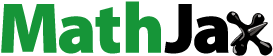
ABSTRACT
Introduction: Aqueous humor and its dynamics (AHD) serve numerous functions to sustain ocular health and clear vision. Current methods to assess AHD have led to improved understanding of ocular physiology, pathologies caused by abnormal intraocular pressures (IOP), and treatments to return IOP to healthy levels. However, the assumptions and limitations inherent in these methods have been problematic. New methods on the horizon are showing promise to greatly advance the field.
Areas covered: This review summarizes AHD assessment techniques in use today including the noninvasive methods of fluorophotometry, tonometry, tonography, venomanometry, and invasive methods of cannulation, infusion, dissection. It also mentions emerging methods utilizing advanced imaging methods, smart phones, cameras, and software.
Expert opinion: Soon new techniques will become available to measure each parameter and even subparts of each parameter of AHD with increased speed, accuracy, and safety. Along with these new methods and the data they generate could emerge the next version of the Goldmann Equation. These new methods would make AHD an important tool in the clinic for precision medicine and in the lab for exploring AHD on a new level.
1. Introduction
Aqueous humor dynamics (AHD) has been investigated for well over a century and continues to be of interest today. This interest persists because it is the AHD that maintains the intraocular pressure (IOP) and when the various parameters of AHD drift outside a narrow range, pathological changes occur in the IOP that may cause hypotony at the one extreme or glaucoma at the other. AHD is characterized by the rate of aqueous production, its circulation mainly into the anterior chamber and its drainage through tissues with inherent resistance. Pathways for inflow and outflow are illustrated in and outlined in . The four main parameters of AHD and how they interrelate are described by the modified Goldmann equation,
Figure 1. Aqueous humor dynamics

Figure 2. Outline of aqueous humor production and drainage

These parameters include the production rate of aqueous humor (Fp), often assessed by measuring the flow rate of fluid from the posterior to the anterior chamber (Fa); uveoscleral outflow rate (Fu), episcleral venous pressure (EVP) and facility in all outflow pathways (C). C is usually considered to be the facility in the trabecular outflow pathway (Ctrab) but can include other factors depending on how it is measured. This equation is a simplified description of the true dynamics of ocular aqueous humor. Important components are missing because they were thought to be constants or were unknown at the time the equation was written. Each of the known parameters in the equation can be divided into unique subparts () as will be described later.
Table 1. Parameters of AHD divided into component parts
Most methods to measure the parameters of AHD were developed decades ago, with only minor improvements added since. An understanding of these methods and their limitations remains vital because it ensures proper interpretation of decades of published data. Better methods, especially noninvasive ones, have been slow to evolve. Now, however, new technologies are emerging that should greatly improve the methods, and enable exploration of AHD in new directions, at a faster pace, and with greater accuracy. This review summarizes methods to measure AHD that are currently in use today () and describes some new methods that are appearing on the horizon. An argument to revise the Goldmann Equation is offered.
Table 2. Current methods to measure IOP and the parameters of aqueous humor dynamics
2. Intraocular pressure
The IOP and its assessment is of great interest in the clinical setting and research lab because IOP is the only clinically modifiable risk factor for glaucoma, a blinding optic neuropathy affecting millions of people worldwide. Accurate assessment of IOP is crucial for the diagnosis and treatment of the disease and for the development of improved therapies. Two methods are available to evaluate IOP, tonometry and manometry. That there are numerous tonometers available for clinical and research use illustrates the unmet need for an instrument that can rise to the Gold Standard with regard to precision, repeatability, reliability, and ease of use in eyes of all sizes and biomechanical properties. All tonometers in their various designs are based on different principles and assumptions and measure one or more non-IOP parameters. Depending on how IOP is measured, corneal properties including rigidity and thickness could affect IOP measurements to some degree. Different tonometers may show changes in IOP, not only from true IOP differences but also from changes in biomechanical properties of the cornea as a result of a treatment such a topical prostaglandin analogue or refractive surgery. Since some tonometers are affected more by cornea properties than others, the various tonometers cannot be used interchangeable. Common tonometers used in humans () are applanation tonometers (Goldmann, pneumatonometer and noncontact tonometers), rebound tonometers (iCare), and a non-applanation contact tonometer (Pascal dynamic contour tonometer). Some of these instruments have been modified for use in animals. Options for research animals are rebound tonometers (TonoLab® for mice and rats and TonoVet® for larger research animals), and MacKay-Marg tonometers (Tono-Pen VET® and the Tono-Pen AVIA VET®). Further discussion of each tonometer is beyond the scope of the current review. We direct the reader to several of many good comprehensive reviews on tonometry [Citation1–3].
Figure 3. Tonometers in use today

Manometry is an invasive method to measure actual IOP. Details of the pros and cons of manometry in laboratory animals can be found in a recent review [Citation4]. Manometry also is used to measure episcleral venous pressure in the mouse [Citation5] which is described later. Briefly, a needle or cannula attached via small diameter tubing to a pre-calibrated pressure transducer, is inserted into the eye. The system is filled with a balanced/buffered saline, cell culture medium or mock aqueous humor, the pressure transducer detects the IOP and the readout is sent to a computer to be converted into units of pressure (). Important design considerations include needle placement, anesthesia, timing, and body position. Firstly, careful placement of the needle/cannula is crucial. Slightly different pressures (mmHg) can be obtained depending on where in the eye the needle/cannula is positioned. This is because a pressure gradient exists from the anterior chamber to Schlemm’s canal and episcleral veins [Citation6] or from the anterior chamber to the supraciliary and suprachoroidal spaces [Citation7]. If these pressure gradients are of interest, then this is the method to use. It should be noted that these pressure gradients are tiny requiring a very sensitive pressure transducer to detect them. Needle touch to surrounding tissues should be avoided to prevent needle clogging and to minimize an inflammatory reaction. Needle puncture of the cornea alone can promote breakdown of the blood-aqueous barrier (especially in rabbits). This has the potential to cause inflammation and secretion of plasmoid aqueous. Even when done carefully, manometry has the potential to change the very parameter that is being measured and results can be suspect.
Figure 4. Invasive methods to measure IOP and outflow facility

Anesthesia is another design consideration when planning an experiment with manometry. Systemic anesthesia is essential to block pain and prevent eye and head movements during the measurement. However, type of anesthetic, and timing of measurement post injection can give different IOPs. Some anesthetics have a rapid blunting effect on IOP in the mouse requiring measurements to be made within a few minutes of administration [Citation8–12]. Other anesthetics may exacerbate rather than still eye movements [Citation13].
The time of day of the measurement is an important design consideration in manometry or tonometry studies. IOP fluctuates throughout the day and night and differs among species of animal. Nocturnal animals such as rabbits and mice have higher IOP at night than day [Citation14–16] whereas diurnal animals have higher IOP during the day. A caveat is that IOP changes as the head changes relative to the heart. IOP is higher when lying than when sitting or standing. Body position should be consistent throughout a study [Citation17–19] especially if repeat measurements are needed.
2.1. Future ideas
Telemetry is very likely to become the IOP measurement technique of the future in research animals. It provides continuous monitoring of IOP in the undisturbed animal once all instrumentation is properly placed. A few animal research labs have developed telemetry methods and sensors [Citation20–24], but these are not readily available to the broader research community. The first of these animal IOP telemetry papers was published more than two decades ago. Probe placement, damage to tissues, high failure rate, unpredictable signal duration, equipment failure, and expense, are just a few of the challenges faced by investigators. All that aside, if sensor design and placement are successful, megabytes of data can be collected continuously for months in the undisturbed animal. This is the only way to get a true assessment of IOP. The struggles of a few pioneers will eventually benefit the many scientists of AHD in the future.
Continuous IOP monitoring methods are being developed for humans in the form of contact lens sensors [Citation25–31] and implantable intraocular sensors [Citation32–34] (). The Triggerfish (Sensimed, Lausanne Switzerland) is CE marked and FDA approved for continuous monitoring of spontaneous changes in the eye, but it does not measure true IOP. The ARGOS-IO telemetric IOP sensor (Implandata Ophthalmic Products GmbH, Hanover, Germany) has undergone some human trials and provides 24-h IOP readings [Citation35,Citation36]. Information from continuous IOP monitoring is particularly useful in subjects with glaucoma in which nocturnal IOPs are needed [Citation34] or in patients having undergone Boston Keratoprosthesis Surgery and routine tonometry is no long possible [Citation33]. Problems with IOP sensors yet to be resolved are reliability, comfort to the wearer, and data not reported in units of pressure [Citation37]. A literature search in PubMed on 28 October 2020 using the keywords, ‘human AND ((intraocular IOP sensor) OR (contact lens IOP sensor)) NOT (review)’, yielded 90 papers. Clearly, this technology is evolving faster than animal telemetry. Interest in continuous, undisturbed IOP measurement remains strong because its value in research, diagnostics and treatment options remains great.
Figure 5. Examples of intraocular pressure sensors

3. Aqueous production
The circulation of aqueous humor in the eye () begins with its production by the ciliary processes and secretion into the posterior chamber at a steady rate (Fp, aqueous production). From there it flows into the anterior chamber (Fa, aqueous flow) or vitreous cavity (Fpost posterior flow) (). Posterior flow cannot be measured noninvasively and, out of necessity, is usually ignored or assumed to be unchanged by any study manipulation. It is not currently described in the Goldmann Equation. Aqueous flow varies among animal species with the general trend of faster rates in larger eyes. The techniques to measure aqueous flow in use today include the noninvasive method of anterior chamber fluorophotometry, and two invasive methods of dye dilution, and constant flow infusion.
3.1. Fluorophotometry
The predominant method to study aqueous flow is anterior chamber fluorophotometry. A scanning ocular fluorophotometer [Citation38] () or a slit-lamp fluorophotometer [Citation39] are used. The former is commercially available (Fluorotron Master, Ocumetrics, Palo Alto, CA), but the latter is not. The technique is used in humans, monkeys, dogs, cats, and rabbits. Fluorophotometry also can be performed in the tiny eye of mice by using a mouse fluorophotometer (Mouse Fluorotron, Ocumetrics, Palo Alto, CA, ) [Citation40]. It has a specialized eyepiece lens to magnify the anterior chamber and produce a focal diamond that fits inside the cornea. Anesthesia is needed to keep the animal still for the scans, but it can recover and be studied again at a later date.
