ABSTRACT
Use of mesenchymal stem cells (MSCs) has been introduced as a promising tool, for structural and functional recovery of damaged tissues/organs. Studies have indicated that interactions between chemokine receptors and their ligands have a critical role in homing of MSCs to the site of injury. Although CXCR4 variants have been characterized, the exact role of each transcript in homing has remained unclear. In this study, cells were pretreated with various hypoxia-mimicking compounds (valproic acid, cobalt-chloride, and deferoxamine mesylate). Results indicated that both variants of CXCR4 were overexpressed after 24 hours of treatments and their expression could cooperatively induce and promote the cell migration. Moreover, deferoxamine mesylate was more effective in overexpression of variant A (lo), which resulted in higher level of CXCR4 protein and the highest rate of migration of the cells. In conclusion, our findings may have important potential implications in clinical applications, reinforcing the concept that manipulating the expression of specific CXCR4 variants may increase migration of MSCs.
Introduction
Stem-cell-based therapy is considered as a promising approach for treatment of a wide variety of diseases [Citation1]. Mesenchymal stem cells (MSCs) are among the most practiced cells in therapeutic applications. These cells are derived from various sources such as adipose tissue (Ad-MSCs), bone marrow (BM-MSCs), peripheral blood, synovium, cord blood, etc. [Citation2]. Among these, Ad-MSCs are preferred option due to their abundance, ease of isolation, differentiation potentials, and immunomodulatory effects. Unlike the embryonic stem cells, there are not much ethical concerns related to these cells. However, the exact mechanism of MSCs recruitment to the site of injury remains less understood. Furthermore, limited survival of transplanted cells and their escape from tissue of interest have restricted the cellular therapeutic potential of MSCs [Citation3,4]. It is likely that chemokines and their receptors are involved in this process, as they are important factors known to control cell migration and homing [Citation5--7]. Ad-MSCs express several homing receptors, some of which are definitely functional, and required for their tissue localization in certain physiological or pathological conditions [Citation8,9].
Recently, a number of chemokine receptors and their specific roles in migration and homing of MSCs have been described [Citation10]. It has been demonstrated that interplay between Stromal cell-derived factor-1 (SDF-1) and its cognate receptor, chemokine (C-X-C motif) receptor 4 (CXCR4), is involved in the migration of stem cells [Citation11,12]. It has also been proposed that SDF-1 acts as a retention agent for CXCR4-expressing cells within the injured tissues [Citation13,14]. There are reports that SDF-1 expressing cells attract CXCR4 positive metastatic cells to their respective organs [Citation15,16]. Another study showed that SDF-1, secreted by carcinoma-associated cells in breast carcinomas, mediate recruitment of CXCR4 expressing progenitor cells [Citation17]. Similar to metastatic cells, MSCs have the capacity to migrate through the bloodstream to different organs under the guidance of chemical signaling navigation [Citation18,19]. However, recent studies have also indicated that expansion of MSCs, ex vivo, can severely impair their homing capacity, resulting in low levels of target tissue engraftment and retention [Citation20,21].
CXCR4 have 2 variants (CXCR4-A and CXCR4-B), from which the second one have been known as CXCR4. These variants are slightly different in the first 9 amino acids at their N-terminals. Variant-A (lo) is encoded by an unspliced mRNA and shown to be expressed in specific tissues and cells, e.g. spleen, lung, leukocytes and some cancerous cell lines like HL-60, HeLa etc. and also cells in the process of metastasis [Citation22,23].
Previous studies have shown that hypoxia or hypoxia-mimicking agents such as cobalt chloride (CoCl2) or deferoxamine mesylate (DFX) can enhance cell migration rate by increasing the stability of a transcription factor hypoxia-inducible factor 1-α (HIF1-α) [Citation24--26]. However, the effects of these agents on differential expression of CXCR4 variants and subsequently migration of MSCs are still not known. In this study, we aimed to test the effects of VPA, CoCl2 and DFX on the expression of different CXCR4 variants and also migration of Ad-MSCs.
Results
Characterization of Ad-MSCs
Primary cultures of lipoaspirates expanded well leading to cells with typical fibroblast-like morphology and colony-forming ability (). To confirm the differentiation potential of these cells, Ad-MSCs were differentiated into osteocytes () and adipocytes (). Furthermore, as shown by flow cytometry, Ad-MSCs were positive for expression of specific cell surface antigens, including CD90, CD44, CD105 and CD73, while these cells were negative for CD11b, CD45 and CD34 ().
