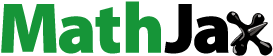
ABSTRACT
Triethyl phosphate (TEP) is a new member of the Green Solid-Phase Peptide Synthesis (GSPPS) toolbox. TEP shows almost no toxicity and no carcinogenic or mutagenic properties. It degrades, giving the rather innocuous phosphoric acid and ethanol. TEP has a low viscosity, which makes it appealing for solid-phase peptide synthesis (SPPS), where the reagents delivered by the solvent should penetrate the polymeric matrix. TEP dissolves most Fmoc-amino acids and key coupling reagents and additives. The use of TEP has allowed the SPPS of several model peptides using the most conventional polystyrene resin at room temperature, while other green solvents required more sophisticated resins and/or elevated temperatures. In conclusion, TEP is an excellent contender to become the green solvent of choice.
1. Introduction
The main focus of the Industrial Revolution in the 18th and 19th centuries was to produce goods to cover the demands of a growing population. Nowadays, although demographic expansion is increasing at an even faster rate, the focus is to produce goods sustainably. In this context, synthetic organic chemistry is, without doubt, the field of science most involved in the production process. The Achilles heel of this process is the large consumption of solvents (Citation1). In the pharmaceutical industry, for instance, solvents account for the major part of industrial waste (Citation2). Given this observation, the development of green solvents is one of the challenges to be tackled by current chemistry. Following the 12 Principles of Green Chemistry proposed by Anastas (Citation3), an ideal green solvent should be produced from renewable sources and it should be non-toxic, non-volatile, recyclable, biodegradable, and inexpensive (Citation1,Citation4–6).
Peptides are an important component of drugs and they are produced mainly using the solid-phase peptide synthesis (SPPS) technique developed by Merrifield (Citation7), whereby the synthesis is carried out while the growing peptide is anchored on a solid support (resin). The use of SPPS using a fluorenylmethoxycarbonyl (Fmoc)-tert-butyl (tBu) protection strategy allows the preparation of small amounts of peptides for drug discovery phases and also peptides in a multi-Kg scale (Citation8,Citation9). SPPS presents some features of sustainability: all reactions are carried out in the same reactor; excellent reaction yields are achieved, with the consequent purity of the final product, thereby facilitating the purification; and low person power ratio per Kg of product produced. However, the consumption of solvents is much greater than that of solution chemistry. The increased need for solvent is inherent to SPPS because, after the incorporation of each amino acid, the excess of reagents and soluble side-products are removed by washing the resin and filtration with solvents. A major drawback of the method lies in the toxicity and environmental concerns of conventionally used solvents such as N,N-dimethylformamide (DMF), N-methyl-2-pyrrolidone (NMP) and dichloromethane (DCM). Solvents are intrinsic to any manufacturing SPPS process and account for almost the total mass of waste products (Citation10–12). Despite considerable efforts made by our group and others in academia, as well as industrial groups, there is still no versatile solvent able to perform efficiently in all the chemical steps of SPPS (Citation13). In this context, 2-methyltetrahydrofurane (2-Me-THF) (Citation14, Citation15), γ-valerolactone (GVL) (Citation16, Citation17), N-formylmorpholine (NFM) (Citation18), N-butylpyrrolidone (NBP) (Citation19), N-octylpyrrolidone (NOP) (Citation20), propylene carbonate (Citation2, Citation21), ethyl-5-(dimethylamino)-2-methyl-5-oxopentanoate (PolarClean) (Citation10, Citation22), and dipropyleneglycol dimethyl ether (DMM) (Citation23) have shown some limitations. The use of a mixture of solvents for SPSS has also been proposed (Citation24, Citation25).
In this context, here we studied the suitability of triethyl phosphate (TEP) for all the steps of our SPPS process (). TEP is an organic compound with the chemical formula OP(OEt)3 and it has a subtle pleasant smell due to its ester moiety. It is a transparent liquid at room temperature (rt) and it dissolves in water and other organic solvents such as ethanol and ether (Citation26). It also shows a similar solubility profile to commonly used SPPS solvents such as DMF and NMP (Citation27). Moreover, TEP has seen wide applications in the manufacturing industry as a flame retardant for plastics (Citation26) or combined with halogenated unsaturated polyester to make resins. The compound is also used in the synthesis of polyvinylidene fluorides (Citation28), whereas on a small scale, it is used as a solvent or intermediate in the preparation of pharmaceuticals, and as a stimulant for Sarin GB in warfare weapons (Citation29).