Figure 6. Fluorophotometry

Anterior chamber fluorophotometry is a noninvasive, repeatable, and relatively precise measurement of aqueous flow. The tracer is easily detectable and is assumed to travel by bulk flow at the same rate and in the same direction as aqueous humor. Tracer movement is not identical to the flow of aqueous humor, but it is a good estimate. It has a long history of use by many labs which enables comparisons among studies. Investigations in healthy humans find similar results and consistent conclusions among the various studies (). The first step in the procedure is to deliver a tracer into the anterior chamber. The most common tracer is fluorescein which can be injected directly into the anterior chamber or applied to the cornea by either topical eye drops or iontophoresis. The injection of fluorescein is rarely done anymore but it did simplify data analysis because no fluorescein stained the cornea to complicate the calculations. In the anterior chamber the tracer is diluted and flushed through the anterior chamber angle by the bulk flow of aqueous humor. The amount of fluorescein in the cornea and anterior chamber at any given time is measured by the fluorophotometer [Citation38,Citation41]. Measurements are repeated at set intervals (usually 45–60 minutes for eyes similar in size to humans and 10–15 minutes for small eyes of rodents). Each measurement finds less fluorescence as the fluorescein is being diluted and flushed through the chamber angle. Depending on the animal model used, one to 3 h of fluorescein monitoring is needed to measure the disappearance rate of fluorescein, which is a measure of aqueous flow rate. The faster the fluorescence signal decays, the faster the flow and conversely, the slower the decay rate, the slower the flow. Many key scientists were involved in the development of fluorophotometry but the main contributors were Michael Yablonski [Citation42], Richard Brubaker [Citation39], David Maurice [Citation43,Citation44] and Maurice Langham [Citation45Citation46].
Table 3. Aqueous flow by fluorophotometry in healthy human eyes
Any secreted aqueous humor that does not enter the anterior chamber is not measured by anterior chamber fluorophotometry. Recall that some aqueous humor does flow posteriorly through the vitreous cavity (posterior flow; ), thus, aqueous flow is less than aqueous production. Any experimental manipulation that might increase posterior flow would divert some aqueous humor from the anterior chamber which would slow the disappearance rate of anterior chamber fluorescein. This would be interpreted erroneously as slowed aqueous humor production rate.
The fluorophotometry method with fluorescein as a tracer requires a clear cornea and anterior chamber, and an intact barrier between the anterior chamber and vitreous cavity. Light from the fluorophotometer becomes scattered when traveling through a cloudy cornea or aqueous humor filled with flare and cells such as in anterior uveitis. Such conditions reduce the accuracy of the readings. The method also does not work well in aphakic eyes or in pseudophakic eyes with ruptured lens capsules because the intraocular compartments become mixed, and some fluorescein flows into the vitreous cavity instead of the anterior chamber angle. Results may be calculated erroneously as an abnormally fast aqueous flow rate.
3.2. Invasive methods
Invasive methods to measure aqueous flow are done mainly in mice. These methods include the dye dilution technique [Citation50] and the constant flow infusion technique [Citation51]. For the dye dilution technique, a dye is placed into the anterior chamber and its mass is measured over time. One microneedle connected to an aspiration system and a second microneedle connected to an infusion system are inserted into the anterior chamber. A dye (usually a fluorescent tracer) is infused into the anterior chamber at a set rate through the infusion needle and aqueous humor is removed at the same rate through the aspiration needle. The perfusion pressure is set at episcleral venous pressure, which in the mouse is approximately 9 mmHg. This pressure at which the outflow through the trabecular meshwork is zero. Over time, the fluid infused into the eye is diluted by secreted aqueous humor. The aspiration tubing filled with the diluted tracer is cut into segments of a certain length containing a set volume. The fluorescence of the fluid aspirated from the tubing is measured with a spectrofluorometer. The magnitude of tracer dilution over time is a measure of aqueous flow [Citation50].
A similar dye dilution technique was designed for use in bovine eyes [Citation52]. The purpose was to study the mechanisms of action of drugs on IOP and aqueous flow, without the effects of the cardiovascular and central nervous systems, and without the need to sacrifice animals.
The constant flow infusion technique [Citation51] determines IOP, episcleral venous pressure and outflow facility separately in mice (described in more detail later) and deduces aqueous flow and uveoscleral outflow from the Goldmann equation. This is an indirect assessment of aqueous flow.
3.3. Future ideas and needs
In the near future, imaging techniques such as ultrasound biomicroscopy (UBM) [Citation53], ocular coherence tomography (OCT) [Citation54,Citation55], or magnetic resonance imaging (MRI) [Citation56] could be used to record the rate and pattern of movement of a tracer that had been delivered to the posterior chamber. The feasibility of this idea is supported by a recent paper using dynamic contrast enhanced MRI [Citation57] in mice given intravenous injections of a contract agent. With this method, differences were found in fluid movement with regard to age and pigmentary glaucoma. In the future, this method could evolve into one that measures the rate of entry of a tracer from the ciliary processes into the posterior chamber (aqueous production, Fp), the rate of entry of tracer into the anterior chamber (aqueous flow, Fa) and rate of entry of tracer into the vitreous body (posterior flow, Fpost). Stimulating Fpost may become a new means to lower IOP or to deliver drugs to the retina.
Improvements in imaging techniques are even now showing great promise in the quantitation of aqueous humor outflow. Lipid emulsion-based OCTA in porcine eyes can provide time-resolved 3D data of aqueous humor outflow [Citation58]. Time-of-flight magnetic resonance tomography [Citation59] allows for noninvasive imaging of fluid flow and may prove useful for functional assessment of outflow pathways. Fluorescein canalograms in regionally discrete outflow tissue can visually show differences in flow such as in whole globes vs anterior segment preparations [Citation60]. New techniques to accurately measure aqueous flow rates are needed not only to measure flow in subjects with clear corneas and lenses, but also in patients who have undergone cataract surgery or who have uveitic glaucomas which have a compromised blood aqueous barrier. Such techniques will enhance our understanding of the pathophysiology of the disease and allow us to customize treatments to individual patients.
4. Trabecular outflow and facility
The trabecular outflow pathway () is exquisitely designed to drain aqueous humor at a slow rate while maintaining an IOP that is sufficient to preserve the shape of the globe without harm to the intraocular structures and optic nerve. The rate of fluid drainage across the trabecular pathway is dependent upon several factors, including the outflow pressure (difference between the IOP and episcleral venous pressure), the rate of flow through the uveoscleral pathway and the facility of trabecular outflow. Because pathology in the trabecular pathway is the primary reason for the elevated IOP in some glaucomas, the tissues of the trabecular outflow pathway are under intense investigation by many research labs. From these efforts we have learned that the trabecular meshwork is not a passive filter as was once thought. In fact, it is a very complex tissue with multiple functions. The trabecular meshwork has a cytoskeleton that makes up its collagen framework that allows its cells to change shape and motility as its environment changes. Trabecular meshwork cells are capable of phagocytosis and autophagy, two very important functions to keep the outflow pathway clean and its cells healthy. These cells secrete extracellular matrix which expresses various cytokines and proteins. The cytoskeleton is connected to the nuclear membrane enabling it to signal the nucleus quickly to alter the expression of genes as it confronts biomechanical challenges [Citation61,Citation62].
Imaging methods, including aqueous angiography and global imaging, have become valuable tools in understanding aqueous humor outflow. These methods cannot measure flow rates, but they can map fluid outflow through Schlemm’s canal and distal pathways. This offers functional, although not quantitative, information on drainage patterns. From these methods we have learned that nasal regions have higher flow than temporal regions [Citation63,Citation64] and that this outflow is not merely segmental but can be dynamic in nature [Citation65,Citation66]. Interestingly, high flow regions can change to low flow regions by some as yet unknown process. The purported purpose is to maintain relatively stable IOP during environmental stresses on the globe. Regulation of these dynamic changes could be at the level of the trabecular meshwork with adjustments in resistance, and/or at the level of the distal outflow pathway with adjustments in tone of drainage vessels [Citation65]. Global imaging methods have found that bypassing some of the TM with a Schlemm’s canal stent leads to increased tracer movement in the vicinity of the stent [Citation63,Citation67].
Key to achieving the optimal IOP is the intrinsic resistance in tissues of the outflow pathways. The reciprocal of the resistance is aqueous humor outflow facility, or conductance (C). Outflow facility in healthy humans range from 0.19 to 0.29 µl/min/mmHg (). Numerous ways to measure C have been developed over the years, all of which determine a ratio of a change in flow (F) to a corresponding change in pressure (P). C represents different facilities depending on how it is measured.
Table 4. Outflow facility in healthy human eyes
The ∆F may be measured directly (by fluorophotometry or invasive methods described above) or obtained from standardized tables that were developed decades ago [Citation68,Citation69]. The ∆P is measured by tonometry or manometry. Techniques for measuring C were established more than 60 years ago and the basic principles are still applied today. Despite decades of investigation, understanding C remains murky and more questions are raised than answered. Until new techniques are developed, these old techniques will continue to be used with all their assumptions and limitations. The measurement techniques include the noninvasive methods of tonography and fluorophotometry, and invasive methods of multilevel constant pressure perfusion and multilevel constant flow perfusion.
4.1. Tonography
A noninvasive measurement technique used in humans and nonhuman primates to assess outflow facility is tonography (). It is the recording of changes in IOP from sustained pressure on the globe. Pressures can be applied to the cornea by a weighted probe or to the limbal region by a suction cup. For the former, an electronic Schiotz tonometer or a pneumatonometer with tonography setting (Reichert, Depew, NY) are the instruments of choice. The Schiotz tonometer was developed in the 1950s and used for decades but is no longer commercially available. It has been replaced by the pneumatonometer. A comparison of the Schiotz and pneumatonometer for outflow facility assessment finds comparable results [Citation70]. Tonography is explained by a series of equations that were described in the 1930s and refined during the next four decades. Jonas Friedenwald, Morton Grant, and Maurice Langham were the main contributors to the development of this technique [Citation68,Citation71–73]. Tonography was originally designed to be a diagnostic tool for glaucoma and it was used in clinical practice throughout the 1960s and 1970s. However, it is rarely used today for diagnostic purposes because of its failure to reliably identify cases of glaucoma. Nevertheless, tonography continues to be of value in the carefully controlled research environment when done by experienced technicians. Differences in C among and between experimental groups can be detected provided the number of subjects and the differences in C are large enough.
Figure 7. Tonography

The advantages of Schiotz or pneumatonography over other methods of measuring outflow facility are that they are quick (2–4 minutes per eye), and noninvasive. The procedure starts by placing a weighted tonometer probe on the anesthetized cornea of the supine subject for 2 or 4 minutes. Animals need to be anesthetized for this test. The 4-min test may be challenging for some subjects and the results are usually no better than the 2-min test in our hands. The weight of the probe on the cornea causes the IOP to rise initially, but, over time, IOP slowly declines. This drop is assumed to be caused solely by increased drainage of aqueous humor through the trabecular outflow pathway. The volume of fluid lost from the eye during the test (∆F) is determined from reference tables [Citation68,Citation69,Citation71–73]. The outflow facility is the ratio of the flow change (from the reference tables) to the change in pressure (from tonometry) during the test (EquationEquation 2(2)
(2) ). If the IOP decreases little during the test, calculated outflow facility would be low which is found in eyes with ocular hypertension. If IOP decreases significantly, outflow facility would be high which is found after some drugs, surgical procedures, or laser treatments.