Figure 1. Differentiation potential and Immunophenotypic results of Ad-MSCs. Evaluation of Ad-MSCs for minimal criteria. (A and B) Adherent stem cells in stromal vascular fraction (SVF) derived from adipose tissue could form colonies after primary culture. The cells at passage 4 were induced with osteogenic medium for 21 d. In contrast to the control cells (C) the calcifications produced by differentiated cells in the extracellular matrix, were stained with Alizarin Red as evident for osteogenesis (D). Additionally, compared to control (E) osteogensis was confirmed by ALP activity of osteoblasts (F). Moreover unlike the control cells (G) adipogenic differentiation of Ad-MSCs was validated by Oil Red O staining of lipid droplets, which were produced by adipogenic induction medium (H). Photographs are representative of 3 independent experiments for each group. (I) Flow cytometry Results showed that Ad-MSCs are positive for CD90, CD44, CD73, and CD105 and negative for CD11b (macrophage marker), CD45 (haematopoietic stem cell marker) and CD34 (endothelial cell marker).
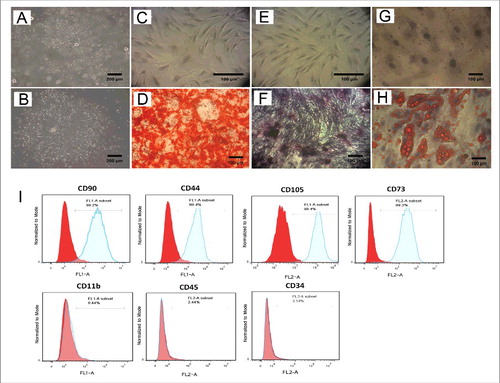
The effects of hypoxia mimicking agents on the expression of CXCR4 variants
In order to verify the effects of hypoxia on the expression of CXCR4, human Ad-MSCs were pretreated for 24 h with hypoxia-mimicking agents including VPA, CoCl2, and DFX. Since the expression of CXCR4-A is very low in MSCs, cDNA prepared from HL60 (Human promyelocytic leukemia cells) was used as a positive control (). RT-PCR results showed that the CXCR4-A (lo) expression was detectable only after treatment with DFX (). Quantitative analysis of the mRNA expressions was carried out by Real-time PCR. Although both variants of CXCR4 were expressed in all pretreated Ad-MSCs, the expression ratios of the 2 variants were different. Result indicated that relative expression of CXCR4-A (lo) had increased 2.24, 6.2, and 8.48 folds after treating with VPA, CoCl2 and DFX, respectively (). Similarly, treatment with these agents resulted in 34.8, 13.35 and 8.56 folds increase in the expression of CXCR4-B, respectively. As seen in , expression of CXCR4-B, was substantially upregulated after DFX treatment, while VPA was more effective in upregulation of CXCR4-B.
Figure 2. Pretreated Ad-MSCs show high expression level of both CXCR4 variants. The effects of different pretreatments on the expression of CXCR4 variants. (A) CXCR4 variants expression in haematopoietic (HL- 60) cell line used as a positive control. (B) In semi q-RT PCR analysis, CXCR4-A (lo) variant expression was appeared only after treatment of Ad-MSCs with DFX for 24h. All treatments (VPA, CoCl2, and DFX) resulted in overexpression of CXCR4-B variant as compared to untreated control. The ACTB was used as an independent housekeeping-gene for both semi q-RT PCR and real-time RT-PCR. (C and D) Quantitative Real time PCR showed that expression of CXCR4-A (lo) variant was more significantly increased after pretreatment with DFX as compared to other treatments rather than other pretreatments and Untreated control. Interestingly expression of CXCR4-B variant had oscillating pattern among VPA, CoCl2, and DFX pretreatments. * p < 0.05, ** p < 0.01 and *** p < 0.001 was considered significant as compared to untreated control. VPA: valproic acid, CoCl2: cobalt-chloride, DFX: deferoxamine mesylate, ACTB: β actin.
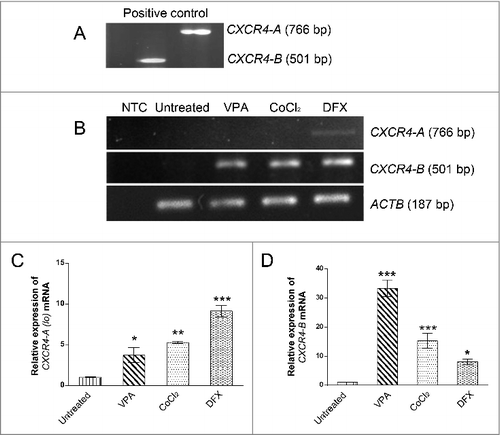
Western blot analysis confirmed results obtained by Real-time PCR regarding CXCR4 gene expression in Ad-MSCs after 24 h of various pretreatments (VPA, CoCl2 and DFX) (). However, as CXCR4-A and B proteins have similar molecular weights, it was impossible to detect the 2 related protein bands separately. As revealed by Western blotting, treatments with VPA, CoCl2 and DFX resulted in 1.3, 1.6 and 2.3 folds increase in the expression of CXCR4, respectively. ImageJ software V2.0 was used to analyze the bands obtained in Western blotting and the results are shown in.
