Abstract
The efficacy of curcumin supplementation is traditionally limited due to its poor bioavailability. Despite this, curcumin has previously been shown to improve biomarkers of muscle damage. The addition of a novel drug delivery system that improves bioavailability could improve exercise recovery. The purpose of this randomized double-blind placebo-controlled study was to assess the effect of curcumin (combined with LipiSperse) when consumed as a drink on exercise recovery in recreationally trained healthy males aged 18–35 yrs. The study included 28 young healthy males with strength training experience. The participants undertook lower limb resistance exercise to exhaustion. Fourteen participants received curcumin dispersed in water pre and postexercise and 14 received a matched placebo drink. Pain (visual analogue scale), thigh circumference (TC), lactate, creatine kinase, lactate dehydrogenase, high sensitivity C-reactive protein, myoglobin, interleukin-6, interleukin-10, and tumor necrosis factor-alpha were assessed pre, postexercise and 1, 2, 3, 24, 48, and 72 h postexercise. There was less appearance of postexercise capillary lactate in the curcumin group compared to placebo (7.4 vs 8.8 mmol/L). The placebo group rated overall muscle pain as higher compared to the curcumin group at 48- and 72-h postexercise. TC was reduced in the curcumin group compared to the placebo group at 24- and 48-h postexercise. The results suggest curcumin may facilitate a quicker return to exercise training and/or allow a higher training intensity than a placebo by reducing postexercise pain, modulating inflammatory pathways and reducing lactate accumulation in an exercising population.
Introduction
Recovery is an important factor for athletes in the exercise and competition phase (Rodriguez et al. Citation2009; Peake, Neubauer, Walsh, et al. Citation2017). Successful recovery from an exercise bout allows for a quicker return to the next bout, and possibly allows for a higher intensity to be achieved or maintained (Carfagno and Hendrix Citation2014). Increasing the intensity and volume of exercise in a given period may allow for improved adaptations and performance in subsequent bouts (Carfagno and Hendrix Citation2014; Dankel et al. Citation2017). Typically, the limiting factor to repeated exercise bouts is delayed onset muscle soreness (DOMS). DOMS is pain and stiffness from muscle damage sustained from exercise (Nicol et al. Citation2015) and may limit physical function and activity for several days (Lewis et al. Citation2012). Traditionally, non-steroidal anti-inflammatory drugs are used to help alleviate DOMS (Lewis et al. Citation2012; Schoenfeld Citation2012). However, long-term use of such drugs may have damaging effects on muscle growth (Schoenfeld Citation2012) and have several side effects (Harirforoosh et al. Citation2013). Thus, alternative therapies for exercise recovery are required.
A natural compound shown to have anti-inflammatory actions and minimal side effects is curcumin (Salehi et al. Citation2019). Specifically, curcumin may reduce inflammatory cytokine and prostaglandin production by modifying the COX-2 pathway signaling (McFarlin et al. Citation2016). Several studies report beneficial outcomes for curcumin as an exercise recovery aid (Davis et al. Citation2007; Kawanishi et al. Citation2013; Drobnic et al. Citation2014; Nicol et al. Citation2015; Tanabe et al. Citation2015; McFarlin et al., Citation2016; Delecroix et al. Citation2017; Jager et al. Citation2019). One study by McFarlin et al. (Citation2016) reported that biomarkers of muscle damage (creatine kinase; CK) decreased by ∼47% after eccentric exercise. McFarlin et al. (Citation2016) showed curcumin supplementation reduced CK, interleukin (IL)-8 and tumor necrosis factor-alpha (TNF-α) during recovery but had no effect on subjective muscle soreness. Similarly, Tanabe et al. (Citation2015) found curcumin reduced serum CK activity and attenuated a decrease in maximal voluntary contraction torque compared to a placebo. In 2014, Drobnic et al. found magnetic resonance imaging evidence that muscle injury was lower, and less pain was reported, in the lower limbs of participants taking curcumin compared to a placebo (Drobnic et al. Citation2014). Furthermore, several studies have shown that curcumin is effective at reducing IL-1β (Kowluru and Kanwar Citation2007), TNF-α (Kuhad et al. Citation2007) and C-reactive protein (CRP; Banerjee et al. Citation2003) and improving flow mediated dilation (Oliver et al. Citation2016).
