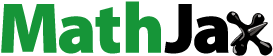
ABSTRACT
Baicalein, usually obtained after the removal of 7-O-β-D-glucuronic acid from baicalin, has strong pharmacological activity. In this study, a novel strategy for efficient and eco-friendly preparation of baicalein was established by high-temperature-little-acid hydrolysis of baicalin. The effects of inorganic acids, solvents, solid–liquid ratio, HCl concentration and hydrolysis duration on the yield of baicalein were investigated by one-factor-at-a-time experiment. Then, an orthogonal experiment design was conducted for the optimal hydrolysis conditions, namely, baicalin was boiled in n-BuOH solution containing 0.50% HCl (w/v) at 130°C for 2.0 h with solid–liquid ratio at 1:25 (g/mL). In the 60-fold scale-up experiment, the yield of baicalein was achieved at 83.5% ± 6.3%, and its purity was increased from 74.2% to 98.7% after purification. Compared with reported methods, acid consumption per unit product of the newly developed strategy was approximately 80 times lower than that of conventional acid hydrolysis, and time consumption was substantially reduced. Overall, the novel strategy showed great prospects for a cleaner large-scale production of baicalein, which provides a new alternative desirable for the preparation of natural products with high efficiency and low consumption in the pharmaceutical industry.
1. Introduction
Scutellariae Radix is the dried root of Scutellaria baicalensis Georgi (Fam. Lamiaceae), which is native to China, Russia, Mongolia, North Korea, and Japan and is widely distributed in these regions nowadays [Citation1,Citation2]. It is one of frequently used herbal medicines for treating colds, fever, diarrhea, jaundice, headache, abdominal pain, and drenching, etc. in clinical practices [Citation3]. Scutellariae Radix is well-known for its functions to clear away heat and dampness, as well as purge fire and detoxify, and this herb has been recorded in the prevailing ‘Chinese Pharmacopoeia (2020 edition),’ ‘British Pharmacopoeia (2023 edition),’ ‘European Pharmacopoeia (11.0 edition)' and ‘Japanese Pharmacopoeia (18th edition)' as an essential herbal medicine [Citation1,Citation4–6].
Flavonoids are the main active constituents of S. baicalensis, which have been widely studied in recent decades [Citation7]. In particular, more than thirty flavonol glycosides have been isolated, including baicalin (CAS: 19057-67-1), wogonoside (CAS: 51059-44-0), scutellarin (CAS: 27740-01-8) and oroxylin A-7-O-glucuronide (CAS: 36948-76-2) [Citation2,Citation8,Citation9]. Among them, baicalin has a much higher content than others and possesses various beneficial effects, including anti-inflammatory [Citation10–12], antioxidant [Citation13–15], antibacterial [Citation16], antiviral [Citation17], anticancer [Citation18–20], and antidepressant activities [Citation21].
In 1922, a trace amount of baicalein (CAS: 491-67-8) was isolated from S. baicalensis for the first time [Citation22]. Further research has shown that baicalein has various pharmacological properties, including neuroprotective [Citation23,Citation24], hepatoprotective [Citation25], antiviral [Citation26] and anti-asthmatic [Citation27] effects. According to recent investigations, baicalein could relieve myocardial injury by suppressing harmful changes caused by lipid peroxidation in cardiomyocytes [Citation28]. However, the content of baicalein in S. baicalensis is extremely low, ranging from only 0.04% to 0.28% naturally, and its yield from raw material by direct extraction was very limited [Citation29–31].
Baicalein can be derived from baicalin by the removal of one glucuronic acid after hydrolysis. The conversion to baicalein largely enhanced its lipid solubility and hydrophobicity, which made it easier to cross gastric mucosa [Citation32,Citation33]. It should be noted that baicalin can only be absorbed in the lower part of the intestine after hydrolysis to baicalein, whereas baicalein showed a broader absorption range throughout the gastrointestinal tract [Citation34,Citation35]. Additionally, baicalein exhibited superior pharmacological activities compared with its original glycoside baicalin [Citation36–39]. On the other hand, baicalin is the most abundant flavonol glycoside in S. baicalensis with a content exceeding 9% (w/w) as required by the prevailing China Pharmacopoeia (2020 edition), the European Pharmacopoeia (11.0 edition) and the British Pharmacopoeia (2023 edition), and even not less than 10% (w/w) by the Japanese Pharmacopoeia (18th edition) [Citation1,Citation4–6]. Furthermore, it was well recognized that a complete hydrolysis of baicalin can help greatly increase the yield of baicalein and reduce production costs [Citation29].
Conventional approaches have been studied for the effective conversion of baicalin or other natural products, including thermal cracking, microbial transformation, enzymatic hydrolysis, and acid hydrolysis. For instance, thermal cracking offered a fast reaction rate, however, accurate temperature manipulation was a challenging operation while the reaction was taking place in a muffle furnace. Consequently, poor experimental reproducibility and large experimental errors have been caused and difficulties in industrialization were to be resolved [Citation40]. Microbial transformation, despite its relatively high specificity and eco-friendliness, usually requires a large time consumption, typically ranging from 5 to 8 days [Citation41,Citation42]. Meanwhile, the purification step of the desired product is always complicated and tedious. Enzymatic hydrolysis, known for its mild reaction conditions and high product quality, suffers from slow reaction rates and relatively high production costs, which much hindered its industrial-scale implementation [Citation43–48]. In addition, conventional acid hydrolysis adopted an aqueous solution of an inorganic acid as the solvent, and a high acid concentration was usually required due to the low boiling point (ca. 100°C) of the reaction system. As a result, significant environmental pollution could be caused unless the acidic liquid waste has been carefully handled [Citation49]. Moreover, strong acids substantially degraded the chemical structure of baicalin, often leading to a low yield [Citation40, Citation50].