Tonography also can be performed by a suction cup (oculopressure tonometry) technique developed in the late 1950s through the 1960s [Citation74–77]. After topical anesthesia, a plastic funnel-shaped cup, with specific diameter, flange size and curvature, is placed on the eye with the flange over the limbal region. Cup designs also have been developed for use in rabbits [Citation78]. The cup is held in place by suction created with a simple android device connected to the cup by a plastic tube. A negative pressure of 30–50 mmHg is applied. IOP is measured by tonometry before placement and immediately after removal of the cup. IOP measurements are repeated at 3 to 5-min intervals until the pressure returns to baseline (usually from 10 to 15 minutes). The facility of outflow is calculated from the pressure decay curve and formulas described elsewhere [Citation77]. The main disadvantages of the method are that the patient must remain still for a relatively long time (10 or more minutes), the suction cup often falls off too soon and hyperemia can occur. An advantage of the method is that it can be done in patients with nystagmus, poor central fixation, or corneal defects that prevent the placement of a weighted probe on the cornea. The technique also is used to raise pressure for measuring retinal and ciliary arterial blood pressures [Citation79] or studying the regulation of choroidal blood flow [Citation80–82].
Outflow facility by tonography (Cton) is assumed to be trabecular outflow facility (Ctrab) but it also includes pseudofacility (Cps) and uveoscleral outflow facility (Cfu).
Ctrab is the primary parameter of interest not only because it is low in ocular hypertension with or without glaucoma [Citation83] but also because it represents the greatest proportion of total facility throughout the entire aqueous humor drainage system. Its increase is a means to lower IOP and treat glaucoma. Ctrab itself can be divided into two parts, starting with the facility in the trabecular meshwork and Schlemm’s canal endothelium (Cts), followed by facility in the downstream tissues (Cd). Evidence for Cd is demonstrated when some facility remains after the trabecular meshwork and Schlemm’s canal endothelium are physically removed. Some evidence suggests that as much as 50% of outflow resistance may be located downstream to Schlemm’s canal [Citation84,Citation85]. Other evidence suggests that most if not all the resistance is found between the trabecular meshwork and Schlemm’s canal [Citation86,Citation87]. These divergent findings might be related to the amount of trabecular meshwork removed. If a small amount, as in the early studies, aqueous humor flows through the site of trabeculectomy and the remaining TM is under perfused causing it to fill with ‘debris’ which increases resistance. If all the TM is removed, then the debris flushes downstream into the collector channels where resistance increases (facility decreases). Whether Cd is small or large, it is part of Ctrab which can be written as
Cfu is the facility of outflow through the uveoscleral outflow pathway. It is approximately 10% of total facility in nonhuman primates [Citation88–90]. These studies measured uveoscleral outflow in eyes perfused with a tracer at normal IOP and at 5 to 10 mmHg above normal. The tracer was infused for a set period of time (approximately 30 minutes) followed by eye dissection and quantitation of the tracer in the tissues of the uveoscleral pathway. In simple terms,
Measurement of Cfu requires a large number of animals and, each animal can be used only once. Until a noninvasive method is developed, Cfu cannot be measured in humans. It is usually considered unchanged during an experimental manipulation, although this assumption is not true at low IOPs [Citation88].
Cps is the facility of the inflow of aqueous humor from the posterior chamber into the anterior chamber. The increased pressure applied to the cornea during tonography, slows this inflow. Cps has been determined in humans by measuring IOP, Ctot and EVP at baseline and when IOP is raised by a sphygmomanometer cuff placed around the neck and inflated to 30 mmHg. Using the formula
Cps was calculated to be approximately 20% of total outflow facility (Ctot) by a tonographic method [Citation91] and 18% to 27% of Ctot by a suction cup method [Citation92]. An important but not entirely accurate assumption in tonography is that the rate of aqueous humor’s inflow into the anterior chamber during the measurement remains unchanged by the applied pressure. If Cps or Cfu were to change during the time of the tonography procedure, a change in Cton would occur. This is usually interpreted as a change in Ctrab which would be erroneous under these circumstances.
Ocular rigidity is another important factor affecting the tonography measurement. This is a measure of the resistance that the eye exerts to distending forces. The amount of applied pressure needed to indent the cornea a specific amount is related to but does not equal IOP. Cornea properties, including cornea thickness and rigidity, can affect the IOP measurement. Stiffer and older eyes have greater ocular rigidity than younger eyes [Citation93–96], and more force is required to indent the cornea. Tonographic outflow facility may be lower in older individuals on the basis of increased ocular rigidity rather than on a true age-related decrease in outflow facility. Tonography performed with Schiotz indentation tonometry assumes that the change in pressure during the test is based on the accuracy of the ocular rigidity coefficient during the same test. Indentation tonography makes no compensation for individual variations in ocular rigidity. Tonography assessed with a pneumatic tonography unit is less affected by ocular rigidity than the Schiotz unit, because the probe that is placed on the eye creates a relatively smaller corneal indentation. All the factors discussed above require careful consideration when interpreting tonography data.
4.2. Fluorophotometry
Instead of using standardized tables to determine ∆F in Equationequation 2(2)
(2) , a fluorophotometric technique [Citation97] was developed which measures aqueous flow by fluorophotometry at two different IOPs. The IOP is changed by giving subjects a drug that slows aqueous flow, such as acetazolamide. C by fluorophotometry is often labeled Cfl to distinguish it from C by tonography (Cton) as the two methods are not synonymous. Ocular rigidity and pseudofacility are not part of Cfl because a weighted probe is not applied to the eye. Occasionally, different results and different conclusions may be obtained depending on the method used. An age-related decrease in outflow facility was reported when measured by tonography [Citation94,Citation98] but not by fluorophotometry [Citation99]. This discrepancy was thought to be related to increased rigidity with aging.
A few problems are associated with the fluorophotometric method that should be taken into consideration when designing studies or interpreting results. First, it is assumed that uveoscleral outflow facility (Cfu) is very small and affected little by the experimental manipulation. Second, the aqueous flow suppressant must cause a substantial decrease in IOP by reducing aqueous flow alone, leaving the other parameters in Equationequation 2(2)
(2) unchanged. The method does not work well in subjects with low baseline IOPs (someone possibly assigned to a drug treatment group). The method also fails if there is no additivity of the aqueous flow suppressant to a study drug. Third, fluorophotometry requires several hours to complete, which makes it impractical for diagnostic purposes.
4.3. Invasive Methods
Outflow facility is a physical property of the tissues of the outflow pathways that is independent of metabolic activity. Unlike aqueous flow that decreases to zero immediately after death, outflow facility in enucleated eyes is measurable for days. As with noninvasive techniques to measure C, invasive methods utilize Equationequation 2(2)
(2) . The relationship between pressure and flow is linear in eyes of live animals [Citation100,Citation101] and non-linear in enucleated eyes at least in mice [Citation102]. Application of a linear fit to a non-linear flow–pressure relationship, introduces error in the estimation of outflow facility. This should be taken into consideration when designing an experiment and interpreting results.
Two similar invasive techniques to assess outflow facility are used in human donor eyes and in eyes of anesthetized or deceased animals. The multi-level, constant-pressure perfusion technique [Citation103] () was originally designed and used in monkeys nearly 60 years ago and continues to be used today. With adjustments for the size of the eye and its chambers, the method can be used in many animal models. Mice are often studied because of their rapid development, large litter sizes, and potential for genetic manipulation. A needle, attached via tubing to a reservoir of a buffered or balanced saline solution or mock aqueous humor, is inserted into either the anterior chamber or posterior chamber. Perfusion of fluid into the anterior chamber causes posterior bowing of the iris, and traction on the scleral spur, which may increase C. Perfusion into the posterior chamber does not have such an effect, but placement of the needle here is technically challenging, especially in the tiny mouse eye. Other design considerations are whether the eyes are alive or dead, the amount of time post mortem and the temperature of the eyes during the measurement [Citation101,Citation104]. Once the needle is placed with no harm to surrounding tissues, the IOP is set by the level of the reservoir above the eye. The rate of fluid flow into the anterior chamber that is needed to maintain a constant IOP during a set time interval is measured. Next, the flow rate needed to maintain a new IOP is measured in a similar manner. The ratio of the change in flow to corresponding change in pressure (EquationEquation 2(2)
(2) ) is used to calculate C. A graph is plotted of the mean pressure (mmHg) at each flow rate versus flow rate (μL/min). A linear regression line is drawn through the data and the slope of this line is the resistance. Outflow facility is simply the reciprocal of this slope.
A similar method to measure outflow facility (constant flow method) is one in which the eye is infused with mock aqueous humor at a constant flow (instead of pressure), and the pressure (instead of flow) that is maintained at that rate is recorded. The flow is set at multiple rates and the corresponding pressures are recorded. The slope of the plotted regression line is the inverse of C. Timing of measurement post anesthesia induction does not change C [Citation12], nor does state of the eye, whether alive or dead [Citation51]. Improvements to these methods include electronic data storage, sophisticated statistical software, and techniques to hold flows or pressures at preprogrammed levels while detecting small changes in pressures or flows. iPerfusion [Citation102] has been developed recently with the aim of reducing operator error and subjectivity, improving precision, and eliminating the burden of constant monitoring. The iPerfusion technique works well in dead mouse eyes.
4.3.1. The flow-to-blood method
The flow-to-blood method [Citation105,Citation106] is the most precise technique to measure true trabecular outflow facility, but it is also the most technically challenging. A radioactive isotope such as iodinated albumin, is infused into the anterior chamber for a set time and at a set pressure. The radioactivity detected in blood samples from a femoral artery collected (every 5 min) at a specified time interval is considered to have drained solely through the trabecular meshwork. During this time, tracer does enter the tissues of the uveoscleral pathway, but it is assumed that little reaches the blood. Flow of aqueous humor to the systemic blood is considered to be the volume of labeled aqueous humor required to have deposited the amount of tracer found in the blood samples divided by the duration of the tracer infusion [Citation107]. To calculate trabecular outflow facility, the flow to blood is determined at different
times (30 min intervals) and infusion pressures (usually 15 and 25 mmHg). EquationEquation 2(2)
(2) is used to calculate trabecular outflow facility. This method can only be used in a live animal with
circulating blood.
4.4. Future ideas and needs
Ocular characteristics can vary with race, genetic background, age, sex, and probably other factors yet unknown. Future measurements of tonography will require consideration of these factors. Imaging techniques could help in this regard. A work in progress is a new tonography/imaging protocol (Toris, unpublished data presented at the 2019 ARVO annual meeting) that includes imaging of the anterior segment via UBM before and immediately after two-minute tonography. Cornea thickness, cornea diameter and anterior chamber depth are measured in the two images. These biometric parameters are used to calculate the anterior chamber volume in each image. Two minutes of pressure on the cornea from the tonography probe reduces the anterior chamber volume as fluid drains through the angle under the increased pressure. The difference in anterior chamber volumes between the two images taken 2 minutes apart gives the change in outflow rate for that individual (∆F). IOP is measured immediately before placement of the weighted probe and immediately after its removal. The change in IOP is ∆P. EquationEquation 2(2)
(2) is used to calculate C. Since flow and pressure changes are collected on an individual and C is calculated solely for that individual, accuracy of tonography data should improve. A technique of this design could become available soon since the pneumatonographer and UBM imaging system are already commercially available. With continued software development and automation of the method, tonography may again become a key tool for diagnostic and research purposes.