Figure 3. Validation of the results of Real time PCR with Western blotting. As shown by Western blot all treatments resulted in overexpression of CXCR4 protein. ImageJ Software was used to quantify CXCR4 expression in comparision to untreated cells after normalization to ACTB. * p < 0.05 was considered significant as compared to untreated control. VPA: valproic acid, CoCl2: cobalt-chloride, DFX: deferoxamine mesylate, ACTB: β actin.
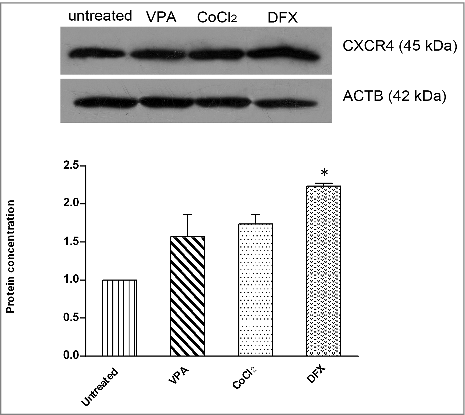
Cell migration assay
The effects of various treatments on cellular migration were assessed using the conventional Boyden chamber assay (Corning). Ad-MSCs from different treatments, were left to migrate for 24 h from the upper chamber through the insert, toward the SDF-1α in receiver wells (). The migration of Ad-MSCs in all treated groups was significantly higher than the untreated control cells. As indicated in , treatments with VPA, CoCl2 and DFX resulted in 4, 5 and 7 folds increase in migration as compared with the untreated control cells. To provide a definite proof that SDF-1α had been the cause for migration of the CXCR4 positive cells, SDF-1α signaling was inhibited using AMD3100, a bicyclam that is extensively used as a CXCR4-specific inhibitor [Citation27]. As shown in , this blockage dramatically decreased migration of the Ad-MSCs; indicating that SDF-1/CXCR4 axis is responsible for migration after these pretreatments.
Figure 4. Pretreated Ad-MSCs display significantly higher migration toward SDF-1α. In vitro migration assay of Ad-MSCs pretreated with various hypoxia-mimicking agents. (A-D) Migration of untreated and pretreated Ad-MSCs toward SDF-1α. After fixation, migrated cells were stained with DAPI. DFX-treatment (D) could induce migration activity of Ad-MSCs more than VPA (B) and CoCl2(C) treated cells. Migration of untreated cells toward SDF-1α was considerably lower (A) than treated cells (B-D). To ensure that enhanced migration of treated cells is because of SDF-1α/CXCR4 axis, AMD3100, a CXCR4-blocking agent, was used to inhibit CXCR4 (E-H). (I) Migration of untreated Ad-MSCs toward FBS was used as a positive control. (J) In absence of FBS and SDF1-1α in lower chamber (as negative control), very few untreated Ad-MSCs migrated only by chance. (K) Quantified average number of migrated Ad-MSCs toward SDF-1α after pretreatments with various hypoxia-mimicking agents (i.e. VPA, CoCl2, and DFX) and also inhibition by AMD3100. Significance of the data was examined in the level of p < 0.001 using the one-way ANOVA and expressed as mean ± SD. # denotes significant difference relative to untreated control, VPA and CoCl2 pretreatment. * denotes significant difference relative to untreated control. VPA: valproic acid, CoCl2: cobalt-chloride, DFX: deferoxamine mesylate, SDF-1α: stromal derived factor-1α.
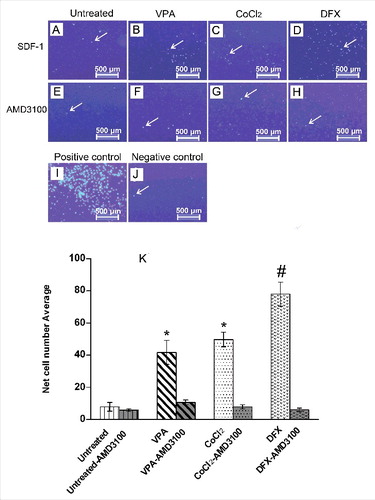
Discussion
Effective use of MSCs as a therapeutic candidate, in part, depends on their ability to home into the target tissues and retain for a long time [Citation28]. During the past decade, preclinical experiments and clinical studies using MSCs have provided data on efficacy and mechanisms involved in therapeutic effects of MSCs [Citation3,4]. Accordingly, there are concerns regarding the outcome of MSC-based clinical trials, particularly those using systemic cell delivery routes. Some studies have focused on the ability of MSCs to activate specific homing receptors [Citation29--31].
