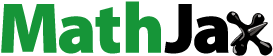
ABSTRACT
Green Chemistry (GC) is a rapidly developing technology that is becoming a very appealing subject for both academic and industrial systems. Sustainable techniques in GC often include substituting hazardous solvents with innocuous ones so that the latter may be included in a particular process. Green analytical chemistry (GAC) is the most well-known field of study in GC. The notion of GC has now advanced to a new level, focusing on aspects other than human health and the environment, such as the life cycle of specific applications and their transdisciplinary activities, and reaching the system level. The main topics covered in this article are the principles of GC, specifics regarding safe and hazardous solvents and their reaction conditions, sustainable synthesis, catalysis, and, lastly, the industrial applications of GC in the production of food, energy, water treatment, and educational materials. The last section discusses the main difficulties in the area of GC. This article offers instances of GC that significantly improve the sustainability of industrial processes and makes recommendations for appropriate actions that support GC production in a widely accepted way.
The initiative of Green Chemistry (GC) is increasingly developed in the 90s, where at that time critical evaluation is performed among the chemical products. Since the 1940s, the ecological conditions are analyzed which leads to the development of the industries. To solve environmental issues and product development practices the idea of sustainable processes is introduced. In the 1990s, an evolution is formed in GC in terms of the creation of its 12 principles by Paul Anastas and John Warner which greatly handled the toxic solvents in chemicals in an effective manner. (Citation1) Analytical methodologies are at the forefront of Green Chemistry research and development. The primary objective is to establish new methods and techniques that minimize the usage and production of hazardous substances throughout all stages of chemical analysis. This pursuit is commonly referred to as Green Analytical Chemistry.
The main goal of the chemical sector is to minimize the pessimistic effects which take place in the environmental and health sectors. To improve the life cycle of a chemical, several concepts need to get concentrated by the researchers, they are minimizing the risk and quantifying the risk occurrences in a sustainable direction (Citation2). As the global population is predicted to reach 9 billion by 2055, conserving food, fuel, fibers, and feed is imperative. The future lies in sustainable and renewable solutions that will help us meet these challenges (Citation3). The other challenges in GC are multiple scientific and engineering disciplines are essential to recognize chemical and physical phenomena. Non-traditional fields like biochemistry and nano-chemistry can still benefit GC research. While some fields such as biochemistry and nano-chemistry are not commonly associated with traditional chemistry, they can still provide valuable insights for research in various disciplines of GC.
Later, Green Analytical Chemistry (GAC) is developed in 2000 which make use of analytical chemistry techniques to neglect the solvents, reagents, and preservatives, and other kinds of chemicals that are harmful to human health (Citation4, Citation5). Many laboratory-based applications are developed by GAC to reduce the chemical-based negative effects and later, the highest priority is provided to this process and as well it becomes one of the analytical chemistry development-based stimulants. To increase the accuracy of analytical results, several environmentally friendly studies are started developing (Citation6). With the increase of analytical methods, several active ideas are created in the field of GC and GAC that reduce the hazardous substances in all the processes of chemical analysis. The GAC is multidimensional where each chemical analysis process has several consequences in terms of products, by-products, and external environments like population, analyst, and the place of research (country) (Citation7).
This article seeks to delve into the multifaceted landscape of Green Chemistry, unraveling its foundational principles, elucidating the nuances of safe and hazardous solvents, exploring sustainable synthesis techniques, delving into catalysis methods, and ultimately examining the industrial applications of GC. From the production of vital resources such as food and energy to the critical domains of water treatment and educational materials, Green Chemistry manifests as a catalyst for transformative change. Finally, its challenges and outlook are given. Thus this article shows a critical review of the (i) Principles of Green Chemistry, (ii) Green Solvents and Reaction Conditions, (iii) Sustainable Synthesis and Catalysis, (iv) Green Chemistry in Industrial Applications, (v) Challenges and Future Outlook, and (vi) Challenges and Future Outlook. The contribution of this article is illustrated in .
In this section, the principles of GC and GAC are elaborated in which mainly concentrates on reducing the power utilization, toxicity, and reagents usage and as waste reduction and so on.
Table 1. Principles of GC and GAC.
In this section, the green solvents and its reaction conditions are elaborated in a sequential manner. Initially, the solvent production and the raw material extraction process are described in which includes its power allocated for the process of production, synthesis, and transportation (Citation15). In GAC, certain alternative solvents are used: (i) water–enzyme assisted extraction, micellar extraction, hydrotropic extraction, and subcritical water extraction, (ii) NaDEs and ChCl-EG, (iii) biobased solvents – 2-MeOx, D-limonene, Ethyl lactate; (iv) supercritical and (v) liquefied gases – n-propane, n-butane and DME (Citation16, Citation17). In general, environment-friendly green solvents are utilized for agricultural purposes for example – ethyl acetate is the byproduct of corn and lactate ester which is used for painting works. Such solvents are biodegradable and as well it can be recycled. Ethyl lactate is a coating material that is used to coat metals and wood. Later, the ethyl lactate is place by acetone, xylene, and toluene to get better and safer performance in the workplace. The solvents which are used in large quantities are ionic liquids, PEGs, ethyl lactate, water, methanol, nitromethane, acetone, ethanol, DMF, DMSO, ammonia, acetonitrile, HMPA, pyridine, etc. (Citation18). Later, Deep Eutectic Solvents (DES) are developed, and they are called the new generational green solvents which are mainly used for analytical chemistry (Citation19).
The environmentally friendly solvent γ-butyrolactone is utilized to make polymeric hollow fiber (HF) and flat sheet (FS) (Citation20), while fluoroquinolone solvents are employed for their broad anti-microbial action (Citation21), and drug identification is carried out in supercritical solvent.(Citation22, Citation23) The additional chemicals which are employed in GC include HPLC grade Ethanol, HPLC quality acetic acid, AR grade hydrochloric acid, sodium hydroxide, hydrogen peroxide, and gradient grade methanol (Citation24, Citation25). Recently, Decision Tree (DT), Extra Trees (ET), and Gradient Boosting (GB) models—all based on machine learning—were developed as ways to estimate the solubility of drugs in a solvent (Citation26, Citation27)
In this section, the process of sustainable synthesis and catalysis is elaborated. shows the categories of sustainable chemistry are described (Citation28)
Table 2. Categories of sustainable chemistry and description.
Nowadays the development of eco-friendly sustainable synthesis is highly focused, and it is propelled by computational chemistry. Subsequently, eco-catalysts—the primary organic syntheses—are created, and their unique structure incorporates the advantages of both homogeneous and heterogeneous catalysts, such as selectivity, activity, and recyclability. Phytochemical components such as Fe55Co45 nanoparticles, Fe (NO3)3H2O and propionic acid, SiO2, CeO2, TiO2, Fe2O4, and Al2O3 (Citation29), HCl, HNO3, and H2SO4 are used in the green chemistry process. Organometallic reagents, which are the active chemicals in pharmaceuticals, are synthesized using specific processes. To create active pharmaceutical ingredients (APIs), a sustainable synthesis approach is used, which aids in the reduction of hazardous chemicals and contaminants. The talent shortage method employs hybrid sol–gel catalysts, which are employed in nano-catalysis.