Unfortunately, the traditionally poor oral bioavailability of curcumin limits the full potential curcumin may provide. Curcumin is extremely hydrophobic due to its structure, aromatic groups and high molecular weight. This hydrophobic nature leads to agglomeration, resulting in poor gastrointestinal absorption and low bioavailability of free curcumin (Ammon and Wahl Citation1991). We have previously shown that a novel delivery system (containing medium chain triglycerides, citrus oil, lecithin, olive oil, polyglycerol polyricinoleate, silica, vitamin E acetate, and mono/diglycerides of fatty acids) was able to improve the bioavailability of curcumin in healthy adults (Briskey et al. Citation2019). Therefore, the aim of this study is to assess the effect a new curcumin formulation (HydroCurc) with a novel delivery system, LipiSperse, has on exercise recovery compared to a placebo. Briefly, LipiSperse is a dispersion technology specifically designed to increase the bioavailability of lipophilic actives. Typically in an aqueous environment, curcumin aglomerates, limiting absorbtion. LipiSperse creates a repulsive force between particles preventing the agglomeration typically seen and thus increasing absorption. It was therefore hypothesized that curcumin supplementation will reduce DOMS by reducing inflammation and CK compared to a placebo.
Methods
A double blind, placebo-controlled study conducted over 72 h. Twenty-eight male participants with experience in strength training (completing at least 2 strength training sessions a week for 6 months in the last 12 months) aged 18–35 yrs were recruited. Experimental procedures and potential risks were explained, and written informed consent was collected prior to participation. Prior to commencing, this study was registered with the Australia and New Zealand Clinical Trials Registry (trial ID: ACTRN12618000285257) and was approved by the Bellberry Human Research Ethics Committee (HREC 2017-11-841) and conformed to the 1964 Declaration of Helsinki and its later amendments.
Exclusion criteria included any uncontrolled or serious illness, use of long-term medications, malignancy or treatment for malignancy within the previous 2 yrs, smoking, non-stable diet, chronic past or current alcohol abuse, allergy to any study ingredients, serious mood disorders, insomnia, night-shift employment, any diagnosed neurological conditions, recent musculoskeletal injuries, and participation in any other clinical trial in the past 3 months. Inclusion criteria included normal dietary habits, body mass index between 18.5 to 35 kg/m2, recreationally trained and achieving at least 150 min of exercise per week, and at least 6 months of resistance training experience in the past year.
After an overnight fast (10 h), participants attended the exercise clinic between 6 a.m. and 8 a.m. to complete measures of height, weight, waist, hip, and thigh circumference (TC), resting brachial blood pressure, and resting heart rate. A venous blood sample from an antecubital vein was obtained and a capillary blood measure of lactate (LAC) was taken from a finger (The Edge Lactate Analyzer; Apex Biotechnology Corporation, Taiwan). Participants completed a visual analogue scale of pain (PVAS) by placing a mark on a continuous 100 mm line when asked about their current whole-body pain (0 = no pain; 100 = maximum pain ever experienced). The PVAS was then measured to the nearest mm.
Once baseline measurements were complete, participants were randomized (sealedenvelope.com, London, UK) to either the placebo group or curcumin group and consumed their allocated study product; 1 g of powder (500 mg HydroCurc with 500 mg of maltodextrin or 1 g of maltodextrin) mixed with 250 mL of water in an opaque bottle. The premixed drinks were created by a third party not associated with the study to adhere to the double-blind protocol. The 500 mg of HydroCurc consists of 450 mg of a curcumin extract (containing 95% or 427 mg of curcuminoids) with 50 mg of the delivery system, LipiSperse (Briskey et al. Citation2019).
Thirty minutes after the consumption of the study product participants completed a 5-min warm-up at a self-selected pace on a cycle ergometer. Participants then stretched major muscle groups of the lower limb followed by 10 repetitions of leg press at 50% of estimated 1 repetition maximum (1RM) and 2-min rest. The weight on the leg press was then increased to approximately 70% of 1RM and participants completed 4 to 6 repetitions followed by 2-min rest. Weight was added onto the leg press to approximately 90% of estimated 1RM and participants completed 1 repetition followed by 2-min rest. Weight was then increased to 100% of estimated 1RM and participants attempted to complete a repetition. If successful, weight was increased, and if unsuccessful weight was decreased. Participants were given up to 7 attempts to achieve 1RM. Participants were rested for 3 min in between attempts. Once 1RM testing was complete, participants rested for 5 min.