It has been shown that Ad-MSCs are directed toward SDF-1 because of its chemotaxic property toward CXCR4 [Citation32]. This migration activity in metastasis state was attributed to overexpression of CXCR4 variant A (lo), which is a subsidiary variant of CXCR4. This expression pattern of CXCR4 in metastasis can be exploited for directing stem cells to specific sites in the body to increase their homing and retention, leading to a better regeneration of a lesion. To this end, we were searching for a way to overexpress CXCR4 variant A (lo) and to investigate whether this variant can increase migration potential of Ad-MSCs. Modulations in CXCR4 expression may greatly affect trafficking of Ad-MSCs into the injured tissue [Citation33], in which higher levels of SDF-1α are expressed [Citation34].
Human CXCR4 gene is expressed in 2 alternative functional forms: the highly expressed CXCR4-B, which is the primary receptor for SDF-1; and a longer, low abundance, but functional CXCR4-A (lo) unspliced variant [Citation35]. Our results of conventional RT-PCR results showed that CXCR4 variant A appears only after treatment of Ad-MSCs with DFX and this hypoxia-mimicking agent caused a significant increase in migration of Ad-MSCs toward SDF-1 as compared with VPA, CoCl2 and untreated control. This would support the notion that increasing the expression level of the CXCR4-A (lo) variant in stem cells may augment their therapeutic effects. Other studies have similarly shown an increase in homing capacity of MSCs, following DFX treatment of the cells [Citation36]. Moreover, experiments reported here, shows that inhibition of CXCR4 receptors impairs Ad-MSCs migration and thus the SDF-1/CXCR4 axis acts as an effective factor in cellular migration. Although, this study does not explain the precise molecular mechanisms of the above mentioned phenomenon, one possibility is the upregulation of HIF-1α [Citation37,38]. In a recently published paper, however it was shown that, although CXCR4-B could be effectively overexpressed in human MSCs by mRNA-nucleofection, no improvement of cell migration could be detected [Citation39]. Therefore, we speculate that CXCR4-A variant, dependent or independent from CXCR4-B, might be responsible for MSC chemotaxis.
As previously mentioned, CXCR4-B is highly expressed in many human tissues, while the expression of CXCR4-A (lo) is either absent or proportionally lower in all tissues [Citation35]. Such tissue-specific manner of expression for the CXCR4-A (lo) implies that its expression is highly regulated. However, the functional results of such an expression patterns for both CXCR4-A (lo) and CXCR4-B is less understood. Gupta et al. showed that CXCR4-A (lo) is less potent and efficient in its response to SDF-1α, with effective concentration (EC50) of ∼20 nM, in comparison with CXCR4-transfected cells (EC50 of ∼6 nM) [Citation35]. Similarly, our results confirmed that the chemotactic response of human Ad-MSCs expressing (DFX-pretreated) CXCR4-Lo was higher in the Transwell migration assay in comparison to the untreated controls and other pretreatments.
Various strategies have been proposed to increase the homing capacity of the MSCs. In this work, an outlook is proposed on the significance of CXCR4 variants and the specific pretreatment for future manipulation of Ad-MSCs, which might be useful in therapeutic applications. The results here show that 2 different variants of this gene responded differently to the treatments and that the variant A was more responsive to DFX treatment as shown by increasing its transcript level. However, this variant is very low in untreated Ad-MSCs. Among the hypoxia-mimicking chemicals, DFX showed higher impact on induction of the CXCR4-A (lo), and subsequently induction of the Ad-MSCs migration. Therefore, the pretreatment of human MSCs with DFX could be a promising therapeutic approach to improve cell transplantation and treatment of various systemic and local degenerative injuries. The data presented here, support notion that subsidiary variant of CXCR4 functions is crucial to increase migration of human Ad-MSCs. Taken together, these findings indicate that MSCs can be handled in vitro to enhance their in vivo homing and efficacy for tissue repair.
Material and methods
Isolation of human MSCs from adipose tissue
All patients included in this study were informed regarding the usage of their samples for experimental analysis and the process was approved by the Ethical Committee of Ferdowsi University of Mashhad.
