1:2, 1:1, w/w) and concentration of PEG was 0.25 mM, there was initial burst release of approximate 45% of drug within 5 h. With the concentration of activated PEG being 1 mM, there was a minimum release of drug of 10% within the 24 h which led us to believe that probably the excess of activated PEG may be hampering the release of the embedded drug. The reason however is quite unclear and needs to be studied. We drew some conclusions from this study that PEG: ZnO 2:1 was the best ratio to form a drug carrier vehicle; there will be a rapid release (to a certain extent) of drug in the initial stage which will drop to slow and sustained release; too much concentration of PEG may hamper the release of drug from the nanoparticle.
Figure 4. 4A in-vitro cumulative drug releases in DUAL-FOL-PEG-ZnO, DOC-FOL-PEG-ZnO and CIS-FOL-PEG-ZnO formulations in pH 7.4 (normal physiological milieu); 4B in-vitro cumulative drug releases in DUAL-FOL-PEG-ZnO, DOC-FOL-PEG-ZnO and CIS-FOL-PEG-ZnO formulations in pH 4.5 (tumor microenvironment).

represents in-vitro release of drug from the nanoparticle formulations prepared at pH 4.5. Interestingly, it was seen that there was a general increase in release of drugs in acidic medium when the molar ratio of PEG: ZnO was 2:1, w/w. however, there were no significant differences in releases of drugs in case of other molar ratios of PEG: ZnO (1:2, 1:1, w/w). This reinstates the fact that PEG: ZnO 2:1 was the best ratio to form a nanocarrier. Therefore, this ability to achieve higher drug release at acidic pH that mimics the tumor microenvironment was favourable for future therapeutic applications.
3.7. Cytotoxicity studies
To evaluate the in-vitro cytotoxicity of the nanoparticle formulations, we chose CNE-2 cell line since it does not express folate receptors [Citation36]. Since we specially designed the nanoparticles formulations with folic acid to target the carcinoma cells which explicitly expressed folate receptors, our aim was to see its cytotoxic effects on normal/cancerous cells which do not express folate receptors. Hence CNE-2 cells were our chosen in-vitro model for cytotoxicity. The IC50 values were determined for the nanoparticles were listed in . The MTT assay determined the cytotoxicity of the PBS (control), free cisplatin, docetaxel, ZnO nanoparticles, DOC-FOL-PEG-ZnO, CIS-FOL-PEG-ZnO and DUAL-FOL-PEG-ZnO which revealed that the nanoformulations are cytocompatible for normal cells even after they have been exposed to them for a longer time period. The dose of formulation chosen for the exposure of cells was high (2.5 µg/ml) but they were cytocompatible comparable to the PBS. In fact, it was noted that free cisplatin and docetaxel were quite cytotoxic ( and ) followed by the ZnO nanoparticles. The protective coating of activated PEG was probably the reason of cytocompatibility of the cells along with the fact the drugs were embedded in the core of the nanoparticles as seen during the in-vitro release assay. Therefore activated PEG coated ZnO nanoparticle may be safely utilized as dual drug carrier too. The live/dead cells assay also reinforced the study. It can be clearly seen in that when treated with free cisplatin and docetaxel there was a significant decrease in cell viabilities. There was negligible decrease in cell viabilities of nanoparticle formulations when compared with control. Acridine orange stains all cells, whether dead or alive and propidium iodide stains all live cells green.
Figure 5. Cytotoxicity of the PBS (control), free cisplatin, docetaxel, DOC-FOL-PEG-ZnO, CIS-FOL-PEG-ZnO and DUAL-FOL-PEG-ZnO.

Figure 6. Cytotoxicity against CNE-2 cells of 6A. PBS (control); 6B. Free cisplatin; 6C.Free docetaxel; 6D. ZnO nanoparticles; 6E. DOC-FOL-PEG-ZnO; 6F. CIS-FOL-PEG-ZnO; 6G. DUAL-FOL-PEG-ZnO.

Table 4. IC50 values free cisplatin, docetaxel, DOC-FOL-PEG-ZnO, CIS-FOL-PEG-ZnO and DUAL-FOL-PEG-ZnO.