To address these challenges, a novel acidic hydrolysis system has been proposed in the current study. The novelty lies in that an organic solvent of high boiling point was used as the solvent instead of water to increase reaction temperature and consequently only a little of inorganic acid was needed as the catalyst. This approach aimed to reduce the consumption of acids in large-scale production processes and achieve efficient conversion, paving a sustainable way to obtain bioactive flavonoid aglycones with high efficiency and low consumption in the pharmaceutical industry.
2. Materials and methods
2.1. Materials and reagents
Baicalin (purity was 92.0% by HPLC-UV, B/N: 21050603) was offered by Chengdu Pufei De Biotech Co., Ltd. (Chengdu, China). Baicalein (purity ≥ 98.9% by HPLC-UV, B/N: C06M11Y112461) was purchased from Shanghai Yuanye Bio-Technology Co., Ltd. (Shanghai, China). HPLC-grade methanol (MeOH, B/N: 220151019M08) was obtained from OmniGene LLC (United States). n-Butanol (n-BuOH, B/N: F2130089) of analytical grade was supplied by Shanghai Aladdin Biochemical Technology Co., Ltd. (Shanghai, China). Hydrochloric acid (HCl, B/N: 20160719), sulfuric acid (H2SO4, B/N: 20180223), 1-pentanol (B/N: 20220607), ethanol absolute (EtOH, B/N: 20220414) and phosphoric acid (H3PO4, B/N: 20130503) were of analytical grade and purchased from Sinopharm Chemical Reagent Co., Ltd. (Shanghai, China). Two macroporous resins D101 and DM130 were bought from Sunresin New Materials Co., Ltd. (Xi’an, China). Ultrapure water (>18.2 MΩ·cm−1) was prepared using a HealForce water purification system (China).
2.2. HPLC conditions
An Agilent 1100 HPLC chromatograph equipped with G1313A autosampler, G1312A pump, G1316A column oven, G1315A diode array detector, and G1322A degasser was used for chromatographic analysis. Data acquisition and post-run processing were performed using HP ChemStation software (Rev.A.07.01[682]). The HPLC analysis was conducted according to the reported conditions with minor modifications [Citation51].
A Waters SunFire C18 column (150 mm L. × 4.6 mm I.D., 5 μm) was used for separation of analytes in HPLC analysis. The injection volume was 5 μL, and the flow rate was set at 1.0 mL/min constantly. Column temperature was maintained at 35°C throughout the analysis, and UV wavelength at 280 nm was employed to monitor HPLC profile. Mobile phases consisted of MeOH (A) and 0.10% H3PO4 aqueous solution (B). Gradient elution program was as follows: 0 min, 57%A: 43%B → 10 min, 57%A: 43%B → 13 min, 70%A: 30%B → 23 min, 90%A: 10%B → 25 min, 90%A: 10% B→ 27 min, 57%A: 43%B → 30 min, 57%A: 43%B.
2.3. Preparation of standard solution
Twenty milligrams of baicalein reference were dissolved in MeOH to be 10 mL, then a stock solution with a concentration of 2.000 mg/mL was prepared. Subsequently, a series of dilutions were performed to obtain standard solutions with concentrations of 3.906 × 10−3, 7.812 × 10−3, 1.562 × 10−2, 3.125 × 10−2, 6.250 × 10−2, 1.250 × 10−1, 2.500 × 10−1, 5.000 × 10−1, and 1.000 mg/mL.
2.4. Preparation of sample solutions by high-temperature-little-acid hydrolysis
To prepare the sample solutions, 4 g of baicalin was accurately weighed and transferred in a round-bottomed flask. Then, 100 mL of acidic n-BuOH solution containing 0.50% HCl (w/v) was added. A heating bowl was heated to 130°C, and then a gentle boiling of the reaction system was observed. After being refluxed for 2.0 h, the system was cooled to ambient temperature. Prior to injection for HPLC analysis, a small portion of the resulting solution was filtered through a 0.22 μm syringe membrane. Determination of baicalein was conducted according to the method described as aforementioned.
2.5. Condition optimization for baicalein preparation
2.5.1. One-factor-at-a-time experiment
One-factor-at-a-time (OFAT) experiment was employed, in which the effects of five variables were investigated, namely inorganic acid (HCl or H2SO4), solvent (n-BuOH, 1-pentanol, pure water), solid–liquid ratio (1:50, 1:25, 1:16, 1:12, 1:10, 1:8, g/mL), HCl concentration (0.10%, 0.50%, 1.00%, 3.00%, w/v), and hydrolysis duration (0.5, 1.0, 1.5, 2.0, 2.5, 3.0, h). Each experiment was performed in triplicate, and the yield of baicalein and its average were calculated. The results from the OFAT experiments were used for the subsequent orthogonal design.
2.5.2. Orthogonal experimental design
Based on the results of OFAT experiments, three factors, namely solid–liquid ratio, HCl concentration and hydrolysis duration, were selected to design a four-factor, three-level L9(34) orthogonal experiment () to further optimize and investigate the significance of these factors on the yield of baicalein by means of range analysis and analysis of variance (ANOVA) through IBM® SPSS statistical software (version 23, IBM SPSS, Armonk, NY, U.S.A.).
Table 1. Factors and levels of L9(34) orthogonal experiment design.
2.6. Scale-up experiments
To further investigate the applicability of this newly developed technique in the pharmaceutical industry, a scale-up experiment was further performed for a 60-fold increase in the reaction volume from 100 mL to 6 L.