In this study, the adipose tissue samples were obtained from healthy women, who underwent cosmetic liposuction at Razavi Hospital (Mashhad, Iran). The adipose tissue was extensively washed with phosphate-buffered saline (PBS) to remove debris and blood cells. The washed aspirates were treated with 1 mg collagenase type I (Invitrogen) in PBS per 3 ml lipoaspirates for 45 min at 37°C, with gentle agitation. Ten mg of bovine serum albumin (BSA, Biowest), as carrier protein, (per 1 mg collagenase) and CaCl2 (2 mM; final concentration) were added to the solution for better activation of the enzyme. After centrifugation (at 800 g for 10 min), the floating adipocyte layer and the aqueous PBS–collagenase supernatants were aspirated off. Then, the infranatant pellet (SVF cells fraction) was washed with PBS, centrifuged at 400 g for 6 min, resuspended in Dulbecco's modified Eagle medium (DMEM) low glucose (Biowest), and cultivated in DMEM containing 2 ng/ml basic fibroblast growth factor (bFGF, Royan Institute, Iran), 10% fetal bovine serum (FBS, Invitrogen), 100 μg/ml streptomycin and 100 U/ml penicillin (Invitrogen). In order to perform Ad-MSCs expansion, the resuspended pellet were seeded in 75 cm2 plastic culture flasks (each 10 ml lipoaspirates for one flasks) containing 15 mL of DMEM/10% FBS. The non-adherent cell population was poured off after 24-48 hrs in culture. The isolated human Ad-MSCs were characterized by morphology, flow cytometry and standard differentiation assays. The Ad-MSCs from passage 4 were characterized and used for further experiments.
Osteogenic and adipogenic differentiation
In order to characterize the Ad-MSCs, they were differentiated into osteoblasts according to Secco et al protocol [Citation40]. For this purpose, the Ad-MSCs were cultured in DMEM low glucose, supplemented with 10% FBS, 0.1 μM dexamethasone, 50 μM ascorbate-2-phosphate and 10 mM β-glycerophosphate for 21 d (all from Sigma-Aldrich). For detection of osteoblast calcium deposits and measurement of the alkaline phosphatase activity, the cells were stained with Alizarin Red S (Sigma-Aldrich) and BCIP/NBT tablet (Becton Dickinson, Bioscience) as a substrate, respectively.
The adipogenic differentiation was performed based on da Silva Meirelles protocol [Citation41]. For this purpose, The Ad-MSCs were cultured in DMEM, supplemented with 10% FBS, 1 μM dexamethasone, 10 mM β-glycerophosphate and 200 μM indomethacin (all from Sigma-Aldrich). After 21 days, the fat vacuoles and nuclei were stained with Oil red O (Sigma-Aldrich) and hematoxylin (Merck), respectively.
Flow cytometry
For further characterization of Ad-MSCs, they were, at passage 4, analyzed by flow cytometry. For this, the cultured cells were dissociated with trypsin/Ethylenediaminetetraacetic acid (EDTA) and 1×106 cells were resuspended in 100 μl cold PBS, containing 5% FBS. Thereafter, the cells were incubated for one hour with respective antibodies or isotype-matched control antibodies. Finally, the cells were resuspended in 400 μl PBS. The data obtained from flow cytometry (BD Accuri™ C6) were analyzed with Flowing Software 2.5 program.
Antibodies used for characterization of Ad-MSC were as follows: mouse anti-CD44 polyclonal antibody, rabbit anti-CD34 polyclonal antibody (all from antibodies-online, Aachen, Germany), mouse anti-CD90 monoclonal antibody, rabbit anti-CD11b polyclonal antibody, mouse anti-CD73 monoclonal antibody (all from Novus Biologicals, Littleton, Colorado, USA), rabbit anti-CD105 polyclonal antibody and rabbit anti-CD45 polyclonal antibody (all from Bioss Inc., Woburn, MA, USA) on the surface of Ad-MSCs. For each antibody, it's appropriate isotype control was used.
Ex vivo pretreatment of MSCs
MSCs were treated with various hypoxia-mimicking chemicals. An incubation time of 24 h was selected on the basis of previous studies showing that expression of CXCR4 increased in a time-dependent manner after treatment with VPA (1.0 mM), CoCl2 (100 μM) and DFX (120 μM) [Citation42,43]. After incubation, MSCs were extensively washed with 1x PBS, dissociated from T75 cell culture flasks with Accutase (Sigma-Aldrich) for 5 min at 37°C, centrifuged (at 400 g for 6 min) and resuspended in DMEM prior to in vitro migration assay.
In vitro migration assay
Ad-MSC chemotaxis assay was performed in 24-well plates, based on the Boyden chamber principle using an 8 μm pore size polycarbonate membrane (Costar, corning) [Citation44]. Ad-MSC suspensions in serum-free DMEM, containing 0.5% BSA at a concentration of 25 × 103 cells/ml in 100 µl, were loaded onto the top wells. Then, 100 ng/ml SDF-1α was added to the bottom chamber in a total volume of 600 μl and after 18-h incubation at 37°C and 5% CO2. The non-migrated cells were completely wiped off from the top surface of the filters by a cotton swab. Finally the migrated cells, adhering to the undersurface of the filter membranes, were stained with DAPI and counted. Moreover, the SDF-1α induced migration activity was inhibited by AMD3100, a CXCR4-blocking agent [Citation27], evaluate correlation between Ad-MSCs migration, toward SDF-1α, with upregulation of CXCR4 expression. To this aim, the Ad-MSCs were preincubated with AMD3100, at a concentration of 10 µg/ml for 30 min at room temperature, before chemotaxis test. Each experiment was performed 2 times in triplicate wells. Five fields were counted from each well and the average number of the migrated cells was determined. P values ≤0 .05 were considered as significant.