Participants then completed 4 sets of leg press at 80% of 1RM. For each set, the participant was asked to perform as many repetitions as possible, until volitional exhaustion. Each repetition was completed as fast as possible with maximal intent. The participants rested for 60 s between each set. Each repetition was recorded using an accelerometer-based system attached to the leg press sled to determine repetition velocity and power (Push Band 2.0; Push Inc., Toronto, Canada). Once the fourth set was complete, participants rested for 5 min and were then taken through testing for their 1RM again.
Immediately post the second 1RM test, the following measurements were completed; finger prick lactate, venous blood sample, PVAS, and TC. The participants cooled down by cycling at a self-selected pace for 5 min. The same measurements were again completed at 1, 2, and 3 h post the completion of the second 1RM test. During this time the participants were rested and consumed water ad libitum. Immediately after the cool down was complete participants were given 2 snack bars (1020 kJ, 4.4 g protein, 7.2 g fat, 37.4 g carbohydrate) to consume along with their study product. After 1 h of rest the participants were prepared 2 sandwiches; 4 slices of white bread, 40 g of fruit jam (1856 kJ, 11.1 g protein, 2.7 g fat, 89.9 g carbohydrates) to eat within a 30-min time window.
At 2 h and 45 min post the second 1RM test the participants performed a third 1RM test after a 5-min self-selected pace cycling warm-up. After a 5-min rest period the participants performed 1 set of leg press exercise at 70% of 1RM achieved at baseline. The participants were instructed to complete as many repetitions as possible with maximal leg speed. A 5-min cycling warm-down was completed at a self-selected pace.
The participants returned at 24, 48, and 72 h after the completion of the second 1RM test for interviews, data collection and study product consumption (24- and 48-h time points only). Participants completed the following measurements during these interviews; LAC, venous blood sample, PVAS, and TC. On completion of the study participants were paid $150 AUD and asked exit interview questions. To examine the “belief” effect a follow-up questionnaire was presented to participants inquiring about which product they believed they were on and whether they would use the product again.
Blood was collected into ethylenediaminetetraacetic acid plasma and serum vacutainers (BD, Plymouth, UK). Plasma samples were immediately centrifuged, whilst serum samples were incubated at room temperature for 30 min before centrifugation at 1500g for 10 min at 4 °C. Separated plasma and serum were aliquoted and stored at −80 °C until biochemical assays were performed.
All data analyses were completed using STATA software (version 14; StataCorp LLC, College Station, TX). Normality testing of data was performed using the Shapiro-Wilk test. Linear mixed models were used for repeated measures when data were normally distributed. Tukey’s post hoc test was utilized for multiple comparisons. Significance was assumed when p < 0.05. A sample size of 14 per group was calculated based on the power to detect a change of 20% in CK (300 IU/L down to 240 IU/L). Effect size: 0.857, alpha error probability: 0.05, power: 0.8.
Results
Twenty-seven male participants completed the entire study protocol (1 participant withdrew after 24 h due to an unrelated bacterial infection). All outcome variables were normally distributed. There were no differences between groups for baseline participant characteristics ().
Table 1. Participant baseline characteristics and clinical measures, mean ± SD.
Curcumin supplementation significantly reduced DOMS pain at 48- and 72-h postexercise compared to the placebo group (; p < 0.05). Curcumin significantly reduced TC between groups at 24 and 48-h time points (; p < 0.05). Lactate was significantly higher postexercise than at baseline in both groups (; p < 0.05), with the curcumin group having a significantly lower post exercise concentration compared to the placebo group (p < 0.05; ). IL-10 was significantly higher in the curcumin group at 24-h post exercise compared to the placebo group (; p < 0.01). IL-6 was significantly higher at 1-h (; p < 0.05), 24-h (p < 0.01) and 72-h (p < 0.05) postexercise in the curcumin group compared to the placebo group. No differences were observed between groups for CK, lactate dehydrogenase (LDH), myoglobin (Mb), high sensitivity-CRP or TNF-α () for any time points.
