ABSTRACT
Discoidin domain receptors, DDR1 and DDR2, are two members of collagen receptor family that belong to tyrosine kinase receptor subgroup. Unlike other matrix receptor-like integrins, these collagen receptors have not been extensively studied. However, more and more studies are focusing on their involvement in cancer. These two receptors are present in several subcellular localizations such as intercellular junction or along type I collagen fibers. Consequently, they are involved in multiple cellular functions, for instance, cell cohesion, proliferation, adhesion, migration and invasion. Furthermore, various signaling pathways are associated with these multiple functions. In this review, we highlight and characterize hallmarks of cancer in which DDRs play crucial roles. We discuss recent data from studies that demonstrate the involvement of DDRs in tumor proliferation, cancer mutations, drug resistance, inflammation, neo-angiogenesis and metastasis. DDRs could be potential targets in cancer and we conclude this review by discussing the different ways to inhibits them.
Introduction
During cancer initiation and progression, cells acquire mutations that promote tumor transformation-inducing anarchic proliferation, resistance to apoptosis and the acquisition of invasive abilities. Cells that escape from the primary tumor form metastases resulting in most cases in the death of patients. Hanahan and Weinberg defined and organized the complex features associated with the multistep development of tumors into “hallmarks of cancer”. First, they defined six major hallmarks including: sustenance of proliferative signaling, evasion from tumor suppressors, activation of invasion and metastasis, enable replicative immortality, induce angiogenesis and resistance to cell death [Citation1]. A decade later, in the light of emerging concepts in cancer, they updated these features, taking into account metabolic dysfunctions, genomic instability and mutations, escape to the immune system as well as tumor-promoting inflammation [Citation2]. These supplemental hallmarks highlight the complexity of the tumor development and progression.
Some of these features highlight the importance of the tumor microenvironment (TME) in tumor progression (i.e. invasion and metastasis, angiogenesis, metabolic dysfunction, inflammation). The TME consists of stromal cells, immune cells, tumor-associated vasculature and extracellular matrix (ECM). This environment is dynamic and the tumor cells are involved in bidirectional communications with different components [Citation3]. The ECM is a major component of tumor stroma and is a key player in the maintenance of cells and tissue integrity. During cancer progression, the physical and chemical properties of the ECM are disrupted by tumor and stromal cells. The fibrillar type I collagen, which is the most-abundant ECM protein, undergoes several types of modifications (such as cross-linking, degradation, fibers alignment etc.) and is known to be associated with metastasis and poor patients survival [Citation4,Citation5]. These collagen modifications by tumor or stromal cells will also affect tumor cell progression mediated through collagen binding receptors at the cell surface.
The discoidin domain receptors (DDRs) are unique collagen receptors which, unlike integrins or CD44, possess an intrinsic tyrosine kinase domain. These transmembrane receptors belong to the receptor tyrosine kinase family (RTK) and consist of two members, DDR1 and DDR2. DDR1 is preferentially expressed in epithelial cells whereas DDR2 is preferentially expressed in connective tissues of the embryonic mesoderm [Citation6,Citation7]. DDR1 has 5 isoforms, DDR1 a, b, c, d and e, derived from an alternative splicing at the messenger RNA level; DDR2, on the other hand, has only one known form. All isoforms possess a conserved extracellular domain. The alternative splicing leads to changes in the intracellular juxtamembrane region or in the kinase domain. Isoforms a, b and c are complete isoforms while isoforms d and e have an inactive or no kinase domain, respectively [Citation7]. Unlike other RTKs such as PDGFR, VEGFR or EGFR, DDRs do not bind growth factors but interact with matrix elements and more precisely, collagens [Citation7]. Moreover, unlike other RTKs which dimerize following ligand binding [8, the DDRs pre-exist as homodimers non-covalently bound at the plasma membrane [Citation9–Citation11]. These receptors consist of an extracellular discoidin domain homologous to discoidin I lectin secreted by the amoeba, Dictyostelium discoideum. This discoidin domain is followed by a discoidin-like domain [Citation12]. These two domains share 59% and 51% sequence homology in DDR1 and DDR2, respectively. These are followed by a juxtamembrane region (weakly conserved between DDR1 and DDR2), a transmembrane domain, a large intracellular juxtamembrane region and finally, the catalytic domain conferring the tyrosine kinase activity of these receptors [Citation13].
DDRs are collagen sensors that bind to collagen in their native triple helix form and do not recognize denatured forms such as gelatin [Citation14–Citation16]. However, they are able to recognize and get activated by the monomeric triple-helical form of collagen (the secreted form of unassembled collagen fibers) [Citation17,Citation18]. Fibrillar collagens are recognized by the two receptors whereas other types of collagen are preferentially recognized by one or other receptors. For example, type IV collagen is a ligand for DDR1 but not for DDR214,15 which seems to preferentially bind type II and X collagens [Citation19,Citation20]. Collagen binding promotes conformational modifications of the receptors associated with a slow but sustained self-phosphorylation in comparison to other RTKs whose activation is rapid following ligand binding [Citation14,Citation15]. Indeed, DDR phosphorylation is maximal after a few hours and can be maintained for several hours or even days. Some studies have shown that Src participates in the phosphorylation of DDRs and is required for complete receptor activation [Citation21–Citation23]. Recently, it has been shown that DDR1 activation induces trans phosphorylation at the juxtamembrane and kinase domains of adjacent dimers. Moreover, this phosphorylation requires specific contacts within the transmembrane domains but not in the extracellular domain [Citation24].
DDRs are involved in various physiological functions and are known to be deregulated in cancer [Citation25,Citation26]. In this review, we highlight the important role DDRs play in cancer development and progression.
DDRs: Multitasking receptor family
DDRs are involved in several cellular processes such as cell adhesion, migration and invasion and hence, are associated with several signaling pathways (). Through their binding to collagen, DDRs are known to be involved in proliferation through the activation of the MAPK pathway, in the promotion of pro-survival signal via the PI3K/Akt pathway, in cell adhesion and cell migration [Citation7,Citation25,Citation27]. As a reciprocal effect, DDR1 remodels collagen through its interaction with the myosin IIA, that contracts the fibers [Citation28]. This remodeling of the collagen matrix is known to aid tumor progression [Citation5]. Moreover, DDR1 has been shown to be involved in invasive structures called linear invadosomes, formed through a Tuba-Cdc42 pathway and allowing the degradation of the collagen matrix through matrix-metalloproteinase (MMPs) [Citation29,Citation30]. In addition to its interaction with collagen fibers, DDR1 has been shown to have other collagen-independent functions. In A431 cells (isolated from an epidermoid carcinoma), DDR1 is concentrated at cell/cell junctions where it is involved in cellular cohesion and the collective migration via its association with the E-Cadherin and the polarity complex Par3/Par6 [Citation31]. However, when these cells are in contact with type I collagen fibers, DDR1 is clustered and aligned along these fibers (unpublished data from our laboratory). Hence, the environment does matter vis-à-vis localization and function of DDRs. This type of localization effect at cellular junctions is unknown for DDR2. Moreover, DDR1 is also found at the leading edge of different cell types including fibroblasts [Citation32] and melanoma cells (A375), where it promotes cell migration (unpublished data from our laboratory). We observed DDR2 at the leading edge but this observation was never published or described elsewhere in the literature yet. Taken together, these data demonstrate that DDRs form different subcellular complexes according to the microenvironment with multiple functions (). In our laboratory, we observed that these receptors, when co-expressed in the same cell type as the A375 melanoma cell line, are able to co-localize along type I collagen fibers (unpublished results). However, it is important to note that this co-localization of DDR1 and DDR2 is not systematic. Moreover, in migrating cells, DDR1 or DDR2 can be observed in lamellipodia (unpublished data). Interestingly, all these different localizations could be observed at the same time in the same cell.
Table 1. DDRs signaling pathways involved in the Hallmarks of cancer. The “+” and the “-” represent DDRs promoting (+) or inhibiting (-) different biological functions depending on the cancer cell type. DDR1 is represented in green and DDR2 in red. (1) Malaguarnera et al., 2015, (2) Xiao et al., 2015, (3) Rudra-ganguly et al., 2014, (4) Assent et al., 2015,(5) Chetoui et al., 2011, (6) Hidalgo-Carcedo et al., 2011, (7) Juin et al., 2014, (8) Ezzoukhry et al., 2016,(9) Shimada et al., 2008, (10) Badiola et al., 2011a, (11) Saby et al., 2016, (12) Iwai et al., 2013, (13) Xie et al., 2015, (14) Wang et al., 2016,(15) Xu et al., 2014,(16) Pourdel et al., 2015, (17) Ren et al., 2014.
Figure 1. Different subcellular localization of DDRs in cells. Schematic representation that illustrates different subcellular localizations of DDRs in cells associated with their functions. 1) In A431 cells, DDR1 interacts with E cadherin and the polarity complex Par3/Par6 in order to maintain cell/cell junction. 2) In A375 cells, DDR1 and DDR2 colocalize together along the type I fibrillar collagen. 3) In A375 melanoma cells, on a collagen I matrix, DDR1 co-localizes with Tks5 (a marker of invadosomes). DDR1 activation induces Tuba/Cdc42 pathway leading to linear invadosome formation. 4) In A375 melanoma migrating cells, both DDR1 and DDR2 co-localize with lamellipodia. Some pathways induced by DDR1 activation are represented in this schematic. Scale bar: 5 µM The cells presented in this figure have been transfected by DDR1 tagged with GFP. Anti-DDR2 antibody was purchased from cell signaling (rabbit, 12133S), E-Cadherin antibody was purchased from abcam (mouse, 1416), anti-Tks5 was purchased from Santa Cruz biotechnology (rabbit, sc30122).