The Material Safety Data Sheet indicated that TEP shows negligible levels of toxicity (Citation30) and is non-carcinogenic, non-mutagenesis, and bioaccumulative, thereby protecting workers and the environment as its treatment as waste is low-risk (Citation31). Given its good toxicology profile and thermal stability, TEP is considered a strong contender for substituting traditional toxic solvents.
2. Experimental part
2.1. Material and method
Triethylphosphate, OxymaPure, and N,N’-diisopropylcarbodiimide (DIC) were a gift from Luxembourg Biotech (Rehovot, Israel). All reagents and solvents were purchased from commercial suppliers and used without further purification. Fmoc-amino acids (Fmoc-AA(PG)-OH) and Fmoc-Rink Amide-amino methyl (AM) resin (loading 0.64 mmol/g) were supplied by Iris Biotech. Piperidine was purchased from Sigma-Aldrich. DMF, other organic solvents, and HPLC-quality CH3CN were purchased from Merck. Milli-Q water was used for RP-HPLC analyses. Analytical HPLC (High Performance Liquid Chromatography) was performed on an Agilent 1100 system using a Phenomenex AerisTMC18 (3.6 μm, 4.6 × 150 mm) column, with a flow rate of 1.0 mL/min and UV detection at 220 nm. Chemstation software was used for data processing. Buffer A: 0.1% trifluoroacetic acid (TFA) in H2O; buffer B: 0.1% TFA in CH3CN. LC-MS (Liquid Chromatography-Mass Spectrometry) was performed on a Thermo Scientific Dionex UltiMate 3000 using Phenomenex AerisTMC18 (3.6 μm, 4.6 × 150 mm) column. Buffer A: 0.1% formic acid in H2O; buffer B: 0.1% formic acid in CH3CN.
2.2. Solubility
Aliquots of 1 mL TEP were successively added to 0.1 mmol of either Fmoc-amino acids, coupling reagents, or additives, and the mixture in each case was stirred until dissolution. Dissolution concentrations were tested from 0.1 M and stopped when 0.9 M was reached or until no further solubilization occurred.
2.3. Resin swelling
A total of 200 mg of resin was placed in a polypropylene syringe (5 mL) fitted with a porous filter. The resin was treated three times with 1 mL of the respective solvent, and then allowed to swell on the orbital shaker for 24 h at rt. Subsequently, the solvent was drained, and the resin was compressed with a piston to release all the solvent until none was left. The piston was then slowly pulled until the resin recovered its maximum volume in the syringe. The degree of swelling was measured using the following formula.
2.4. Procedure for SPPS
All peptides (0.1 mmol scale) were manually synthesized using the Fmoc/tBu strategy on a Fmoc-Rink Amide-AM (Citation17) resin (loading 0.64 mmol/gram) in polypropylene syringes (5 mL) fitted with a porous polyethylene disc. Couplings were carried out by pre-activating the Fmoc-aa-OH with DIC and OxymaPure (3 eq. each) for 30 sec. The mixture was then added to the resin and left to stir for 1 h. Fmoc was removed with piperidine-TEP (2:8) (1 × 1 min + 1 × 10 min). Washes between coupling and deprotection steps were performed with TEP (3 × 1 min). All steps were carried out at rt. Once the final peptide was achieved, the peptide-resins were dried. Peptide cleavage from the dried resin and removal of the side-chain protecting groups were performed with trifluoroacetic acid (TFA)-H2O-triisopropylsilane (TIS) (95:2.5:2.5) for 1 h at rt. The peptide was precipitated with cold cyclopentylmethyl ether (CPME) and, after centrifugation, the solvent was removed by decantation. This process was repeated three times by washing the precipitate with cold DEE. The precipitate was collected after centrifugation, dissolved in CH3CN/H2O, and then analyzed by HPLC. HPLC gradients were: 5–60% B into A in 15 min.