In the scale-up experiments, 240 g of baicalin was accurately weighed in round-bottom flasks, and 6 L of n-BuOH solution containing 0.50% HCl (w/v) was added and mixed. The mixture was heated to 130°C to a slight boiling state and then refluxed for 2.0 h. After cooling to room temperature, n-BuOH in the reaction solution was recycled under reduced pressure at 50°C. After suction filtration on filter paper, crude baicalein was washed with distilled water and then dried at 50°C for 20 h.
2.7. Purification of baicalein
Fifty grams of crude baicalein were dissolved in 30% EtOH (v/v). 150 mL of the sample solution was then loaded onto a glass column packed with DM130 resin. Gradient elution was performed using 30%, 40%, 60%, and 80% EtOH (v/v) (4 BV for each). Its effluent was concentrated and loaded onto another column filled with D101 resin. Elution was performed using 70% EtOH (v/v) at a constant flow rate (2 mL/min). The collected effluent was analyzed by HPLC-UV, and the fractions containing baicalein was lyophilized.
2.8. Identification of baicalein
The purified product was subjected to ESI + -MS, 1H-NMR, and 13C-NMR for structure identification. The NMR analysis was conducted on a Bruker Advance II 400 MHz spectrometer. The solution of the product was injected into a Shim-pack UFLC SHIMADZU CBM30A system in tandem with ESI-QQQ detector for MS data acquisition.
2.9. Calculations
The baicalein concentration (C, mg/mL) of the sample solution was calculated using the calibration curve based on its peak area. The yield of baicalein (Y, %) was calculated using the following equations:
(1)
(1) In the equations, V represents the volume of the reaction system in mL, D represents the dilution factor of sample solution, m represents the mass of baicalin added in acid hydrolysis in grams, ω represents the purity of baicalin (92.0%), M represents the molar mass of baicalein (M: 270.237 g/mol), and M" represents the molar mass of baicalin (M": 446.361 g/mol).
3. Results and discussion
3.1. One-factor-at-a-time experiment
3.1.1. Inorganic acid
The solid–liquid ratio of baicalin to n-BuOH in the reaction system was 1:25 (g/mL), with an acid concentration of 0.50% (w/v) and a hydrolysis duration of 2.0 h. The effect of inorganic acid (HCl or H2SO4) on the yield of baicalein was investigated. As shown in (a), the yield of baicalein was negligible when no acid was added to the reaction with n-BuOH, which confirms the necessity of acid usage during hydrolysis. The average yield of baicalein using HCl and H2SO4 as catalysts was 70.4% ± 3.3% and 62.0% ± 1.0%, respectively. The yield of baicalein with HCl as a catalyst was significantly higher than that of baicalein with H2SO4 as a catalyst (13.5%; P < 0.05). Therefore, HCl was selected as the catalyst to construct the novel high-temperature-low-acid hydrolysis system. In this reaction system, HCl provided an acidic environment, the 7-position glycosidic bond atom of baicalin was protonated, and the glycosidic bond was broken to form the aglycone baicalein and the cationic carbon ion intermediate of glucuronic acid, which was solvated in the solvent n-BuOH and then removes hydrogen ions to form glucuronic acid [Citation52,Citation53].
3.1.2. Solvent
n-BuOH or 1-pentanol containing HCl was used as the reaction solvent to reach a higher temperature than acidic aqueous solution, and the yield of baicalein was investigated and compared. As shown in (b), the yield of baicalein was only 1.5% ± 0.4% when pure water was used as the solvent with a low HCl concentration at 0.50%. Instead, when n-BuOH and 1-pentanol were used as reaction solvents, the average yield of baicalein was greatly increased to 67.8% ± 1.6% and 74.0% ± 6.5%, respectively. As the boiling point of n-BuOH (ca. 118°C) and 1-pentanol (ca. 137°C) is much higher than that of water, the reaction system composed of either one achieved a greater temperature, which largely promoted the hydrolysis of baicalin. Although the yield of baicalein was slightly higher when 1-pentanol was used as the reaction solvent other than n-BuOH, there was no significant difference between them (P > 0.05). Given the economical aspect, n-BuOH is more cost-effective than 1-pentanol as a reaction solvent.
3.1.3. Solid–liquid ratio
To optimize the solid–liquid ratio, various amounts of baicalin were mixed with 100 mL of acidic n-BuOH solution for hydrolysis and the results are shown in (c). When the solid–liquid ratio of baicalin to n-BuOH was changed from 1:50 to 1:25 or 1:16 (g/mL), the yield of baicalein slightly varied around 60% without significance (P > 0.05). A sufficient n-BuOH solution could have made the substrate baicalin dissolved fully, which ensured its complete and efficient hydrolysis. When the solid–liquid ratio was further reduced to 1:12 (g/mL) or less, the yield of baicalein tended to dramatically decline to 34.1% ± 4.0% at 1:8 (g/mL). Due to the limited solubility of baicalin in the reaction system, a large amount of baicalin could not be dissolved in the solvent even if the temperature had reached a high level, which prevented the hydrolysis from accomplishment. Hence, to save the amount of n-BuOH and increase the efficiency of the reaction, the solid–liquid ratio of 1:16 was selected in the optimal hydrolysis conditions.
3.1.4. HCl concentration
When the ratio of baicalin to n-BuOH was 1:16 (g/mL) and the hydrolysis duration was 2.0 h, the effect of HCl concentration on the yield of baicalein was investigated and the results were shown in (d). With the increase of HCl concentration from 0.10% to 0.50%, the yield of baicalein increased significantly (P < 0.01) to the point where insufficient acid did not result in a desired full hydrolysis. However, when HCl concentration was further increased to 3.00%, the yield has been decreased to 45.5% ± 5.4%. The resulting baicalein could be extensively converted to byproducts due to the presence of excess acid in the reaction system. The yield of baicalein was the highest (P < 0.05) when the HCl concentration was 0.50%, which was then chosen for subsequent hydrolysis.