Figure 1. Pain Visual Analogue Scale (mm) in curcumin and placebo groups at all timepoints. “*” denotes difference between groups, “a” denotes difference from baseline (curcumin), "b" denotes difference from baseline (placebo), “c” denotes difference from postexercise (curcumin), “d” denotes difference from postexercise (placebo) (p < 0.05).
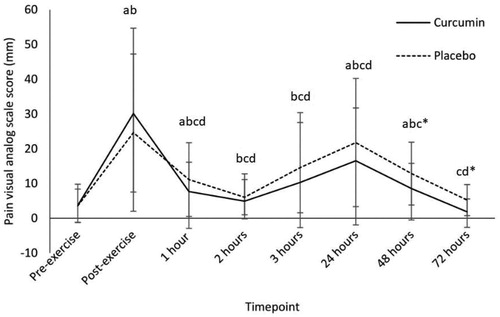
Figure 2. Thigh circumference (cm)—adjusted means ± SD. “*” denotes significant difference between groups (p < 0.05).
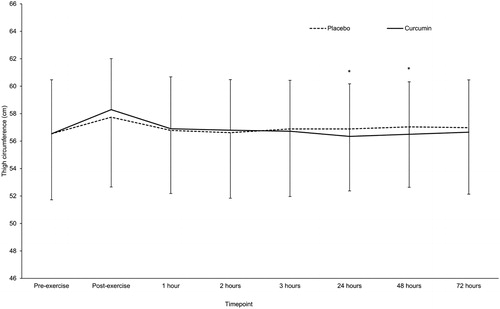
Figure 3. Capillary lactate (mmol/L)—adjusted means ± SD. “*” denotes significant difference between groups (p < 0.05).
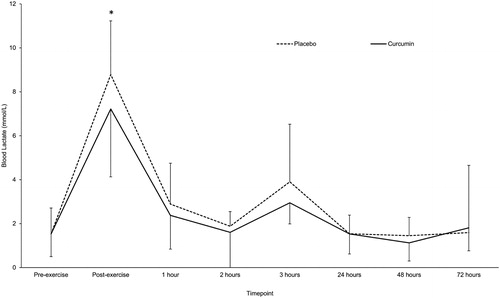
Table 2. Muscle damage and inflammation plasma biomarkers, mean ± SD.
Table 3. Biomechanical output from leg press exercise, mean ± SD.
The average leg press 1RM, was more than 3 times bodyweight (between an intermediate and advanced lifter for normative data). Leg press power was greater for the third set in curcumin group compared to the placebo (; p < 0.05). There was no difference between groups in average velocity or number of repetitions performed in the set. There were no differences between groups for 1RM leg press. Out of those that responded to the post-study survey, 55% of the curcumin group (6 of 11 responders) stated that they would use the product again compared to 31% of the placebo group (4 of 13 responders). No participant in the curcumin group believed the product caused them more pain than normal, however, 15% (2 of 13 responders) of the placebo group thought they experienced more pain than usual.
Discussion
The aim of this study was to assess the effect of orally dosed curcumin on acute exercise recovery compared to a placebo. Our main hypothesis was that curcumin supplementation would reduce DOMS (pain) by reducing inflammation and CK. This hypothesis was partially supported.
The reduced pain experienced by the curcumin group at 48 h postexercise suggests that a return to training may be possible earlier than the placebo group (). A quicker return to exercise training, or less pain at training, may lead to improved training adaptations and subsequently improved exercise performance. This finding is supported by previous studies showing an improvement in exercise recovery/DOMS following curcumin supplementation (Davis et al. Citation2007; Kawanishi et al. Citation2013; Nicol et al. Citation2015; Tanabe et al. Citation2015; McFarlin et al. Citation2016). However, although there was significantly lower perceived pain in the curcumin group, this was not reflected in CK as previously shown (Nicol et al. Citation2015) (). One possible explanation may be the reduced lactate production prevented a decrease in muscle pH, subsequently reducing acidosis related pain and/or tissue breakdown from occurring (). With no differences between groups in Mb and CK this would suggest that curcumin did not protect against exercise induced muscle damage.