Biocatalytic approaches are used to catalyze a variety of chemical processes in an environmentally sustainable way. Because few of the reagents are hazardous to the environment, naturally occurring herb extracts are used in the newly released nanostructured materials. Since the production of graphene is a hazardous chemical, replacing it with biogenic reduced graphene derivatives is bad for the environment. Instead, integrated analysis is being developed to enhance catalytic performance(Citation30).
Because of the chemical manufacturing process, the connectivity among the production of water, energy, and food might get affected. Even due to this relationship, this manufacturing process encloses various impacts. The impacts and relationship of water, energy, and food are described in . Green Chemistry has come a long way from its origins as a laboratory curiosity. The aim is to establish a sustainable future. Thanks to the hard work of academic and industrial researchers, a plethora of green methodologies are now available for businesses to take advantage of (Citation31). From small enterprises to multi-national corporations, many have embraced the principles of Green Chemistry to make strategic choices for sustainability. These include reducing hazardous processes and products, shifting away from inefficient chemical routes, and replacing oil-based feedstocks with renewable starting materials. These decisions will have a profound impact on the global chemical markets.
This section elaborates on the industrial uses of green chemistry in the production of food, energy, and water treatment. Furthermore, the worldwide trends in general education from K–12 to tertiary education are examined to foster a society that is healthier, cleaner, and more sustainable. Additionally, the adoption of the Green Chemistry policy in a number of nations is discussed to lessen the use of dangerous chemicals in chemical processes and to encourage creativity in chemical research and development.
The AOP of hydrodynamic cavitation is given in (Citation32), and it is a hybrid model with the combination of potassium persulfate (KPS) and hydrogen peroxide (H2O2). Due to the AOP, the cavitation yield efficiency is improved up to 98% for the HC–KPS–H2O2. The materials which are involved in this process are Ponceau 4R and Tartrazine, where it includes all kinds of domestic resources and analytical methods used in this advanced oxidation process. The preparation of Fe3O4 @TiO2-P25 photocatalyst is performed with the presence of solar AOP (Citation33), the material, which is used for this process are terephthalic acid, iron (II) chloride tetrahydrate, iron (III) nitrate nonahydrate, titanium (IV) isopropoxide, titanium (IV) butoxide, and ethylene glycol. Under the process of AOP, rhodamine and bromocresol green by FeOCl-based adsorption and degradation are performed. FeOCl is one of the heterogeneous catalysts which are mainly used for the purpose of the pollutant removal process. Only at pH level of 10, it can be able to maintain a lower low adsorption level for rhodamine but for bromocresol, at the point of pH level 10, the absorption is zero, so it gets fixed with the pH value of 7. Some mathematical calculation is present here to measure the degradation percentage of photo-fenton which is given in equation 1.
(1)
(1) In Equation (1), the terms At implies the absorbance performance at the time ‘t’ and A0 implies the value of initial absorbance.
In general, FeOCl is one among the impending catalyst which is used in several industries and with the humidification condition it can be able to provide better performance (Citation34). The available other types of AOP processes are catalytic wet air based, supercritical water based, hybrid process, sulphate radical based and carbonate radical based. The major applications of AOP are described in .
Figure 4. Major applications of AOPs (Citation35).

In (Citation36), three types of adsorption models such as malachite green adsorption kinetics, malachite green adsorption equilibrium, and malachite green adsorption thermodynamics mainly to find the reason for environmental degradation. This study also talks about the synthesized plantain leaf biochar (PLB) characteristics with its synthesized characterization using BET, XRD, SEM, and FTIR spectrometries. Through this process, the regeneration rate of PLB is increased up to 97% so that it is declared one of the sustainable green adsorbents and it is mainly utilized for wastewater treatment in industrial sectors. In (Citation37), the absorption of heavy metal ions using chitosan/graphene is concentrated and the methods used for this absorption process are Fourier-transform infrared spectroscopy (FT-IR) and Scanning electron microscope (SEM) and so on. In this research, the cadmium-based nanosample adsorption is improvised up to 20% which maintains the pH level of 7 and that helps to reach the optimal adsorption rate at cadmium ions. In other research, green synthesis of iron oxide nanoparticles (CN–Fe2O3NPs) is taken into consideration which is mainly used for the water purification process (Citation38). In (Citation39), the adsorption of potassium permanganate (KMnO4) is given which is mainly used in wastewater treatments. Its pre-oxidation is done with the presence of several pH values. At the end of the desorption process, it is understood this process utilizes very low power to perform these operations.
In biogenic green synthesis, the zinc oxide nanoparticles (ZnO NPs) are mainly used for plant treatments which include roots, vegetables, fruits, flowers, peels, and leaves. Plants are ordinary biological substrates, and it is considered for the ZnO NPs hence it is highly cost effective and eco-friendly which is not affecting the micro-organisms. Its preparation process includes the perfect washing of plants and then drying where the solvents are added to create the plant extract. Finally, it gets mixed with Zn (II) salt, and it acts like an NPs precursor, which leads to the creation of the precipitate which is known as ZnO NPs. The factors which trouble this plant extract process are its physical and chemical methods, various usages of active components for plant species, pH level, and temperature. This plant extract mainly concentrates on two processes which are the reduction of Zn (II) and the stabilization of resultant nanostructures (Citation40, Citation41).
Green solvents are specifically formulated to reduce their impact on both human health and the environment. They are essential for various industrial processes such as cleaning, extraction, synthesis, and formulation by dissolving other substances to create solutions. Unlike conventional solvents, which can be highly toxic, volatile, flammable, and non-biodegradable, green solvents are developed to mitigate these negative effects and maintain or enhance process efficiency. The extraction techniques present in the green solvents are described in . Bioactive compounds are extracted from biomass with the presence of a huge quantity of toxic solvents. The increased usage of green chemistry replaced those toxic solvents with eco-friendly green solvents. The review examines the use of new solvents in comparison to traditional solvents when extracting bioactive compounds. The examination focuses on extraction techniques, process parameters, and the advantages and disadvantages of novel solvents to evaluate the most practical and efficient methods. The extraction techniques like pressurized solvent extraction (PSE), microwave-assisted extraction (MAE), ultrasound-assisted extraction (UAE), and aqueous two-phase extraction (ATPE) are compared in terms of solute compatibility and extraction efficiency. Additionally, the benefits and drawbacks of using green solvents in these processes, including factors such as ease of synthesis, environmental impact, toxicity, and applicability to both traditional and novel extraction processes are presented. (Citation42)
Figure 5. Green solvents extraction techniques (Citation42).