3.1.5. Hydrolysis duration
The acid hydrolysis was then performed under the optimum conditions defined above for various durations. As shown in (e), the yield of baicalein increased rapidly from 32.5% ± 4.3% to 76.5% ± 7.7% as the hydrolysis lasted from 0.5 h to 2.5 h (P < 0.01). The highest yield of baicalein was obtained at a hydrolysis duration of 2.5 h, indicating the best performance in the conversion of baicalin to baicalein which was required to consume sufficient time. However, the yield was decreased moderately when an additional half an hour was spent to hydrolyze the substrate baicalin (P > 0.05). This could be because 0.50% HCl (w/v) was completely consumed within 2.0 h, which negatively affected baicalein production.
3.2. Orthogonal experimental design
The results of the L9(3)4 orthogonal experiment were presented in , and range analysis was performed using the yield of baicalein as the index. The R-values of the three factors, namely solid–liquid ratio (A), HCl concentration (B), and hydrolysis duration (C), indicated that the influence on the yield of baicalein followed the order of B > A > C. The results of variance analysis, as presented in , revealed that all three factors had a significant impact on the yield of baicalein. The best conditions for high-temperature-little-acid hydrolysis were determined to be B2A1C2, which corresponded to a solid–liquid ratio of 1:25, HCl concentration of 0.50%, and hydrolysis duration of 2.0 h.
Table 2. Results and analysis of L9(3)4 orthogonal experiment.
Table 3. Variance analysis of L9(3)4 orthogonal experiment.
3.3. Scale-up experiment
The hydrolysis efficiency of high-temperature-little-acid hydrolysis of baicalin was further investigated in a sixty-time scale-up experiment. The results demonstrated that after a 2-h hydrolysis of baicalin, a crude product of baicalein was obtained by the newly proposed approach, and complete hydrolysis of the substrate was observed in a typical HPLC chromatogram (Figure S2). The purity of baicalein in the crude product was determined to be 74.2%, and the yield of baicalein was calculated to be 83.5% ± 6.3%.
3.4. Purification of baicalein
In the subsequent purification of baicalein from a crude product, DM130 and D101 macroporous resins were utilized in sequence according to the routine procedure. As a result, the purity of baicalein was increased from 74.2% to 98.7%, and the resulting product was exhibited as yellow powder (). The improved product purity was attributed to the removal of the by-product (glucuronic acid) as well as other impurities caused by butyl esterification of baicalin and additional reaction between baicalein, glucuronic acid and n-BuOH.
3.5. Identification of baicalein
After sequential purification by two types of macroporous resin, the fraction containing baicalein was collected and pooled. Its purity was determined to be ≥ 98% by HPLC-UV analysis. Furthermore, to confirm the chemical structure of the resulting baicalein, spectrometric techniques including LC-ESI + -MS, 1H-NMR, and 13C-NMR were employed (Figure S3).
LC-ESI + -MS: m/z 271.10 [M + H]+, 269.10 [M-H]; 1H-NMR (400 MHz, DMSO-d6) δ: 12.66 (1H, s, 5-OH), 10.58 (1H, s, 7-OH), 8.82 (1H, s, 6-OH), 8.06 (2H, dd, J = 8.0, 1.5 Hz, H-1′, 6′), 7.68–7.45 (3H, m, H-3′, 4′, 5′), 6.93 (1H, s, H-3), 6.63 (1H, s, H-8); 13C-NMR (100 MHz, DMSO-d6) δ: 182.40 (CO), 163.18 (C-2), 153.91 (C-7), 150.11 (C-9), 147.25 (C-5), 132.10 (C-4′), 131.25 (C-1′), 129.60 (C-6), 129.39 (C-3′), 126.58 (C-2′), 104.76 (C-3), 104.56 (C-10), 94.29 (C-8). The above data were consistent with references [Citation54–56], and accordingly the compound was identified as baicalein.
3.6. Comparison between the novel strategy and reported methods
To validate the applicability of the new strategy proposed in this study, it was compared with reported methods for the preparation of baicalein from baicalin. The results of the comparison are summarized in . Compared with the conventional acid hydrolysis, the yield of baicalein by the newly proposed strategy was 83.5% ± 6.3%, and the amount of acid consumed per gram of baicalein was only 0.6683 mL, which was approximately 80 times lower than that of the conventional acid hydrolysis [Citation50]. In addition, a complete conversion of baicalin was not achieved even after 20-h enzymatic hydrolysis or 7-d fermentation [Citation57,Citation58], whereas the conversion rate of the new method reached 100% in 2.0 h. Therefore, the method newly developed in this study was much more efficient and environmentally friendly. In recent decades, many comprehensive utilization approach of natural resources have been developed to reduce environmental pollution and make value-added products, meeting the principles of green chemistry [Citation59–61]. In future studies, efforts could be put into the recovery of free glucuronic acid released from high-temperature-little-acid hydrolysis of baicalin.
Table 4. Verification experiments and comparison with other methods.
4. Conclusion
In this study, high-temperature-little-acid hydrolysis of baicalin has been proposed to minimize acid usage for efficient preparation of baicalein. The reaction system was composed of n-BuOH instead of water to achieve a high temperature and only a little HCl was added as catalyst. The reaction time of the newly developed strategy was significantly shorter than enzymatic hydrolysis and fermentation. In addition, the acid consumption per gram of baicalein was approximately 80-fold reduction in comparison with conventional acid hydrolysis. The novel strategy established in this study can reduce the amount of acid and complete the conversion reaction in a short time, which is very suitable for the large-scale preparation of natural active ingredients in the pharmaceutical industry.