3. Result and discussion
3.1. Physical properties
TEP is an aprotic solvent that is less polar than DMF, NMP, and the green solvents GVL, NBP, PolarClean. However, it is more polar than 2-Me-THF and ethyl acetate (EtOAc) (). Currently, its price in bulk is slightly higher than that of DMF, but an increase in its consumption is expected to impact its price. To the best of our knowledge, TEP has not been used for SPPS.
Table 1. Physical properties of current and green solvents used for SPPS*.
In terms of physical properties, the viscosity of TEP is similar to that of NMP (1.60 vs. 1.65) and much lower than that of other green solvents such as NBP (4.00), NOP (6.60), and PolarClean (9.78). The viscosity is a key physical property required for SPPS. The penetration of highly viscous solvents into the polymeric matrix is hindered and this often brings about incomplete reactions. Some heating is applied to such solvents. Green solvents are characterized by a high boiling point (215 ⁰C) and this property facilitates its use in microwave- or thermal heating-assisted SPPS. The high flash point of TEP (105 ⁰C), which is much greater than that of DMF (58 ⁰C), is also important for its use at elevated temperatures. In contrast, solvents with a low flash point have an elevated ignition risk. The low melting point of TEP (−56.5 ⁰C) could also facilitate its use in automatic synthesizers.
3.2. Ability to solubilize Fmoc-aa-OH
Next, the capacity of TEP to dissolve the Fmoc-amino acids, coupling reagents, and additives was addressed. This property can be determinant for a solvent being considered suitable for SPPS. Reagent solubility and resin swelling capacity are probably the most limiting factors for the use of a given solvent in this synthetic approach.
The studied dissolution started from the standard amino acid concentration of 0.1 M to 0.9 M in TEP solvent. lists the solubility of the amino acids and coupling reagents in TEP. Most amino acids were soluble in TEP from 0.1 M to 0.9 M, except those containing a functional Trt group such as Fmoc-Cys(Trt)-OH, Fmoc-His(Trt)-OH, Fmoc-Asn(Trt)-OH, and Fmoc-Arg(Pbf)-OH, all of which showed poor solubility of ≤ 0.1 M in TEP. Interestingly, Fmoc-Gln(Trt)-OH showed greater solubility than its parent Asn derivative. These findings highlight the influence of side chain protecting groups on the solubility of amino acids. Indeed, this effect was observed when examining Fmoc-Cys(Thp)-OH, which showed superior solubility results up to a high concentration of 0.9 M compared to the corresponding Fmoc-Cys(Trt)-OH.
Table 2. Solubility efficacy of Fmoc-AA(PG)-OH, coupling reagents, and additives in TEP at rt.
Given that an ideal solvent for SPPS should also dissolve the reagents used in the coupling step, the solubility screening was extended to coupling reagents and additives in TEP. The most common additives, such as HOAt, HOBt, and OxymaPure, showed excellent solubility (0.9 M) in TEP. On the other hand, among the stand-alone coupling reagents, only the Oxyma derivative COMU presented good solubility, while the benzotriazole derivatives HATU and HBTU showed poor solubility.
With these results in hand and trying to see if the activated species of the less soluble Fmoc-amino acids show better solubility, DIC/OxymaPure coupling reagents were added to the suspension of Fmoc-Asn(Trt)-OH, Fmoc-His(Trt)-OH, and Fmoc-Arg(Pbf)-OH. However, the concentration did not exceed 0.1 M (). Finally, Fmoc-Asn(Trt)-OH and Fmoc-Arg(Pbf)-OH were properly dissolved in mixtures on TEP and NBP, which is also a green solvent ().
Table 3. Solubility efficacy of a mixture of Fmoc-AA(PG)-OH with coupling additives in TEP.
Table 4. Solubility efficacy of Fmoc-AA(PG)-OH in mixture of TEP-NBP solvents.