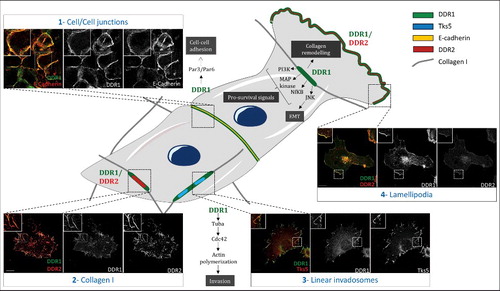
These multiple functions of DDRs correspond to several hallmarks of cancer. In the following paragraphs, we will highlight those in which DDRs are implicated. We will present data showing the involvement of DDRs in cell proliferation, DDRs mutations in cancers, role of DDRs in drug resistance, angiogenesis, inflammation and metastasis formation. However, in most studies, DDR1 and DDR2 are analyzed separately. For this reason, we will illustrate the role of each one apart.
Proliferation
Several publications discussed the role of DDRs in cell proliferation. DDR1 and DDR2 can promote or inhibit proliferation depending on tumor cell type [Citation25].
DDR1
DDR1 induces pro- or anti-apoptotic effect, depending on the context and tumor cell type. DDR1 expression is correlated with increasing tumor proliferation in many cancers: human esophageal [33, gastric cancer [34, glioma [35, breast [36, lung [Citation37]. There are numerous reports on cell proliferation promoting pathways activated by DDR1. In breast cancer, the insulin-like growth factor I is regulated by the activation of DDR1 promoting cell proliferation [Citation36]. In lung cancer cells, DDR1 depletion decreased Erk and Akt phosphorylation leading to reduced cell proliferation. DDR1 activation leads to lung cancer cell proliferation by activation of MAP and PI3k kinase pathways [Citation37]. All these results indicate that DDR1 can act as a major player that promotes tumor cell proliferation. However, one study has demonstrated that, in breast cancer carcinoma, collagen I-DDR1 interaction promotes apoptosis through BIK induction (pro apoptotic Bcl-2 interacting killer protein) [Citation38].
DDR1 involvement in tumor proliferation has been demonstrated in vitro as well as in vivo. In a xenograft mouse model, DDR1 depletion in pancreatic adenocarcinoma led to TGFβI expression and inhibition of tumor cell proliferation in vivo [ Citation39]. DDR1 overexpression promotes proliferation and accelerates tumor growth in xenograft mouse model using gastric cells [Citation34]. DDR1 promotes tumor growth in xenograft mouse model in vivo in osteosarcoma cells [40, human pancreatic adenocarcinoma cells [Citation39] and in bladder cancer cells [Citation41].
DDR2
DDR2 promotes or inhibits proliferation in a context-dependent manner. DDR2 expression promotes tumor cell proliferation in squamous cell lung cancer [42, prostate cancer [Citation43] and melanoma [Citation44]. In A375 melanoma cells, SK-Hepatoma and HT-29 colon carcinoma cell lines, DDR2 depletion decreased cell proliferation. Furthermore, in A375 cells, DDR2 activation induced Jnk pathway leading to tumor cell proliferation [Citation44]. To illustrate this duality, in human fibrosarcoma cell line and in human breast adenocarcinoma, old (extracted from 2-month-old rats) or adult collagen type 1 (extracted from 2-year-old rats) increased or decreased cell proliferation through DDR2, respectively. Indeed, DDR2 activation with adult collagen leads to a decrease in Erk 1 and 2 activity and inhibited proliferation. On the contrary, DDR2 activation by old collagen increases Erk 1 and 2 activity and promotes cell proliferation [Citation45]. Lung cancer is another example of DDR2's double role [Citation46]. In this study, HEK 293K cells with or without DDR2 mutation found in lung cancer were used (refer to mutations paragraph in this review). The authors demonstrated that whilst DDR2 expression activates SHP2 and inhibits cell growth, mutated DDR2 (the same mutations found in lung cancer) does not activate SHP2 but promotes cell growth [Citation46]. In melanoma cell lines, collagen fibers activated DDR2, which inhibited cell proliferation by promoting G0/G1 cell cycle arrest of tumor cells [Citation47].
In vivo, in a xenograft mouse model, dasatinib treatment (inhibitor of tyrosine kinase receptors including DDRs [Citation48] reduced tumor growth of squamous cell lung cancer harboring DDR2 oncogenic mutations [Citation42]. Unfortunately, the number of studies that confirm DDR2 involvement in tumor cell proliferation is not sufficient and its role in this process in vivo remains to be explored further.
Mutations
DDR1 and DDR2 mutations that occur at different position on the receptor sequences have been identified in a large number of cancers [Citation25]. Indeed, most of DDRs mutations have been observed in lung cancer: in small cell lung carcinoma, 2 novel mutations [49, or 11 novel mutations [Citation42] and in primary lung carcinoma 2 novel mutations [Citation50]. Furthermore, DDR1 is mutated in acute myeloid leukemia [Citation51] and endometrial cancers [Citation52]. However, there is no hot spot for mutations in DDRs and there is a lack of functional analysis of mutations. In fact, no correlation has been found between DDR mutations and the overall survival of the patient. On tumor portal, no relevant DDR mutations have been associated with cancer (www.tumorportal.org).
Resistance
Over the past few years, the role of DDRs in the resistance of tumor cells to chemotherapy has emerged. Some studies have demonstrated that DDRs could be a good potential therapeutic target in resistance.
DDR1
Under genotoxic stress, DDR1 activation by p53 induces MAP kinase pathway and increases resistance to apoptosis [Citation53]. Furthermore, in wt-p53 containing cells, cyclooxygenase 2 is upregulated under genotoxic stress and DDR1 activated cyclooxygenase 2 expression by NFKB pathway, leading to the resistance of breast cancer cells to etoposide [Citation54]. Several studies demonstrated that DDR1 expression at protein level promotes the resistance of tumor cells to chemotherapeutic drugs like etoposide in lymphoma [55, cisplastine in ovarian cancer [Citation56] and gefitinib in glioblastoma cells (both at the protein but also at RNA level) [Citation57]. However, molecular mechanisms by which DDR1 leads to chemotherapy resistance remain unknown. In lung adenocarcinoma, DDR1 is required, through the activation of Notch pathway, for Kras G12V driven progression in vivo [ Citation58]. Moreover, in a review, Ambrogio et al. suggest studying tumor samples from patients after relapse in order to analyze the impact of DDR1 mutations in chemo-resistant patients [Citation59]. All these data show that DDR1 is involved in tumor resistance in vitro but its involvement in vivo needs further analysis and confirmation.
DDR2
Contrary to DDR1, little is known about the role of DDR2 in the acquisition of tumor cell resistance to chemotherapy. In one study, it was shown that when non-small cell lung carcinoma acquired resistance to the treatment (dasatinib), the resistance was due to DDR2-T654I mutation [Citation60]. However, DDR2 involvement in drug resistance needs to be further explored.
Tumor-promoting inflammation
During the first steps of tumor progression, the presence of an immune infiltrate – composed of macrophages, lymphocytes T and B, natural killer cells, neutrophils and myeloid-derived suppressor cells – will create a pro-inflammatory environment with the aim of stopping tumor cell progression. The recruitment of these cells to the tumor site requires infiltration through the extracellular matrix [Citation61]. DDR expression has been reported in several immune cell types and is associated with their recruitment and activation.
DDR1
DDR1 is involved in the migration and invasion of T cells in 3D collagen matrices [Citation62,Citation63]. The expression of DDR1 in Th17 cells is promoted by the MAPK/Erk signaling pathway [Citation64]. Two recent studies by the same team highlight that DDR1, through the RhoA-ROCK-MAPK-ERK and p38 pathways, induces the migration of these immune cells in 3D collagen matrices [Citation65,Citation66].
DDR2
DDR2 activation is involved in the migration of neutrophils via the secretion of MMP and the generation of chemotactic peptide gradients derived from collagen in 3D collagen matrices [Citation67]. DDR2 has also been shown to increase bone marrow-derived and monocyte-derived dendritic cell activation via its stimulation by type I collagen [Citation68,Citation69]. Dendritic cells are involved in antigen presentation and the activation of T cells.
Thus, DDR1 and DDR2, through their roles in promoting the migration of immune cells, could participate in the development of a pro-inflammatory program.
Angiogenesis
The growth of new blood vessels from existing vasculature is the main driving force of tumor proliferation. Indeed, tumor neo-angiogenesis is important for delivering oxygen and nutrients to tumor growth, and therefore considered as an essential pathological feature of cancer. Tumor cells secrete cytokines such as vascular endothelial growth factor (VEGF), fibroblast growth factors (FGFs), epidermal growth factor (EGF) and platelet derived growth factor (PDGF) to stimulate sprouting, proliferation and migration of endothelial cells (ECs) by increasing permeability of vessels [Citation70]. For new vessel formation, ECs must degrade the basement membrane and the surrounding ECM, in response to VEGF. For this purpose, ECs form structures called invadosomes which are filamentous actin microdomains with a proteolytic activity [Citation71,Citation72]. ECs form several invadosome-like rosettes, dots [Citation71,Citation73] and linear invadosomes [Citation29]. Furthermore, linear invadosome formation and activity depend on DDR1 in type I collagen context [Citation30].
DDR1
DDRs are implicated in angiogenesis and apart from one study on DDR1's role in arterial wound repair [74, all other studies are on DDR2. In that single DDR1 study, the authors demonstrated a high DDR1 expression both at mRNA and protein levels in balloon-injured rat carotid artery. In addition, they have shown very high DDR1 expression by immunostaining of rat carotid biopsies after injury in vascular smooth muscle cells (SMCs). In vitro, SMCs isolated from DDR1-null mice showed impairment in neointimal formation following arterial injury. This is a result of dramatic decrease of DDR1-null SMCs to attach to collagen, proliferate on collagen and migrate toward collagen, as well as the reduced MMPs activity.