Supplemental Material
Download MS Word (111.4 KB)Acknowledgements
Lingling Tang: Investigation, Validation, Writing – Original draft preparation. Jiali Shao: Methodology, Visualization. Hanxue Zheng: Investigation, Formal analysis. Jixuan Chen: Data Curation. Guohua Xia: Resources, Funding acquisition. Huan Yang: Supervision, Resources. Yaya Yang: Project administration, Writing – Review & Editing. Yuping Shen: Conceptualization, Supervision, Writing – Review & Editing, Funding acquisition. All the authors gave their final approval for publication.
Disclosure statement
No potential conflict of interest was reported by the author(s).
Additional information
Funding
References
- Chinese Pharmacopoeia Commission. The Pharmacopoeia of the People’s Republic of China (Volume I); Chinese Medical Science and Technology Press: Beijing, 2015; pp. 417–418.
- Zhao, T.T.; Tang, H.L.; Xie, L.; Zheng, Y. Scutellaria baicalensis Georgi. (Lamiaceae): A Review of Its Traditional Uses, Botany, Phytochemistry, Pharmacology and Toxicology. J. Pharm. Pharmacol. 2019, 71 (9), 1353–1369. doi:10.1111/jphp.13129.
- Song, J.W.; Long, J.Y.; Xie, L.; Zhang, L.L.; Li, X.F. Applications, Phytochemistry, Pharmacological Effects, Pharmacokinetics, Toxicity of Scutellaria baicalensis Georgi. and Its Probably Potential Therapeutic Effects on Covid-19: A Review. Chin. Med-UK. 2020, 15 (1), 102. doi:10.1186/s13020-020-00384-0.
- British Pharmacopoeia Commission. British Pharmacopoeia, 2023 ed.; The Stationery Office: London, 2023.
- European Pharmacopoeia Commission. European Pharmacopoeia, 11.0 ed.; European Directorate for the Quality Control of Medicines: Strasbourg, 2023.
- Japanese Pharmacopoeia Commission. Japanese Pharmacopoeia, 18th ed.; Ministry of Health, Labour and Welfare: Tokyo, 2021.
- Ahmadi, F.I.; Fathollahi, R.; Dastan, D. Phytochemical Constituents and Biological Properties of Scutellaria condensata Subsp. Pycnotricha. J. Appl. Organomet. Chem. 2022, 2 (3), 119–128. doi:10.22034/jaoc.2022.154719.
- Jang, J.Y.; Im, E.; Kim, N.D. Therapeutic Potential of Bioactive Components from Scutellaria baicalensis Georgi in Inflammatory Bowel Disease and Colorectal Cancer: A Review. Int. J. Mol. Sci. 2023, 24 (3), 1954–1954. doi:10.3390/ijms24031954.
- Wang, Z.L.; Wang, S.; Kuang, Y.; Hu, Z.M.; Qiao, X.; Ye, M. A Comprehensive Review on Phytochemistry, Pharmacology, and Flavonoid Biosynthesis of Scutellaria baicalensis. Pharm. Biol. 2018, 56 (1), 465–484. doi:10.1080/13880209.2018.1492620.
- Lee, W.; Ku, S.K.; Bae, J.S. Anti-Inflammatory Effects of Baicalin, Baicalein, and Wogonin In Vitro and In Vivo. Inflammation 2015, 38 (1), 110–125. doi:10.1007/s10753-014-0013-0.
- Mirshekar, A. Chemical Composition and Insecticidal Activities of Mentha Longifolia and Mentha Mozaffarianii Essential Oils Against Callosobruchus maculatus. Chem. Methodol. 2021, 5 (6), 454–463. doi:10.22034/chemm.2021.136811.
- Monjezi, A.; Karimian, P.; Yousofvand, V. Therapeutic Applications of Salvadora persica Plant in Medical Sciences: A Review Article. Asian J. Green Chem. 2023, 7 (3), 180–188. doi:10.22034/ajgc.2023.388764.1374.
- Wang, X.; Yu, J.Y.; Sun, Y.; Wang, H.; Shan, H.; Wang, S.B. Baicalin Protects LPS-Induced Blood-Brain Barrier Damage and Activates Nrf2-Mediated Antioxidant Stress Pathway. Int. Immunopharmacol. 2021, 96, 107725. doi:10.1016/j.intimp.2021.107725.
- Shakil Ahmed, F. R.; Sultana, M. J.; Sultana, A.; Alom, M. F. Study of Anti-Thrombocyte Activity of Cassia Fistula Seeds Extract and It’s Total Phenolic and Flavonoid Content, In Vitro Antioxidant and Anti-Inflammatory Activities. Asian J. Green Chem. 2023, 7 (4), 258–268. doi:10.22034/ajgc.2023.398285.1393.
- Masjedi, M.; Nateghi, L.; Berenjy, S.; Eshaghi, M.R. Determination of Antioxidant and Antimicrobial Compounds of Ganoderma lucidum Extract in Laboratory Different Conditions. Chem. Methodol. 2022, 6 (3), 212–227. doi:10.22034/chemm.2022.316157.1398.
- Zhao, D.J.; Du, B.H.; Xu, J.H.; Xie, Q.Z.; Lu, Z.; Kang, Y.H. Baicalin Promotes Antibacterial Defenses by Modulating Mitochondrial Function. Biochem. Biophys. Res. Co. 2022, 621, 130–136. doi:10.1016/j.bbrc.2022.06.084.