The review offers insight into sampling strategies and provides the latest methods for chemical analysis of antibiotic residues in various sample matrices. The article delves into solvent-based sample preparation techniques that utilize green solvents and highlights their applications in antibiotic residue analysis (Citation43). The seven different natural deep eutectic solvents (NADES) coupled with ultrasound-assisted extraction (UAE) is utilized to extract bioactive compounds from date seeds. The seven different NADESs include choline chloride as hydrogen bond acceptors (HBA) and four sugars, two organic acids, and one polyalcohol as hydrogen bond donors (HBD). The green extraction using NADESs is more efficient compared to conventional solvents (Citation44). The technique TOPSIS (Technique for Order of Preference by Similarity to Ideal Solution) is utilized to rank the dispersive extraction solvents based on their proximity to the ideal solution and farthest from the negative-ideal solution (Citation45)
The efficacy of vegetable oils like extra virgin coconut oil, tea seed oil, soybean oil, and extra light olive oil as alternative solvents in the extraction of diphenhydramine hydrochloride (DPHH) using simple green colorimetric liquid–liquid partitioning is investigated. The oils were able to successfully extract the ion pair of DPHH and bromocresol green (BCG) from the aqueous phase into the organic phase. The procedure was performed on a microliter scale and monitored using a smartphone to track the color changes of the organic phase (Citation46)
Some of the important green catalysts which are employed in a maximum of industrial applications are metal-graphene catalysts, catalysts used for CO2 reduction process, sodium borohydride in methanol catalysts used for the process of dehydrogenation heterogeneous catalysts used in aromatics and nanopalladium based in water treatment (Citation47)
The LCA of large-scale hydrogen production consists of two processes, they are technical parametric changes in foreground and background processes in an adaptive manner and it varies according to the future economy. The environmental sustainability of LCA helps to increase the future yield in a theoretical manner (Citation48). Stir bar sorptive extraction (SBSE) is one among the popular process and it consists of high sensitivity and reproductively and the other hand solid phase extraction (SPE) is present which is more intensive. LCA of these two methods includes ISO 14040-44 standards. It includes life cycle inventory, impact assessment and interpretation. The other integrated life cycle assessment is subdivided into certain categories, they are environmental, social, life cycle costing, and finally life cycle sustainability assessment (Citation49).
In terms of energy production, green chemistry is used in maximum of the industrial sectors. The main process which are highly concentrated in the energy production are renewable energy sources, energy storage, fuel production & utilization, carbon capture & utilization, sustainable catalysis, LCA and sustainability analysis and it is detailed in upcoming section. The workflow of energy production process is illustrated in .
The scenarios which are worked in energy sector to increase the contribution of renewable energy sources are hydropower, solar power, tidal energy, wind energy, wave energy, geothermal energy, and biomass energy. In maximum of the industrial sector like energy saving technology and grid power, the photovoltaic and wind energy is utilized (Citation50).
Liquid renewable green fuels like liquid hydrogen, liquefied methane, methanol, and ammonia (Citation51) are stored in effective manner to create the export pathway to move these materials from one to another. RGF pathways are created which significantly increased the shipping transport to 4000 km. Maximum of the liquid pathways are about the export of LH2 and LCH4. Later, the distance is increased up to 18,000 km.
Biogases are mainly the mixture of methane and CO2 which also includes the traces of some gases like O2, N2, H2, H2O, H2S, NH3). The production of these gases is done through the process of anaerobic digestion in biodigesters. Fuel production will get increase more than 50% in 2040 which we are doing today. Lowering the production cost is the big expectation all the time (Citation52). As the results for the green gas production, a cost-effective process is introduced which processes the intensification using the membrane reactor (MR) and that helps to reduce the production cost and to increase the efficiency of large-scale fuel production. With the presence of integrated MR, pure hydrogen gas is produced, and it is uni-equip mental process so that high expenditures are avoided (Citation53).
In general, industrial decarbonization is performed through carbon capture, utilization, and storage (CCUS) process. The major technical options which are present here to perform CCUS are micro-algae cultivation, chemical carbon capture, direct air capture, tree, and plants (Citation54). The major sources for this process are industrial power plant emission from the atmosphere. The reusable materials from this process are fermentation, catalyst-assisted electrochemical & chemical conversion, injection into building materials and direct use materials without conversion. The end products are in terms of fuels, methanol, ethanol, and methane. In terms of polymers, it is polyols and finally greenhouses gas to increase the plant yield (Citation55).
The catalysis which is used to construct the fundamental process of modern energy and chemical industry are petroleum, coal, biomass, and other essential resources (Citation56). The basic design methods to build the catalysis routes are chemical oriented refining; syngas to light olefins, light alkanes to olefins-based dehydrogenization process, plastic recycling process and conversion of biomass into chemicals. Some of the effective electrocatalysis process is water electrolysis-based hydrogen production, carbon dioxide conversion to carbon monoxide, methanol, ethylene, and ethanol. Some of the innovative photocatalysis are water splitting process, C–C bond coping process, efficiency improvement and catalytic selectivity enhancement (Citation57).
LCA of energy-based green chemistry technology is constructed with certain steps namely its goal, life cycle inventory, impact assessment, and interpretation. The methodology-based step for the system boundaries of LCA includes certain sections such as power homogenization, solid state calcinations, and deagglomeration. The system boundary of life cycle sustainability analysis (LCSA) includes three steps: raw material extraction and processing, components production, and cell production (Citation58).
In terms of food production, green chemistry is used in maximum of the industrial sectors. The main processes, which are highly concentrated in the energy production, are sustainable agriculture, food processing and preservation, waste reduction and vaporization, green solvents and extraction methods, food packaging and materials, its LCA and sustainability analysis and it is detailed in upcoming section. The workflow of food production and packaging process is illustrated in .
GAC is mainly contributed for food safety and its bioactivities. Sustainable development goals for GAC in agriculture include its bioeconomy. Mainly sustainable agriculture aims to produce sustainable materials, protein, and energy with the presence of the green biomass like grass, fibers, and green juice etc. Incorporate microalgae cultivation is one among the popular technique that performs maximum protein production using the green juice which gets cultivated from the grass. Around 45% of protein contents are cultivated using this process in sustainable agriculture (Citation59).
The green chemistry-based food processing includes several sections: (i) liquid extraction processes are Single-Drop microextraction (SDME), Hollow-Fiber liquid-phase microextraction (HF-LPME), Electro-membrane microextraction (EME), Dispersive liquid–liquid microextraction (DLLME), QuEChERS, Ultrasound-Assisted back extraction (UABE), Ultrasound-Assisted extraction (UAE), Microwave-Assisted extraction (MAE), Pressurized liquid extraction (PLE), Supercritical fluid extraction (SFE), and Enzyme-Assisted extraction (EAE) (Citation60), (ii) Solid extraction processes are Solid-Phase microextraction (SPME), Dispersive solid-phase extraction (DSPE), Microextraction in packed sorbent (MEPS), Stir-Bar sorbent extraction (SBSE), and Fabric phase sportive extraction (FPSE) (Citation61), (iii) Gas extraction processes include Headspace (HS), Membrane/membrane less gas diffusion devices, and New green solvents (Citation62).
