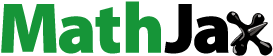
Abstract
North East India is a highly seismically active zone of India due to the collision tectonics between the Indian and the Eurasian plate in the north and subduction tectonics along the Indo-Myanmar range (IMR) in the east. It is utmost important to investigate the seismicity of this region along with uniform hazard spectra (UHS). In this paper, seismic hazard study has been performed at the Silchar district headquarters of Assam state, located in North East India. The earthquake data have been collected from various agencies from 1762 to 2020 and the fault data has been collected from seismotectonic atlas (SEISAT). Earthquake and fault data are superimposed on the Northeast India map using MapInfo software. The fault zone is defined based upon the earthquake’s density near the fault. Seismicity parameters and maximum magnitude have been estimated using Gutenberg-Richter (G-R) relationship. The best-fitted ground motion equation is considered. The outcome is represented in terms of controlling source, seismic hazard curve and UHS for Silchar city with varying ground motions. Finally, the results are presented as PGA based upon DSHA and PSHA methods. The effect of Importance Factor (I) and Response reduction factor (R) have been investigated by comparing UHS with IS: 1893-2016 code response spectra.
1. Introduction
There are several different types of natural hazards in India, such as earthquakes, cyclones, landslides, floods, and tsunamis involving natural and artificial causes. As per the seismic zonation map, North East India has been considered in zone v, whose average PGA value is 0.36g (IS 1893:2016, Citation2016). In North East India, some significant earthquakes have occurred in the past like North East region Cachar 1869(Mw7.4), Shillong1897 (Mw 8.1), Assam1950 (Mw 8.6), Shrimangal 1918(Ms 7.6), Dhubri1930 (Ms 7.1), Manipur1988 (Mw 7.2), Dibrugarh EQ 2015 (Mw 5.5), Manipur 2016 (Mw 6.8) and Sikkim 2011(Mw 6.9). As a result of earthquakes that cause severe damage to people and significant structures such as dams, roads, bridges, and buildings, it is essential to design these structures after assessing the ground motions to minimize further damage. Thus, it becomes very important to correctly estimate the seismic hazard of any given location so that the buildings can be constructed with adequate earthquake resistance to reduce the effects of earthquakes. The purpose of seismic hazard analysis is to estimate ground motion values to estimate horizontal forces due to an earthquake so that buildings can be designed to resist the forces. Seismic risk is the combination of vulnerability and hazard. So, risk can be minimized only by considering vulnerability, but the hazard is a natural phenomenon that cannot be controlled.
According to Verma & Bansal (Citation2013), seismic hazard studies were started in the country after the Shillong earthquake (Mw: 8.1) in 1897, and the Geological Survey of India was the first agency to take the initiative in India in 1898. NDMA (Citation2011) performed the probabilistic hazard analysis of India by dividing the whole of India into seven geographical regions, and 32 fault source zone and past Fault and earthquake data have been considered to define the seismic characteristics of the source zones. The whole country has been divided into (0.2 × 0.2) grid in the paper, and new attenuation equations have been developed for each region. Probabilistic analysis has been done, and the hazards are computed in terms of peak ground acceleration (PGA) and short and long-period spectral acceleration for different return periods. For a different type of site, correction factors have been applied according to standard codes. Sharma and Malik (Citation2006) and Nath and Thingbaijam (Citation2012) have performed probabilistic Seismic Hazard analysis (PSHA) of India.
Also, studies are available on seismic hazard analysis for different cities in India, such as Devi and Sarbeswar (Citation2014) conducted a hazard analysis by the PSHA method for North East India and its surrounding area . Menon et al. (Citation2010) have performed a probabilistic seismic hazard analysis of the South Indian state of Tamil Nadu. Baro et al. (Citation2020) conducted a hazard study for the Shillong plateau region. Raghu Kanth and Iyengar (Citation2006) have carried out seismic hazard studies on Mumbai city, and Joshi et al. (Citation2007) have plotted a seismic Hazard map for North East India. Sil et al. (Citation2013) have performed seismic hazard assessments for Tripura and Mizoram states. Bahuguna and Sil (Citation2018) have performed a hazard study for Assam state. Raghu Kanth et al. (Citation2009) conducted seismic hazard analysis by the DSHA method for Imphal city, and Das et al. (Citation2016) performed a probabilistic seismic Hazard map of North East India.
Aman et al. (Citation1995) and Singh et al. (Citation1996) have developed attenuation relations for strong seismic ground motion in the Himalayan region. Ahmed and Singh (Citation2016) developed GMPE for the Gorkha Nepal Earthquake of 25 April 2015. These ground motion prediction equations are helpful in estimating hazards.
The seismicity of any particular region changes as the number of earthquakes and the maximum magnitude of earthquakes increase, which affects seismic hazard of the region. In the present study, Seismic hazard curves and uniform Hazard spectra are plotted for the city by considering revised fault and earthquake events, which were not considered in the previous study. Additionally, uniform hazard spectra were not correlated with the Indian code (IS 1893:2016, Citation2016) hazard spectra in any of the cases, which was important since the designer use these spectra to assess the seismic hazard for the structures. So, the earthquake hazard study needs to incorporate updated seismicity along with the Codal provisions, which may assist disaster management and mitigation. A study of seismic hazards for Silchar city has been conducted in this paper. shows the location of Silchar district headquarters with a longitude of 92.80 and latitude of 24.82 in the Assam state and the seismotectonic map of North East India. According to Haloi and Sil (Citation2015), In Silchar city, silty alluvial soil dominates, has Vs30 between 180 to 360 m/s, and the average SPT-N value ranges between 15 to 50, falls under site class D type according to the provisions of NEHRP. Moreover, the hazard can be calculated at the surface level by calculating the amplification factor based on Vs30.