Conventional and real-time RT-PCR
Total RNA was extracted using TriPure reagent (Roche, Germany) according to the manufacturer's instructions. For all RNA samples quantity and purity were determined using Nanodrop ND-1000 spectrophotometer (Nanodrop, BIO-TEK). First strand cDNA synthesis of both variants of CXCR4 and β actin (ACTB, as housekeeping gene) genes was performed using a cDNA synthesis kit (Fermentas), following the manufacture's protocol. False positives and DNA contamination were controlled by removal of the reverse transcriptase enzyme in the control reactions. The thermal profile for the conventional PCR were 35 cycles for CXCR4-B, 40 cycles for CXCR4-A and 25 cycles for ACTB genes, with each cycle consisting of 94°C for 30 seconds, 57°C for 40 seconds and 72°C for 30 seconds. Amplification was carried out in 25 μl reaction mixtures, containing 0.4 U Taq polymerase (Fermentas). The sequences of primers are shown in .
Table 1. Description of the semi-quantitative RT-PCR primers.
Table 2. Description of the Real time-PCR primers.
Protein extraction and western blotting
Ad-MSCs were washed with ice-cold PBS and the cells were lysed using TriPure (Roch, Germany) for protein extraction according to the manufacturer's protocols with some modifications. Briefly, after centrifugation, the upper phase, containing RNA, was collected for RNA purification and the remaining 2 phases, containing DNA and protein, were aspirated completely. DNA was precipitated with 100% ethanol and precipitated by centrifuging from the organic phase. Acetone and glycerol (2%) were added to the supernatant for precipitation of the proteins. The precipitated proteins were washed 3 times with 0.3 M guanidine hydrochloride, in 95% ethanol and 2% glycerol. The proteins were precipitated in ethanol and 2% glycerol and dissolved in 1% warm sodium dodecyl sulfate polyacrylamide (SDS). For maximum protein dissolution, the precipitate was incubated at 60°C for 10 min, with periodic vortexing. Finally the protein samples were labeled and stored at −80°C until the time for Western blot.
The stored protein samples were thawed and dissolved in 5X sample buffer and subjected to SDS–PAGE gel electrophoresis. The gel separated proteins were transferred onto a polyvinylidene fluoride (PVDF) membrane and then blocked with Tris Buffered Saline (TBS) plus Tween 20 (TBST), containing 5% non-fat dry milk for 3 h at room temperature. The membrane was incubated about 2 h with CXCR4 antibody (Santa-Cruz Biotechnology, 1:300) and ACTB (Santa-Cruz Biotechnology, 1:300) as housekeeping antibody, and was diluted in TBST, containing 3% non-fat dry milk. The immune complexes were then visualized with appropriate horseradish peroxidase-conjugated secondary antibodies (Santa-Cruz Biotechnology, 1:2000) and enhanced chemiluminescence (Bio-Rad, USA). Each experiment was performed 2 times.
Statistical analysis
Statistical analysis was performed using Graph Pad Prism, version 6.0. The significance of difference between the experimental groups and controls was assessed by one-way ANOVA dunnett's test. The results are presented as the means ± standard deviation (SD).
Abbreviations
ACTB | = | β actin |
Ad-MSCs | = | Adipose tissue-derived Mesenchymal Stem Cells |
AMD3100 | = | 1,1′-[1,4-Phenylenebis(methylene)]bis-1,4,8,11-tetraazacyclotetradecane octahydrochloride |
bFGF | = | Basic fibroblast growth factor |
BSA | = | Bovine serum albumin |
CoCl2 | = | Cobalt-Chloride |
DAPI | = | 4′,6-diamidino-2-phenylindole |
DFX | = | deferoxamine mesylate |
DMEM | = | Dulbecco's modified Eagle (DME)-medium |
EDTA | = | trypsin/Ethylenediaminetetraacetic acid |
FBS | = | Fetal bovine serum |
HIF1-α | = | hypoxia-inducible factor 1-α |
HL60 | = | Human promyelocytic leukemia cells |
PBS | = | phosphate buffer saline |
SDF-1α | = | Stromal cell-derived factor-1 α |
SVF | = | Stromal vascular fraction |
VPA | = | Valproic Acid |
Acknowledgments
We thank Mrs. Maryam Sheikh Bahaei for her expert technical assistance.