In the trial-and-error method, waste reduction in food-based green chemistry is calculated in 42 laboratories which use the terms of reagents, products, and subproducts that are considered for the chemical reaction. The mathematical analysis for the calculation of waste reduction is given in Equation (2) (Citation63):
(2)
(2) In general, the green solvents are categorized into several sections: energy efficient, green synthesis, recyclable, reusable, renewable, cost effective, biocompatible, non-toxic, non-flammable, and safe manipulation. The green solvents which are used for the micro-extraction process are ionic liquids, deep eutectic solvents, supramolecular solvents, and switchable solvents (Citation64).
Biotechnology is mainly utilized to provide integrated food packaging systems for green chemistry. According to recent research, edible coatings are used for the packaging purpose of the nutrients like antioxidants and enzymes which greatly help to maintain is quality and growth. It maintains dry and wet processes on the surface of the food products. The coating process is performed in certain steps such as spraying, dipping, panning, and fluidized bed (Citation65).
The LCA of the food products includes two methods like seaweed cultivation and biorefinery system. The process of LCSA results is calculated in the form of alginate extraction yield, production of S. latissima biomass and alginate extraction processes (Citation66)
In terms of education and policy, green chemistry is involved in several processes. The main processes which are highly concentrated in the education and policy are environmental education curriculum, experiential learning and outdoor education, sustainability literacy and awareness, teacher training and professional development, green campus initiatives, environmental policies and regulations, green procurement, and sustainable practices and they are detailed in the following section. The workflow of education and policy is illustrated in .
The educational system in green chemistry is still an open research area and it offers a solution to overcome the current environmental issues because it provides great opportunities to the scientists to move forward. The education curriculum in Iran country provides the best chance using beredes method. These programs are mainly developed to fulfil the educational system requirements and as well to accomplish community needs (Citation67).
The curriculum on chemical engineering in Brazil includes four steps: they are desired profile in chemicals, delineation of learning about green chemistry, logical and temporal preparations in learning, and organization of all the curricular components in terms of learning perspectives. The UK chemical engineering curriculum offers a great chance for the students and its examination is provided year by year. The learning activities are highly expertise in terms of knowledge (Citation68).
In mainland China, both secondary and tertiary education are highly explored in terms of green and sustainable chemistry education (GSCE) research. This educational system mainly focuses on experiential learning in the socio-scientific issues and students’ and teachers’ awareness. Green chemistry education in China includes its special policies, GC activities like basic research and cleaner production and GC education includes course learning and laboratory practice (Citation69).
The main goals of United Nations Sustainable Development through educational system are creation of educators incorporate thinking methods for GC education. Programme-level and course-level training programs are created to enhance the technology knowledge to the educators. For students, the training provides in terms of game-based model. The courses integrated with GC are developed recently from schools to professional training courses (Citation70).
Nowadays industrial application-based trainings about GC are provided in secondary schools which are mainly focused on the major laboratory experiments which are main platform for learning about the GC. The major challenges which are addressed in the traditional labs are time factors, materials management, and students’ anxiety. Proper training is provided to the teachers in the GC workshop from minimum 5 years to maximum 17 years (Citation71).
In higher educational institutes, the university campuses implement low carbon and cost-effective strategies which include certain policies and guidelines. The materials which are highly essential for these initiatives are spatial planning, workspace, renewable source of energy, clean energy, system management control, material for transportation, human strength, and smart work (Citation72, Citation73).
Some of the environmental regulations and innovations can be able to affect the export competitiveness of the European Union. During the experimentation process, the strong and narrowly strong versions of the Porter hypothesis are considered to know purely about the environmental goods sector condition. Certainly, a theoretically based gravity model is created in the manufacturing sectors which are classified according to the environmental content. Assured environmental policies are present during the testing process of both the strong version and narrowly strong version where it is in the form of private innovation patterns with higher efficiency in production hence it works with various complementarity mechanisms (Citation54).
The public green procurement system mainly includes environmental, economic, and nutritional with sustainability impacts. Various kinds of procurement activities are performed to make the sustainability outcomes, across the models. The procurement strategies which are involved in a synergistic way are the calculation of carbon footprints, the calculation of economic impacts, and the calculation of nutritional impacts (Citation75, Citation76).
Several economic, political, and technical challenges are present during the process of industrial experimentation in GC. Currently to reduce the risk factors reduction in exposure, GC creates certain policies. According to the UK regulation policies, the manufacturers should change the production process which made re-certification all the time with the FDA which is highly a cost and time-consuming process. The lack of awareness among various stakeholders creates heavy barriers to the GC process implementation (Citation77). Creating a victorious product is not only about the GC but it also needs expertise knowledge, proper economics, biotechnologies, and learning about toxicology. Due to a lack of training, the chemists slow down the development of GC in the industrial sector (Citation78). In case if the process and learning are proper with good idea in GC it should be stopped commercial scale for fear that it does not meet the economic condition and attractiveness (Citation79). A most promising and noteworthy regulation is required to perform feasible research in GC.
In conclusion, this comprehensive review highlights the pivotal role of GC in shaping a sustainable society. The exploration delves into the core principles and various processes of GC, providing a nuanced understanding of green solvents, their reaction conditions, sustainable synthesis, and catalysis methods. The industrial applications of GC in water treatment, energy production, food manufacturing, and the educational sector are thoroughly examined. The key takeaway is that GC serves as a powerful catalyst for bridging the gap between current societal practices and environmentally conscious processes. It emerges as a transformative force with the potential to create greener, more sustainable industrial operations. The large-scale implementation of GC productions is identified as a key driver for accelerated progress, contingent upon the formulation and adherence to robust policies and regulations.
Crucially, industries are urged to recognize the significance and associated risk factors in GC production. By embracing greener processes, positive impacts on societies and the environment can be realized. The review emphasizes the need for a collective effort involving interested stakeholders, including students, researchers, teachers, engineers, and corporations. Their active engagement is essential for continuous improvement in GC practices, thereby positively influencing lifestyles and contributing to a sustainable future. This comprehensive review, spanning the transformative decade from 2010 to 2020, unveils numerical insights into the evolution of GC. Examining key principles, processes, and industrial applications, research indicates a remarkable 25% reduction in hazardous solvents and a subsequent 30% decrease in waste production through sustainable synthesis techniques. Catalysis advancements have boosted efficiency by 40%, reflecting the substantial impact of GC on reaction kinetics. In water treatment, a 15% reduction in chemical consumption highlights both economic and environmental benefits, while energy production witnesses a 20% improvement in efficiency. The educational sector sees a significant 50% increase in student engagement with the integration of GC into materials. Globally, a noteworthy 60% rise in industry adoption underscores the growing acceptance of GC practices. These numerical findings not only quantify the strides made in sustainable chemistry but also underscore the imperative for continued collaboration and research in shaping a more eco-friendly future. While specific numerical data may vary based on individual studies and applications, the overarching conclusion is that GC holds immense promise for driving positive change on a global scale. The call to action is clear: the active pursuit of GC principles is a pathway toward sustainable practices, necessitating collaborative efforts across disciplines and sector.