One of the novel findings of this study is the observed capillary blood lactate accumulation being lower immediately postexercise in the curcumin group compared to the placebo group (). The reduction in blood lactate likely explains why participants in the curcumin group were also able to maintain a higher power in the third set of leg press exercises (). One possible reason for this effect may be that curcumin is acting as a buffer to prevent the formation of lactic acid. Alternatively, the reduced appearance of lactate in the blood could suggest that curcumin interacts with the energy metabolism pathway. Blood lactate concentration increases when the tricarboxylic acid (TCA) cycle can no longer receive pyruvate to metabolize (i.e., saturation). Pyruvate is then reduced to lactate via LDH and NADH conversion to NAD + in an oxygen poor environment. This could be due to a few reasons, e.g., decreased TCA enzymes, increased pyruvate concentration, reduced cellular oxygen capacity, etc.
Another important finding was the change in plasma inflammatory markers. Participants who consumed curcumin had increased blood IL-10 (anti-inflammatory) concentration 24 h postexercise compared to the placebo group (). This increase in anti-inflammatory signaling could explain the quicker return to baseline TC that was seen in the curcumin group compared to the placebo group. A stable blood concentration of IL-6 in the curcumin group could also be interacting with IL-10 and TC. IL-6 is known to interact with IL-10, activating the anti-inflammatory properties of IL-10 (Kovacs Citation2010; Jin et al. Citation2013). A quicker return to baseline TC indicates less swelling from extra-cellular fluid (associated with DOMS, inflammation and decreased exercise performance) is occurring in the curcumin group (Proske and Morgan Citation2001; Peake, Neubauer, Della Gatta, et al. Citation2017).
It must be noted that the changes in lactate accumulation occurred after a single dose of curcumin (). This pronounced effect demonstrates that even a small dose of curcumin has potential effect on the energy metabolism pathway. Whether this effect is enhanced with a larger dose of curcumin or whether daily supplementation continues this trend remains unknown and are subjects of future research.
Though we have shown a number of differences between the biochemical, inflammatory and protein markers of exercise recovery between the curcumin and placebo groups, much still remains unanswered. How these changes affect exercise performance and recovery in the long term remains unclear. Whether curcumin could affect exercise recovery in an endurance exercise population, specifically in lactate production and power output, would be an interesting direction for future studies. It must also be noted that the observed changes in this study could be due to increased bioavailability of curcumin through the LipiSperse advanced dispersion technology as shown in our previous work (Briskey et al. Citation2019). LipiSperse increased the max concentration of plasma curcumin by ∼3× and total area under the curve by ∼2× compared to standard curcumin. The increased bioavailability may have contributed to changes observed; however, we did not have a comparator standard curcumin treatment arm in this study.
Although all efforts to develop and execute a rigorously designed study were made, there some limitations we would like to acknowledge. Ideally, we would have had a cross-over design, but we felt that this may introduce a training effect and diminish the DOMS effect for the cross-over. Furthermore, it would have also meant a long washout period extending the study by months. Secondly, completion of the 1RM on a separate day would have been ideal. However, from our experience that makes subject recruitment and retention harder. Therefore, all efforts were made to ensure all participants completed the same protocol to minimize any effect that may have caused.
Conclusion
The results suggest that curcumin with added LipiSperse may allow for a quicker return to exercise training, or to exercise at higher thresholds than the placebo drink. This may be due to an interaction between IL-6 and IL-10 eliciting anti-inflammatory properties which reduce TC, pain, and modulate energy metabolism. It is recommended that future studies examine the effects of curcumin in chronic exercise performance for both endurance and resistance type exercise.
Author contributions
Conceptualization: Alistair R. Mallard, David Briskey, and Amanda Rao; Methodology: Alistair R. Mallard, David Briskey, Andrew Richards, and Amanda Rao; Formal analysis and investigation: Alistair R. Mallard, David Briskey, Andrew Richards, and Amanda Rao; Funding acquisition: Amanda Rao; Writing—original draft: Alistair R. Mallard, and David Briskey; Writing—review and editing: Alistair R. Mallard, David Briskey, Andrew Richards, and Amanda Rao.
Declaration of interest
The authors declare no conflicts of interest. The authors alone are responsible for the content and writing of the article.
Additional information
Funding
Notes on contributors
Alistair R. Mallard
Alistair R. Mallard, PhD is a Postdoctoral Research Fellow at the University of Queensland, Australia. He is an exercise biochemist and physiologist who specialises in redox biology. He has worked both in industry and academia and has special interests in the effect of different exercise training techniques and supplementation on the quality and quantity of musculature gains.