DDR2
Proteomic studies have demonstrated an overexpression of DDR2 in ECs such as HUVEC in response to VEGF stimulation [Citation75,Citation76]. DDR2 was shown to be present in vascular muscle and mural cells but was absent in tumoral pericytes [Citation75,Citation77]. Using immunohistochemistry and immunofluorescence, strong expression level of DDR2 was detected in both tumors (human colon carcinoma and melanoma tissues) and the surrounding ECs. Besides, hypoxic conditions boosted DDR2 expression at mRNA and proteins levels [Citation75], which may explain the enhanced expression of DDR2 in tumor ECs during vascular initiation. Zhang et al. were the first to investigate the relation between DDR2 and angiogenesis. In vivo study by subcutaneous matrigel plug assay of DDR2-expressing HUVEC in nude mice showed an increased vessel sprouting. This effect was increased when cells were mixed with type I collagen [Citation75]. Furthermore, DDR2-mutant mice, named slie (mice have a spontaneous, autosomal-recessive mutation at DDR2 locus and are considered like DDR2 knockout), lost the capacity to form neovessels induced by either VEGF or tumor cells, among other defects like dwarfism and infertility [Citation75]. In addition, the injecting DDR2-expressing adenovirus into slie mice restored capillary vessel formation and enhanced their numbers compared to control mice [Citation75]. Furthermore, this study has found a marked decrease of pro-angiogenic mRNA levels (Vegfr2, Ang-2 and Mmp9) and an intense elevation of anti-angiogenic (Ang-1) mRNA levels in slie mice using real-time PCR analysis. Conversely, the same team has demonstrated an anti-angiogenic effect of DDR2 in ocular disease (Choroidal neovascularization-CNV) [Citation78]. In vivo study by the intravitreal injection of siDDR2 and DDR2-expressing adenovirus has shown that DDR2 is responsible for the severity state of CNV. They also showed that DDR2 negatively regulates the expression of pro-angiogenic factors (VEGF-A and Ang-2) at mRNA and protein levels as well as the activation status of PI3K/Akt/mTOR pathway in CNV context. Similarly, another study showed that DDR2 deficiency enhanced tumor angiogenesis in liver and increased VEGF expression in tumor-derived hepatic stellate cells (HSCs) [Citation79].
It's worth noting that there is a lack of studies that explain the mechanism of DDR2 guiding tumor angiogenesis. Moreover, based on previous investigations showing the link of DDR2 with the microenvironment components such as VEGFR2 and Ang-2 [80, and on its ability to regulate MMPs activity (MMP2 and MMP14) in a collagen rich environment, which is essential for ECs to breach the basement membrane during sprouting [Citation71,Citation81,Citation82], we could propose that these candidates are molecular actors that modulate tumor angiogenesis mediated by DDR2.
All these data suggest that DDR2, more than DDR1, could be a potential player in tumor angiogenesis depending on the cellular context. It is important to elucidate the underlying molecular mechanisms by which DDR2 modulates tumor vessel formation.
Invasion and metastasis
The most important role of the DDRs reported in the literature is their involvement in tumor invasion and metastasis [Citation25,Citation26]. DDRs are involved in several steps of the metastatic process like the epithelial to mesenchymal transition (EMT), the cell/cell junctions and the activation of an invasive behavior by promoting cell migration, ECM degradation and thus, metastasis formation.
DDR1
DDR1 is overexpressed in many cancers such as lung [83,84, breast [85, brain [35, esophagus [33, head and neck [31, liver [86, hepatocellular carcinoma [Citation86] and prostate cancers [Citation87]. In most of these studies, overexpression of DDR1 has been demonstrated at mRNA and / or protein levels and correlates with poor prognosis and the occurrence of metastases [Citation25]. Moreover, DDR1 acts as a major player in breast cancer metastasis reactivation by non-canonical signaling. Actually, atypical tetraspanin protein TM4SF1 promotes non-canonical DDR1 signaling leading to JAK2 and STAT3 activation [Citation88]. All these data suggest that DDR1 could be a biomarker for prognosis and metastasis formation in those cancers.
The EMT is a dynamic and reversible biological process leading to a phenotypic modification of epithelial cells into mesenchymal cells. The mesenchymal phenotype allows cells to acquire migratory and invasive abilities, resist apoptosis and participate in ECM remodelling [Citation89,Citation90]. Several studies have reported a decrease in DDR1 expression during EMT induction in human breast cancer [Citation91]. A potential binding site of the transcription factor ZEB1 has been identified in the DDR1 promoter [92, and was shown to decrease DDR1 expression [Citation91]. ZEB 1 is a transcriptional factor that promotes tumor invasion, metastasis and acts as a driver of EMT and cancer progression. Another study shows that the expression of DDR1 inversely correlates with the progression of the EMT process in epithelial ovarian cancer cell line due to the CpG methylation at the DDR1 promoter [Citation93]. In contrast to these studies, others have shown a role of DDR1 in EMT induction. For example, in a pancreatic cancer cell line, DDR1 cooperated with integrins in EMT induction mediated by type I collagen [Citation94]. In addition, in renal cancer cells, inhibition of DDR1 expression showed a decrease in N-cadherin and other mesenchymal markers and an increase in E-cadherin. Also, in gastric cancer, DDR1 correlates with the expression of the EMT markers (vimentin and Snail), and favors invasion and metastasis [Citation34]. Furthermore, in osteosarcoma cells, E2FI induces DDR1 expression enhancing STAT3 phosphorylation, which promotes EMT [41, cell migration and invasion. E2F1 is a transcription factor that acts as a tumor suppressor, an oncogene and is a driver of EMT. All these data show DDR1's role in promoting EMT [Citation95]. However, the role of DDR1 in EMT cannot be generalized as it depends on the cancer cell type and certainly to the ECM context.
DDR1 could also function independent of collagen binding. DDR1 has been identified at cell/cell junctions in a E-cadherin-dependent manner [Citation31,Citation96] (). Indeed, in A431 squamous cell carcinoma cells, DDR1 has been identified within E-cadherin, Par3 and Par6 complex which is required to maintain cellular cohesion during collective migration [Citation31]. This function is independent of its collagen binding as well as its tyrosine kinase activity. This highlights the complexity of this receptor and that it is not just a collagen sensor. It is therefore necessary to better understand its various functions and their resulting signaling pathways.
Tumor invasion is a result of both cell migration and matrix degradation. DDR1 is also involved in invasion of NSCLC cell lines in vitro [ Citation97]. DDR1 controls MMP expression and thereby modulates ECM remodeling, facilitating migration and invasion in cancer [Citation7]. From a mechanistic point of view, DDR1 induces overexpression of MMP-2 in colon cancer [98, activates MMP9 in NSCLC [Citation83] and overexpresses MMP-2 and MMP-9 in pituitary adenoma lines [Citation99] and in hepatocellular carcinoma cells [Citation100]. DDR1 induces or inhibits cell migration depending on the context or co-factor expression. For example, in MCF7 cells, DDR1 promotes cell migration when it is associated with myosin IIa [Citation32]; on the other hand, when DDR1 is associated with phosphoDARP32, it suppresses cell migration [Citation101]. Furthermore, DDR1 overexpression decreased cell migration in Huh7 cells [Citation102] and in Hs587T breast cancer cells [Citation91]. In our laboratory, we demonstrated that DDR1, through the Rho-GTPase Cdc42 and its GEF Tuba, modulates the actin cytoskeleton leading to linear invadosome formation [Citation30]. In hepatocellular carcinoma, we demonstrated that the activation of TGFβ1 leads to nuclear translocation of Smad4 and an increase in DDR1 mRNA and protein levels inducing linear invadosomes and ECM degradation [Citation103].
As mentioned in the introduction, there are 5 different isoforms DDR1, but most of the studies do not distinguish them, notably isoforms a and b. These isoforms of DDR1 appear to be involved in cell migration and invasion [Citation62,Citation100,Citation104]. In addition, these two isoforms colocalized with E-cadherin at the cell/cell junctions [Citation96]. The isoform b is responsible for the increase in the expression of N-cadherin following its binding to type I collagen through the phosphorylation of its tyrosine residue 513 which induces a signaling via the JNK pathway. The isoform a is not involved in the expression of N-cadherin which is explained by an absence of this tyrosine residue [Citation105]. The differences in tyrosine residues, and more broadly, in sequence between the isoforms could be responsible for the activation of various signaling and interaction with different partners that can lead to specific isoform roles. To date, no study has investigated the role of these isoforms in the same cell model and in the same context.
In vivo
DDR1 role in EMT in vivo has been demonstrated in few studies. For instance, DDR1 inhibited cell migration and invasion in a chariollantoic membrane (CAM) assay [Citation91]. Furthermore, in gastric cancer cells [Citation34] or in osteosarcoma cells, DDR1 is involved in tumor growth in xenograft mouse model [Citation40]. The role of DDR1 in EMT in vivo remains unclear as most studies focus on tumor growth and needs to be investigated further.
Few studies demonstrated DDR1 role in migration and invasion in an in vivo model. In prostate cancer, silencing of PCA-1 (prostate cancer Ag) induced a decrease of DDR1 expression leading to the inhibition of cancer cell invasion in vivo in a CAM [Citation87]. PCA-1 activated DDR1 which induced Bcl-xl and MMP9, promoted cancer invasion and survival. Furthermore, in human pancreatic adenocarcinoma cells [Citation39] or in cancer cell bladder [41, DDR1 promotes tumor growth in xenograft mouse model in vivo.