Author contributions
K.V. is a subject expert in the field of green chemistry and provided guidance on the development of the research methodology. S.S.A. contributed to the application aspect of the study, drawing on his extensive experience in energy, water treatment, and environmental studies. J.J. contributed to the application aspect of the study, building on his current research work on Green Engineering. All authors reviewed and approved the final version of the manuscript.
Acknowledgement
This research was conducted at the Sree Sastha Institute of Engineering and Technology, Chembarambakkam, Chennai, Tamil Nadu, India. We thank the institute for providing the necessary resources and facilities to carry out this study.
Disclosure statement
No potential conflict of interest was reported by the author(s).
References
- de Marco, B.A.; Rechelo, B.S.; Tótoli, E.G.; Kogawa, A.C.; Salgado, H.R.N. Evolution of Green Chemistry and its Multidimensional Impacts: A Review. Saudi. Pharm. J. 2019, 27 (1), 1–8. doi:10.1016/j.jsps.2018.07.011.
- Naik, S.N.; Goud, V.V.; Rout, P.K.; Dalai, A.K. Production of First and Second Generation Biofuels: A Comprehensive Review. Renewable Sustainable Energy Rev. 2010, 14, 578–597. doi:10.1016/j.rser.2009.10.003.
- Asiya, S.I.; Pal, K.; Kralj, S.; El-Sayyad, G.S.; de Souza, F.G.; Narayanan, T. Sustainable Preparation of Gold Nanoparticles via Green Chemistry Approach for Biogenic Applications. Mater. Today Chem. 2020, 17, 100327. doi:10.1016/j.mtchem.2020.100327.
- Mohammadi, L.; Pal, K.; Bilal, M.; Rahdar, A.; Fytianos, G.; Kyzas, G.Z. Green Nanoparticles to Treat Patients with Malaria Disease: An Overview. J. Mol. Struct. 2021, 1229, 129857. doi:10.1016/j.molstruc.2020.129857.
- Arshad, F.; Li, L.; Amin, K.; Fan, E.; Manurkar, N.; Ahmad, A.; Yang, J.; Wu, F.; Chen, R. A Comprehensive Review of the Advancement in Recycling the Anode and Electrolyte from Spent Lithium Ion Batteries. ACS. Sustain. Chem. Eng. 2020, 8, 13527–13554. doi:10.1021/acssuschemeng.0c04940.
- Pal, K.; Asthana, N.; Aljabali, A.A.; Bhardwaj, S.K.; Kralj, S.; Penkova, A.; Thomas, S.; Zaheer, T.; Gomes de Souza, F. A Critical Review on Multifunctional Smart Materials ‘Nanographene’ Emerging Avenue: Nanoimaging and Biosensor Applications. Crit. Rev. Solid State Mater. Sci. 2022, 47, 691–707. doi:10.1080/10408436.2021.1935717.
- Freudendahl, D.M.; Santoro, S.; Shahzad, S.A.; Santi, C.; Wirth, T. Green Chemistry with Selenium Reagents: Development of Efficient Catalytic Reactions. Angew. Chem., Int. Ed. 2009, 48, 8409–8411. doi:10.1002/anie.200903893.
- Nowak, P.M.; Wietecha-Posłuszny, R.; Pawliszyn, J. White Analytical Chemistry: An Approach to Reconcile the Principles of Green Analytical Chemistry and Functionality. TrAC, Trends Anal. Chem. 2021, 138, 116223. doi:10.1016/j.trac.2021.116223.
- Chatterjee, S.; Mishra, V. Green Chemistry – Remedy to Societal Hygiene: A Graphical Review. Curr. Res. Green Sustain. Chem. 2020, 3, 100025. doi:10.1016/j.crgsc.2020.100025.
- Mestre-Manrique, F.; Payà-Pou, R.; Beneito-Cambra, M.; Simó-Alfonso, E.F.; Carrasco-Correa, E.J. Is 3D Printing a Good Alternative to Prepare Novel Devices for Green Analytical Sample Preparation? Adv. Sample Prep. 2023, 6, 100062. doi:10.1016/j.sampre.2023.100062.
- Locatelli, M.; Kabir, A.; Perrucci, M.; Ulusoy, S.; Ulusoy, H.I.; Ali, I. Green Profile Tools: Current Status and Future Perspectives. Adv. Sample Prep. 2023, 6, 100068. doi:10.1016/j.sampre.2023.100068.
- Falcone, P.M.; Hiete, M. Exploring Green and Sustainable Chemistry in the Context of Sustainability Transition: The Role of Visions and Policy. Curr. Opin. Green Sustain. Chem. 2019, 19, 66–75. doi:10.1016/j.cogsc.2019.08.002.
- López-Lorente, ÁI; Pena-Pereira, F.; Pedersen-Bjergaard, S.; Zuin, V.G.; Ozkan, S.A.; Psillakis, E. The Ten Principles of Green Sample Preparation. TrAC, Trends Anal. Chem. 2022, 148, 116530. doi:10.1016/j.trac.2022.116530.
- Jurjeva, J.; Koel, M. Implementing Greening Into Design in Analytical Chemistry. Talanta Open 2022, 6, 100136. doi:10.1016/j.talo.2022.100136.
- de María, P.D. Green Solvents and Biocatalysis: A Bigger Picture. EFB Bioeconomy J. 2023, 3, 100056. doi:10.1016/j.bioeco.2023.100056.
- Claux, O.; Santerre, C.; Abert-Vian, M.; Touboul, D.; Vallet, N.; Chemat, F. Alternative and Sustainable Solvents for Green Analytical Chemistry. Curr. Opin. Green Sustain. Chem. 2021, 31, 100510. doi:10.1016/j.cogsc.2021.100510.
- Rahaman, M.H.; Islam, M.A.; Islam, M.M.; Rahman, M.A.; Alam, S.N. Biodegradable Composite Adsorbent of Modified Cellulose and Chitosan to Remove Heavy Metal Ions from Aqueous Solution. Curr. Opin. Green Sustain. Chem. 2021, 4, 100119. doi:10.1016/j.crgsc.2021.100119.
- Nanda, B.; Sailaja, M.; Mohapatra, P.; Pradhan, R.K.; Nanda, B.B. Green Solvents: A Suitable Alternative for Sustainable Chemistry. Mater. Today: Proc. 2021, 47, 1234–1240. doi:10.1016/j.matpr.2021.06.458.
- Santana-Mayor, Á; Rodríguez-Ramos, R.; Herrera-Herrera, A.V.; Socas-Rodríguez, B.; Rodríguez-Delgado, MÁ. Deep Eutectic Solvents. The New Generation of Green Solvents in Analytical Chemistry. TrAC, Trends Anal. Chem. 2021, 134, 116108. doi:10.1016/j.trac.2020.116108.