Additional information
Funding
References
- Nadig RR. Stem cell therapy - Hype or hope? A review. J Conservative Dentistry 2009;12:131–8. doi:10.4103/0972-0707.58329
- Via AG, Frizziero A, Oliva F. Biological properties of mesenchymal stem cells from different sources. Muscles Ligaments Tendons J 2012;2:154–62
- Zimmermann CE, Gierloff M, Hedderich J, et al. Survival of transplanted rat bone marrow-derived osteogenic stem cells in vivo. Tissue Engineering Part A 2011;17:1147–56. doi:10.1089/ten.tea.2009.0577
- Eggenhofer E, Luk F, Dahlke MH, et al. The life and fate of mesenchymal stem cells. Front Immunol 2014;5:148. doi:10.3389/fimmu.2014.00148
- Xing J, Hou T, Jin H, et al. Inflammatory microenvironment changes the secretory profile of mesenchymal stem cells to recruit mesenchymal stem cells. Cell Physiol Biochem 2014;33:905–19. doi:10.1159/000358663
- Andreas K, Sittinger M, Ringe J. Toward in situ tissue engineering: chemokine-guided stem cell recruitment. Trends Biotechnol 2014;32:483–92. doi:10.1016/j.tibtech.2014.06.008
- Pereira CL, Gonçalves RM, Peroglio M, et al. The effect of hyaluronan-based delivery of stromal cell-derived factor-1 on the recruitment of MSCs in degenerating intervertebral discs. Biomaterials 2014;35:8144–53. doi:10.1016/j.biomaterials.2014.06.017
- Honczarenko M, Le Y, Swierkowski M, et al. Human bone marrow stromal cells express a distinct set of biologically functional chemokine receptors. Stem Cells (Dayton, Ohio) 2006;24:1030–41. doi:10.1634/stemcells.2005-0319
- Naderi-Meshkin H, Bahrami AR, Bidkhori HR, et al. Strategies to improve homing of mesenchymal stem cells for greater efficacy in stem cell therapy. Cell Biol Int 2015;39:23–34. doi:10.1002/cbin.10378
- Andreas K, Sittinger M, Ringe J. Toward in situ tissue engineering: chemokine-guided stem cell recruitment. Trends Biotechnol 2014;32:483–92. doi:10.1016/j.tibtech.2014.06.008
- Schulte A, Gunther HS, Phillips HS, et al. A distinct subset of glioma cell lines with stem cell-like properties reflects the transcriptional phenotype of glioblastomas and overexpresses CXCR4 as therapeutic target. Glia 2011;59:590–602. doi:10.1002/glia.21127
- Vagima Y, Lapid K, Kollet O, et al. Pathways implicated in stem cell migration: the SDF-1/CXCR4 axis. Methods Mol Biol 2011;750:277–89. doi:10.1007/978-1-61779-145-1_19
- Ma J, Liu N, Yi B, et al. Transplanted hUCB-MSCs migrated to the damaged area by SDF-1/CXCR4 signaling to promote functional recovery after traumatic brain injury in rats. Neurol Res 2015;37(1):50–56
- Saito Y, Shimada M, Utsunomiya T, et al. Homing effect of adipose-derived stem cells to the injured liver: the shift of stromal cell-derived factor 1 expressions. J Hepato-Biliary-Pancreatic Sci 2014;21(12):873–80
- Delongchamps NB, Beuvon F, Mathieu JR, et al. CXCR4 is highly expressed at the tumor front but not in the center of prostate cancers. World J Urol 2015;33(2):281–7
- Trautmann F, Cojoc M, Kurth I, et al. CXCR4 as biomarker for radioresistant cancer stem cells. Int J Rad Biol 2014;90:687–99
- Jin F, Brockmeier U, Otterbach F, et al. New insight into the SDF-1/CXCR4 axis in a breast carcinoma model: hypoxia-induced endothelial SDF-1 and tumor cell CXCR4 are required for tumor cell intravasation. Mol Cancer Res 2012;10:1021–31
- Krebs MG, Metcalf RL, Carter L, et al. Molecular analysis of circulating tumour cells-biology and biomarkers. Nat Rev Clin Oncol 2014;11:129–44
- Ullah M, Eucker J, Sittinger M, et al. Mesenchymal stem cells and their chondrogenic differentiated and dedifferentiated progeny express chemokine receptor CCR9 and chemotactically migrate toward CCL25 or serum. Stem Cell Res Ther 2013;4:99
- Rombouts WJ, Ploemacher RE. Primary murine MSC show highly efficient homing to the bone marrow but lose homing ability following culture. Leukemia 2003;17:160–70
- Naderi-Meshkin H, Matin MM, Heirani-Tabasi A, et al. Injectable hydrogel delivery plus preconditioning of mesenchymal stem cells: exploitation of SDF-1/CXCR4 axis towards enhancing the efficacy of stem cells' homing. Cell Biol Int 2016;40(7):730–41