- Li, K.W.; Liang, Y.Y.; Cheng, A.; Wang, Q.; Li, Y.; Wei, H.C.; Zhou, C.Z.; Wan, X.H. Antiviral Properties of Baicalin: A Concise Review. Rev. Bras. Farmacogn. 2021, 31, 408–419. doi:10.1007/s43450-021-00182-1.
- Xiang, L.; Gao, Y.; Chen, S.Y.; Sun, J.Y.; Wu, J.S.; Meng, X.L. Therapeutic Potential of Scutellaria baicalensis Georgi in Lung Cancer Therapy. Phytomedicine. 2021, 95, 153727. doi:10.1016/j.phymed.2021.153727.
- Wang, D.N.; Li, Y. Pharmacological Effects of Baicalin in Lung Diseases. Front. Pharmacol. 2023, 14, 1188202. doi:10.3389/fphar.2023.1188202.
- Wang, L.; Feng, T.; Su, Z.L.; Pi, C.; Wei, Y.M.; Zhao, L. Latest Research Progress on Anticancer Effect of Baicalin and Its Aglycone Baicalein. Arch. Pharm. Res. 2022, 45 (8), 535–557. doi:10.1007/s12272-022-01397-z.
- Zhao, F.; Tao, W.W.; Shang, Z.Y.; Zhang, W.H.; Ruan, J.; Zhang, C.Y.Y.; Zhou, L.P.; Aiello, H.T.; Lai, H.Z.; Qu, R. Facilitating Granule Cell Survival and Maturation in Dentate Gyrus with Baicalin for Antidepressant Therapeutics. Front. Pharmacol. 2020, 11, 556845. doi:10.3389/fphar.2020.556845.
- Tseng, K.F.; Chang, T.Y. Studies on the Flavonoids in Chinesis Drugs Ⅴ. The Chemical Composition of Huang-Chin (Scutellaria Baicalensis Georgi.) (Ⅰ) An Improved Method for Extracting Baicalein and the Preparation of New Methylated Compound. Acta Pharmacol. Sin. 1957, 1, 47–57. doi:10.16438/j.0513-4870.1957.01.007.
- Li, Q.; Li, Q.Q.; Jia, J.N.; Sun, Q.Y.; Zhou, H.H.; Jin, W.L.; Mao, X.Y. Baicalein Exerts Neuroprotective Effects in FeCl3-Induced Posttraumatic Epileptic Seizures Via Suppressing Ferroptosis. Front. Pharmacol. 2019, 10, 638. doi:10.3389/fphar.2019.00638.
- Zhu, Q.; Zhuang, X.X.; Lu, J.H. Neuroprotective Effects of Baicalein in Animal Models of Parkinson's Disease: A Systematic Review of Experimental Studies. Phytomedicine. 2019, 55, 302–309. doi:10.1016/j.phymed.2018.09.215.
- Huang, H.L.; Wang, Y.J.; Zhang, Q.Y.; Liu, B.; Wang, F.Y.; Li, J.J.; Zhu, R.Z. Hepatoprotective Effects of Baicalein Against CCl₄-Induced Acute Liver Injury in Mice. World J. Gastroentero. 2012, 18 (45), 6605–6613. doi:10.3748/wjg.v18.i45.6605.
- Low, Z.X.; OuYong, B.M.; Hassandarvish, P.; Poh, C.L.; Ramanathan, B. Antiviral Activity of Silymarin and Baicalein Against Dengue Virus. Sci. Rep-UK. 2021, 11 (1), 21221. doi:10.1038/s41598-021-98949-y.
- Lee, J.Y.; Kim, J.M.; Kim, C.J. Flavones Derived from Nature Attenuate the Immediate and Late-Phase Asthmatic Responses to Aerosolized-Ovalbumin Exposure in Conscious Guinea Pigs. Inflamm. Res. 2014, 63 (1), 53–60. doi:10.1007/s00011-013-0670-8.
- Chmiel, M.; Stompor-Gorący, M. Promising Role of the Scutellaria baicalensis Root Hydroxyflavone-Baicalein in The Prevention and Treatment of Human Diseases. Int. J. Mol. Sci. 2023, 24 (5), 4732. doi:10.3390/ijms24054732.
- Wang, H. Application of Deep Eutectic Solvents in Extraction and Preparation of Active Ingredient of Scutellaria baicalensis Georgi. Ph.D. Thesis, Shanxi University, 2019.
- Anayat, S.; Ahmed, D.; Anis, M.; Anis, N. Glycerol-Water Green Solvent for Bioactive Metabolites Extraction from Ranunculus arvensis and Extraction Optimization by Response Surface Methodology. Asian J. Green Chem. 2023, 7 (4), 283–299. doi:10.22034/ajgc.2023.399624.1395.
- Aduloju, E.I.; Yahaya, N.; Mohammad Zain, N.; Anuar Kamaruddin, M.; Ariffuddin Abd Hamid, M. An Overview on the Use of Deep Eutectic Solvents for Green Extraction of Some Selected Bioactive Compounds from Natural Matrices. Adv. J. Chem. A. 2023, 6 (3), 253–300. doi:10.22034/ajca.2023.389403.1356.
- Wang, C.Z.; Zhang, C.F.; Chen, L.; Samantha, A.; Lu, F.; Yuan, C. Colon Cancer Chemopreventive Effects of Baicalein, an Active Enteric Microbiome Metabolite from Baicalin. Int. J. Oncol. 2015, 47 (5), 1749–1758. doi:10.3892/ijo.2015.3173.