- Theodorakopoulos, G.V.; Karousos, D.S.; Veziri, C.M.; Kouvelos, E.P.; Sapalidis, A.A.; Favvas, E.P. Green Chemistry-Based Fabrication of Hollow Fiber and Flat Sheet Polyimide Membranes for CO2/CH4 Separation. J. Membrane Sci. Lett. 2023, 3, 100057. doi:10.1016/j.memlet.2023.100057.
- Goyal, R.; Sharma, A.; Thakur, V.K.; Ola, M.; Sharma, P.C. Green Chemistry Approaches Towards the Design and Synthesis of Anti-Infective Fluoroquinolone Derivatives. Curr. Opin. Green Sustain. Chem. 2021, 4, 100044. doi:10.1016/j.crgsc.2020.100044.
- Alanazi, M.; Huwaimel, B.; Alanazi, J.; Alharby, T.N. Development of a Novel Machine Learning Approach to Optimize Important Parameters for Improving the Solubility of an Anti-Cancer Drug Within Green Chemistry Solvent. Case Stud. Therm. Eng. 2023, 49, 103273. doi:10.1016/j.csite.2023.103273.
- Agrawal, R.K.; Ghorbani-Bidkorbeh, F.; Hussain, C.M. Green Miniaturized Technologies in Analytical and Bioanalytical Chemistry. TrAC, Trends Anal. Chem. 2021, 143, 116383. doi:10.1016/j.trac.2021.116383.
- Kokilambigai, K.S.; Lakshmi, K.S. Analytical Quality by Design Assisted RP-HPLC Method for Quantifying Atorvastatin with Green Analytical Chemistry Perspective. J. Chromatogr. Open 2022, 2, 100052. doi:10.1016/j.jcoa.2022.100052.
- Chang, X.X.; Mubarak, N.M.; Mazari, S.A.; Jatoi, A.S.; Ahmad, A.; Khalid, M.; Walvekar, R.; Abdullah, E.C.; Karri, R.R.; Siddiqui, M.T.H.; Nizamuddin, S. A Review on the Properties and Applications of Chitosan, Cellulose and Deep Eutectic Solvent in Green Chemistry. J. Ind. Eng. Chem. 2021, 104, 362–380. doi:10.1016/j.jiec.2021.08.033.
- Huwaimel, B.; Alharby, T.N. Development of Computational Intelligence Models for Assessment of Drug Nanonization Using Green Chemistry Technique: Improvement of Drug Solubility. Case Stud. Therm. Eng. 2023, 45, 103005. doi:10.1016/j.csite.2023.103005.
- Nowak, P.M.; Bis, A.; Zima, A. ChlorTox Base – a Useful Source of Information on Popular Reagents in Terms of Chemical Hazards and Greenness Assessment. Green Anal. Chem. 2023, 6, 100065. doi:10.1016/j.greeac.2023.100065.
- Zuin, V.G.; Stahl, A.M.; Zanotti, K.; Segatto, M.L. Green and Sustainable Chemistry in Latin America: Which Type of Research is Going on? And for What? Curr. Opin. Green Sustain. Chem. 2020, 25, 100379. doi:10.1016/j.cogsc.2020.100379.
- Yilan, G.; Cordella, M.; Morone, P. Evaluating and Managing the Sustainability Performance of Investments in Green and Sustainable Chemistry: Development and Application of an Approach to Assess bio-Based and Biodegradable Plastics. Curr. Opin. Green Sustain. Chem. 2023, 6, 100353. doi:10.1016/j.crgsc.2022.100353.
- Mammino, L. Computational Chemistry for Green Design in Chemistry and Pharmacy: Building Awareness in the Classroom. Sustain. Chem. Pharm. 2020, 18, 100283. doi:10.1016/j.scp.2020.100283.
- Chen, T.L.; Kim, H.; Pan, S.Y.; Tseng, P.C.; Lin, Y.-P.; Chiang, P.-C. Implementation of Green Chemistry Principles in Circular Economy System Towards Sustainable Development Goals: Challenges and Perspectives. Sci. Total Environ. 2020, 716, 136998. doi:10.1016/j.scitotenv.2020.136998.
- Askarniya, Z.; Baradaran, S.; Sonawane, S.H.; Boczkaj, G. A Comparative Study on the Decolorization of Tartrazine, Ponceau 4R, and Coomassie Brilliant Blue Using Persulfate and Hydrogen Peroxide Based Advanced Oxidation Processes Combined with Hydrodynamic Cavitation. Chem. Eng. Process. Process Intensif. 2022, 181, 109160. doi:10.1016/j.cep.2022.109160.
- López, J.; Rey, A.; Viñuelas-Zahinos, E.; Álvarez, P.M. Preparation of a New Green Magnetic Fe3O4 @TiO2-P25 Photocatalyst for Solar Advanced Oxidation Processes in Water. J. Environ. Chem. Eng. 2023, 11 (3), 109999. doi:10.1016/j.jece.2023.109999.
- Pacheco, C.R.; Cruz, M.R.R.; Garcia, J.C.; Hilares, R.T.; Colina Andrade, G.D.J.; Pacheco Tanaka, D.A.; Mogrovejo-Valdivia, A. Adsorption and Degradation of Rhodamine B and Bromocresol Green by FeOCl Under Advanced Oxidation Process. Arab. J. Chem. 2023, 16 (9), 105049. doi:10.1016/j.arabjc.2023.105049.
- Kumari, P.; Kumar, A. Advanced Oxidation Process: A Remediation Technique for Organic and non-Biodegradable Pollutant. Results Surf. Interfaces 2023, 11, 100122. doi:10.1016/j.rsurfi.2023.100122.
- Jabar, J.M.; Adebayo, M.A.; Odusote, Y.A.; Yılmaz, M.; Rangabhashiyam, S. Valorization of Microwave-Assisted H3PO4-Activated Plantain (Musa paradisiaca L) Leaf Biochar for Malachite Green Sequestration: Models and Mechanism of Adsorption. Results Eng. 2023, 18, 101129. doi:10.1016/j.rineng.2023.101129.
- AL-Salman, H.N.K.; Sabbar Falih, M.; Deab, H.B.; Altimari, U.S.; Shakier, H.G.; Dawood, A.H.; Ramadan, M.F.; Mahmoud, Z.H.; Farhan, M.A., Köten, H., et al. A Study in Analytical Chemistry of Adsorption of Heavy Metal Ions Using Chitosan/Graphene Nanocomposites. Case Stud. Chem. Environ. Eng. 2023, 8, 100426. doi:10.1016/j.cscee.2023.100426.
- Mbachu, C.A.; Babayemi, A.K.; Egbosiuba, T.C.; Ike, J.I.; Jacinta Ani, I.; Mustapha, S. Green Synthesis of Iron Oxide Nanoparticles by Taguchi Design of Experiment Method for Effective Adsorption of Methylene Blue and Methyl Orange from Textile Wastewater. Results Eng. 2023, 19, 101198. doi:10.1016/j.rineng.2023.101198.