- Gupta SK, Pillarisetti K. Cutting edge: CXCR4-Lo: molecular cloning and functional expression of a novel human CXCR4 splice variant. J Immunol 1999;163:2368–72
- Duquenne C, Psomas C, Gimenez S, et al. The Two Human CXCR4 isoforms display different HIV receptor activities: Consequences for the emergence of X4 Strains. J Immunol 2014;193:4188–94
- Bae S, Jeong HJ, Cha HJ, et al. The hypoxia-mimetic agent cobalt chloride induces cell cycle arrest and alters gene expression in U266 multiple myeloma cells. Int J Mol Med 2012;30:1180–6
- Ramesh Reddy B, Kloner RA, Przyklenk K. Early treatment with deferoxamine limits myocardial ischemic/reperfusion injury. Free Radical Biol Med 1989;7:45–52
- Hao H-H, Wang L, Guo Z-J, et al. Valproic acid reduces autophagy and promotes functional recovery after spinal cord injury in rats. Neuroscience Bulletin 2013;29:484–92
- De Clercq E. The bicyclam AMD3100 story. Nat Rev Drug Discov 2003;2:581–7
- Chavakis E, Urbich C, Dimmeler S. Homing and engraftment of progenitor cells: a prerequisite for cell therapy. J Mol Cell Cardiol 2008;45:514–22
- Henschler R, Deak E, Seifried E. Homing of mesenchymal stem cells. Transfusion Med Hemotherapy 2008;35:7
- Viswanathan A. Enhanced expression of N-formyl peptide receptor in mesenchymal stem cells facilitates homing to inflammatory lungs. J Genet Syndr Gene Therapy 2014;05:227
- Ge J, Hu Y, Gui Y, et al. Chemotherapy-induced alteration of SDF-1/CXCR4 expression in bone marrow–derived mesenchymal stem cells from adolescents and young adults with acute lymphoblastic leukemia. J Pediatric Hematol/Oncol 2014;36:617–23
- Lau TT, Wang DA. Stromal cell-derived factor-1 (SDF-1): homing factor for engineered regenerative medicine. Exp Opin Biol Ther 2011;11:189–97
- Bobis-Wozowicz S, Miekus K, Wybieralska E, et al. Genetically modified adipose tissue-derived mesenchymal stem cells overexpressing CXCR4 display increased motility, invasiveness, and homing to bone marrow of NOD/SCID mice. Exp Hematol 2011;39:686–96 e4
- Kean TJ, Lin P, Caplan AI, et al. MSCs: Delivery routes and engraftment, cell-targeting strategies, and immune modulation. Stem Cells Int 2013;2013:732742
- Gupta SK, Pillarisetti K. Cutting edge: CXCR4-Lo: molecular cloning and functional expression of a novel human CXCR4 splice variant. J Immunol 1999;163:2368–72
- Hung S-C, Pochampally RR, Hsu S-C, et al. Short-term exposure of multipotent stromal cells to low oxygen increases their expression of CX3CR1 and CXCR4 and their engraftment in vivo. PLoS One 2007;2(5):e416
- Hou Z, Nie C, Si Z, et al. Deferoxamine enhances neovascularization and accelerates wound healing in diabetic rats via the accumulation of hypoxia-inducible factor-1alpha. Diabetes Res Clin Pract 2013;101(1):62–71
- Yu X, Lu C, Liu H, et al. Hypoxic preconditioning with cobalt of bone marrow mesenchymal stem cells improves cell migration and enhances therapy for treatment of ischemic acute kidney injury. PLoS One 2013;8:e62703, PONE-D-12-39161 [pii]
- Wiehe J, Kaya Z, Homann J, et al. GMP-adapted overexpression of CXCR4 in human mesenchymal stem cells for cardiac repair. Int J Cardiol 2013;167:2073–81
- Secco M, Zucconi E, Vieira NM, et al. Multipotent stem cells from umbilical cord: cord is richer than blood! Stem Cells 2008;26:146–50
- da Silva Meirelles L, Caplan AI, Nardi NB. In search of the in vivo identity of mesenchymal stem cells. Stem Cells (Dayton, Ohio) 2008;26:2287–99
- Liu H, Xue W, Ge G, et al. Hypoxic preconditioning advances CXCR4 and CXCR7 expression by activating HIF-1α in MSCs. Biochem Biophys Res Commun 2010;401:509–15
- Yamanegi K, Yamane J, Kobayashi K, et al. Valproic acid cooperates with hydralazine to augment the susceptibility of human osteosarcoma cells to Fas-and NK cell-mediated cell death. Int J Oncol 2012;41:83–91
- Ganju RK, Brubaker SA, Meyer J, et al. The α-chemokine, stromal cell-derived factor-1α, binds to the transmembrane G-protein-coupled CXCR-4 receptor and activates multiple signal transduction pathways. J Biol Chem 1998;273:23169–75