3.3. Swelling capacity
Although the paradigm is changing, an ideal resin-solvent pair is considered to be when a determined solvent swells the resin well (Citation21, Citation32). However, excess swelling will be translated into inadequate solvent consumption, with a negative impact in terms of sustainability. We compared the capacity of TEP vs. DMF using two polystyrene (PS)-based resins, namely Fmoc-Rinkamide-amino methyl(AM)-PS-resin and AM-PS-resin, and one polyethylenglycol (PEG)-based resin, namely Fmoc-Rinkamide-ChemMatrix, which swells better than PS in all solvents (the description of loading, bead size and cross-linking of selected resins is reported in Table S1) (Citation33). DMF outperformed TEP with respect to swelling the three resins (, Figure S1). However, the higher swelling of the Fmoc-Rinkamide-AM-PS-resin (more similar to the growing peptide) than the AM-PS-resin suggests TEP may be compatible with the SPPS method.
Table 5. Swelling results of different resins in DMF and TEP.
3.4. Solid-phase peptide synthesis
We tested the efficiency of TEP for the SPPS of several model peptides. First, the synthesis of H-Tyr-Gly–Gly-Phe-Leu-NH2 (Leu enkephalinamide) was attempted on Fmoc-Rink Amide AM resin (0.1 mmol scale, loading 0.64 mmol/g) using TEP for all the synthetic steps, namely Fmoc removal, coupling, and washings, at rt. The coupling was carried out with pre-activation of 1 min with Fmoc-aa-OH, N,N’-diisopropylcarbodiimide (DIC)-OxymaPure (3 eq.) (1:1:1) for 1 h; and Fmoc was removed with two treatments of 20% piperidine in TEP (1 min + 7 min). The global deprotection was carried out as indicated above.
Coupling was performed using a three-fold excess of equimolar Fmoc-AA(PG)-OH-DIC-OxymaPure (1:1:1) with respect to resin, with 1 min pre-activation followed by coupling for 1 h. Fmoc was removed with a 20% piperidine solution in the respective solvent for 10 min. After global deprotection with TFA-TIS-H2O (9.5:2.5:2.5), the peptide was precipitated in ether and lyophilized. HPLC analysis () showed that the target peptide was obtained with excellent purity.
Figure 2. HPLC of crude H-YGGFL-NH2; gradient: 5–60% B into A in 15 min; flow rate: 1 mL/min; detection at 220 nm (see Figure S2 for MS).

Next, the synthesis of the most demanding model peptide, H-YIIFL-NH2, was attempted using the same conditions at rt. This time, the target peptide was obtained with a purity of 75.4%. The preparation of the same peptides was repeated at 40–45°C, which yielded better purity (88.2%) ().
Figure 3. HPLC of crude H-YIIFL-NH2 at room temperature and 45–45°C; gradient: 5–60% B into A in 15 min; flow rate: 1 mL/min; detection at 220 nm (see Figure S3 for MS).

Although the presence of deletion peptides has normally been attributed to incomplete incorporation of the protected amino acids, the work carried out by our group and others in developing new green strategies, using mainly different solvents, indicates that the presence of the such peptides is often due to incomplete Fmoc removal (Citation34–39). This is easily explained by the narrow window of basicity in which Fmoc is efficiently removed. In addition, it is important to bear in mind that Fmoc is an aromatic protecting group that can favor aggregation through π- π interactions.
Next, we repeated the synthesis of H-YIIFL-NH2 using DMF for Fmoc removal (20% piperidine-DMF) and TEP for the coupling at 40–45°C. In this case, the target peptide was obtained with practically 100% purity (see Figure S4 for HPLC), thereby indicating that TEP is suitable for the coupling but has some detrimental effect in the Fmoc-removal step. Next, we studied the Fmoc-removal step of this Ile-Ile demanding sequence in greater depth following the strategy shown in Scheme 1.
As shown in the scheme, the tripeptide Fmoc-Ile-Phe-Leu-NH-Rinkamide-resin was subjected to several Fmoc removal conditions and then a new Fmoc-Ile-OH was incorporated. The resulting peptide-resin was cleaved with TFA and analyzed by HPLC. The presence of the tetrapeptide Fmoc-Ile-Ile-Phe-Leu-NH2 and tripeptide H-Ile-Phe-Leu-NH2 indicates that the Fmoc of the first residue of Ile had been removed properly.
The results shown in confirm the difficulty of removing the Fmoc of the first Ile. Unfortunately, the use of co-solvents of a different polarity (from H2O to 2MeTHF) does not solve the problems adequately.
Table 6. Study of the Fmoc-removal conditions.