DDR2
DDR2 involvement in invasion and metastasis processes has also been reported. Several studies on human tissue samples highlight a correlation between DDR2 expression and a poor prognosis in several types of cancers like head and neck squamous cell carcinoma (HNSC) [106,107, nasopharyngeal carcinoma [Citation108], breast carcinoma [Citation109–Citation111], hepatocellular carcinoma [Citation112,Citation113], gastric cancer [Citation114,Citation115], gallbladder squamous cell/adenosquamous carcinoma and adenocarcinoma [Citation116], urothelial carcinoma [Citation117], colorectal cancer [Citation118] and ovarian cancer [Citation119]. DDR2 is also overexpressed in breast cancer metastatic sites [Citation120]. From a mechanistic point of view, DDR2 has been identified in several molecular pathways, modulating different aspects of the invasion process. DDR2 is involved in the EMT process by stabilizing SNAIL1 expression [Citation109] and correlates with SNAIL1 expression in HCC samples [Citation113]. DDR2 is induced during the EMT but is not required for the induction of this process [Citation109,Citation110]. DDR2 expression is also linked with some EMT markers, as it increases vimentin and decreases E-Cadherin expression [Citation113,Citation114]. Moreover, it has been shown that DDR2 can promote the EMT through mTORC2 in a gastric cancer cell line model [Citation114]. Like DDR1, DDR2 is involved in migration and invasion through the induction of MMP2 and MMP9 in several models [Citation106,Citation121,Citation122]. DDR2 induces Erk-MAPK and NF-κB pathways [Citation110,Citation122]. DDR2-induced Erk2/SNAIL1 has been shown to be linked with MT1-MMP and MMP2 expression in HCC cells [Citation113]. DDR2 has also been identified to be a driver gene for gastric cancer dissemination, to promote prostate and breast cancer metastasis [Citation115,Citation120,Citation123].
DDR2 has also been shown to be crucial for the tumor microenvironment impacting the tumor cells dissemination. Using DDR2 knock-out (KO) mice, Badiola et al. showed that DDR2 depletion promotes the transdifferentiation of myofibroblasts leading to a pro-metastatic environment [Citation124]. In contrast, Corsa et al., using DDR2 KO mice, highlight that DDR2 depletion reduces breast cancer metastasis to the lung by affecting collective cell migration but not the tumor growth. They show that DDR2 expressed by stromal cells is important for breast cancer cell metastasis; these cells impact the extracellular matrix deposition and remodeling involved in tumor progression [Citation125]. Another recent study confirmed these data by showing that multipotent stromal cells expressing DDR2 favor matrix deposition and remodeling, inducing breast cancer cell invasion and metastasis [Citation120].
In vivo
Some studies used in vivo models to define the role of DDR2 in tumor cell invasion and metastasis, using DDR2 overexpression or depletion. HNSC model cells overexpressing DDR2 injected in the vein tail revealed an increase in metastatic foci in lung [Citation106]. In another study, breast cancer cells transduced with shRNA against DDR2 injected into the mammary gland showed a decrease in lung metastasis [Citation109]. In another study on breast cancer cells, the authors injected the cells in the mammary fat pads of mice. They observed a decrease of survival and an increase in lung metastasis when cells overexpress DDR2 and the opposite effects in cells with shDDR2110. When melanoma cells transfected with siRNA targeted DDR2 were injected into the spleen, a decrease in liver metastases was observed [Citation121]. In another study, HNSC injected in the Duct of Cuvier in zebrafish showed an increase of invasion with cells overexpressing DDR2; an opposite effect was observed with cells treated with dasatinib [Citation48,Citation107].
Inhibition of the DDRs
DDRs are crucial players in the hallmarks of cancer, notably in invasion and metastasis formation. Furthermore, as discussed before in the invasion part, overexpression of DDRs is correlated with a poor overall survival in many cancers. Consequently, DDR expression could be a potential biomarker and a therapeutic target in cancers.
DDRs may be targeted by tyrosine kinase receptor inhibitors such as dasatinib, imatinib, nilotinib, ponatinib with an IC50 in the low nanomolar range [Citation48,Citation126]. Nilotinib and dasatinib are more potent than imatinib toward DDR1 and DDR2 [Citation48]. These inhibitors are approved by the FDA and could constitute a new treatment for patients overexpressing DDRs in cancers. For example, recently it has been shown that nilotinib inhibits DDR1 kinase activation and reduces invasion in metastatic colorectal cancer [Citation127]. Dasatinib efficiency has been proven several times. Dasatinib inhibits proliferation of DDR2 mutated L63V in squamous cell lung cancer [Citation42] or in head and neck squamous cell carcinoma [Citation128]. Those DDR2 mutations have been shown to enhance dasatinib sensitivity. Furthermore, dasatinib inhibits peritoneal dissemination in a xenograft mouse model of gastric cancer cells overexpressing DDR2 [Citation115]. Dasatinib also inhibits tumor growth in vivo in a xenograft mouse model of lung cancer with DDR2 mutation in combination with BET inhibitor [Citation129]. The combination of dasatinib targeting DDR1 with other chemotherapy (anti-Notch) was shown to be efficient in xenograft mouse model of lung adenocarcinoma leading to decreased tumor cell proliferation [Citation58]. In clinical context, a squamous cell lung tumor in a patient harboring DDR2 mutation shrunk when treated with dasatinib [Citation130]. Additionally, DDRs can be targeted by other tyrosine kinase inhibitors, for example, bafetenib, sorafenib and a thienopyridine (LCB 03–0110) [Citation131]. The disadvantage of these inhibitors is that they not only target DDRs but also other receptor tyrosine kinases. In recent years, selective DDRs kinase inhibitors have emerged. For instance, DDR1 could be targeted specifically by a pyrazolopyramidine alkyne derivative (7rh and 7rj) [Citation132], by DDR1-IN-1 or DDR1-IN-2 [Citation133] and by KST9046 [Citation134]. The efficiency of 7rh has been proven by the tumor regression in xenograft mouse model of pancreatic ductal carcinoma [135, lung adenocarcinoma [59, nasopharyngeal carcinoma [Citation136] and gastric cancer [Citation137]. Regarding DDR2, selective inhibitors are very limited. For instance, DDR2 interaction with collagen could be disrupted by actinomycin D with no effect on DDR1 activation [Citation138]. It has been demonstrated that excess expression of juxtamembrane 2 domain of DDR2 inhibits its activation [Citation139]. In fact, in lung cancer cells, the delivery of gold nano-particle DNA aptamer containing the transmembrane-juxtamembrane 1/2 domain of DDR2 inhibits its activation by collagen, inhibits cell proliferation and invasion [Citation140]. Terai et al. have developed new inhibitors that specifically target DDR2 for treating DDR2-mutated non-small cell lung cancer [Citation141].
In conclusion, DDRs could be a good biomarker in a large number of cancers. Furthermore, these receptors can be targeted using FDA-approved tyrosine kinase inhibitors. Unfortunately, these protein tyrosine kinase inhibitors are not specific of DDRs. However, the emergence in recent years of small molecules that specifically target DDRs could potentially help developing drugs for treating cancers. It could also be interesting to target DDR1 or DDR2 specifically with a blocking antibody. A DDR1 blocking antibody (48B3), developed by Santa Cruz biotechnology, was shown to decrease glioma cell invasion and adhesion mediated by DDR1a [ Citation104].
Conclusions
In this review, we highlight specific hallmarks of cancer where DDRs play crucial roles. Nevertheless, DDRs functions can vary depending on the cellular context. All these data confirm that DDR1 or/and DDR2 could be very interesting targets to block cancer progression at different levels (). Moreover, it clearly appears that there is more data about DDR1 than on DDR2. The kinase activity of these receptors is already targeted by drugs like nilotinib or dasatinib [Citation48]; however, DDRs have some functions independent of this kinase activity such as invasion and cell/cell adhesion. Consequently, it is necessary to find and develop other strategies to target these receptors. Another complexity in targeting DDR1 is its 5 isoforms. It is essential to better understand the roles, the functions and the molecular pathways associated with those isoforms in order to generate specific inhibitors.
Figure 2. DDRs contribution in cancer hallmarks. Representative circular diagram of the hallmarks of cancer, adapted from Hanahan and Weinberg, 2011. In the center, a representative confocal image of a melanoma cancer cell (A375) seeded into collagen fibers (grey) and expressing DDR1 (red). The nucleus is in blue. The difference in the front size is related to the reported involvement of one of hte DDR more than the other in the literature.
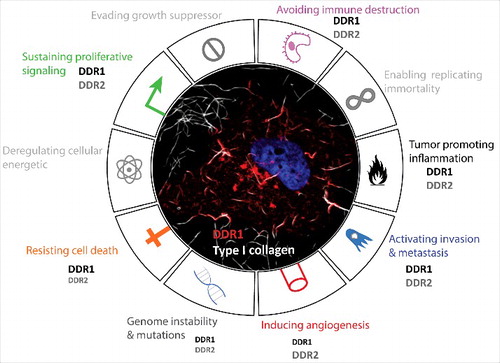
Furthermore, most studies on DDRs focus only on one of the two receptors; however, some cancer cell lines such as melanoma cell lines overexpress both these receptors (). It is essential to determine the relevance and the recurrence of this co-overexpression in cancers. In parallel, the specific role of each receptor and a possible functional compensation in this context has to be established. Indeed, we showed that DDR1 and DDR2 co-localized along type I collagen fibers. This DDR1/DDR2 clustering raises the question of a lateral activation between these two different dimers (as shown for DDR1 dimers) [Citation24]. This review highlights that DDRs are complex receptors localized in different subcellular compartments and are involved in several crucial cellular processes essential for cancer progression. All these data reinforce the necessity to better characterize/understand molecular mechanisms associated with DDRs in cancer.
Disclosure of potential conflicts of interest
No potential conflicts of interest were disclosed.
Acknowledgments
We thank the Bordeaux Imaging Center (BIC) for fluorescence image.
Additional information
Funding
References
- Hanahan D, Weinberg RA. The hallmarks of cancer. Cell [Internet]. 2000;100:57–70. Available from: http://www.ncbi.nlm.nih.gov/pubmed/10647931. doi:10.1016/S0092-8674(00)81683-9.