- Yang, X.R.; Zhang, Q.; Gao, Z.M.; Yu, C.Y.; Zhang, L. Baicalin Alleviates IL-1β-Induced Inflammatory Injury via Down-Regulating MiR-126 in Chondrocytes. Biomed. Pharmacother. 2018, 99, 184–190. doi:10.1016/j.biopha.2018.01.041.
- Ng, S.P.; Wong, K.Y.; Zhang, L.; Zuo, Z.; Lin, G. Evaluation of The First-Pass Glucuronidation of Selected Flavones in Gut by Caco-2 Monolayer Model. J. Pharm. Pharm. Sci. 2004, 8 (1), 1–9.
- Zhang, L.; Lin, G.; Chang, Q.; Zuo, Z. Role of Intestinal First-Pass Metabolism of Baicalein in Its Absorption Process. Pharm. Res-Dordr. 2005, 22 (7), 1050–1058. doi:10.1007/s11095-005-5303-7.
- Noh, K.; Kang, Y.; Nepal, M.R.; Jeong, K.S.; Oh, D.G.; Kang, M.J.; Lee, S.K.; Kang, W.K.; Jeong, H.; Jeong, T.C. Role of Intestinal Microbiota in Baicalin-Induced Drug Interaction and Its Pharmacokinetics. Molecules 2016, 21 (3), 337. doi:10.3390/molecules21030337.
- Johari, J.; Kianmehr, A.; Mustafa, M.R.; Abubakar, S.; Zandi, K. Antiviral Activity of Baicalein and Quercetin Against The Japanese Encephalitis Virus. Int. J. Mol. Sci. 2012, 13 (12), 16785–16795. doi:10.3390/ijms131216785.
- He, G.Y.; Zhang, S.X.; Xu, L.; Xia, Y.L.; Wang, P.; Li, S.Y.; Zhu, L.L.; Xu, H.X.; Ge, G.B.; Yang, L. C-8 Mannich Base Derivatives of Baicalein Display Improved Glucuronidation Stability: Exploring the Mechanism by Experimentation and Theoretical Calculations. RSC Adv. 2015, 5 (109), 89818–89826. doi:10.1039/C5RA20213B.
- Guo, Z.X.; Hu, X.L.; Xing, Z.Q.; Xing, R.; Lv, R.G.; Cheng, X.G.; Su, J.; Zhou, Z.L.; Xu, Z.H.; Nilsson, S. Baicalein Inhibits Prostate Cancer Cell Growth and Metastasis Via The Caveolin-1/akt/mtor Pathway. Mol. Cell. Biochem. 2015, 406, 111–119. doi:10.1007/s11010-015-2429-8.
- Xu, M.; Xiao, G.S.; Yang, Y. Study on the Prepared Process for Baicalein. China Prac. Med. 2008, 3 (12), 3–4. doi:10.3969/j.issn.1673-7555.2008.12.002.
- Xu, C.; Ji, G.E. Bioconversion of Flavones During Fermentation in Milk Containing Scutellaria baicalensis Extract by Lactobacillus brevis. J. Microbiol. Biotechnol. 2013, 23 (10), 1422–1427. doi:10.4014/jmb.1305.05001.
- Kostrzewa-Susłow, E.; Dmochowska-Gładysz, J.; Oszmiański, J. Microbial Transformation of Baicalin and Baicalein. J. Mol. Catal. B-Enzym. 2008, 49 (1–4), 113–117. doi:10.1016/j.molcatb.2007.08.009.
- Zhang, L.; Wang, L.W.; Chen, Y.F.; Yang, Y.Y.; Xia, G.H.; Guo, Y.A.; Yang, H.; Shen, Y.P.; Meyer, A.S. Biotransformation of Ginsenoside Rb1 and Rd to Four Rare Ginsenosides and Evaluation of Their Anti-Melanogenic Effects. J. Nat. Med. 2023, 77 (4), 939–952. doi:10.1007/s11418-023-01719-5.
- Xie, H.C.; Zhang, L.; Jing, S.L.; Zhou, J.W.; Wu, Q.; Yang, Y.Y.; Chen, Y.F.; Yang, C.Y.; Xia, G.H.; Shen, Y.P.; Yang, H. Efficient Enzymatic Hydrolysis of Protogracillin for Clean Preparation of Prosapogenin A by Response Surface Methodology Optimization. Green Chem. Lett. Rev. 2022, 15 (3), 837–846. doi:10.1080/17518253.2022.2138723.
- Liu, C.; Zhang, L.M.; Tan, L.; Liu, Y.P.; Tian, W.Q.; Ma, L.Q. Immobilized Crosslinked Pectinase Preparation on Porous Zsm-5 Zeolites as Reusable Biocatalysts for Ultra-Efficient Hydrolysis of β-Glycosidic Bonds. Front. Chem. 2021, 9, 677868. doi:10.3389/fchem.2021.677868.
- Yang, C.Y.; Li, B.; Zhou, J.W.; Chen, Y.F.; Xia, G.H.; Shen, Y.P.; Chen, J.X.; Shao, J.L.; Yang, Y.Y.; Yang, H. Conversion of Dioscorea zingiberensis Saponins to Prosapogenin A by Enzymatic Hydrolysis. Nat. Prod. Res. 2023, 37 (9), 1421–1428. doi:10.1080/14786419.2021.2011273.
- Zhu, Y.T.; Peng, J.S.; Zhao, Y.Q.; Wu, M.R.; Chen, S.P.; Shao, J.L.; Wang, X.B.; Xia, G.H.; Shen, Y.P. Obtaining Acid-Sensitive Prosaikogenin F by Enzymatic Hydrolysis of Saikosaponin A. Pharmacogn. Mag. 2023, 19 (3), 689–699. doi:10.1177/09731296231169577.