As Fmoc removal can be problematic during SPPS, we started the synthesis of the acetamidomethyl (Acm)-protected oxytocin [H-Cys(Acm)-Tyr-Ile-Gln-Asn-Cys(Acm)-Pro-Leu-Gly-NH2] at rt using TEP as the sole solvent. Only a mixture of N-butylpyrrolidone (NBP)-TEP (1:1) was used to dissolve the Fmoc-Asn(Trt)-OH, which is one of the amino acids that is not readily solubilized in TEP (). NBP is a green solvent. Although highly viscous, it has been reported to perform well in SPPS (Citation12, Citation40). Fmoc-Cys(Acm)-OH was used to overcome the problems associated with the Trt derivative (). To study the scope and limitations of TEP as a solvent for SPPS, a first synthesis was carried out under ‘standard’ conditions at rt. Couplings were carried out using 3 eq. each of Fmoc-AA-OH, DIC, or OxymaPure in TEP (except Fmoc-Asn(Trt)-OH) for 1 h, and Fmoc was removed in 20% piperidine-TEP (1 × 1 min + 7 min). HPLC analysis (Figure S6) showed a more than decent profile for the first amino acid of the sequence but with a major impurity [around 40% of the des-Cys(Acm)] (Citation1). These observations indicate that both Fmoc removal, including the Ile residue, and coupling went smoothly until the removal of the Fmoc of the last before amino acid [Tyr(tBu)]. Encouraged by these positive results, we repeated the syntheses but with an extended Fmoc-removal time (2 × 10 min). The LC-MS of the crude product showed that the target peptide was obtained with good purity, with just a tiny amount of des-Cys(Acm) peptide ().
4. Conclusion
Despite enormous efforts on the part of academic and industrial groups, there is still no one solvent to replace DMF or NMP for SPPS. Here we have demonstrated that TEP could be a suitable contender for this role. TEP shows almost no toxicity (Citation30) and it is non-carcinogenic and non-mutagenic (Citation31). It degrades, giving innocuous compounds such as phosphoric acid and ethanol. In the context of SPPS, the viscosity of TEP is slightly greater than that of NMP and much greater than that of other green solvents already used, such as NBP NFM, NOP, and PolarClean. Our results reveal that TEP can dissolve most of the Fmoc protected amino acids tested. Only Asn and Arg derivatives [Fmoc-Asn(Trt)-OH, Fmoc-Arg(Pbf)-OH] showed insolubility, but these can be dissolved in mixtures of TEP-NBP (1:1). TEP also dissolves the stand-alone coupling reagent COMU, and the most common peptide coupling additives, namely OxymaPure and HOBt. In terms of coupling, TEP showed excellent performance in all the model peptides synthesized. Furthermore, the Fmoc-removal step using 20% piperidine in TEP proved highly effective in most cases, while in some this step requires fine-tuning. In the case of the synthesis of the bis-Acm oxytocin, excellent purity was achieved just by a moderate extension of the deprotection times. We envisage that for other cases, bases that are stronger than piperidine, such as DBU, could overcome this issue.
Finally, TEP and NBP are the best green solvents for the direct substitution of DMF/NMP because they allowed the synthesis of model peptides using conventional polystyrene resins at rt. Other green solvents have given good results using more sophisticated resins such as those based on PEG, and/or high temperatures, including micro-wave assisted chemistry. Due to these first promising results, TEP with low viscosity also seems a good candidate for further investigation as part of mixtures (Citation24, Citation34).
Supplemental Material
Download MS Word (9.5 MB)Acknowledgments
We thank Yoav Luxembourg and Gyorgy Orosz (Luxembourg Biotech, Rehovot, Israel) for supporting this research and for generously providing TEP solvent and coupling reagents. The work was funded in part by the following: the National Research Foundation (NRF) (# 105892 and Blue Sky’s Research Programme # 120386) and the University of KwaZulu-Natal (South Africa).
Disclosure statement
No potential conflict of interest was reported by the author(s).
References
- Prat, D.; Pardigon, O.; Flemming, H.-W.; Letestu, S.; Ducandas, V.; Isnard, P.; Guntrum, E.; Senac, T.; Ruisseau, S.; Cruciani, P.; Hosek, P. Sanofi’s Solvent Selection Guide: A Step Toward More Sustainable Processes. Org. Process Res. Dev. 2013, 17 (12), 1517–1525.