David Briskey
David Briskey, PhD is a research fellow at The University of Queensland, Brisbane, Australia. His area of expertise is in biochemistry and biomedical science. In recent years his particular area of focus has been in nutraceuticals with a particular focus on inflammation and pain pathways. His interest in this area has been created by new technology being created that enables previously poorly absorbed substances to be absorbed at levels that make them potentially therapeutic. He has no known conflict of interests and no financial interest with any industry ties.
Andrew Richards, BExSSc
Andrew M. Richards, BExSSc is an accredited exercise scientist with a bachelors degree from the Australian Catholic University and works within the injury prevention field. He has previously worked with a elite Australian Rules Football team with a focus on load management and post match recovery and monitoring. His interest in recovery supplementation comes from a wish to provide his athletes with the best available supplements to aid in recovery.
Amanda Rao
Amanda Rao, MSc is the founder and Managing Director of RDC Clinical, a clinical research organisation specialising in Integrative and Complementary Medicines. She is trained in physiology and biochemistry with further expertise in endocrinology and hormonal changes over the lifespan. Her interest is in increasing the evidence base for supplements and herbal extracts to support health and improve quality of life as we age. She has no financial industry ties.
References
- Ammon HP, Wahl MA. 1991. Pharmacology of Curcuma longa. Planta Med. 57(1):1–7. doi:10.1055/s-2006-960004.
- Banerjee M, Tripathi L, Srivastava V, Puri A, Shukla RJI. 2003. Modulation of inflammatory mediators by ibuprofen and curcumin treatment during chronic inflammation in rat. Immunopharmacol Immunotoxicol. 25(2):213–224. doi:10.1081/IPH-120020471.
- Briskey D, Sax A, Mallard AR, Rao A. 2019. Increased bioavailability of curcumin using a novel dispersion technology system (LipiSperse). Eur J Nutr. 58(5):2087–2011. doi:10.1007/s00394-018-1766-2.
- Carfagno DG, Hendrix JC III. 2014. Overtraining syndrome in the athlete: current clinical practice. Curr Sports Med Rep. 13(1):45–51. doi:10.1249/JSR.0000000000000027.
- Dankel SJ, Mattocks KT, Jessee MB, Buckner SL, Mouser JG, Counts BR, Laurentino GC, Loenneke JP. 2017. Frequency: the overlooked resistance training variable for inducing muscle hypertrophy? Sports Med. 47(5):799–805. doi:10.1007/s40279-016-0640-8.
- Davis JM, Murphy EA, Carmichael MD, Zielinski MR, Groschwitz CM, Brown AS, Gangemi JD, Ghaffar A, Mayer EP. 2007. Curcumin effects on inflammation and performance recovery following eccentric exercise-induced muscle damage. Am J Physiol Regul Integr Comp Physiol. 292(6):R2168–R2173. doi:10.1152/ajpregu.00858.2006.
- Delecroix B, Abaidia AE, Leduc C, Dawson B, Dupont G. 2017. Curcumin and piperine supplementation and recovery following exercise induced muscle damage: a randomized controlled trial. J Sports Sci Med. 16(1):147–153.
- Drobnic F, Riera J, Appendino G, Togni S, Franceschi F, Valle X, Pons A, Tur J. 2014. Reduction of delayed onset muscle soreness by a novel curcumin delivery system (Meriva): a randomised, placebo-controlled trial. J Int Soc Sports Nutr. 11:31. doi:10.1186/1550-2783-11-31.
- Harirforoosh S, Asghar W, Jamali F. 2013. Adverse effects of nonsteroidal antiinflammatory drugs: an update of gastrointestinal, cardiovascular and renal complications. J Pharm Pharm Sci. 16(5):821–847. doi:10.18433/J3VW2F.
- Jager R, Purpura M, Kerksick CM. 2019. Eight weeks of a high dose of curcumin supplementation may attenuate performance decrements following muscle-damaging exercise. Nutrients. 11(7):1692. doi:10.3390/nu11071692.
- Jin JO, Han X, Yu Q. 2013. Interleukin-6 induces the generation of IL-10-producing Tr1 cells and suppresses autoimmune tissue inflammation. J Autoimmun. 40:28–44. doi:10.1016/j.jaut.2012.07.009.