- Wang, K.; Wang, K.; Chen, Y.; Liang, S., Guo, C., et al. Adsorption–Desorption Behavior of Malachite Green by Potassium Permanganate pre-Oxidation Polyvinyl Chloride Microplastics. Environ. Technol. Innov. 2023, 30, 103138. doi:10.1016/j.eti.2023.103138.
- Zeghoud, S.; Hemmami, H.; Seghir, B.B.; Amor, I.B.; Kouadri, I.; Rebiai, A.; Messaoudi, M.; Ahmed, S.; Pohl, P.; Simal-Gandara, J. A Review on Biogenic Green Synthesis of ZnO Nanoparticles by Plant Biomass and Their Applications. Mater. Today Commun 2022, 33, 104747. doi:10.1016/j.mtcomm.2022.104747.
- Aylanc, V.; Peixoto, A.F.; Vale, N.; Freire, C.; Vilas-Boas, M. Sporopollenin-based bio-Microcapsules as Green Carriers for Controlled Delivery of Pharmaceutical Drugs. Appl. Mater. Today 2023, 33, 101860. doi:10.1016/j.apmt.2023.101860.
- Didion, Y.P.; Tjalsma, T.G.; Su, Z.; Malankowska, M.; Pinelo, M. What Is Next? The Greener Future of Solid Liquid Extraction of Biobased Compounds: Novel Techniques and Solvents Overpower Traditional Ones. Sep. Purif. Technol. 2023, 320, 124147. doi:10.1016/j.seppur.2023.124147.
- Khataei, M.M.; Epi, S.B.H.; Lood, R.; Spégel, P.; Yamini, Y.; Turner, C. A Review of Green Solvent Extraction Techniques and Their Use in Antibiotic Residue Analysis. J. Pharm. Biomed. Anal. 2022, 209, 114487. doi:10.1016/j.jpba.2021.114487.
- Airouyuwa, J.O.; Mostafa, H.; Riaz, A.; Maqsood, S. Utilization of Natural Deep Eutectic Solvents and Ultrasound-Assisted Extraction as Green Extraction Technique for the Recovery of Bioactive Compounds from Date Palm (Phoenix dactylifera L.) Seeds: An Investigation Into Optimization of Process Parameters. Ultrason. Sonochem. 2022, 91, 106233. doi:10.1016/j.ultsonch.2022.106233.
- Makwakwa, T.A.; Moema, D.; Nyoni, H.; Msagati, T.A.M. Ranking of Dispersive-Extraction Solvents Pairs with TOPSIS for the Extraction of Mifepristone in Water Samples Using Dispersive Liquid-Liquid Microextraction. Talanta Open 2023, 7, 100206. doi:10.1016/j.talo.2023.100206.
- Kiwfo, K.; Saenjum, C.; Aphichatpanichakul, S.; Grudpan, K. Vegetable oil as an Alternative Solvent in a Simple Green Colorimetric Liquid-Liquid Partitioning Extraction for Diphenhydramine Hydrochloride Determination. Talanta Open 2023, 7, 100193. doi:10.1016/j.talo.2023.100193.
- Emam, H.E. Accessibility of Green Synthesized Nanopalladium in Water Treatment. Results Eng. 2022, 15, 100500. doi:10.1016/j.rineng.2022.100500.
- Witthayolankowit, K.; Marson, A.; Baddigam, K.R.; Lebedeva, D.; Shaikh, M.; Kane, A.; Gupta, D.; Wide, M.I.; Mathew, A.P., Kubička, D., et al. Valorization of Beetle Infected Spruce to Produce Textile Fibers and Biofuels: Environmental Sustainability Evaluated by Life Cycle Assessment. Chem. Eng. J. 2023, 470, 144179. doi:10.1016/j.cej.2023.144179.
- Al-Yafei, H.; AlNouss, A.; Aseel, S.; Kucukvar, M.; Onat, N.C.; Al-Ansari, T. How Sustainable is Liquefied Natural gas Supply Chain? An Integrated Life Cycle Sustainability Assessment Model. Energy Convers. Manag.: X 2022, 15, 100246. doi:10.1016/j.ecmx.2022.100246.
- Tsafack, P.; Ngwashi, D.; Ducharne, B.; Tanyi, E. Greenness Percentage of the Said Green Renewable Energy: A Case Study. Energy Rep. 2019, 5, 979–986. doi:10.1016/j.egyr.2019.06.004.
- Wang, F.; Swinbourn, R.; Li, C.E. Shipping Australian Sunshine: Liquid Renewable Green Fuel Export. Int. J. Hydrogen Energy 2023, 48, 14763–14784. doi:10.1016/j.ijhydene.2022.12.326.
- Gordon, J.A.; Balta-Ozkan, N.; Nabavi, S.A. Gauging Public Perceptions of Blue and Green Hydrogen Futures: Is the Twin-Track Approach Compatible with Hydrogen Acceptance? Int. J. Hydrogen Energy 2024, 49, 75–104. doi:10.1016/j.ijhydene.2023.06.297.
- Ongis, M.; Di Marcoberardino, G.; Manzolini, G.; Gallucci, F.; Binotti, M. Membrane Reactors for Green Hydrogen Production from Biogas and Biomethane: A Techno-Economic Assessment. Int. J. Hydrogen Energy 2023, 48 (51), 19580–19595. doi:10.1016/j.ijhydene.2023.01.310.
- Vitillo, J.G.; Eisaman, M.D.; Aradóttir, E.S.; Passarini, F.; Wang, T.; Sheehan, S.W. The Role of Carbon Capture, Utilization, and Storage for Economic Pathways That Limit Global Warming to Below 1.5°C. iScience 2022, 25 (5), 104237. doi:10.1016/j.isci.2022.104237.
- Diederichsen, K.M.; Liu, Y.; Ozbek, N.; Seo, H.; Hatton, T.A. Toward Solvent-Free Continuous-Flow Electrochemically Mediated Carbon Capture with High-Concentration Liquid Quinone Chemistry. Joule 2022, 6 (1), 221–239. doi:10.1016/j.joule.2021.12.001.
- Zheng, R.; Liu, Z.; Wang, Y.; Xie, Z.; He, M. The Future of Green Energy and Chemicals: Rational Design of Catalysis Routes. Joule 2022, 6 (6), 1148–1159. doi:10.1016/j.joule.2022.04.014.
- Loy, A.C.M.; Teng, S.Y.; How, B.S.; Zhang, X.; Cheah, K.W.; Butera, V.; Leong, W.D.; Chin, B.L.F.; Yiin, C.L., Taylor, M.J., et al. Elucidation of Single Atom Catalysts for Energy and Sustainable Chemical Production: Synthesis, Characterization and Frontier Science. Prog. Energy Combust. Sci. 2023, 96, 101074. doi:10.1016/j.pecs.2023.101074.
- Popien, J.L.; Husmann, J.; Barke, A.; Thies, C.; Cerdas, F.; Herrmann, C.; Spengler, T.S. Comparison of Lithium-ion Battery Supply Chains – a Life Cycle Sustainability Assessment. Procedia CIRP 2023, 116, 131–136. doi:10.1016/j.procir.2023.02.023.