3.8. Cellular uptake studies
To understand the cellular uptake efficiency of the nanoparticle formulation, folate positive (HNE-1) cells and folate receptor negative (CNE-2) cells were used in the study. demonstrates the efficiency of cellular uptake of free cisplatin, docetaxel, DOC-FOL-PEG-ZnO, CIS-FOL-PEG-ZnO and DUAL-FOL-PEG-ZnO. The free drug concentration was adjusted with the concentration of the drug in the nanoparticle which was 2.5 µg/ml. It was seen that cellular uptake of free cisplatin and docetaxel were less than that of nanoparticle formulations in both cell lines. The cellular uptake of the nanoparticle formulations were calculated in ng/mol. It was seen that there was an accelerated cellular uptake of DOC-FOL-PEG-ZnO, CIS-FOL-PEG-ZnO and DUAL-FOL-PEG-ZnO by the HNE-1 uptake when compared to free docetaxel and cisplatin. However, the CNE-2 uptake of free cisplatin, docetaxel and nanoformulations was less than that of HNE-1.This may be attributed to the presence of folate receptors in the HNE-1 cells which easily conjugated with the folate in the nanoparticles. This was a perfect example of selected drug delivery to the targeted tissue. The increased accumulation of drugs in the HNE-1 cells may increase the therapeutic efficiency by resulting in cell death which may be caused by DNA damage. The CNE-2 uptake efficiency of free cisplatin & docetaxel was less when compared with DOC-FOL-PEG-ZnO, CIS-FOL-PEG-ZnO and DUAL-FOL-PEG-ZnO respectively. The process of increased cellular uptake in case of free drugs and nanoparticle formulation may be due to the small size which advances the process of endocytosis. This aids in the internalization of nanoparticles easily within the cells which improves the cellular uptake efficiency. However, it is not easy to generalize the process of internalization of nanoparticles by cells and the mechanism is still not very well known [Citation37]. But it is understood that nanoparticle formulations having a size < 100 nm are internalized via endocytosis. The surface charge on the nanoparticles also plays an important role in internalization. Nanoparticle formulations having a positive charge were known to be internalized rapidly.
4. Discussion and conclusion
Recent studies have focused on few areas of carcinoma nanotechnology research which includes loading the nanoparticles with anti-cancer drug and then targeting it to the cancer cells [Citation2]. This mechanism of therapy has been specially endowed with folate to improve the targeting to specific cancer cells. Therefore in this study, we have specifically used the combinatorial drug to be loaded in the nanoparticle for synergistic therapeutic effects against nasopharyngeal carcinoma. This may provide an added thrust in the various targeted drug delivery nanoplatforms that are continuously being researched nowadays. We have synthesized and characterized a novel dual drug loaded, folate conjugated, PEG activated ZnO nanoparticle which may be extremely useful in nasopharyngeal carcinoma therapeutics. Zinc oxide nanoparticle has been proven to be promising theranostic aid for anticancer therapy [Citation38, Citation39]. The preparation of the ZnO particle was being carried out via sol-gel therapy followed by conjugation with activated PEG and folic acid to specially target cancerous cells since they possess the folate receptors. The characterization of the DUAL-FOL-PEG-ZnO indicated that they were of size big enough not being cleared out of the system and small enough to escape the gastrointestinal mechanism. The hypothesis behind the loading of dual drug was to increase the cytotoxic potential of the drug by synergism between the drugs along with the ZnO nanoparticle. In this study, it was particularly important to note that the coating or conjugation with PEG had a significant effect on the morphology of the ZnO nanoparticle. The aggregates of ZnO nanoparticles were duly organized in monodispersed and uniform nanoparticles upon addition of PEG. An optimal concentration of PEG with the ZnO nanoparticle also resulted in slow and sustained released of drugs over a period of approximately 92 h as duly observed in the drug release kinetics both in acidic and normal pH which mimicked the normal physiology and tumor microenvironment respectively. The increased percentage of EE and LE also indicated the formation of a stable nanoformulation. The coating of PEG also ensued in drug being embedded in the core of nanoparticle and not adsorbed on the surface. The conjugation with folate led to targeted drug delivery and cytocompatibility with normal cells. The anti-cancer activity of the DUAL-FOL-PEG-ZnO was also enhanced when compared with DOC-FOL-PEG-ZnO and CIS-FOL-PEG-ZnO proving our hypothesis correct. The study reflects that the DUAL-FOL-PEG-ZnO selectively targets and internalizes the cancer cells but the mechanism to do so was still unclear and has to be looked into.
Therefore it may be concluded that DUAL-FOL-PEG-ZnO may be a highly effective and novel therapy for nasopharyngeal carcinoma with targeted chemotherapeutics dual drug delivery to the cancer cells and minimal effects on other normal cells. This reduction in side-effects might have a huge effect in moving towards the right direction in cancer theranostics. However, in-vivo studies on animals need to be done before the transfer of the therapy may be carried out from bench to trials. The in-vivo studies may help us understand the mechanism of DUAL-FOL-PEG-ZnO better and identify/rectify the side effects, if any. This combination of nanotechnology and biomedicine promises of significant change in the development of anti-cancer therapeutics. Nevertheless, there may be lots of challenges which have to be addressed in impending studies.