5. Episcleral venous pressure
The episcleral veins are the drainage vessels for the aqueous humor that traversed the trabecular meshwork [Citation108]. The pressure within the episcleral veins (EVP) has a direct effect on IOP. One can see from the Goldmann equation [Citation109] that a 1 mmHg rise in EVP corresponds to a 1 mmHg rise in IOP, provided all other parameters are unchanged.
Clinically, EVP is elevated in a variety of conditions associated with increased venous pressure in the orbit and in the rest of the body. Orbital diseases such as thyroid associated ophthalmopathy, amyloidosis and retrobulbar tumors can cause congestion of the orbital veins and subsequent rise in EVP. Large vessel venous obstruction (superior vena cava syndrome), cavernous sinus thrombosis, and carotid cavernous sinus fistulas can cause an increase in superior ophthalmic vein pressure and a rise in EVP [Citation110,Citation111]. Sturge Weber Syndrome in older children and young adults, with port wine stains (hemangiomas) on the face near the eye can include intrascleral or episcleral anastomoses that increase episcleral venous pressure [Citation112] which in turn elevates IOP [Citation113,Citation114]. Glaucoma often follows. Elevated EVP can be idiopathic and occur without apparent underlying disease [Citation115]. Patients may present with proptosis, conjunctival hyperemia, chemosis, orbital bruit or raised IOP. Indicative of raised EVP are dilated, tortuous episcleral veins and gonioscopy revealing an open angle with blood in the Schlemm’s canal. Accurate assessment of EVP would help in diagnosis, treatment and understanding of these ocular pathologies.
5.1. Noninvasive methods
The noninvasive techniques to assess EVP are based on the principle that EVP is the force required to physically compress the episcleral veins. Historical methods used a Torsion balance [Citation116] and an Air jet [Citation117] to compress the vein with mean values in healthy humans of 14.3 and 17.5 mmHg, respectively. The pressure chamber method () is the method in use today. It has found EVPs in healthy humans to be in the range of 6 to 10 mmHg (). The pressure chamber method uses an episcleral venomanometer [Citation118] consisting of a flexible transparent tip made of silicone with a 3 mm diameter indicator ring on it marking the area that should be in contact with the eye. This tip is connected to an air chamber, with volume and pressure controlled by an air sealed piston threaded to a rotating dial. The rotating dial is calibrated in millimeters of mercury from 0 to 32. The venomanometer can be mounted directly on a slit-lamp microscope and is commercially available (Episcleral Venomanometer, Model EV-310, EyeTech Ltd, Boca Raton FL). The membrane touches an episcleral vein approximately 3 mm from the limbus. The dial is gradually rotated to increase the pressure in the chamber. The point where the vessel just collapses is assumed to be the EVP. This method is quick and repeatable, but it does have a few challenges. One is identifying an appropriate vein. Episcleral veins are straighter, less mobile, and deeper than the conjunctival vessels and not as deep as intrascleral veins. Another challenge is identifying the most appropriate endpoint. Various endpoints have been defined including slight indentation [Citation119,Citation120], half collapse [Citation118], and sustained collapse [Citation120]. When the three endpoints were compared [Citation120], the pressure corresponding to the slightest change in vessel diameter correlated the best with the pressure obtained from invasive methods. The determination of the precise endpoint at which the vessels collapse is very subjective and can lead to variable results. Pressing the membrane to the sclera beyond the indicator ring when the dial reads 0 could also lead to erroneous results. The tactile sense of how much the dial is being turned could account for a measurement bias. Different observers may select different vessels or endpoints leading to operator variability. All these limitations stress the need for a noninvasive and more objective method of measuring EVP.
Figure 8. Pressure chamber method to measure episcleral venous pressure

Table 5. Episcleral venous pressure in healthy human eyes
A video enhanced EVP measurement method [Citation119] () is a modification of the commercially available venomanometer in which a computer controlled motor drive is added to the venomanometer. This modification automatically increases the pressure and simultaneously records a video of the vein collapsing. The pressure associated with each video frame is stamped on the image. Each image is meticulously examined by hand for change in vessel diameter and brightness relative to baseline. This method is more objective than the others and allows for precise measurement at specific endpoints resulting in detection of small changes in EVP. The method is evolving to increase automation and improve image quality. This modified venomanometer is not commercially available.
5.2. Invasive methods
Two invasive methods to measure EVP involve cannulating the episcleral veins to get a direct measurement of EVP or cannulating the anterior chamber to change the IOP to get an indirect measurement of EVP. Cannulating the episcleral vein is technically challenging because of the small diameter (50–100 microns) and low fluid volume (less than 4.3 mm/sec) of the episcleral veins [Citation122]. Once cannulated, the EVP is measured by a pressure transducer. The measurement can be affected by the direction in which cannula is placed relative to the flow. EVP is likely overestimated if the cannula is oriented against the flow [Citation123]. An even more sensitive method is a servo-null micropipette system utilizing a tiny micropipette tip with an internal diameter of 2–4 microns to cannulate the episcleral veins [Citation6,Citation124]. The micropipette is filled with a fluid of a different electrical resistance than serum which is attached to a piezoelectric valve connected to an air pressure source. A difference between the pressure in the pipette and the pressure in the vein causes the fluid to move either into or out of the pipette. The valve pressure is adjusted accordingly until the fluid returns to its null position. This endpoint is considered equivalent to the pressure within the vein.
An anterior chamber cannulation method [Citation5] is used to measure EVP in mice. A 35-gauge needle is placed in the anterior chamber connected to a fluid-filled reservoir to adjust the IOP and a pressure transducer. The fluid is a mock aqueous humor or buffered/balanced saline. The EVP is the pressure in the anterior chamber when a reflux of erythrocytes from the episcleral vein into the Schlemm’s canal is observed under an operating microscope. This method is difficult to use in heavily pigmented mice, in which the reflux is difficult or impossible to observe. If the reflux can be seen, the pressure in which the reflux appears is somewhat subjective.
These cannulation methods are technically challenging, invasive in ways that the procedures themselves could alter the EVP, and cannot be done in humans. Nevertheless, they have provided valuable information on EVP in research animals, and they have been used to validate noninvasive techniques.
5.3. Future ideas and needs
EVP measurement designs of the future will require increased precision, ease of use and easily identifiable endpoints (the point of compression of the episcleral vein that gives true EVP). New ideas are emerging to improve the design of an objective noninvasive venomanometer. Adding portability and flexibility to the current design will offer new avenues for research and diagnostics. A portable venomanometer (), that is a modified design of the commercially available unit, is being developed at the University of Nebraska Medical Center [Citation125]. The commercially available venomanometer is housed on a 3D printed stand with a smartphone case holder and an electrical component housing. A Google Pixel 3 smartphone is placed in the phone case with its camera aligned directly with the venomanometer’s viewing pane and 0.36x super wide-angle lens stacked with a 20x macro lens are attached to the phone case to further enhance the magnification capability in visualizing the episcleral veins. The pressure dial on the venomanometer is read using a potentiometer attached to a microcontroller and the reading is overlaid on the camera image. A smartphone application is being developed on top of existing open-source code for Android image and video capture to fulfill the simultaneous implementation of the smartphone as a user interface, viewing device, camera, and data logger. The video recording is saved for further analysis. A floor mounted tripod stand with adjustable dolly enables adjustments of the venomanometer assembly in the x, y and z direction, enabling measurements of the patient in numerous body positions. The episcleral vein is observed through the smartphone and the pressure dial on the venomanometer is turned until the vein half-collapses, at which time the pressure is read off the phone screen. To develop a holistic device for objective EVP measurement, further research is needed to combine portability, precision in measurement, and speed of data collection and analysis.
The need to improve measurement methods is mounting because reasons to know true EVP are growing. We mention just a few here. 1. An instrument able to detect small changes may show that EVP varies depending upon the location of an episcleral vein in relation to the limbus and quadrant of the eye. Collector channels are more concentrated nasally [Citation126] and patterns of flow through the trabecular meshwork vary with location [Citation64]. These findings may have a bearing on the trabecular outflow and EVP values in different quadrants. This could help to explain why there is segmental flow through the trabecular meshwork. 2. Since EVP is neurally regulated [Citation127], more studies on neural control of EVP would enhance our knowledge of EVP and its role in aqueous humor dynamics. 3. Recent evidence suggests that EVP could be a biomarker for intracranial pressure (ICP). High ICP is a critical prognostic marker for severe traumatic brain injury (TBI) [Citation128,Citation129]. Early recognition and management of high ICP is critical to reduce morbidity and mortality. Current methods to measure ICP are invasive and require cannulation of the cerebrospinal fluid space or placing of intra-parenchymal brain probes. An increase in ICP can cause an increase in IOP which is hypothesized to be due mainly to an increase in EVP [Citation130]. EVP may be a better biomarker for ICP as compared to IOP because of smaller population variability [Citation118], and smaller diurnal variability [Citation131]. In addition, EVP is relatively less affected by systemic medications given to lower ICP (like acetazolamide) [Citation132]. The value of EVP for diagnostic purposes is apparent. A portable venomanometer would be a very helpful tool to predict ICP in emergency and ICU settings.
All of the noninvasive methods yet developed or under development to measure EVP require a clear view of an episcleral vein. The method does not work if the view is obstructed by disease, scarring or heavily pigmented conjunctiva (as in cynomolgus macaques). The problem might be circumvented in the future by using auricular (ultrasound) rather than optical cues to detect blood flow changes while an episcleral vein is being compressed.
6. Uveoscleral outflow
Uveoscleral outflow is the flow rate, in µl/min, of aqueous humor out of the anterior chamber angle in many different directions other than through the trabecular meshwork. Unlike trabecular drainage that follows a linear path through tubes and channels, flow through the uvea is unconventional in that it seeps into the ciliary muscle and then through or around the supraciliary space, suprachoroidal space, choroid, sclera, emmisarial canals, choroidal blood vessels and lymphatic vessels (). Uveoscleral outflow rate changes little at IOPs in the normal to high range and for this reason, uveoscleral outflow is considered pressure independent. Uveoscleral outflow is difficult to measure making accurate assessment a challenge. It has been studied by mathematical calculation or by monitoring tracer movement. In healthy humans, uveoscleral outflow is approximately 1.5 µl/min (). It decreases with aging, a conclusion supported by studies in healthy humans [Citation99], monkeys [Citation133] and mice [Citation134]. Slowing of uveoscleral outflow contributes to the increased IOP in patients with ocular hypertension [Citation135] and exfoliation syndrome with or without high IOP [Citation136].
Table 6. Uveoscleral outflow in healthy human eyes
6.1. Mathematical Calculation
Currently, the only noninvasive means to assess uveoscleral outflow (Fu) is via mathematical calculation using a rearrangement of Equationequation 1(1)
(1) .
In simple terms, IOP, Fa, C and EVP are measured and what remains to balance the equation is Fu. This is a very indirect assessment that yields data with large standard deviations from the inherent variability of each of the other parameters in the equation. Many subjects/animals per group are needed to achieve sufficient power to detect significant differences between groups or between baseline and treatments. This method cannot accurately measure true Fu on an individual level, but it can detect changes in Fu provided the changes and the sample size are big enough. Some important findings resulting from use of this method are that Fu is decreased in exfoliation syndrome [Citation136], decreased with aging [Citation99], reduced at night [Citation17] and increased with prostaglandin analogs [Citation137].