- Hanahan D, Weinberg RA. Hallmarks of cancer: The next generation. Cell [Internet]. 2011;144:646–74. doi:10.1016/j.cell.2011.02.013.
- Quail DF, Joyce JA. Microenvironmental regulation of tumor progression and metastasis. Nat Med [Internet]. 2013;19:1423–37. Available from: http://www.ncbi.nlm.nih.gov/pubmed/24202395. doi:10.1038/nm.3394.
- Ramaswamy S, Ross KN, Lander ES, et al. A molecular signature of metastasis in primary solid tumors. Nat Genet [Internet]. 2003;33:49–54. Available from: http://www.ncbi.nlm.nih.gov/pubmed/12469122. doi:10.1038/ng1060.
- Levental KR, Yu H, Kass L, et al. Matrix Crosslinking Forces Tumor Progression by Enhancing Integrin Signaling. Cell [Internet]. 2009;139:891–906. doi:10.1016/j.cell.2009.10.027.
- Alves F, Vogel W, Mossie K, et al. Distinct structural characteristics of discoidin I subfamily receptor tyrosine kinases and complementary expression in human cancer. Oncogene [Internet]. 1995;10:609–618. Available from: http://europepmc.org/abstract/MED/7845687
- Leitinger B. Discoidin domain receptor functions in physiological and pathological conditions [Internet]. 1st ed. Elsevier Inc.; 2014. doi:10.1016/B978-0-12-800180-6.00002-5
- Lemmon MA, Schlessinger J. Cell signaling by receptor tyrosine kinases. Cell. 2010;141:1117–34. doi:10.1016/j.cell.2010.06.011.
- Noordeen NA, Carafoli F, Hohenester E, et al. A transmembrane leucine zipper is required for activation of the dimeric receptor tyrosine kinase DDR1. J Biol Chem. 2006;281:22744–51. doi:10.1074/jbc.M603233200.
- Mihai C, Chotani M, Elton TS, et al. Mapping of DDR1 Distribution and Oligomerization on the Cell Surface by FRET Microscopy. J Mol Biol [Internet]. 2009;385:432–45. doi:10.1016/j.jmb.2008.10.067.
- Xu H, Abe T, Liu JKH, et al. Normal activation of discoidin domain receptor 1 mutants with disulfide cross-links, insertions, or deletions in the extracellular juxtamembrane region: Mechanistic implications. J Biol Chem. 2014;289:13565–74. doi:10.1074/jbc.M113.536144.
- Carafoli F, Hohenester E. Collagen recognition and transmembrane signalling by discoidin domain receptors. Biochim Biophys Acta – Proteins Proteomics [Internet]. 2013;1834:2187–97. doi:10.1016/j.bbapap.2012.10.014.
- Leitinger B. Transmembrane collagen receptors. Annu Rev Cell Dev Biol [Internet]. 2011;27:265–90. Available from: http://www.ncbi.nlm.nih.gov/pubmed/21568710. doi:10.1146/annurev-cellbio-092910-154013.
- Shrivastava A, Radziejewski C, Campbell E, et al. An Orphan Receptor Tyrosine Kinase Family Whose Members Serve as Nonintegrin Collagen Receptors. Mol Cell. 1997;1:25–34. doi:10.1016/S1097-2765(00)80004-0.
- Vogel W, Gish GD, Alves F, et al. The Discoidin Domain Receptor Tyrosine Kinases Are Activated by Collagen. Mol Cell [Internet]. 1997;1:13–23. Available from: http://dx.doi.org/10.1016/S1097-2765(00)80003-9%5Cnhttp://www.sciencedirect.com/science/article/pii/S1097276500800039%5Cnhttp://www.sciencedirect.com/science?_ob=MImg&_imagekey=B6WSR-4194JBT-3-K&_cdi=7053&_user=9799020&_pii=S1097276500800039&_origin=&_cov. doi:10.1016/S1097-2765(00)80003-9.
- Leitinger B. Molecular analysis of collagen binding by the human discoidin domain receptors, DDR1 and DDR2. Identification of collagen binding sites in DDR2. J Biol Chem. 2003;278:16761–9. doi:10.1074/jbc.M301370200.
- Xu H, Raynal N, Stathopoulos S, et al. Collagen binding specificity of the discoidin domain receptors: Binding sites on collagens II and III and molecular determinants for collagen IV recognition by DDR1. Matrix Biol [Internet]. 2011;30:16–26. doi:10.1016/j.matbio.2010.10.004.
- Konitsiotis AD, Raynal N, Bihan D, et al. Characterization of high affinity binding motifs for the discoidin domain receptor DDR2 in collagen. J Biol Chem. 2008;283:6861–8. doi:10.1074/jbc.M709290200.
- Leitinger B, Steplewski A, Fertala A. The D2 period of collagen II contains a specific binding site for the human discoidin domain receptor, DDR2. J Mol Biol. 2004;344:993–1003. doi:10.1016/j.jmb.2004.09.089.
- Leitinger B, Kwan APL. The discoidin domain receptor DDR2 is a receptor for type X collagen. Matrix Biol. 2006;25:355–64. doi:10.1016/j.matbio.2006.05.006.
- Yang K, Kim JH, Kim HJ, et al. Tyrosine 740 phosphorylation of discoidin domain receptor 2 by Src stimulates intramolecular autophosphorylation and Shc signaling complex formation. J Biol Chem. 2005;280:39058–66. doi:10.1074/jbc.M506921200.
- Lu KK, Trcka D, Bendeck MP. Collagen stimulates discoidin domain receptor 1-mediated migration of smooth muscle cells through Src. Cardiovasc Pathol [Internet]. 2011;20:71–6. doi:10.1016/j.carpath.2009.12.006.
- Ikeda K, Wang LH, Torres R, et al. Discoidin domain receptor 2 interacts with Src and Shc following its activation by type I collagen. J Biol Chem. 2002;277:19206–12. doi:10.1074/jbc.M201078200.
- Juskaite V, Corcoran DS, Leitinger B. Collagen induces activation of DDR1 through lateral dimer association and phosphorylation between dimers. Elife [Internet]. 2017;6:e25716. Available from: http://elifesciences.org/lookup/doi/10.7554/eLife.25716
- Valiathan RR, Marco M, Leitinger B, et al. Discoidin domain receptor tyrosine kinases: New players in cancer progression. Cancer Metastasis Rev. 2012;31:295–321. doi:10.1007/s10555-012-9346-z.
- Rammal H, Saby C, Magnien K, et al. Discoidin domain receptors: Potential actors and targets in cancer. Front Pharmacol. 2016;7:1–13.
- Payne LS, Huang PH. Discoidin Domain Receptor 2 Signaling Networks and Therapy in Lung Cancer. J Thorac Oncol [Internet]. 2014;9:900–4. doi:10.1097/JTO.0000000000000164.
- Coelho NM, Arora PD, van Putten S, et al. Discoidin Domain Receptor 1 Mediates Myosin-Dependent Collagen Contraction. Cell Rep [Internet]. 2017;18:1774–90. doi:10.1016/j.celrep.2017.01.061.
- Juin A, Billottet C, Moreau V, et al. Physiological type I collagen organization induces the formation of a novel class of linear invadosomes. Mol Biol Cell [Internet]. 2012;23:297–309. doi:10.1091/mbc.E11-07-0594.
- Juin A, Di Martino J, Leitinger B, et al. Discoidin domain receptor 1 controls linear invadosome formation via a Cdc42-Tuba pathway. J Cell Biol. 2014;207:517–33. doi:10.1083/jcb.201404079.
- Hidalgo-Carcedo C, Hooper S, Chaudhry SI, et al. Collective cell migration requires suppression of actomyosin at cell-cell contacts mediated by DDR1 and the cell polarity regulators Par3 and Par6. Nat Cell Biol [Internet]. 2011;13:49–58. Available from: http://www.pubmedcentral.nih.gov/articlerender.fcgi?artid = 3018349&tool = pmcentrez&rendertype = abstract. doi:10.1038/ncb2133.
- Huang Y, Arora P, McCulloch CA, et al. The collagen receptor DDR1 regulates cell spreading and motility by associating with myosin IIA. J Cell Sci [Internet]. 2009;122:1637–46. Available from: http://www.ncbi.nlm.nih.gov/pubmed/19401332%5Cnhttp://jcs.biologists.org/cgi/content/abstract/122/10/1637. doi:10.1242/jcs.046219.
- Nemoto T, Ohashi K, Akashi T, et al. Overexpression of protein tyrosine kinases in human esophageal cancer. Pathobiology. 1997;65:195–203. doi:10.1159/000164123.
- Xie R, Wang X, Qi G, et al. DDR1 enhances invasion and metastasis of gastric cancer via epithelial-mesenchymal transition. Tumor Biol [Internet]. 2016;37:12049–59. doi:10.1007/s13277-016-5070-6.
- Yamanaka R, Arao T, Yajima N, et al. Identification of expressed genes characterizing long-term survival in malignant glioma patients. Oncogene. 2006;25:5994–6002. doi:10.1038/sj.onc.1209585.
- Malaguarnera R, Nicolosi ML, Sacco A, et al. Novel cross-talk between IGF-IR and DDR1 regulates IGF-IR trafficking, signaling and biological responses. Oncotarget. 2015;6:16084–105. doi:10.18632/oncotarget.3177.
- Xiao Q, Jiang Y, Liu Q, et al. Minor Type IV Collagen α 5 Chain Promotes Cancer Progression through Discoidin Domain Receptor-1. PLoS Genet. 2015;11:e1005249. doi:10.1371/journal.pgen.1005249.
- Assent D, Bourgot I, Hennuy B, et al. A Membrane-Type-1 Matrix Metalloproteinase (MT1-MMP) – Discoidin Domain Receptor 1 Axis Regulates Collagen- Induced Apoptosis in Breast Cancer Cells. PLoS One. 2015;10:e0116006. doi:10.1371/journal.pone.0116006.