Disclosure statement
No potential conflict of interest was reported by the author(s).
References
- Akbarzadeh F, Khoshgard K, Hosseinzadeh L, et al. Investigating the cytotoxicity of folate-conjugated bismuth oxide nanoparticles on KB and A549 cell lines. Adv Pharm Bull. 2018;8(4):627–635.
- Beik J, Khademi S, Attaran N, et al. A nanotechnology-based strategy to increase the efficiency of cancer diagnosis and therapy: folate-conjugated gold nanoparticles. Curr Med Chem. 2017;24(39):4399–4416.
- Sanna V, Pala N, Sechi M. Targeted therapy using nanotechnology: focus on cancer. Int J Nanomed. 2014;9:467–483.
- Beik J, Abed Z, Ghoreishi FS, et al. Nanotechnology in hyperthermia cancer therapy: From fundamental principles to advanced applications. J Control Release. 2016;235:205–221.
- Her S, Jaffray DA, Allen C. Gold nanoparticles for applications in cancer radiotherapy: Mechanisms and recent advancements. Adv Drug Deliv Rev. 2017;109:84–101.
- Beik J, Abed Z, Ghadimi-Daresajini A, et al. Measurements of nanoparticle-enhanced heating from 1MHz ultrasound in solution and in mice bearing CT26 colon tumors. J Therm Biol. 2016;62(Pt A):84–89.
- Shakeri-Zadeh A, Kamrava SK, Farhadi M, et al. A scientific paradigm for targeted nanophotothermolysis; the potential for nanosurgery of cancer. Lasers Med Sci. 2014;29(2):847–853.
- Shakeri-Zadeh A, Khoee S, Shiran M-B, et al. Synergistic effects of magnetic drug targeting using a newly developed nanocapsule and tumor irradiation by ultrasound on CT26 tumors in BALB/c mice. J Mater Chem B. 2015;3(9):1879–1887.
- Asadishad B, Vossoughi M, Alemzadeh I. Folate-receptor-targeted delivery of doxorubicin using polyethylene glycol-functionalized gold nanoparticles. Ind Eng Chem Res. 2010;49(4):1958–1963.
- Chua ML, Wee JT, Hui EP, et al. Nasopharyngeal carcinoma. The Lancet. 2016;387(10022):1012–1024.
- Wei K-R, Zheng R-S, Zhang S-W, et al. Nasopharyngeal carcinoma incidence and mortality in China, 2013. Chin J Cancer. 2017;36(1):90.
- Chen Y, Xu G, Zheng Y, et al. Nanoformulation of D-α-tocopheryl polyethylene glycol 1000 succinate-b-poly(ε-caprolactone-ran-glycolide) diblock copolymer for siRNA targeting HIF-1α for nasopharyngeal carcinoma therapy. Int J Nanomed. 2015;10:1375–1386.
- Feng GK, Zhang MQ, Wang HX, et al. Identification of an Integrin α6‐targeted peptide for nasopharyngeal carcinoma‐specific nanotherapeutics. Adv Therap. 2019;2(7):1900018.
- Abrahamse H, Kruger CA, Kadanyo S, et al. Nanoparticles for advanced photodynamic therapy of cancer. Photomed Laser Surg. 2017;35(11):581–588.
- Samadian H, Hosseini-Nami S, Kamrava SK, Ghaznavi H, et al. Folate-conjugated gold nanoparticle as a new nanoplatform for targeted cancer therapy. J Cancer Res Clin Oncol. 2016;142(11):2217–2229.
- Khoshgard K, Hashemi B, Arbabi A, et al. Radiosensitization effect of folate-conjugated gold nanoparticles on HeLa cancer cells under orthovoltage superficial radiotherapy techniques. Phys Med Biol. 2014;59(9):2249–2263.
- Zhang H, Li J, Hu Y, et al. Folic acid-targeted iron oxide nanoparticles as contrast agents for magnetic resonance imaging of human ovarian cancer. J Ovarian Res. 2016;9(1):19.
- Majd MH, Barar J, Asgari D, et al. Targeted fluoromagnetic nanoparticles for imaging of breast cancer mcf-7 cells. Adv Pharm Bull. 2013;3(1):189.
- Prasanth R, Gopinath D. Effect of ZnO nanoparticles on nasopharyngeal cancer cells viability and respiration. Appl Phys Lett. 2013;102(11):113702.