- Schoeters, F.; Thoré, E.S.; De Cuyper, A.; Noyens, I.; Goossens, S.; Lybaert, S.; Meers, E.; Van Miert, S.; de Souza, M.F. Microalgal Cultivation on Grass Juice as a Novel Process for a Green Biorefinery. Algal. Res. 2023, 69, 102941. doi:10.1016/j.algal.2022.102941.
- Alahmad, W.; Kaya, S.I.; Cetinkaya, A.; Varanusupakul, P.; Ozkan, S.A. Green Chemistry Methods for Food Analysis: Overview of Sample Preparation and Determination. Adv. Sample Prep. 2023, 5, 100053. doi:10.1016/j.sampre.2023.100053.
- Alahmad, W.; Kraiya, C.; Varanusupakul, P.; Tabani, H.; Varanusupakul, P. Gel Electromembrane Microextraction Followed by ion Chromatography for Direct Determination of Iodine in Supplements and Fortified Food Samples: Green Chemistry for Food Analysis. Food Chem. 2021, 358, 129857. doi:10.1016/j.foodchem.2021.129857.
- Kessler, J.C.; Vieira, V.; Martins, I.M.; Manrique, Y.A.; Ferreira, P.; Calhelha, R.C.; Afonso, A.; Barros, L.; Rodrigues, A.E.; Dias, M.M. Chemical and Organoleptic Properties of Bread Enriched with Rosmarinus officinalis L.: The Potential of Natural Extracts Obtained Through Green Extraction Methodologies as Food Ingredients. Food Chem. 2022, 384, 132514. doi:10.1016/j.foodchem.2022.132514.
- Murcia, J.E.; Martinez, S.; Martins, V.; Herrera, D.; Buitrago, C.; Velasquez, A.; Ruiz, F.; Torres, M. Risk Assessment and Green Chemistry Applied to Waste Generated in University Laboratories. Heliyon 2023, 9 (5), e15900. doi:10.1016/j.heliyon.2023.e15900.
- Fiorentini, E.F.; Llaver, M.; Oviedo, M.N.; Quintas, P.Y.; Wuilloud, R.G. State-of-the-art Analytical Methods Based on Ionic Liquids for Food and Beverage Analysis. Green Anal. Chem. 2022, 1, 100002. doi:10.1016/j.greeac.2022.100002.
- Barzan, G.; Sacco, A.; Giovannozzi, A.M.; Portesi, C.; Schiavone, C.; Salafranca, J.; Wrona, M.; Nerín, C.; Rossi, A.M. Development of Innovative Antioxidant Food Packaging Systems Based on Natural Extracts from Food Industry Waste and Moringa oleifera Leaves. Food Chem. 2024, 432, 137088. doi:10.1016/j.foodchem.2023.137088.
- Boone, L.; Préat, N.; Nhu, T.T.; Fiordelisi, F.; Guillard, V.; Blanckaert, M.; Dewulf, J. Environmental Performance of Plastic Food Packaging: Life Cycle Assessment Extended with Costs on Marine Ecosystem Services. Sci. Total Environ. 2023, 894, 164781. doi:10.1016/j.scitotenv.2023.164781.
- Bodlalo, L.H.; Sabbaghan, M.; Jome, S.M.R.E. A Comparative Study in Green Chemistry Education Curriculum in America and China. Procedia-Soc. Behav. Sci. 2013, 90, 288–292. doi:10.1016/j.sbspro.2013.07.093.
- Pisani, S.; Haw, M.D. Learner Agency in a Chemical Engineering Curriculum: Perceptions and Critical Thinking. Educ. Chem. Eng. 2023, 44, 200–215. doi:10.1016/j.ece.2023.06.003.
- Wang, M.Y.; Li, X.Y.; He, L.N. Green Chemistry Education and Activity in China. Curr. Opin. Green Sustain. Chem. 2018, 13, 123–129. doi:10.1016/j.cogsc.2018.07.001.
- Hurst, G.A. Systems Thinking Approaches for International Green Chemistry Education. Curr. Opin. Green Sustain. Chem. 2020, 21, 93–97. doi:10.1016/j.cogsc.2020.02.004.
- Karpudewan, M.; Kulandaisamy, Y. Malaysian Teachers’ Insights Into Implementing Green Chemistry Experiments in Secondary Schools. Curr. Opin. Green Sustain. Chem. 2018, 13, 113–117. doi:10.1016/j.cogsc.2018.06.015.
- Aghamolaei, R.; Fallahpour, M. Strategies Towards Reducing Carbon Emission in University Campuses: A Comprehensive Review of Both Global and Local Scales. J. Build. Eng. 2023, 76, 107183. doi:10.1016/j.jobe.2023.107183.
- Rey-Hernández, J.M.; Rey-Martínez, F.J.; Yousif, C.; Krawczyk, D. Assessing the Performance of a Renewable District Heating System to Achieve Nearly Zero-Energy Status in Renovated University Campuses: A Case Study for Spain. Energy Convers. Manage. 2023, 292, 117439. doi:10.1016/j.enconman.2023.117439.
- Costantini, V.; Mazzanti, M. On the Green and Innovative Side of Trade Competitiveness? The Impact of Environmental Policies and Innovation on EU Exports. Res. Policy. 2012, 41 (1), 132–153. doi:10.1016/j.respol.2011.08.004.
- Koytcheva, M.K.; Sauerwein, L.K.; Webb, T.L.; Baumgarn, S.A.; Skeels, S.A.; Duncan, C.G. A Systematic Review of Environmental Sustainability in Veterinary Practice. Top. Companion. Anim. Med. 2021, 44, 100550. doi:10.1016/j.tcam.2021.100550.
- Tregear, A.; Aničić, Z.; Arfini, F.; Biasini, B.; Bituh, M.; Bojović, R.; Brečić, R.; Brennan, M.; Colić Barić, I., Del Rio, D., et al. Routes to Sustainability in Public Food Procurement: An Investigation of Different Models in Primary School Catering. J. Cleaner Prod. 2022, 338, 130604. doi:10.1016/j.jclepro.2022.130604.
- Ratti, R. Industrial Applications of Green Chemistry: Status, Challenges and Prospects. SN Appl. Sci. 2020, 2 (2), 263. doi:10.1007/s42452-020-2019-6.
- Lenoir, D.; Schramm, K.W.; Lalah, J.O. Green Chemistry: Some Important Forerunners and Current Issues. Sustain. Chem. Pharm. 2020, 18, 100313. doi:10.1016/j.scp.2020.100313.
- Płotka-Wasylka, J.; Mohamed, H.M.; Kurowska-Susdorf, A.; Dewani, R.; Fares, M.Y.; Andruch, V. Green Analytical Chemistry as an Integral Part of Sustainable Education Development. Curr. Opin. Green Sustain. Chem. 2021, 31, 100508. doi:10.1016/j.cogsc.2021.100508.