- Rudra-ganguly N, Lowe C, Mattie M, et al. Discoidin Domain Receptor 1 Contributes to Tumorigenesis through Modulation of TGFBI Expression. PLoS One. 2014;9:1–11. doi:10.1371/journal.pone.0111515.
- Wang Z, Sun X, Bao Y, et al. E2F1 silencing inhibits migration and invasion of osteosarcoma cells via regulating DDR1 expression. Int J Oncol. 2017;51:1639–50. doi:10.3892/ijo.2017.4165.
- Xie X, Rui W, He W, et al. Discoidin domain receptor 1 activity drives an aggressive phenotype in gastric carcinoma. Am J Transl Res [Internet]. 2017;9:2500–7. Available from: http://www.ncbi.nlm.nih.gov/pubmed/28143619%0Ahttp://www.pubmedcentral.nih.gov/articlerender.fcgi?artid = PMC5286810%0Ahttp://bmccancer.biomedcentral.com/articles/10.1186/s12885-017-3051-9
- Hammerman PS, Sos ML, Ramos AH, et al. Mutations in the DDR2 Kinase Gene identify a Novel therapeutic target in squamous cell lung cancer. Cancer Discov. 2011;1:78–89. doi:10.1158/2159-8274.CD-11-0005.
- Azemikhah M, Ashtiani HA, Aghaei M, et al. Evaluation of discoidin domain receptor-2 (DDR2) expression level in normal, benign, and malignant human prostate tissues. Res Pharm Sci. 2015;10:356–63.
- Badiola I, Villacé P, Basaldua I, et al. Downregulation of discoidin domain receptor 2 in A375 human melanoma cells reduces its experimental liver metastasis ability. Oncol Rep. 2011;26:971–8.
- Saby C, Buache E, Brassart-Pasco S, et al. Type I collagen aging impairs discoidin domain receptor 2-mediated tumor cell growth suppression. Oncotarget [Internet]. 2016;7:24908–27. Available from: http://www.oncotarget.com/abstract/8795
- Iwai L., Payne L., Luczynski M., et al. Phosphoproteomics of collagen receptor networks reveals SHP-2 phosphorylation downstream of wild-type DDR2 and its lung cancer mutants. Biochem J. 2013;454:501–13. doi:10.1042/BJ20121750.
- Wall SJ, Werner E, Werb Z, et al. Discoidin Domain Receptor 2 Mediates Tumor Cell Cycle Arrest Induced by Fibrillar Collagen. J Biol Chem. 2005;280:40187–94. doi:10.1074/jbc.M508226200.
- Day E, Waters B, Spiegel K, et al. Inhibition of collagen-induced discoidin domain receptor 1 and 2 activation by imatinib, nilotinib and dasatinib. Eur J Pharmacol [Internet]. 2008;599:44–53. doi:10.1016/j.ejphar.2008.10.014.
- Davies H, Hunter C, Smith R, et al. Somatic mutations of the protein kinase gene family in human lung cancer. Cancer Res. 2005;65:7591–5. doi:10.1158/0008-5472.CAN-05-1855.
- Ding L, Getz G, Wheeler DA, et al. Somatic mutations affect key pathways in lung adenocarcinoma. Nature [Internet]. 2008;455:1069–75. doi:10.1038/nature07423.
- Loriaux MM, Levine RL, Tyner JW, et al. High-throughput sequence analysis of the tyrosine kinome in acute myeloid leukemia High-throughput sequence analysis of the tyrosine kinome in acute myeloid leukemia. Blood. 2008;111:4788–96. doi:10.1182/blood-2007-07-101394.
- Rudd ML, Mohamed H, Price JC, et al. Mutational analysis of the tyrosine kinome in serous and clear cell endometrial cancer uncovers rare somatic mutations in TNK2 and DDR1. BMC Cancer [Internet]. 2014;14:884. Available from: http://bmccancer.biomedcentral.com/articles/10.1186/1471-2407-14-884. doi:10.1186/1471-2407-14-884.
- Ongusaha PP, Kim J il, Fang L, et al. p53 induction and activation of DDR1 kinase counteract p53-mediated apoptosis and influence p53 regulation through a positive feedback loop. EMBO J. 2003;22:1289–301. doi:10.1093/emboj/cdg129.
- Das S, Ongusaha PP, Yang YS, et al. Discoidin domain receptor 1 receptor tyrosine kinase induces cyclooxygenase-2 and promotes chemoresistance through nuclear factor-kappaB pathway activation. Cancer Res. 2006;66:8123–30. doi:10.1158/0008-5472.CAN-06-1215.
- Cader FZ, Vockerodt M, Bose S, et al. The EBV oncogene LMP1 protects lymphoma cells from cell death through the collagen-mediated activation of DDR1. Blood. 2017;122:4237–46. doi:10.1182/blood-2013-04-499004.
- Deng Y, Zhao F, Hui L, et al. Suppressing miR-199a-3p by promoter methylation contributes to tumor aggressiveness and cisplatin resistance of ovarian cancer through promoting DDR1 expression. J Ovarian Res. 2017;1:1–11.
- Aljohani H, Koncar RF, Zarzour A, Park BS, Lee SH, Bahassi El M. ROS1 amplification mediates resistance to gefitinib in glioblastoma cells. Oncotarget. 2015;6:20388–95. doi:10.18632/oncotarget.3981.
- Ambrogio C, Gómez-López G, Falcone M, et al. Combined inhibition of DDR1 and Notch signaling is a therapeutic strategy for KRAS-driven lung adenocarcinoma. Nat Med. 2016;22:270–7. doi:10.1038/nm.4041.
- Ambrogio C, Nadal E, Villanueva A, et al. KRAS-driven lung adenocarcinoma: combined DDR1/Notch inhibition as an effective therapy. ESMO Open [Internet]. 2016;1:e000076. doi:10.1136/esmoopen-2016-000076.
- Beauchamp EM, Woods BA, Dulak AM, et al. Acquired Resistance to Dasatinib in Lung Cancer Cell Lines Conferred by DDR2 Gatekeeper Mutation and NF1 Loss. Mol Cancer Ther. 2014;13:475–83. doi:10.1158/1535-7163.MCT-13-0817.
- Fridman WH, Zitvogel L, Sautès–Fridman C, et al. The immune contexture in cancer prognosis and treatment. Nat Rev Clin Oncol [Internet]. 2017;5–8. Available from: http://www.nature.com/doifinder/10.1038/nrclinonc.2017.101
- Kamohara H, Yamashiro S, Galligan C, et al. Discoidin domain receptor 1 isoform-a (DDR1alpha) promotes migration of leukocytes in three-dimensional collagen lattices. FASEB J. 2001;15:2724–6. doi:10.1096/fj.01-0359fje.
- Hachehouche LN, Chetoui N, Aoudjit F. Implication of discoidin domain receptor 1 in T cell migration in three-dimensional collagen. Mol Immunol [Internet]. 2010;47:1866–9. doi:10.1016/j.molimm.2010.02.023.
- Chetoui N, El azreq M-A, Boisvert M, et al. Discoidin domain receptor 1 expression in activated T cells is regulated by the ERK MAP kinase signaling pathway. J Cell Biochem [Internet]. 2011;112:3666–74. doi:10.1002/jcb.23300.
- Kadiri M, El Azreq M-A, Berrazouane S, et al. Human Th17 Migration in Three-Dimensional Collagen Involves p38 MAPK. J Cell Biochem. 2017;118:2819–27. doi:10.1002/jcb.25932.
- El Azreq M, Kadiri M, Boisvert M, et al. Discoidin domain receptor 1 promotes Th17 cell migration by activating the RhoA / ROCK / MAPK / ERK signaling pathway. Oncotarget. 2016;7:44975–44990. doi:10.18632/oncotarget.10455.
- Afonso P V, Mccann CP, Kapnick SM, et al. Discoidin domain receptor 2 regulates neutrophil chemotaxis in 3D collagen matrices. Blood. 2013;121:1644–51. doi:10.1182/blood-2012-08-451575.
- Lee Y, Moon W, Kim D. Discoidin domain receptor 2 is involved in the activation of bone marrow-derived dendritic cells caused by type I collagen. Biochem Biophys Res Commun. 2007;352:244–50. doi:10.1016/j.bbrc.2006.11.010.
- Poudel B, Yoon D, Lee J, et al. Collagen I enhances functional activities of human monocyte-derived dendritic cells via discoidin domain receptor 2. Cell Immunol [Internet]. 2012;278:95–102. doi:10.1016/j.cellimm.2012.07.004.
- Rafii S, Lyden D, Benezra R, et al. Vascular and haematopoietic stem cells: Novel targets for anti-angiogenesis therapy? Nat Rev Cancer. 2002;2:826–35. doi:10.1038/nrc925.
- Seano G, Chiaverina G, Gagliardi PA, et al. Endothelial podosome rosettes regulate vascular branching in tumour angiogenesis. Nat Cell Biol. 2014;16:931–8. doi:10.1038/ncb3036.
- Seano G, Daubon T, Génot E, et al. Podosomes as novel players in endothelial biology. Eur J Cell Biol [Internet]. 2014;93:405–12. doi:10.1016/j.ejcb.2014.07.009.
- Tatin F, Varon C, Genot E, et al. A signalling cascade involving PKC, Src and Cdc42 regulates podosome assembly in cultured endothelial cells in response to phorbol ester. J Cell Sci [Internet]. 2006;119:769–81. doi:10.1242/jcs.02787.
- Hou G, Vogel W, Bendeck MP. The discoidin domain receptor tyrosine kinase DDR1 in arterial wound repair. J Clin Invest [Internet]. 2001;107:727–35. Available from: http://www.jci.org/articles/view/10720. doi:10.1172/JCI10720.