- Sharma H, Kumar K, Choudhary C, et al. Development and characterization of metal oxide nanoparticles for the delivery of anticancer drug. Artif Cells Nanomed Biotechnol. 2016;44(2):672–679.
- Shi D. Cancer cell surface negative charges: A bio-physical manifestation of the Warburg effect. Nano Life. 2017; 07(03n04):1771001.
- Hui EP, Ma BB, Leung SF, et al. Randomized phase II trial of concurrent cisplatin-radiotherapy with or without neoadjuvant docetaxel and cisplatin in advanced nasopharyngeal carcinoma. J Clin Oncol. 2009;27(2):242–249.
- Forastiere AA, Shank D, Neuberg D, et al. Final report of a phase II evaluation of paclitaxel in patients with advanced squamous cell carcinoma of the head and neck: an Eastern Cooperative Oncology Group trial (PA390). Cancer: Interdiscip Int J Am Cancer Soc. 1998;82(11):2270–2274.
- Au E, Tan E, Ang P. Activity of paclitaxel by three-hour infusion in Asian patients with metastatic undifferentiated nasopharyngeal cancer. Ann Oncol. 1998;9(3):327–329.
- Dreyfuss AI, Clark JR, Norris CM, et al. Docetaxel: an active drug for squamous cell carcinoma of the head and neck. J Clin Oncol. 1996;14(5):1672–1678.
- Catimel G, Verweij J, Mattijssen V, et al. Docetaxel (Taxotere): an active drug for the treatment of patients with advanced squamous cell carcinoma of the head and neck. Ann Oncol. 1994;5(6):533–537.
- Tshabalala M, Dejene B, Swart H. Synthesis and characterization of ZnO nanoparticles using polyethylene glycol (PEG). Phys B. 2012;407(10):1668–1671.
- Namazi H, Adeli M. Dendrimers of citric acid and poly (ethylene glycol) as the new drug-delivery agents. Biomaterials. 2005;26(10):1175–1183.
- Zhang J, Srivastava R, Misra R. Core-shell magnetite nanoparticles surface encapsulated with smart stimuli-responsive polymer: synthesis, characterization, and LCST of viable drug-targeting delivery system. Langmuir. 2007;23(11):6342–6351.
- Malamatari M, Somavarapu S, Bloxham M, et al. Nanoparticle agglomerates of indomethacin: The role of poloxamers and matrix former on their dissolution and aerosolisation efficiency. Int J Pharm. 2015;495(1):516–526.
- Zhang T, Chen Y, Ge Y, et al. Inhalation treatment of primary lung cancer using liposomal curcumin dry powder inhalers. Acta Pharm Sin B. 2018;8(3):440–448.
- Wei Y, Liang J, Zheng X, et al. Lung-targeting drug delivery system of baicalin-loaded nanoliposomes: development, biodistribution in rabbits, and pharmacodynamics in nude mice bearing orthotopic human lung cancer. Int J Nanomed. 2017;12:251–261.
- Yoo HS, Park TG. Folate-receptor-targeted delivery of doxorubicin nano-aggregates stabilized by doxorubicin-PEG-folate conjugate. J Control Release. 2004;100(2):247–256.
- Tippayamontri T, Kotb R, Paquette B, et al. Cellular uptake and cytoplasm / DNA distribution of cisplatin and oxaliplatin and their liposomal formulation in human colorectal cancer cell HCT116. Invest New Drugs. 2011; 29(6):1321–1327.
- Yamamoto O. Influence of particle size on the antibacterial activity of zinc oxide. Int J Inorg Mater. 2001;3(7):643–646.
- Xie M, Zhang H, Xu Y, et al. Expression of folate receptors in nasopharyngeal and laryngeal carcinoma and folate receptor-mediated endocytosis by molecular targeted nanomedicine. Int J Nanomed. 2013;8:2443–2451.
- Panariti A, Miserocchi G, Rivolta I. The effect of nanoparticle uptake on cellular behavior: disrupting or enabling functions? Nanotechnol Sci Appl. 2012;5:87–100.
- Lee J-H, Kim Y-G, Cho MH, et al. ZnO nanoparticles inhibit Pseudomonas aeruginosa biofilm formation and virulence factor production. Microbiol Res. 2014;169(12):888–896.
- Seker S, Elçin AE, Yumak T, et al. In vitro cytotoxicity of hydrothermally synthesized ZnO nanoparticles on human periodontal ligament fibroblast and mouse dermal fibroblast cells. Toxicol in Vitro. 2014;28(8):1349–1358.