- Henderson, R.K.; Jiménez-González, C.; Constable, D.J.; Alston, S.R.; Inglis, G.G.; Fisher, G.; Sherwood, J.; Binks, S.P.; Curzons, A.D. Expanding GSK's Solvent Selection Guide–Embedding Sustainability into Solvent Selection Starting at Medicinal Chemistry. Green Chem. 2011, 13 (4), 854–862.
- Anastas, P.; Eghbali, N. Green Chemistry: Principles and Practice. Chem. Soc. Rev. 2010, 39 (1), 301–312.
- Jad, Y.E.; Kumar, A.; El-Faham, A.; de la Torre, B.G.; Albericio, F. Green Transformation of Solid-Phase Peptide Synthesis. ACS Sustainable Chem. Eng. 2019, 7 (4), 3671–3683.
- Dong, X.; Al-Jumaily, A.; Escobar, I.C. Investigation of the Use of a Bio-Derived Solvent for Non-Solvent-Induced Phase Separation (NIPS) Fabrication of Polysulfone Membranes. Membranes. 2018, 8 (2), 1–17.
- Al Musaimi, O.; de la Torre, B.G.; Albericio, F. Greening Fmoc/t Bu Solid-phase Peptide Synthesis. Green Chem. 2020, 22 (4), 996–1018.
- Merrifield, R.B. Solid-Phase Peptide Synthesis. In The Chemistry of Polypeptides: Essays in Honor of Dr. Leonidas Zervas, Katsoyannis, P. G., Ed.; Springer US: Boston, MA, 1973; pp 335–361.
- Bruckdorfer, T.; Marder, O.; Albericio, F. From Production of Peptides in Milligram Amounts for Research to Multi-tons Quantities for Drugs of the Future. Curr. Pharm. Biotechnol. 2004, 5 (1), 29–43.
- Zompra, A.; Galanis, A.; Werbitzky, O.; Albericio, F. Preparation of Peptides as Active Pharmaceutical Ingredients (API). Future Med. Chem. 2009, 1, 361–377.
- Pawlas, J.; Rasmussen, J.H. Re Green SPPS: Enabling Circular Chemistry in Environmentally Sensible Solid-phase Peptide Synthesis. Green Chem. 2019, 21, 5990–5998.
- Pawlas, J.; Antonic, B.; Lundqvist, M.; Svensson, T.; Finnman, J.; Rasmussen, J.H. 2D Green SPPS: Green Solvents for On-resin Removal of Acid Sensitive Protecting Groups and Lactamization. Green Chem. 2019, 21 (10), 2594–2600.
- Isidro-Llobet, A.; Kenworthy, M.N.; Mukherjee, S.; Kopach, M.E.; Wegner, K.; Gallou, F.; Smith, A.G.; Roschangar, F. Sustainability Challenges in Peptide Synthesis and Purification: From R&D to Production. J. Org. Chem. 2019, 84 (8), 4615–4628.
- Ferrazzano, L.; Catani, M.; Cavazzini, A.; Martelli, G.; Corbisiero, D.; Cantelmi, P.; Fantoni, T.; Mattellone, A.; De Luca, C.; Felletti, S.; Cabri, W.; Tolomelli, A. Sustainability in Peptide Chemistry: Current Synthesis and Purification Technologies and Future Challenges. Green Chem. 2022, 24 (3), 975–1020.
- Jad, Y.E.; Acosta, G.A.; Khattab, S.N.; de la Torre, B.G.; Govender, T.; Kruger, H.G.; El-Faham, A.; Albericio, F. 2-Methyltetrahydrofuran and Cyclopentyl Methyl Ether for Green Solid-phase Peptide Synthesis. Amino Acids 2016, 48 (2), 419–426.
- Jad, Y.E.; Acosta, G.A.; Govender, T.; Kruger, H.G.; El-Faham, A.; de la Torre, B.G.; Albericio, F. Green Solid-phase Peptide Synthesis 2. 2-Methyltetrahydrofuran and Ethyl Acetate for Solid-phase Peptide Synthesis under Green Conditions. ACS Sustainable Chem. Eng. 2016, 4 (12), 6809–6814.