- Zhang S, Bu X, Zhao H, et al. A host deficiency of discoidin domain receptor 2 (DDR2) inhibits both tumour angiogenesis and metastasis. J Pathol. 2014;232:436–48. doi:10.1002/path.4311.
- Katanasaka Y, Asai T, Naitou H, et al. Proteomic Characterization of Angiogenic Endothelial Cells Stimulated with Cancer Cell-Conditioned Medium. Biol Pharm Bull. 2007;30:2300–7. doi:10.1248/bpb.30.2300.
- Chen SC, Wang BW, Wang DL, et al. Hypoxia induces discoidin domain receptor-2 expression via the p38 pathway in vascular smooth muscle cells to increase their migration. Biochem Biophys Res Commun. 2008;374:662–7. doi:10.1016/j.bbrc.2008.07.092.
- Zhu T, Zhu J, Bu X, et al. The anti-angiogenic role of discoidin domain receptor 2 (DDR2) in laser-induced choroidal neovascularization. J Mol Med. 2015;2:187–98. doi:10.1007/s00109-014-1213-7.
- Badiola I, Olaso E, Crende O, et al. Discoidin domain receptor 2 deficiency predisposes hepatic tissue to colon carcinoma metastasis. Gut. 2012;61:1465–72. doi:10.1136/gutjnl-2011-300810.
- Augustin HG, Young Koh G, Thurston G, et al. Control of vascular morphogenesis and homeostasis through the angiopoietin – Tie system. Nat Rev Mol Cell Biol. 2009;10:165–77. doi:10.1038/nrm2639.
- Olaso E, Labrador J-P, Wang L, et al. Discoidin domain receptor 2 regulates fibroblast proliferation and migration through the extracellular matrix in association with transcriptional activation of matrix metalloproteinase-2. J Biol Chem [Internet]. 2002;277:3606–13. Available from: http://www.jbc.org/content/277/5/3606.long. doi:10.1074/jbc.M107571200.
- Majkowska I, Shitomi Y, Ito N, et al. Discoidin domain receptor 2 mediates collagen-induced activation of membrane-type 1 matrix metalloproteinase in human fibroblasts. J Biol Chem. 2017;292:6633–43. doi:10.1074/jbc.M116.770057.
- Yang SH, Baek HA, Lee HJ, et al. Discoidin domain receptor 1 is associated with poor prognosis of non-small cell lung carcinomas SUN. Oncol Rep. 2010;24:311–9.
- Valencia K, Ormazábal C, Zandueta C, et al. Inhibition of collagen receptor discoidin domain receptor-1 (DDR1) reduces cell survival, homing, and colonization in lung cancer bone metastasis. Clin Cancer Res. 2012;18:969–80. doi:10.1158/1078-0432.CCR-11-1686.
- Barker KT, Martindale JE, Mitchell PJ, et al. Expression patterns of the novel receptor-like tyrosine kinase, DDR, in human breast tumours. Oncogene. 1995;10:569–75.
- Shen Q, Cicinnati VR, Zhang X, et al. Role of microRNA-199a-5p and discoidin domain receptor 1 in human hepatocellular carcinoma invasion. Mol Cancer [Internet]. 2010;9:227. Available from: http://www.pubmedcentral.nih.gov/articlerender.fcgi?artid = 2939569&tool = pmcentrez&rendertype = abstract. doi:10.1186/1476-4598-9-227.
- Shimada K, Nakamura M, Ishida E, et al. Prostate cancer antigen-1 contributes to cell survival and invasion though discoidin receptor 1 in human prostate cancer. Cancer Sci. 2008;99:39–45.
- Gao H, Chakraborty G, Zhang Z, et al. Multi-organ Site Metastatic Reactivation Mediated by Non- canonical Discoidin Domain Receptor 1 Signaling. Cell. 2016;166:47–62. doi:10.1016/j.cell.2016.06.009.
- Thiery JP, Acloque H, Huang RYJ, et al. Epithelial-Mesenchymal Transitions in Development and Disease. Cell. 2009;139:871–90. doi:10.1016/j.cell.2009.11.007.
- Nieto MA. The ins and outs of the epithelial to mesenchymal transition in health and disease. Annu Rev Cell Dev Biol [Internet]. 2011;27:347–76. Available from: http://www.annualreviews.org/doi/pdf/10.1146/annurev-cellbio-092910-154036. doi:10.1146/annurev-cellbio-092910-154036.
- Koh M, Woo Y, Valiathan RR, et al. Discoidin domain receptor 1 is a novel transcriptional target of ZEB1 in breast epithelial cells undergoing H-Ras-induced epithelial to mesenchymal transition. Int J Cancer. 2015;136:508–20. doi:10.1002/ijc.29154.
- Taube JH, Herschkowitz JI, Komurov K, et al. Core epithelial-to-mesenchymal transition interactome gene-expression signature is associated with claudin-low and metaplastic breast cancer subtypes. Proc Natl Acad Sci [Internet]. 2010;107:15449–54. Available from: http://www.pnas.org/cgi/doi/10.1073/pnas.1015095107%5Cnpapers2://publication/doi/10.1073/pnas.1015095107. doi:10.1073/pnas.1004900107.
- Chung VY, Tan TZ, Huang R, et al. Loss of discoidin domain receptor 1 (DDR1) via CpG methylation during EMT in epithelial ovarian cancer. Gene [Internet]. 2017;635:9–15. doi:10.1016/j.gene.2017.09.001.
- Shintani Y, Fukumoto Y, Chaika N, et al. Collagen I-mediated up-regulation of N-cadherin requires cooperative signals from integrins and discoidin domain receptor. J Cell Biol. 2008;180:1277–89. doi:10.1083/jcb.200708137.
- Song J, Chen X, Bai J, et al. Discoidin domain receptor 1 (DDR1), a promising biomarker, induces epithelial to mesenchymal transition in renal cancer cells. Tumor Biol [Internet]. 2016;37:11509–21. doi:10.1007/s13277-016-5021-2.
- Wang C, Yeh Y, Tang M-J. DDR1 / E-cadherin complex regulates the activation of DDR1 and cell spreading. Am J Physiol Cell Physiol [Internet]. 2009;297:419–29. Available from: http://www.ncbi.nlm.nih.gov/pubmed/19474292. doi:10.1152/ajpcell.00101.2009.
- Miao L, Zhu S, Wang Y, et al. Discoidin domain receptor 1 is associated with poor prognosis of non-small cell lung cancer and promotes cell invasion via epithelial-to-mesenchymal transition. Med Oncol. 2013;30:626. doi:10.1007/s12032-013-0626-4.
- Hu Y, Liu J, Jiang B, et al. MiR-199a-5p Loss Up-Regulated DDR1 Aggravated Colorectal Cancer by Activating Epithelial-to-Mesenchymal Transition Related Signaling. Dig Dis Sci. 2014;59:2163–72. doi:10.1007/s10620-014-3136-0.
- Yoshida D, Teramoto A. Enhancement of pituitary adenoma cell invasion and adhesion is mediated by discoidin domain receptor-1. J Neurooncol. 2007;82:29–40. doi:10.1007/s11060-006-9246-6.
- Park HS, Kim KR, Lee HJ, et al. Overexpression of discoidin domain receptor 1 increases the migration and invasion of hepatocellular carcinoma cells in association with matrix metalloproteinase. Oncol Rep. 2007;18:1435–41.
- Hansen C, Greengard P, Nairn AC, et al. Phosphorylation of DARPP-32 regulates breast cancer cell migration downstream of the receptor tyrosine kinase DDR1. Exp Cell Res. 2006;312:4011–8. doi:10.1016/j.yexcr.2006.09.003.
- Song S, Shackel NA, Wang XM, et al. Discoidin Domain Receptor 1. Am J Pathol [Internet]. 2011;178:1134–44. Available from: http://linkinghub.elsevier.com/retrieve/pii/S0002944010002051. doi:10.1016/j.ajpath.2010.11.068.
- Ezzoukhry Z, Henriet E, Piquet L, et al. TGF-β1 promotes linear invadosome formation in hepatocellular carcinoma cells, through DDR1 up-regulation and collagen I cross-linking. Eur J Cell Biol [Internet]. 2016;95:503–12. doi:10.1016/j.ejcb.2016.09.003.
- Ram R, Lorente G, Nikolich K, et al. Discoidin domain receptor-1a (DDR1a) promotes glioma cell invasion and adhesion in association with matrix metalloproteinase-2. J Neurooncol. 2006;76:239–48. doi:10.1007/s11060-005-6874-1.
- Huang H, Svoboda RA, Lazenby AJ, et al. Up-Regulation of N-Cadherin by Collagen I-activated discoidin domain receptor 1 in pancreatic cancer requires the adaptor molecule Shc. J Biol Chem. 2016;291:23208–23. doi:10.1074/jbc.M116.740605.
- Xu J, Lu W, Zhang S, et al. Overexpression of DDR2 contributes to cell invasion and migration in head and neck squamous cell carcinoma. Cancer Biol Ther [Internet]. 2014;15:612–22. Available from: http://www.tandfonline.com/doi/abs/10.4161/cbt.28181. doi:10.4161/cbt.28181.
- von Mässenhausen A, Sanders C, Brägelmann J, Konantz M, et al. Targeting DDR2 in head and neck squamous cell carcinoma with dasatinib. Int J Cancer. 2016;139:2359–69. doi:10.1002/ijc.30279.
- Chua H, Yeh T, Wang Y, et al. Upregulation of discoidin domain receptor 2 in nasopharyngeal carcinoma. Head Neck. 2008;30:427–36.
- Zhang K, Corsa CA, Ponik SM, et al. The collagen receptor discoidin domain receptor 2 stabilizes SNAIL1 to facilitate breast cancer metastasis. Nat Cell Biol [Internet]. 2013;15:677–87. doi:10.1038/ncb2743.