6.2. Invasive Methods
The ‘intracameral tracer method’ () involves infusing a fluorescent tracer into the anterior chamber at a set pressure for a set time. Radioactive isotopes also have been used as a tracer, but they are rarely used today. The infusion time should be long enough to fill the anterior uvea with tracer but not so long as to lose measurable tracer into the vasculature. At a set time (approximately 30 minutes), the infusion is stopped, the animal is euthanized, and the eyes enucleated. The cornea, vitreous and anterior chamber contents are discarded, and the other tissues are diluted, homogenized and centrifuged. The fluorescence in the supernatant is measured. The volume of tracer in the supernatant (taking into account the dilution factor) and the infusion time are used to calculate Fu in µl/min. The need to euthanize animals makes the method non-repeatable and costly.
Figure 9. Uveoscleral outflow by an invasive method

The ‘dead eye perfusion method’ [Citation51] involves measuring fluid inflow at set pressures in the dead eye, when EVP and aqueous production are zero. Outflow facility is determined by the multilevel constant pressure perfusion method described above. Estimates of Fu at each flow rate are derived from the relationship: Fu = Flow Rate-Mean Pressure at that Flow Rate x C. The value of Fu at each flow rate is determined. The reported Fu is the average value from each flow rate.
6.3. Future ideas and needs
Ever since uveoscleral outflow was first described, there has been an unfulfilled need for a rapid, precise, direct, noninvasive method to measure Fu. Finally, possibilities to meet this need may be forthcoming. Nontoxic opaque tracers delivered to the anterior chamber noninvasively, clear images of the tracer in the ciliary muscle, fast computers with large storage capacity, and software to automate the system, may be the needed tools. One idea is to acquire serial MRI images of the uvea over time after delivery of a known amount of tracer, such as iron oxide/dextran nanoparticles, into the anterior chamber. Intensity of tracer signal in serial images of the ciliary muscle, time between each image, accurate calculation of uveal volume, and the decay rate of the tracer from the uvea could be used to calculate uveoscleral outflow. Such an approach would not depend on first measuring the other parameters of AHD in the Goldmann Equation.
It may become scientifically advantageous to distinguish, and examine separately, the different routes of uveoscleral egress (). Aqueous humor that enters the ciliary muscle can exit across the sclera all around the globe (Fs) [Citation138], drain into the lymphatic vessels (Fl) [Citation139], become absorbed into uveal blood vessels (Fbv) [Citation140], travel through uveal channels Fchan [Citation141], and even become reabsorbed back into the ciliary processes (Fcp) [Citation142]. The Goldmann Equation might be revised in the future taking into consideration all of these components of Fu.
7. Expert Opinion
Techniques to measure AHD have revealed the complexities of aqueous humor dynamic beyond the current Goldmann Equation. Each major parameter of AHD is described by its own set of components () which are not described in the Equation. It may be time to consider revising this iconic equation. Others have proposed changes in the past [Citation109,Citation143,Citation144], but only when better methods are developed to reliably measure the components with precision, will a revised Equation be of value. Nevertheless, we offer some ideas to bring the Goldmann Equation into the 21st century.
We have mentioned in this Review, that aqueous production (Fp) in the equation is estimated by measuring flow into the anterior chamber (Fa), but that it also includes flow across the vitreous and retina (Fpost). When Fpost becomes measurable it may attract more scientific interest as its stimulation may become a new means to treat some glaucomas or retinovascular diseases. Fp in the Equation could be separated into Fa+Fpost.
We have discussed how the methods to measure outflow facility do not simply measure trabecular outflow (Ctrab) but other components as well. Facility measured by tonography (Cton) does not include only Ctrab but also uveoscleral facility (Cfu) and pseudofacility (Cps). Facility measured by fluorophotometry (Cfl) includes Ctrab and Cfu. Recalling that C is 1/R, one could substitute Cton for 1/Rtrab + 1/Rfu + 1/Rps or Cfl for 1/Rtrab + 1/Rfu. The Goldmann Equation could be adapted depending on measurement technique used. We also discussed dividing Ctrab into proximal (facility in the trabecular meshwork and Schlemm’s canal endothelium (Cts)) and distal components (downstream facility (Cd)). The equation could be adapted depending on the tissue of interest along the outflow pathways.
Recent research has found that even EVP is not a simple, straightforward parameter. Flow through the 360° circumference of the trabecular meshwork is non-uniform. Some areas drain larger volumes of fluid than other areas. This suggests that EVP may vary in vessels around the eye as well [Citation119]. When EVP was measured in four quadrants of the eye, differences in EVP were not found [Citation119] but maybe with multiple measurements using more sensitive instruments, such differences may be detectable one day. Areas of high flow across the TM might be associated with episcleral veins having low EVP (EVPl) and areas of low flow might be associated with episcleral veins having high EVP (EVPh). When the relative numbers of vessels with high and low EVP and the variability of pressure within each vessel is determined, then the equation could be adjusted accordingly. Obviously, measuring the pressure in just one vein does not give the true picture of EVP.
Uveoscleral outflow is often called the unconventional drainage route for good reason. Fluid seeps in many directions as described above (). In the future, it might be of interest to investigate one of these components separately from the rest. Fu could be substituted for Fs+Fl+Fbv+Fchan+Fcp in the Equation. Realistically, this division of uveoscleral outflow into its component parts will occur only after new technologies can measurement fluid movement and rate in each tissue region separately.
Research on AHD of the whole eye of humans and research animals has fallen behind molecular and cellular research in the outflow pathways. Replacement of old AHD techniques with promising new methods will rapidly advance the field. AHD findings in eyes where blood is flowing, hormones are circulating, and axons are firing could inform the direction of the molecular/cellular research in the near future and will fill in the many gaps in our knowledge of normal ocular health and pathologies.
New methods to measure AHD would benefit clinical diagnosis and treatments as well as research. Important clinical dilemmas could be resolved with individualized, reliable, and valid aqueous humor dynamics measurements. An example is hypotony in the setting of previous glaucoma surgery. Measurement of inflow and outflow rates would allow the surgeon to determine if the hypotony is due to inadequate aqueous production or to excessive drainage. Aqueous humor dynamic measurements could help in decision-making for cyclophotocoagulation procedures in complex glaucomas such as after multiple penetrating keratoplasties. Knowledge of an individual’s aqueous flow rate would help the surgeon to titrate the cyclodestruction to prevent post-operative hypotony. Use of tracers to determine the proportion of aqueous humor passing through trabeculectomies and tubes, would allow surgeons to better plan surgical procedures. Measurements using tracer techniques may no longer need optical clarity of the cornea and aqueous humor, and thus even eyes with opaque corneas or keratoprosthesis could be measurable in the future.
Identifying genetic markers for AHD abnormalities and genetic markers for response to medications will enable treatments to be matched to the individual. It could also be used to explain poor response to glaucoma treatments or the paradoxical rise in IOP with medications. Aqueous humor dynamics measurements soon may be utilized for individualized medication, laser, and surgical recommendations for each patient. It could revolutionize the way glaucoma is practiced and truly pave the way for individualized glaucoma treatment paradigms.
Declarations of Interest
The authors have no relevant affiliations or financial involvement with any organization or entity with a financial interest in or financial conflict with the subject matter or materials discussed in the manuscript. This includes employment, consultancies, honoraria, stock ownership or options, expert testimony, grants or patents received or pending, or royalties.
Reviewer Disclosures
Peer reviewers on this manuscript have no relevant financial or other relationships to disclose
Article Highlights
Current methods to measure the components of aqueous humor dynamics are described along with their assumptions and limitations.
New ideas to improve measurements of aqueous humor dynamics are offered.
With the advancements in methodologies come greater knowledge of aqueous humor dynamics requiring modification of Goldmann’s Equation. Some thoughts of how the equation should be modified are proposed.
Declaration of interest
No potential conflict of interest was reported by the authors.
Additional information
Funding
References
- Kniestedt C, Punjabi O, Lin S, et al. Tonometry through the ages. Surv Ophthalmol. 2008 Nov-Dec;;53(6):568–591. PubMed PMID: 19026320.
- Aziz K, Friedman DS. Tonometers—which one should I use? Eye (Lond). 2018 May;32(5):931–937. . PubMed PMID: 29456251; PubMed Central PMCID: PMCPMC5944656. eng.
- Nuyen B, Mansouri K. Fundamentals and Advances in Tonometry. Asia-Pac J Ophthalmol. 2015 Mar-Apr;;4(2):66–75. . PubMed PMID: 26065347; eng.
- Millar JC, Pang I-H. Non-continuous measurement of intraocular pressure in laboratory animals. Exp Eye Res. 2015 Dec;141:74–90. PubMed PMID: 25933714; eng.
- Aihara M, Lindsey JD, Weinreb RN. Episcleral venous pressure of mouse eye and effect of body position. Curr Eye Res. 2003 Dec;27(6):355-62. doi: 10.1076/ceyr.27.6.355.18194. PMID: 14704919.
- Maepea O, Bill A. The pressures in the episcleral veins, Schlemm’s canal and the trabecular meshwork in monkeys: effects of changes in intraocular pressure. Exp Eye Res. 1989 Oct;49(4):645–663. . PubMed PMID: 2806429; eng.
- Emi K, Pederson JE, Toris CB. Hydrostatic pressure of the suprachoroidal space. Invest Ophthalmol Vis Sci. 1989 Feb;30(2):233–238. PubMed PMID: 2914753..
- Ding C, Wang P, Tian N. Effect of general anesthetics on IOP in elevated IOP mouse model. Exp Eye Res. 2011 Jun;92(6):512–520. . PubMed PMID: 21457709; PubMed Central PMCID: PMCPMC3116023.
- Johnson TV, Fan S, Toris CB. Rebound tonometry in conscious, conditioned mice avoids the acute and profound effects of anesthesia on intraocular pressure. J Ocul Pharmacol Ther. 2008 Apr;24(2):175–185. . PubMed PMID: 18355131.
- Qiu Y, Yang H, Lei B. Effects of three commonly used anesthetics on intraocular pressure in mouse. Curr Eye Res. 2014 Apr;39(4):365–369. . PubMed PMID: 24215504; eng.
- Cone FE, Steinhart MR, Oglesby EN, et al. The effects of anesthesia, mouse strain and age on intraocular pressure and an improved murine model of experimental glaucoma. Exp Eye Res. 2012 Jun;99:27–35. PubMed PMID: 22554836; PubMed Central PMCID: PMCPMC3375133. eng.
- Camras LJ, Sufficool KE, Camras CB, et al. Duration of anesthesia affects intraocular pressure, but not outflow facility in mice. Curr Eye Res. 2010 Sep;35(9):819–827. PubMed PMID: 20795864.
- Nair G, Kim M, Nagaoka T, et al. Effects of common anesthetics on eye movement and electroretinogram. Doc Ophthalmol. 2011 Jun;122(3):163–176. PubMed PMID: 21519880; PubMed Central PMCID: PMCPMC3154733.eng.