- Kumar, A.; Jad, Y.E.; Collins, J.M.; Albericio, F.; de la Torre, B.G. Microwave-assisted Green Solid-phase Peptide Synthesis Using γ-Valerolactone (GVL) as Solvent. ACS Sustainable Chem. Eng. 2018, 6 (6), 8034–8039.
- Kumar, A.; Thompson-Adewumi, A.; Nandhini, K.P.; Collins, J.M.; Albericio, F.; de la Torre, B.G. Troubleshooting When Using γ-Valerolactone (GVL) in Green Solid-Phase Peptide Synthesis. Org. Process Res. Dev. 2019, 23 (5), 1096–1100.
- Kumar, A.; Jad, Y.E.; El-Faham, A.; de la Torre, B.G.; Albericio, F. Green Solid-phase Peptide Synthesis 4. γ-Valerolactone and N-formylmorpholine as Green Solvents for Solid Phase Peptide Synthesis. Tetrahedron Lett. 2017, 58 (30), 2986–2988.
- Lopez, J.; Pletscher, S.; Aemissegger, A.; Bucher, C.; Gallou, F. N-Butylpyrrolidinone as Alternative Solvent for Solid-phase Peptide Synthesis. Org. Process Res. Dev. 2018, 22 (4), 494–503.
- Martelli, G.; Cantelmi, P.; Tolomelli, A.; Corbisiero, D.; Mattellone, A.; Ricci, A.; Fantoni, T.; Cabri, W.; Vacondio, F.; Ferlenghi, F.J.G.C.; Mor, M.; Ferrazzano, L. Steps towards Sustainable Solid Phase Peptide Synthesis: Use and Recovery of N-octyl Pyrrolidone. Green Chem. 2021, 23 (11), 4095–4106.
- Lawrenson, S.; North, M.; Peigneguy, F.; Routledge, A. Greener Solvents for Solid-phase Synthesis. Green Chem. 2017, 19 (4), 952–962.
- Kumar, A.; Sharma, A.; de la Torre, B.G.; Albericio, F. Rhodiasolv PolarClean – A Greener Alternative in Solid-phase Peptide Synthesis. Green Chem. Lett. Rev. 2021, 14 (3), 545–550.
- Vivenzio, G.; Scala, M.C.; Marino, P.; Manfra, M.; Campiglia, P.; Sala, M. Dipropyleneglycol Dimethylether, New Green Solvent for Solid-Phase Peptide Synthesis: Further Challenges to Improve Sustainability in the Development of Therapeutic Peptides. Pharmaceutics. 2023, 15 (6), 1773.
- Ferrazzano, L.; Corbisiero, D.; Martelli, G.; Tolomelli, A.; Viola, A.; Ricci, A.; Cabri, W. Green Solvent Mixtures for Solid-Phase Peptide Synthesis: A Dimethylformamide-Free Highly Efficient Synthesis of Pharmaceutical-Grade Peptides. ACS Sustainable Chem. Eng. 2019, 7 (15), 12867–12877.
- Jadhav, S.; Martin, V.; Egelund, P.H.; Castro, H.J.; Krüger, T.; Richner, F.; Le Quement, S.T.; Albericio, F.; Dettner, F.; Lechner, C.; Schönleber, R.; Pedersen, D.S. Replacing DMF in Solid-phase Peptide Synthesis: Varying the Composition of Green Binary Solvent Mixtures as a Tool to Mitigate Common Side-reactions. Green Chem. 2021, 23 (9), 3312–3321.
- Zhao, H.; Yuan, B.; Zhan, Y.; Yang, F.; Zhou, J.; Qi, C.; Lei, C.; Li, Y. Upgrading the Pore-size Scale of MIL-53 from Microporous to Macroporous for Adsorbing Triethyl Phosphate and Reducing the Fire Risk of Polystyrene. Composites Part A 2022, 159, 107003.
- Bai, Y.; Essehli, R.; Jafta, C.J.; Livingston, K.M.; Belharouak, I. Recovery of Cathode Materials and Aluminum Foil Using a Green Solvent. ACS Sustainable Chem. Eng. 2021, 9 (17), 6048–6055.