- Ren T, Zhang W, Liu X, et al. Discoidin domain receptor 2 (DDR2) promotes breast cancer cell metastasis and the mechanism implicates epithelial-mesenchymal transition programme under hypoxia. J Pathol. 2014;234:526–37. doi:10.1002/path.4415.
- Toy KA, Valiathan RR, Núñez F, et al. Tyrosine kinase discoidin domain receptors DDR1 and DDR2 are coordinately deregulated in triple-negative breast cancer. Breast Cancer Res Treat. 2015;150:9–18. doi:10.1007/s10549-015-3285-7.
- Park J-W, Lee Y-S, Kim JS, et al. Downregulation of discoidin domain receptor 2 decreases tumor growth of hepatocellular carcinoma. J Cancer Res Clin Oncol [Internet]. 2015;1973–83. Available from: http://www.ncbi.nlm.nih.gov/pubmed/25842034. doi:10.1007/s00432-015-1967-5.
- Xie B, Lin W, Ye J, et al. DDR2 facilitates hepatocellular carcinoma invasion and metastasis via activating ERK signaling and stabilizing SNAIL1. J Exp Clin cancer Res [Internet]. 2015;34:101. Available from: http://jeccr.biomedcentral.com/articles/10.1186/s13046-015-0218-6. doi:10.1186/s13046-015-0218-6.
- Wang YG, Xu L, Jia RR, et al. DDR2 Induces Gastric Cancer Cell Activities via Activating mTORC2 Signaling and Is Associated with Clinicopathological Characteristics of Gastric Cancer. Dig Dis Sci. 2016;61:2272–83. doi:10.1007/s10620-016-4116-3.
- Kurashige J, Hasegawa T, Niida A, et al. Integrated Molecular Profiling of Human Gastric Cancer Identifies DDR2 as a Potential Regulator of Peritoneal Dissemination. Sci Rep. 2016;6:1–11. doi:10.1038/srep22371.
- Li D, Yang Z, Liu Z, et al. DDR2 and IFITM1 Are Prognostic Markers in Gallbladder Squamous Cell/Adenosquamous Carcinomas and Adenocarcinomas. Pathol Oncol Res. 2017;1–11. doi:10.1007/s12253-017-0314-3. [Epub ahead of print]
- Tsai M-C, Li W-M, Huang C-N, et al. DDR2 overexpression in urothelial carcinoma indicates an unfavorable prognosis: a large cohort study. Oncotarget. 2016;7:78918–31. doi:10.18632/oncotarget.12912.
- Sasaki S, Ueda M, Iguchi T, et al. DDR2 Expression Is Associated with a High Frequency of Peritoneal Dissemination and Poor Prognosis in Colorectal Cancer. Anticancer Res [Internet]. 2017;37:2587–91. Available from: http://ar.iiarjournals.org/content/37/5/2587.abstract. doi:10.21873/anticanres.11603.
- Fan Y, Xu Z, Fan J, et al. Prognostic significance of discoidin domain receptor 2 (DDR2) expression in ovarian cancer. Am J Transl Res. 2016;8:2845–50.
- Gonzalez ME, Martin E, Anwar T, et al. Mesenchymal stem cell induced DDR2 mediates stromal-breast cancer interactions and metastasis growth. Cell Rep. 2017;18:1215–28. doi:10.1016/j.celrep.2016.12.079.
- Badiola I, Villacé P, Basaldua I, et al. Downregulation of discoidin domain receptor 2 in A375 human melanoma cells reduces its experimental liver metastasis ability. Oncol Rep. 2011;26:971–8.
- Poudel B, Lee YM, Kim DK. DDR2 inhibition reduces migration and invasion of murine metastatic melanoma cells by suppressing MMP2/9 expression through ERK/NF-κB pathway. Acta Biochim Biophys Sin (Shanghai). 2015;47:292–8. doi:10.1093/abbs/gmv005.
- Yan Z, Jin S, Wei Z, et al. Discoidin domain receptor 2 facilitates prostate cancer bone metastasis via regulating parathyroid hormone-related protein. Biochim Biophys Acta – Mol Basis Dis [Internet]. 2014;1842:1350–63. Available from: http://linkinghub.elsevier.com/retrieve/pii/S0925443914001057. doi:10.1016/j.bbadis.2014.04.018.
- Badiola I, Olaso E, Crende O, et al. Discoidin domain receptor 2 deficiency predisposes hepatic tissue to colon carcinoma metastasis. Gut [Internet]. 2012;61:1465–72. Available from: http://gut.bmj.com/lookup/doi/10.1136/gutjnl-2011-300810. doi:10.1136/gutjnl-2011-300810.
- Corsa CAS, Brenot A, Grither WR, et al. The Action of Discoidin Domain Receptor 2 in Basal Tumor Cells and Stromal Cancer-Associated Fibroblasts Is Critical for Breast Cancer Metastasis. Cell Rep [Internet]. 2016;15:2510–23. doi:10.1016/j.celrep.2016.05.033.
- Canning P, Tan L, Chu K, et al. Structural mechanisms determining inhibition of the collagen receptor DDR1 by selective and multi-targeted type II kinase inhibitors. J Mol Biol [Internet]. 2014;426:2457–70. doi:10.1016/j.jmb.2014.04.014.
- Jeitany M, Leroy C, Tosti P, et al. Inhibition of DDR 1 -BCR signalling by nilotinib as a new therapeutic strategy for metastatic colorectal cancer. EMBO Mol Med. 2018;10:1–16.
- Hedberg ML, Goh G, Chiosea SI, et al. Genetic landscape of metastatic and recurrent head and neck squamous cell carcinoma. J Clin Invest. 2015;126:169–80. doi:10.1172/JCI82066.
- Xu C, Buczkowski KA, Zhang Y, et al. NSCLC Driven by DDR2 Mutation Is Sensitive to Dasatinib and JQ1 Combination Therapy. Mol Cancer Ther [Internet]. 2015;14:2382–9. Available from: http://mct.aacrjournals.org/cgi/doi/10.1158/1535-7163.MCT-15-0077. doi:10.1158/1535-7163.MCT-15-0077.
- Pitini V, Arrigo C, Di Mirto C, et al. Response to dasatinib in a patient with SQCC of the lung harboring a discoid-receptor-2 and synchronous chronic myelogenous leukemia. Lung Cancer [Internet]. 2013;82:171–2. doi:10.1016/j.lungcan.2013.07.004.
- Li Y, Lu X, Ren X, et al. Small molecule discoidin domain receptor kinase inhibitors and potential medical applications. J Med Chem. 2015;58:3287–301. doi:10.1021/jm5012319.
- Gao M, Duan L, Luo J, et al. Discovery and optimization of 3-(2-(Pyrazolo[1,5- a]pyrimidin-6-yl) ethynyl)benzamides as novel selective and orally bioavailable discoidin domain receptor 1 (DDR1) inhibitors. J Med Chem. 2013;56:3281–95. doi:10.1021/jm301824k.
- Kim H-G, Tan L, Weisberg EL, et al. Discovery of a Potent and Selective DDR1 Receptor Tyrosine Kinase Inhibitor. ACS Chem Biol [Internet]. 2013;8:2145–50. Available from: http://pubs.acs.org/doi/abs/10.1021/cb400430t. doi:10.1021/cb400430t.
- Elkamhawy A, Park J-E, Cho N-C, et al. Discovery of a broad spectrum antiproliferative agent with selectivity for DDR1 kinase: cell line-based assay, kinase panel, molecular docking, and toxicity studies. J Enzyme Inhib Med Chem. 2016;31:158–66. doi:10.3109/14756366.2015.1004057.
- Aguilera KY, Huang H, Du W, et al. Inhibition of Discoidin Domain Receptor 1 Reduces Collagen-mediated Tumorigenicity in Pancreatic Ductal Adenocarcinoma. Mol Cancer Ther [Internet]. 2017;16:2473–85. Available from: http://mct.aacrjournals.org/lookup/doi/10.1158/1535-7163.MCT-16-0834. doi:10.1158/1535-7163.MCT-16-0834.
- Lu Q, Chen W, Peng J, et al. Antitumor activity of 7RH, a discoidin domain receptor?1 inhibitor, alone or in combination with dasatinib exhibits antitumor effects in nasopharyngeal carcinoma cells. Oncol Lett [Internet]. 2016;3598–608. Available from: http://www.spandidos-publications.com/10.3892/ol.2016.5088. doi:10.3892/ol.2016.5088.
- Hur H, Ham I-H, Lee D, et al. Discoidin domain receptor 1 activity drives an aggressive phenotype in gastric carcinoma. BMC Cancer. 2017;17:87. doi:10.1186/s12885-017-3051-9.
- Siddiqui K, Kim GW, Lee DH, et al. Actinomycin D identified as an inhibitor of discoidin domain receptor 2 interaction with collagen through an insect cell based screening of a drug compound library. Biol Pharm Bull. 2009;32:136–41. doi:10.1248/bpb.32.136.
- Kim D, Ko P, You E, et al. The intracellular juxtamembrane domain of discoidin domain receptor 2 (DDR2) is essential for receptor activation and DDR2-mediated cancer progression. Int J Cancer. 2014;135:2547–57. doi:10.1002/ijc.28901.
- Kim D, Yeom JH, Lee B, et al. Inhibition of discoidin domain receptor 2-mediated lung cancer cells progression by gold nanoparticle-aptamer-assisted delivery of peptides containing transmembrane-juxtamembrane 1/2 domain. Biochem Biophys Res Commun [Internet]. 2015;464:392–5. doi:10.1016/j.bbrc.2015.06.044.
- Terai H, Tan L, Beauchamp EM, et al. Characterization of DDR2 Inhibitors for the Treatment of DDR2 Mutated Nonsmall Cell Lung Cancer. ACS Chem Biol. 2015;10:2687–96. doi:10.1021/acschembio.5b00655.