- Anjou CI. INFLUENCE OF LIGHT ON THE 24-HOUR VARIATION IN AQUEOUS FLARE DENSITY AND INTRA-OCULAR PRESSURE IN NORMAL RABBITS‘ EYES. PubMed PMID: 13861660; eng Acta Ophthalmol (Copenh). 2009;395:852–873.
- Aihara M, Lindsey JD, Weinreb RN. Twenty-four-hour pattern of mouse intraocular pressure. Exp Eye Res. 2003 Dec;77(6):681–686. . PubMed PMID: 14609556; eng.
- Rowland JM, Potter DE, Reiter RJ. Circadian rhythm in intraocular pressure: a rabbit model. PubMed PMID: 7297102; eng Curr Eye Res. 1981;13:169–173.
- Liu H, Fan S, Gulati V, et al. Aqueous humor dynamics during the day and night in healthy mature volunteers. Arch Ophthalmol. 2011 Mar;129(3):269–275. PubMed PMID: 21402980; eng.
- Liu JH, Kripke DF, Twa MD, et al. Twenty-four-hour pattern of intraocular pressure in the aging population. Invest Ophthalmol Vis Sci. 1999 Nov;40(12):2912–2917. PubMed PMID: 10549652; eng.
- Fan S, Agrawal A, Gulati V, et al. Daytime and nighttime effects of brimonidine on IOP and aqueous humor dynamics in participants with ocular hypertension. J Glaucoma. 2014 Jun-Jul;23(5):276–281. PubMed PMID: 24886701; PubMed Central PMCID: PMCPMC4435779. eng.
- Downs JC. IOP telemetry in the nonhuman primate. Exp Eye Res. 2015 Dec;141:91–98. PubMed PMID: 26216571; PubMed Central PMCID: PMCPMC5576718.
- McLaren JW, Brubaker RF, FitzSimon JS. Continuous measurement of intraocular pressure in rabbits by telemetry. Investigative Ophthalmology & Visual Science. 1996 May;37(6):966–975. PubMed PMID: 8631640..
- Schnell CR, Debon C, Percicot CL. Measurement of intraocular pressure by telemetry in conscious, unrestrained rabbits. Invest Ophthalmol Vis Sci. 1996 May;37(6):958–965. PubMed PMID: 8631639..
- Percicot CL, Schnell CR, Debon C, et al. Continuous intraocular pressure measurement by telemetry in alpha-chymotrypsin-induced glaucoma model in the rabbit:effects of timolol, dorzolamide, and epinephrine. J Pharmacol Toxicol Methods. 1996 Dec;36(4):223–228. PubMed PMID: 9040114.
- Mariacher S, Ebner M, Januschowski K, et al. Investigation of a novel implantable suprachoroidal pressure transducer for telemetric intraocular pressure monitoring. Exp Eye Res. 2016 Oct;151:54–60. PubMed PMID: 27456134; eng.
- Leonardi M, Leuenberger P, Bertrand D, et al. First steps toward noninvasive intraocular pressure monitoring with a sensing contact lens. Invest Ophthalmol Vis Sci. 2004 Sep;45(9):3113–3117. PubMed PMID: 15326128; eng.
- Leonardi M, Pitchon EM, Bertsch A, et al. Wireless contact lens sensor for intraocular pressure monitoring: assessment on enucleated pig eyes. Acta Ophthalmol. 2009 Jun;87(4):433–437. PubMed PMID: 19016660; eng.
- Aptel F, Weinreb RN, Chiquet C, et al. 24-h monitoring devices and nyctohemeral rhythms of intraocular pressure. Prog Retin Eye Res. 2016 Nov;55:108–148. PubMed PMID: 27477112; eng.
- Campigotto A, Leahy S, Zhao G, et al. Non-invasive Intraocular pressure monitoring with contact lens. Br J Ophthalmol. 2019 Jul;2. PubMed PMID: 31266776; eng. DOI:10.1136/bjophthalmol-2018-313714..
- Chen GZ, Chan IS, Leung LK, et al. Soft wearable contact lens sensor for continuous intraocular pressure monitoring. Med Eng Phys. 2014 Sep;36(9):1134–1139. PubMed PMID: 25034639; eng.
- Hsu SH, Chiou JC, Liao YT, et al. An RFID-based on-lens sensor system for long-term IOP monitoring. Conference proceedings: Annual International Conference of the IEEE Engineering in Medicine and Biology Society IEEE Engineering in Medicine and Biology Society Annual Conference. Milan, Italy; 2015:7526–7529. doi: 10.1109/embc.2015.7320133. PubMed PMID: 26738033; eng.
- Xu SC, Gauthier AC, Liu J. The Application of a Contact Lens Sensor in Detecting 24-Hour Intraocular Pressure-Related Patterns. J Ophthalmol. 2016;2016:4727423. PubMed PMID: 27525110; PubMed Central PMCID: PMCPMC4976175. eng.
- Donida A, Di Dato G, Cunzolo P, et al. A Circadian and Cardiac Intraocular Pressure Sensor for Smart Implantable Lens. IEEE Trans Biomed Circuits Syst. 2015 Dec;9(6):777–789. PubMed PMID: 26800549; eng.
- Enders P, Hall J, Bornhauser M, et al. Telemetric Intraocular Pressure Monitoring after Boston Keratoprosthesis Surgery. Ophthalmology. 2019 Feb;126(2):322–324. PubMed PMID: 30366796; eng.
- Mansouri K, Rao HL, Weinreb RN. Short-Term and Long-Term Variability of Intraocular Pressure Measured with an Intraocular Telemetry Sensor in Patients with Glaucoma. Ophthalmology. 2020 Jul ; 12. PubMed PMID: 32663530; eng. DOI:10.1016/j.ophtha.2020.07.016.
- Koutsonas A, Walter P, Roessler G, et al. Implantation of a novel telemetric intraocular pressure sensor in patients with glaucoma (ARGOS study): 1-year results. Invest Ophthalmol Vis Sci. 2015 Jan 22;56(2):1063–1069. PubMed PMID: 25613949; eng.
- Choritz L, Mansouri K, Van Den Bosch J, et al. Telemetric Measurement of Intraocular Pressure via an Implantable Pressure Sensor—12-Month Results from the ARGOS-02 Trial. Am J Ophthalmol. 2020 Jan;209:187–196. PubMed PMID: 31545953; eng.
- Okafor KC, Brandt JD. Measuring intraocular pressure. Curr Opin Ophthalmol. 2015 Mar;26(2):103–109. . PubMed PMID: 25594767; eng.
- Gray JR, Mosier MA, Ishimoto BM. Optimized protocol for Fluorotron Master. Graefes Arch Clin Exp Ophthalmol. 1985;222(4–5):4–5. 225-9. doi: 10.1007/bf02133684 PubMed PMID: 3979849.
- Brubaker RF, McLaren JW. Uses of fluorophotometry in glaucoma research. Ophthalmology. 1985 Jul;92(7):884–890. . PubMed PMID: 4022573; eng.
- Toris CB, Fan S, Johnson TV, et al. Aqueous Flow Measured by Fluorophotometry in the Mouse. Invest Ophthalmol Vis Sci. 2016 Jul 1;57(8):3844–3852. PubMed PMID: 27447085; PubMed Central PMCID: PMCPMC4968429.
- Zeimer RC, Blair NP, Rusin MM, et al. The performance of a new commercial ocular fluorophotometer in the clinical environment. Graefes Arch Clin Exp Ophthalmol. 1985;222(4–5):223–224. PubMed PMID: 3979848.
- Yablonski ME, Zimmerman TJ, Waltman SR, et al. A fluorophotometric study of the effect of topical timolol on aqueous humor dynamics. Exp Eye Res. 1978 Aug;27(2):135–142. PubMed PMID: 680032; eng.
- Maurice DM. A new objective fluorophotometer. Exp Eye Res. 1963 Jan;2(1):33-IN5. . PubMed PMID: 13934003; eng.
- Jones RF, Maurice DM. New methods of measuring the rate of aqueous flow in man with fluorescein. Exp Eye Res. 1966 Jul;5(3):208–220. . PubMed PMID: 5914653; eng.
- Langham M, Wybar KC. Fluorophotometric apparatus for the objective determination of fluorescence in the anterior chamber of the living eye. Br J Ophthalmol. 1954 Jan;38(1):52–57. . PubMed PMID: 13115621; PubMed Central PMCID: PMCPMC1324260. eng.
- Reiss GR, Lee DA, Topper JE, et al. Aqueous humor flow during sleep. Invest Ophthalmol Vis Sci. 1984 Jun;25(6):776–778. PubMed PMID: 6724850; eng.
- Sit AJ, Nau CB, McLaren JW, et al. Circadian variation of aqueous dynamics in young healthy adults. Invest Ophthalmol Vis Sci. 2008 Apr;49(4):1473–1479. PubMed PMID: 18385065; PubMed Central PMCID: PMCPMC2747618. eng.
- Brubaker RF. Flow of aqueous humor in humans [The Friedenwald Lecture]. Invest Ophthalmol Vis Sci. 1991 Dec;32(13):3145–3166. PubMed PMID: 1748546; eng.
- Guo T, Sampathkumar S, Fan S, et al. Aqueous humour dynamics and biometrics in the ageing Chinese eye. Br J Ophthalmol. 2017 Sep ;101(9):1290–1296. PubMed PMID: 28607174.
- Aihara M, Lindsey JD, Weinreb RN. Aqueous humor dynamics in mice. Invest Ophthalmol Vis Sci. 2003 Dec;44(12):5168–5173. . PubMed PMID: 14638713; eng.
- Millar JC, Clark AF, Pang I-H. Assessment of aqueous humor dynamics in the mouse by a novel method of constant-flow infusion. Invest Ophthalmol Vis Sci. 2011 Feb 3;52(2):685–694. . PubMed PMID: 20861483.
- Wilson WS, Shahidullah M, Millar C. The bovine arterially-perfused eye: an in vitro method for the study of drug mechanisms on IOP, aqueous humour formation and uveal vasculature. Curr Eye Res. 1993 Jul;12(7):609–620. . PubMed PMID: 7693396; eng.
- Ishikawa H, Schuman JS. Anterior segment imaging: ultrasound biomicroscopy. Ophthalmol Clin North Am. 2004 Mar;17(1):7–20. . PubMed PMID: 15102510; PubMed Central PMCID: PMCPMC1978090. eng.
- Ang M, Baskaran M, Werkmeister RM, et al. Anterior segment optical coherence tomography. Prog Retin Eye Res. 2018 Sep;66:132–156. PubMed PMID: 29635068; eng.
- Jiao H, Hill LJ, Downie LE, et al. Anterior segment optical coherence tomography: its application in clinical practice and experimental models of disease. Clin Exp Optom. 2019 May;102(3):208–217. PubMed PMID: 30270476; eng.
- Langner S, Martin H, Terwee T, et al. 7.1 T MRI to assess the anterior segment of the eye. Invest Ophthalmol Vis Sci. 2010 Dec;51(12):6575–6581. PubMed PMID: 20688731; eng.