- Pagliero, M.; Comite, A.; Costa, C.; Rizzardi, I.; Soda, O. A Single Step Preparation of Photothermally Active Polyvinylidene Fluoride Membranes Using Triethyl Phosphate as a Green Solvent for Distillation Applications. Membranes. 2021, 11 (11), 896.
- Neupane, S.; Rahman, R.K.; Masunov, A.E.; Vasu, S.S. Theoretical Calculation of Reaction Rates and Combustion Kinetic Modeling Study of Triethyl Phosphate (TEP). J. Phys. Chem. A 2019, 123 (22), 4764–4775.
- www.sigmaaldrich.com/ZA/en/sds/aldrich/538728.
- Marino, T.; Blefari, S.; Di Nicolò, E.; Figoli, A. A More Sustainable Membrane Preparation Using Triethyl Phosphate as Solvent. Green Process. Synth. 2017, 6 (3), 295–300.
- Magtaan, J.K.; Devocelle, M.; Kelleher, F. Assessing the Correlation of Microscopy-based and Volumetry-based Measurements for Resin Swelling in a Range of Potential Greener Solvents for SPPS. J. Pept. Sci. 2020, 26 (6), e3250.
- García-Martín, F.; Quintanar-Audelo, M.; García-Ramos, Y.; Cruz, L.J.; Gravel, C.; Furic, R.; Côté, S.; Tulla-Puche, J.; Albericio, F. ChemMatrix, a Poly(ethylene glycol)-Based Support for the Solid-Phase Synthesis of Complex Peptides. J. Comb. Chem. 2006, 8 (2), 213–220.
- Jad, Y.E.; Govender, T.; Kruger, H.G.; El-Faham, A.; de la Torre, B.G.; Albericio, F. Green Solid-phase Peptide Synthesis (GSPPS) 3. Green Solvents for Fmoc Removal in Peptide Chemistry. Org. Process Res. Dev. 2017, 21 (3), 365–369.
- Přibylka, A.; Krchňák, V.; Schütznerová, E. Environmentally Friendly SPPS II: Scope of Green Fmoc Removal Protocol Using NaOH and Its Application for Synthesis of Commercial Drug Triptorelin. J. Org. Chem. 2020, 85 (14), 8798–8811.
- Přibylka, A.; Pastorek, M.; Grepl, M.; Schütznerová Přibylka, E. The Application of Anisole in Greener Solid-phase Peptide Synthesis Protocols – Compatibility with Green Bases in Fmoc Removal and New Green Binary Mixture for Coupling. Tetrahedron 2021, 99, 132452.
- Martelli, G.; Cantelmi, P.; Palladino, C.; Mattellone, A.; Corbisiero, D.; Fantoni, T.; Tolomelli, A.; Macis, M.; Ricci, A.; Cabri, W.; Ferrazzano, L. Replacing Piperidine in Solid Phase Peptide Synthesis: Effective Fmoc Removal by Alternative Bases. Green Chem. 2021, 23 (20), 8096–8107.
- Egelund, P.H.G.; Jadhav, S.; Martin, V.; Johansson Castro, H.; Richner, F.; Le Quement, S.T.; Dettner, F.; Lechner, C.; Schoenleber, R.; Sejer Pedersen, D. Fmoc-Removal with Pyrrolidine Expands the Available Solvent Space in Green Solid-Phase Peptide Synthesis. ACS Sustainable Chem. Eng. 2021, 9 (42), 14202–14215.
- Mthembu, S.N.; Chakraborty, A.; Schönleber, R.; Albericio, F.; de la Torre, B.G. Morpholine, a Strong Contender for Fmoc Removal in Solid-phase Peptide Synthesis. J. Pept. Sci. 2024, 30, e3538.
- de la Torre, B.G.; Kumar, A.; Alhassan, M.; Bucher, C.; Albericio, F.; Lopez, J. Successful Development of a Method for the Incorporation of Fmoc-Arg (Pbf)-OH in Solid-phase Peptide Synthesis Using N-butylpyrrolidinone (NBP) as Solvent. Green Chem. 2020, 22 (10), 3162–3169.