- Kawanishi N, Kato K, Takahashi M, Mizokami T, Otsuka Y, Imaizumi A, Shiva D, Yano H, Suzuki K. 2013. Curcumin attenuates oxidative stress following downhill running-induced muscle damage. Biochem Biophys Res Commun. 441(3):573–578. doi:10.1016/j.bbrc.2013.10.119.
- Kovacs E. 2010. Interleukin-6 leads to interleukin-10 production in several human multiple myeloma cell lines. Does interleukin-10 enhance the proliferation of these cells? Leuk Res. 34(7):912–916. doi:10.1016/j.leukres.2009.08.012.
- Kowluru RA, Kanwar M. 2007. Effects of curcumin on retinal oxidative stress and inflammation in diabetes. Nutr Metab (Lond). 4(1):8. doi:10.1186/1743-7075-4-8.
- Kuhad A, Pilkhwal S, Sharma S, Tirkey N, Chopra K. 2007. Effect of curcumin on inflammation and oxidative stress in cisplatin-induced experimental nephrotoxicity. J Agric Food Chem. 55(25):10150–10155. doi:10.1021/jf0723965.
- Lewis PB, Ruby D, Bush-Joseph CA. 2012. Muscle soreness and delayed-onset muscle soreness. Clin Sports Med. 31(2):255–262. doi:10.1016/j.csm.2011.09.009.
- McFarlin BK, Venable AS, Henning AL, Sampson JN, Pennel K, Vingren JL, Hill DW. 2016. Reduced inflammatory and muscle damage biomarkers following oral supplementation with bioavailable curcumin. BBA Clin. 5:72–78. doi:10.1016/j.bbacli.2016.02.003.
- Nicol LM, Rowlands DS, Fazakerly R, Kellett J. 2015. Curcumin supplementation likely attenuates delayed onset muscle soreness (DOMS). Eur J Appl Physiol. 115(8):1769–1777. doi:10.1007/s00421-015-3152-6.
- Oliver JM, Stoner L, Rowlands DS, Caldwell AR, Sanders E, Kreutzer A, Mitchell JB, Purpura M, Jäger R. 2016. Novel form of curcumin improves endothelial function in young, healthy individuals: a double-blind placebo controlled study. J Nutr Metab. 2016:1089653. doi:10.1155/2016/1089653.
- Peake JM, Neubauer O, Della Gatta PA, Nosaka K. 2017. Muscle damage and inflammation during recovery from exercise. J Appl Physiol. 122(3):559–570. doi:10.1152/japplphysiol.00971.2016.
- Peake JM, Neubauer O, Walsh NP, Simpson RJ. 2017. Recovery of the immune system after exercise. J Appl Physiol. 122(5):1077–1087. doi:10.1152/japplphysiol.00622.2016.
- Proske U, Morgan DL. 2001. Muscle damage from eccentric exercise: mechanism, mechanical signs, adaptation and clinical applications. J Physiol (Lond). 537(Pt 2):333–345. doi:10.1111/j.1469-7793.2001.00333.x.
- Rodriguez NR, Di Marco NM, Langley S. 2009. American College of Sports Medicine position stand. Nutrition and athletic performance. Med Sci Sports Exerc. 41(3):709–731. doi:10.1249/MSS.0b013e31890eb86.
- Salehi B, Stojanovic-Radic Z, Matejic J, Sharifi-Rad M, Anil Kumar NV, Martins N, Sharifi-Rad J. 2019. The therapeutic potential of curcumin: a review of clinical trials. Eur J Med Chem. 163:527–545. doi:10.1016/j.ejmech.2018.12.016.
- Schoenfeld BJ. 2012. The use of nonsteroidal anti-inflammatory drugs for exercise-induced muscle damage: implications for skeletal muscle development. Sports Med. 42(12):1017–1028. doi:10.2165/11635190-000000000-00000.
- Tanabe Y, Maeda S, Akazawa N, Zempo-Miyaki A, Choi Y, Ra S-G, Imaizumi A, Otsuka Y, Nosaka K. 2015. Attenuation of indirect markers of eccentric exercise-induced muscle damage by curcumin. Eur J Appl Physiol. 115(9):1949–1957. doi:10.1007/s00421-015-3170-4.