- Crosbie DE, Keaney J, Tam LCS, et al. Age-related changes in eye morphology and aqueous humor dynamics in DBA/2J mice using contrast-enhanced ocular MRI. Magn Reson Imaging. 2019 Jun;59:10–16. PubMed PMID: 30660703; eng.
- Gottschalk HM, Wecker T, Khattab MH, et al. Lipid Emulsion–Based OCT Angiography for Ex Vivo Imaging of the Aqueous Outflow Tract. Invest Ophthalmol Vis Sci. 2019 Jan 2;60(1):397–406. PubMed PMID: 30682210; eng.
- Wecker T, Van Oterendorp C, Reichardt W. Functional assessment of the aqueous humour distal outflow pathways in bovine eyes using time-of-flight magnetic resonance tomography. Exp Eye Res. 2018 Jan;166:168–173. PubMed PMID: 29074388; eng.
- Loewen RT, Brown EN, Roy P, et al. Regionally Discrete Aqueous Humor Outflow Quantification Using Fluorescein Canalograms. PLoS One. 2016;11(3):e0151754. PubMed PMID: 26998833; PubMed Central PMCID: PMCPMC4801333. eng.
- Acott TS, Vranka JA, Keller KE, et al. Normal and glaucomatous outflow regulation. Prog Retin Eye Res. 2020 Aug;11:100897. PubMed PMID: 32795516; eng.
- Saccà SC, Gandolfi S, Bagnis A, et al. The Outflow Pathway: a Tissue With Morphological and Functional Unity. J Cell Physiol. 2016 Sep;231(9):1876–1893. PubMed PMID: 26754581; eng.
- Huang AS, Saraswathy S, Dastiridou A, et al. Aqueous Angiography–Mediated Guidance of Trabecular Bypass Improves Angiographic Outflow in Human Enucleated Eyes. Invest Ophthalmol Vis Sci. 2016 Sep 1;57(11):4558–4565. PubMed PMID: 27588614; PubMed Central PMCID: PMCPMC5017267. eng.
- Cha EDK, Xu J, Gong L, et al. Variations in active outflow along the trabecular outflow pathway. Exp Eye Res. 2016 May;146:354–360. PubMed PMID: 26775054; PubMed Central PMCID: PMCPMC4893924. eng.
- Huang AS, Li M, Yang D, et al. Aqueous Angiography in Living Nonhuman Primates Shows Segmental, Pulsatile, and Dynamic Angiographic Aqueous Humor Outflow. Ophthalmology. 2017 Jun;124(6):793–803. PubMed PMID: 28237425; PubMed Central PMCID: PMCPMC5484000. eng.
- Xie X, Akiyama G, Bogarin T, et al. Visual Assessment of Aqueous Humor Outflow. Asia-Pacific Journal of Ophthalmology (Philadelphia, Pa.). 2019 Mar 27 PubMed PMID: 30916496; PubMed Central PMCID: PMCPMC7028348. eng. 10.22608/apo.201911..
- Gong H, Cha ED, Gorantla V, et al. Characterization of Aqueous Humor Outflow through Novel Glaucoma Devices-A Tracer Study. Invest Ophthalmol Vis Sci. 2012;53(14):3743.
- Friedenwald JS. Contribution to the theory and practice of tonometry. Am J Ophthalmol. 1937;20(10):985–1024. .
- Friedenwald JS. Tonometer calibration; an attempt to remove discrepancies found in the 1954 calibration scale for Schiotz tonometers. Trans Am Acad Ophthalmol Otolaryngol. 1957 Jan-Feb;61(1):108–122. PubMed PMID: 13409426; eng.
- Kazemi A, McLaren JW, Lin S-C, et al. Comparison of Aqueous Outflow Facility Measurement by Pneumatonography and Digital Schiøtz Tonography. Invest Ophthalmol Vis Sci. 2017 Jan 1;58(1):204–210. PubMed PMID: 28114581; PubMed Central PMCID: PMCPMC5256680. eng.
- Friedenwald JS. Some problems in the calibration of tonometers. Am J Ophthalmol. 1948 Aug;31(8):935–944. . PubMed PMID: 18874334.
- Grant WM. Tonographic method for measuring the facility and rate of aqueous flow in human eyes. Arch Ophthalmol. 1950 Aug;44(2):204–214. . PubMed PMID: 15433671; eng.
- Langham ME, Leydhecker W, Krieglstein G, et al. Pneumatonographic studies on normal and glaucomatus eyes. Adv ophthalmol = Fortschritte der Augenheilkunde = Progres en ophtalmologie. 1976;32:108–133, PubMed PMID: 952242; eng
- Langham ME. A NEW PROCEDURE FOR THE ANALYSIS OF INTRAOCULAR DYNAMICS IN HUMAN SUBJECTS. Exp Eye Res. 1963 Oct;2:314–324. PubMed PMID: 14073603; eng.
- ,Langham ME. Evaluation of the pressure cup technique for the measurement of aqueous humor formation. PubMed PMID: 14462146; eng. Investigat Ophthalmol. 1962 Aug;1:484–492
- Ericson LA. Twenty-four hourly variations of the aqueous flow; examinations with perilimbal suction cup. Acta Ophthalmologica. Supplementum. 1958;37(50): 1–95. PubMed PMID: 13544914; eng.
- MacFaul PA. Comparison of perilimbal suction cup analysis and tonography in the diagnosis of glaucoma. Br J Ophthalmol. 1966 Jan;50(1):12–20. . PubMed PMID: 5903828; PubMed Central PMCID: PMCPMC506184. eng.
- Stahnke T, Siewert S, Walther E, et al. Adopting oculopressure tonometry as a transient in vivo rabbit glaucoma model. Curr Direct Biomed Eng. 2015 Sep;1(1):127. English.
- Ulrich WD, Ulrich C. Oculo-oscillo-dynamography: a diagnostic procedure for recording ocular pulses and measuring retinal and ciliary arterial blood pressures. PubMed PMID: 4069567; eng Ophthalmic Res. 1985;175:308–317.
- Popa-Cherecheanu A, Schmidl D, Werkmeister RM, et al. Regulation of Choroidal Blood Flow During Isometric Exercise at Different Levels of Intraocular Pressure. Invest Ophthalmol Vis Sci. 2019 Jan 2;60(1):176–182. PubMed PMID: 30640970; eng.
- Schmidl D, Weigert G, Dorner GT, et al. Role of adenosine in the control of choroidal blood flow during changes in ocular perfusion pressure. Invest Ophthalmol Vis Sci. 2011 Jul 29;52(8):6035–6039. PubMed PMID: 21697134; eng.
- Ravalico G, Toffoli G, Pastori G, et al. Age-related ocular blood flow changes. Invest Ophthalmol Vis Sci. 1996 Dec;37(13):2645–2650. PubMed PMID: 8977478; eng.
- Toris CB Aqueous Humor Dynamics and Intraocular Pressure Elevation. In: Shaarawy S, Hitchings andCrowston editor. Glaucoma, Second Edition. 1. San Diego: Saunders Elsevier; 2014.47–56.
- Rosenquist R, Epstein D, Melamed S, et al. Outflow resistance of enucleated human eyes at two different perfusion pressures and different extents of trabeculotomy. Curr Eye Res. 1989 Dec;;8(12):1233–1240. PubMed PMID: 2627793; eng.
- Waxman S, Wang C, Dang Y, et al. Structure-Function Changes of the Porcine Distal Outflow Tract in Response to Nitric Oxide. Invest Ophthalmol Vis Sci. 2018 Oct 1;59(12):4886–4895. PubMed PMID: 30347083; PubMed Central PMCID: PMCPMC6181305. eng.
- Bäräny EH, Linnër E, Lütjen-Drecoll E, et al. Structural and functional effects of trabeculectomy in cynomolgus monkeys. I. Light microscopy. Albrecht Von Graefes Archiv Fur Klinische Und Experimentelle Ophthalmologie Albrecht Von Graefe’s Archive for Clinical and Experimental Ophthalmology. 1972;184(1):1–28. PubMed PMID: 4623578; eng.
- Lütjen-Drecoll E, Bárány EH. Functional and electron microscopic changes in the trabecular meshwork remaining after trabeculectomy in cynomolgus monkeys. Investigat Ophthalmol. 1974 Jul;13(7):511–524. PubMed PMID: 4209932; eng.
- Toris CB, Pederson JE. Effect of intraocular pressure on uveoscleral outflow following cyclodialysis in the monkey eye. Invest Ophthalmol Vis Sci. 1985 Dec;26(12):1745–1749. PubMed PMID: 4066210..
- Bill A. The aqueous humor drainage mechanism in the cynomolgus monkey (Macaca irus) with evidence for unconventional routes. Investigat Ophthalmol. 1965 Oct;4(5):911–919. PubMed PMID: 4157891..
- Bill A. The routes for bulk drainage of aqueous humour in the vervet monkey (Cercopithecus ethiops). Exp Eye Res. 1966 Jan;5(1):55–57. . PubMed PMID: 4160407.
- Kupfer C, Sanderson P. Determination of pseudofacility in the eye of man. Arch Ophthalmol. 1968 Aug;80(2):194–196. . PubMed PMID: 5661885; eng.
- Todd R, Woodhouse D. Pseudofacility: a method of measurement using suction-cup tonography. Exp Eye Res. 1973 Oct 24;17(2):173–182. . PubMed PMID: 4759534; eng.
- Armaly MF. The consistency of the 1955 calibration for various tonometer weights. Am J Ophthalmol. 1959 Nov;48(5):Pt 2:602–11. . PubMed PMID: 13794267.
- Gaasterland D, Kupfer C, Milton R, et al. Studies of aqueous humour dynamics in man VI. Effect of age upon parameters of intraocular pressure in normal human eyes. Exp Eye Res. 1978;26(6):651–656. 1978/06/01/;doi: 10.1016/0014-4835(78)90099-4 en.
- Pallikaris IG, Kymionis GD, Ginis HS, et al. Ocular rigidity in living human eyes. Invest Ophthalmol Vis Sci. 2005 Feb;46(2):409–414. PubMed PMID: 15671262; eng.
- Palko JR, Morris HJ, Pan X, et al. Influence of Age on Ocular Biomechanical Properties in a Canine Glaucoma Model with ADAMTS10 Mutation. PLoS One. 2016;11(6):e0156466. PubMed PMID: 27271467; PubMed Central PMCID: PMCPMC4894564. eng.
- Hayashi M, Yablonski ME, Novack GD. Trabecular outflow facility determined by fluorophotometry in human subjects. Experimental eye research. 1989 May;48(5):621–625. . PubMed PMID: 2737260.
- Becker B. The decline in aqueous secretion and outflow facility with age. Am J Ophthalmol. 1958 Nov;46(5 Part 1):731–736. . PubMed PMID: 13595051.
- Toris CB, Yablonski ME, Wang YL, et al. Aqueous humor dynamics in the aging human eye. Am J Ophthalmol. 1999;127(4):407–412.