- Lu, Y.; Luo, Q.L.; Jia, X.B.; Tam, J.P.; Yang, H.; Shen, Y.P.; Li, X. Multidisciplinary Strategies to Enhance Therapeutic Effects of Flavonoids from Epimedii Folium: Integration of Herbal Medicine, Enzyme Engineering, and Nanotechnology. J. Pharm. Anal. 2023, 13 (3), 239–254. doi:10.1016/j.jpha.2022.12.001.
- Yang, H.; Yin, H.W.; Wang, X.W.; Li, Z.H.; Shen, Y.P.; Jia, X.B. In Situ Pressurized Biphase Acid Hydrolysis, a Promising Approach to Produce Bioactive Diosgenin from the Tubers of Dioscorea zingiberensis. Pharmacogn. Mag. 2015, 11 (43), 636–642. doi:10.4103/0973-1296.160472.
- Jiang, J.J.; Dong, H.R. Preparation of High-Purity Baicalein from Scutellaria baicalensis Georgi. Nat. Prod. Res. 2008, 22 (16), 1410–1412. doi:10.1080/14786410701823967.
- Bai, X.Y.; Bian, S.; Yu, S.T.; Zhang, W.; Zhao, D.Q.; Zhao, Y. Simultaneous Determination of Baicalin, Baicalein and Wogonin in Naosaitong Pills by HPLC. Tradit. Chin. Drug. Res. Clin. Pharmacol. 2016, 27 (4), 555–557. doi:10.19378/j.issn.1003-9783.2016.04.019.
- Esteves, L.C.; Pinheiro, A.C.; Pioli, R.M.; Penna, T.C.; Baader, W.J.; Correra, T.C.; Bastos, E.L. Revisiting the Mechanism of Hydrolysis of Betanin. Photochem. Photobiol. 2018, 94 (5), 853–864. doi:10.1111/php.12897.
- Grohmann, K.; Manthey, J.A.; Cameron, R.G. Acid-Catalyzed Hydrolysis of Hesperidin at Elevated Temperatures. Carbohyd. Res. 2000, 328 (2), 141–146. doi:10.1016/s0008-6215(00)00081-1.
- Kim, S.; Sohn, D.W.; Kim, Y.C.; Kim, S.A.; Lee, S.K.; Kim, H.S. Fine Tuning of a Reported Synthetic Route for Biologically Active Flavonoid, Baicalein. Arch. Pharm. Res. 2007, 30 (1), 18–21. doi:10.1007/BF02977773.
- Jiang, X.Y.; Zhou, J.T.; Lin, Q.H.; Gong, G.Y.; Sun, H.P.; Liu, W.Y.; Guo, Q.L.; Feng, F.; Qu, W. Anti-Angiogenic and Anticancer Effects of Baicalein Derivatives Based on Transgenic Zebrafish Model. Bioorgan. Med. Chem. 2018, 26 (15), 4481–4492. doi:10.1016/j.bmc.2018.07.037.
- Yang, P.; Kong, X.Z.; Cheng, C.M.; Li, C.Y.; Yang, X.M.; Zhao, X.E. Synthesis and Biological Evaluation of 8-Substituted and Deglucuronidated Scutellarin and Baicalin Analogues as Antioxidant Responsive Element Activators. Sci. China Chem. 2011, 54 (10), 1565–1575. doi:10.1007/s11426-011-4361-4.
- Yu, H.S.; Han, Y.T.; Liu, C.Y.; Wu, X.B.; Sun, C.K.; Xu, L.Q.; Jin, F.X. Preparation of Baicalein from Baicalin Using A Baicalin-β-D-Glucuronidase from Aspergillus niger b.48 Strain. Process Biochem. 2020, 97, 168–175. doi:10.1016/j.procbio.2020.05.030.
- Yao, L.; Zhang, M.; Wang, P.J.; Long, H.N.; He, H.; Gao, X.L. Optimization of Bioconversion Process of Baicalin in Scutellariae Radix. Chin. J. Exp. Tradit. Med. Form. 2015, 21 (9), 22–24. doi:10.13422/j.cnki.syfjx.2015090022.
- Shen, Y.P.; Zhou, J.W.; Yang, C.Y.; Chen, Y.F.; Yang, Y.Y.; Zhou, C.S.; Wang, L.W.; Xia, G.H.; Yu, X.J.; Yang, H. Preparation and Characterization of Oregano Essential Oil-Loaded Dioscorea zingiberensis Starch Film with Antioxidant and Antibacterial Activity and Its Application in Chicken Preservation. Int. J. Biol. Macromol. 2022, 212 (8), 20–30. doi:10.1016/j.ijbiomac.2022.05.114.
- Chen, Y.F.; Wang, L.W.; Guo, Y.A.; Zhang, M.J.; Xie, H.C.; Xia, G.H.; Xu, L.L.; Yang, H.; Shen, Y.P. Preparation of Isoquercitrin and Rhamnose from Readily Accessible Rutin by a Highly Specific Recombinant α-L-Rhamnosidase (r-Rha1). Nat. Prod. Res. 2024. doi:10.1080/14786419.2024.2303600.
- Wang, L.W.; Yang, C.Y.; Deng, X.L.; Peng, J.S.; Zhou, J.W.; Xia, G.H.; Zhou, C.S.; Shen, Y.P.; Yang, H. A pH-Sensitive Intelligent Packaging Film Harnessing Dioscorea zingiberensis Starch and Anthocyanin for Meat Freshness Monitoring. Int. J. Biol. Macromol. 2022, 245, 125485. doi:10.1016/j.ijbiomac.2023.125485.