- Yelenskiy A, Ko MK, Chu ER, et al. Total Outflow Facility in Live C57BL/6 Mice of Different Age. Biomedicine Hub. 2017 Sep-Dec;2(3):1–10. PubMed PMID: 31988919; PubMed Central PMCID: PMCPMC6945946. eng.
- Lopez NN, Patel GC, Raychaudhuri U, et al. Anterior chamber perfusion versus posterior chamber perfusion does not influence measurement of aqueous outflow facility in living mice by constant flow infusion. Exp Eye Res. 2017 Nov;164:95–108. PubMed PMID: 28822760; eng.
- Sherwood JM, Reina-Torres E, Bertrand JA, et al. Measurement of Outflow Facility Using iPerfusion. PLoS One. 2016;11(3):e0150694. PubMed PMID: 26949939; PubMed Central PMCID: PMCPMC4780770. eng.
- Barany EH. Simultaneous Measurement of Changing Intraocular Pressure and Outflow Facility in the Vervet Monkey by Constant Pressure Infusion. PubMed PMID: 14166270. Investigat Ophthalmol. 1964 Apr;3:135–143
- Boussommier-Calleja A, Li G, Wilson A, et al. Physical Factors Affecting Outflow Facility Measurements in Mice. Invest Ophthalmol Vis Sci. 2015 Dec;56(13):8331–8339. PubMed PMID: 26720486; PubMed Central PMCID: PMCPMC4699419. eng.
- Gabelt BT, Kaufman PL. The effect of prostaglandin F2 alpha on trabecular outflow facility in cynomolgus monkeys. Exp Eye Res. 1990 Jul;51(1):87–91. . PubMed PMID: 2373185; eng.
- Poyer JF, Gabelt B, Kaufman PL. The effect of topical PGF2 alpha on uveoscleral outflow and outflow facility in the rabbit eye. Exp Eye Res. 1992 Feb;54(2):277–283. . PubMed PMID: 1559555; eng.
- Bill A. Conventional and uveo-scleral drainage of aqueous humour in the cynomolgus monkey (Macaca irus) at normal and high intraocular pressures. Exp Eye Res. 1966 Jan;5(1):45–54. . PubMed PMID: 4160221.
- Ascher KW The Aqueous Veins: i. Physiologic importance of the visible elimination of intraocular fluid Am J Ophthalmol. 2018 //08/;192:xxix-liv. eng. 192 xxix-liv
- Brubaker RF. Goldmann’s equation and clinical measures of aqueous dynamics. Exp Eye Res. 2004;78(3):633–637.doi: 10.1016/j.exer.2003.07.002
- Greslechner R, Oberacher-Velten I. [Glaucoma due to elevated episcleral venous pressure]. Der Ophthalmologe: Zeitschrift der Deutschen Ophthalmologischen Gesellschaft. 2019 May;116(5):423–429.10.1007/s00347-018-0828-4 PubMed PMID: 30506098; ger.
- Celebi S, Alagöz G, Aykan U. Ocular findings in Sturge-Weber syndrome. Eur J Ophthalmol. 2000 Jul-Sep;10(3):239–243. PubMed PMID: 11071032; eng.
- Phelps CD. The pathogenesis of glaucoma in Sturge-Weber syndrome. Ophthalmology. 1978 Mar 85;3(3):276–286. . PubMed PMID: 662281; eng.
- Shiau T, Armogan N, Yan DB, et al. The role of episcleral venous pressure in glaucoma associated with Sturge-Weber syndrome. J Am Assoc Pediat Ophthalmol Strabismus. 2012;16(1):61–64. 2012/02/01.
- Silverstein M, Salvin J. Ocular manifestations of Sturge-Weber syndrome. Curr Opin Ophthalmol. 2019 Sep;30(5):301–305. . PubMed PMID: 31313748; eng.
- Foroozan R, Buono LM, Savino PJ, et al. Idiopathic dilated episcleral veins and increased intraocular pressure. Br J Ophthalmol. 2003 May;87(5):652–654. PubMed PMID: 12714421; PubMed Central PMCID: PMCPMC1771676. eng.
- Linner E. Measurement of the pressure in Schlemm’s canal and in the anterior chamber of the human eye. Experientia. 1949;5(11):451. 1949/11/15/;doi: 10.1007/bf02165263 eng.
- Krakau CET, Widakowich J, Wilke K. Measurements of the Episcleral Venous Pressure by Means of an Air Jet. Acta Ophthalmol. 1973;51(2):185–196.
- Zeimer RC, Gieser DK, Wilensky JT, Noth JM, Mori MM, Odunukwe EE. A practical venomanometer. Measurement of episcleral venous pressure and assessment of the normal range. Arch Ophthalmol. 1983 Sep;101(9):1447-9. doi: 10.1001/archopht.1983.01040020449024. PMID: 6615314.:
- Sit AJ, Ekdawi NS, Malihi M, et al. A novel method for computerized measurement of episcleral venous pressure in humans. Exp Eye Res. 2011. 2011/06//;92(6):537–544. eng.
- Gaasterland DE, Pederson JE Episcleral venous pressure: a comparison of invasive and noninvasive measurements. Invest Ophthalmol Vis Sci. 1983 1983/10//;24( 10):1417–1422. eng.
- Sultan M, Blondeau P. Episcleral venous pressure in younger and older subjects in the sitting and supine positions. J Glaucoma. 2003;12(4):370–3. doi: 10.1097/00061198-200308000-00013. PMID: 12897584.
- Meyer PA. Patterns of blood flow in episcleral vessels studied by low-dose fluorescein videoangiography. PubMed PMID: 3256492; eng Eye (Lond). 1988;2Pt 5:533–546.
- Brubaker RF. Determination of episcleral venous pressure in the eye. A comparison of three methods. Archives of Ophthalmology (Chicago, Ill. : 1960) Archives of Ophthalmology. 1967 1967/01/01/; 77(1):110–114. doi: 10.1001/archopht.1967.00980020112024. PMID: 6015706.
- Reitsamer HA, Kiel JW, Rabbit A. Model to Study Orbital Venous Pressure, Intraocular Pressure, and Ocular Hemodynamics Simultaneously. Invest Ophthalmol Vis Sci. 2002;43(12): 3728–3734. 2002/12/01/.
- Yap TY, Nelson CA, Ghate D, et al.. Design of a Portable Venomanometer System for Episcleral Venous Pressure Measurement. 2020 Design of Medical Devices Conference; Minneapolis, Minnesota, USA. April 6–9, 2020. V001T10A004. ASME. https://doi.org/10.1115/DMD2020-9021
- Dvorak-Theobald G. Further studies on the canal of Schlemm; its anastomoses and anatomic relations. Am J Ophthalmol. 1955 Apr;39(4):65–89. . PubMed PMID: 14361607; eng.
- Selbach JM, Rohen JW, Steuhl KP, et al. Angioarchitecture and innervation of the primate anterior episclera. Curr Eye Res. 2005 May;30(5):337–344. PubMed PMID: 16020264; eng.
- Brain Trauma F. American Association of Neurological S, Congress of Neurological S, et al. Guidelines for the management of severe traumatic brain injury. eng VIII. Intracranial Pressure Thresholds. J Neurotrauma. 2007;241:S55–58.
- Kim H, Lee H-J, Kim Y-T, et al. Novel index for predicting mortality during the first 24 hours after traumatic brain injury. J Neurosurg. 2018. 2018/12/21/:19. doi: 10.3171/2018.7.JNS18995 eng. .
- Jonas JB, Wang N, Yang D, et al. Facts and myths of cerebrospinal fluid pressure for the physiology of the eye. Prog Retin Eye Res. 2015. 2015/05//;46:67-83. 10.1016/j.preteyeres.2015.01.002. eng. .
- Blondeau P, Tétrault J-P JP, Papamarkakis C. Diurnal variation of episcleral venous pressure in healthy patients: a pilot study. Journal of Glaucoma. 2001/02//A Pilot Study. Journal of glaucoma;10(1):18–24. doi: 10.1097/00061198-200102000-00005. PMID: 11219634.
- Kupfer C, Gaasterland D, Ross K. Studies of Aqueous Humor Dynamics in Man: II. Measurements in Young Normal Subjects Using Acetazolamide and L-Epinephrine. Invest Ophthalmol Vis Sci. 1971 1971/07/01/;10(7):523–533.
- Gabelt BT, Kaufman PL. Changes in aqueous humor dynamics with age and glaucoma. Prog Retin Eye Res. 2005 Sep;24(5):612–637. . PubMed PMID: 15919228; eng.
- Millar JC, Phan TN, Pang IH, et al. Strain and Age Effects on Aqueous Humor Dynamics in the Mouse. Invest Ophthalmol Vis Sci. 2015 Sep;56(10):5764–5776. PubMed PMID: 26325415; eng.
- Toris CB, Koepsell SA, Yablonski ME, et al. Aqueous humor dynamics in ocular hypertensive patients. J Glaucoma. 2002 Jun;11(3):253–258. PubMed PMID: 12140404..
- Johnson TV, Fan S, Camras CB, et al. Aqueous humor dynamics in exfoliation syndrome. Arch Ophthalmol. 2008 Jul;126(7):914–920. PubMed PMID: 18625936.
- Lim KS, Nau CB, O’Byrne MM, et al. Mechanism of action of bimatoprost, latanoprost, and travoprost in healthy subjects. A crossover study. Ophthalmology. 2008 May;115(5):790–795 e4. PubMed PMID: 18452763; PubMed Central PMCID: PMCPMC2769924.
- Inomata H, Bill A. Exit sites of uveoscleral flow of aqueous humor in cynomolgus monkey eyes. Exp Eye Res. 1977 Aug;25(2):113–118. . PubMed PMID: 410651; eng.
- Yücel YH, Johnston MG, Ly T, et al. Identification of lymphatics in the ciliary body of the human eye: a novel “uveolymphatic” outflow pathway. Exp Eye Res. 2009 Nov;89(5):810–819. PubMed PMID: 19729007; eng.
- Green K, Sherman SH, Laties AM, et al. Fate of anterior chamber tracers in the living rhesus monkey eye with evidence for uveo-vortex outflow. Trans Ophthalmol Soc UK. 1977 Sep;97(4):731–739. PubMed PMID: 103255; eng.
- Krohn J, Bertelsen T. Corrosion casts of the suprachoroidal space and uveoscleral drainage routes in the human eye. Acta Ophthalmol Scand. 2009 Feb;75(1):32–35. . PubMed PMID: 9088397; eng.
- Toris CB. The eye’s aqueous humor. Aqueous Humor Dynamics I, Measurement methods and animal studies. In: Civan MM, editor. Current Topics in Membranes. Vol. 62. San Diego: Elsevier, Inc; 2008. p. 193–229.
- Becker B, Neufeld AH. Pressure dependence of uveoscleral outflow. J Glaucoma. 2002 Oct;11(5):464. . PubMed PMID: 12362090; eng.
- Kaufman PL. Some thoughts on the pressure dependence of uveoscleral flow. J Glaucoma. 2003 Feb;12(1):93–94. 89; author reply . doi: 10.1097/00061198-200302000-00018 PubMed PMID: 12619645; eng.