When earthquakes occur, the energy is released as waves, which cause ground motions. Seismic hazard analysis is a method to evaluate any phenomenon associated with the earthquake, such as ground motion, ground failure, and liquefaction. The main objective of this study is to estimate the ground motion due to various Faults around the city. Ground motion is generally estimated in terms of peak ground displacement (PGD), peak ground velocity (PGV), and peak ground acceleration (PGA). All these ground motion values benefit the earthquake-proof structure design for estimating horizontal forces due to the earthquake.
In order to assess seismic hazards, earthquake data were initially collected from a variety of seismological agencies. All different earthquake magnitude scales were converted into one moment magnitude for uniformity. Afterward, the data were declustered to remove duplicate and post-earthquake events from the main events. Data about sources near the city have been collected from the SEISAT. After that, earthquake and fault data were combined to define the fault zone. Then, seismicity parameters were calculated for the selected source. Various GMPE (ground motion prediction equation) for North East India have been compared with ground motion data collected from the various seismic stations in North East India to select the best ground motion attenuation equation. In the present study, Silchar headquarters is assumed as the most vulnerable location of Silchar city because population density is high at the Silchar headquarters. The UHS has been plotted for the selected location, and the Fault with the maximum ground motion has been selected as the final UHS for Silchar. Finally, the results have been presented as a controlling source, seismic hazard curve, and uniform hazard spectra for Silchar city. Flow charts of the seismic hazard study are shown in .
2. Collection of data
A seismic Hazard study requires many steps to estimate the hazard of any city. In this paper, the study has been divided into two parts. The first part is data collection, and the second is data analysis, as shown in . Data collection included both earthquake data collection and fault data collection.
2.1. Earthquake Data collection
For the study purpose, the earthquake catalogue from the longitude range 86° −100° and the longitude range from 18° to 30° are considered, covering the entire North East region of India, as shown in . The earthquake event details have been collected from various international seismological agencies, including the International Seismological Centre (ISC), United States Geological Survey (USGS) and Incorporated Research Institutions for Seismology (IRIS), European-Mediterranean Seismological Centre (EMSC), National Oceanic and Atmospheric Administration (NOAA). The magnitude conversion from Mb, Ms, Ml to MW moment magnitude (Mw) relationship given by Sitharam and Sil (Citation2014) has been considered. The equation given by Sitharam and Sil (Citation2014) has been developed based on past North East Indian data. So, in the present study, it is best to use this equation for the Mw conversion for the same region.
The equations given by Sitharam and Sil (Citation2014) for magnitude conversion are-
(1)
(1)
(2)
(2)
(3)
(3)
where Mw, Mb, Ml, and Ms are Moment Magnitude, Body wave magnitude, Local Magnitude, and Surface Wave Magnitude, respectively.
After the conversion of magnitude, data are declustered with the help of MATLAB by ZMAP software using the technique described by Gardner and Knopoff (Citation1974), assuming that the desclustered catalogue holds the Poisson assumptions. The goal of declustering is to remove from seismic catalogue dependent earthquakes – foreshocks, aftershocks, and earthquake clusters, so the remaining earthquakes occur independently in time and are distributed according to a Poisson process. In this study, a complete 4940 earthquake catalogue of magnitude greater than 4 is collected from various agencies, and after declustering the earthquake data, 3473 events remain. The cumulative number of earthquakes before and after the declustering is shown in .
In order to define the seismicity near Silchar City, a total of 3470 earthquake data are considered for the seismic hazard study. Data completeness is shown in for different magnitudes sizes of the earthquake. Since no appropriate instrument was available to record lower-sized earthquakes before 1964, there have been very few recorded earthquakes between magnitudes 4 and 4.9. Thus, data completeness has been determined for magnitudes 4 to 4.9 from 1964 to 2020 (57 years), 5 to 4.9 from 1922 to 2020 (99 years), 6 to 6.9 from 1920 to 2020 (101 years), and above 7 from 1762 to 2020 (259 years) as shown in .
Figure 4. Data completeness of earthquake catalogue of magnitude size (a) MW 4–4.9 (b) MW 5–5.9 (c) MW 6–6.9 (d) MW above 7.

Table 1. Data completeness of different magnitude size earthquake.
2.2. Fault data collection
The most important thing about the present seismic hazard analysis study is identifying seismic sources. The fault line data are collected from the seismotectonic atlas (SEISAT2000). The collection of seismic source data involves the collection of fault parameters, such as the Fault’s length, depth, and location and the maximum earthquake magnitude that has occurred near that Fault. In this study, a fault line is considered, which has been taken from the various SEISAT maps. For the present study, point sources near the fault line are assumed to have occurred due to the fault line. All point sources are included in the fault zone. Here, fault zone is defined for each line source based on earthquake density near the Fault. if an earthquake occurs in this zone, that Fault will be considered as a source of the event. Moreover, the minimum source-to-site distance is calculated by considering the line source. In PSHA method, Line sources are divided into n parts, based on which source to site distances are calculated.
In North East India, a total of 28 faults are present, some of which are active and some are inactive; hence in the present study, those faults have been chosen which are active and produce reasonable ground motion at the selected site. This study considers 17 faults that can generate ground motion greater than 0.1 g at Silchar city are selected are shown in .
Table 2. Details of a various fault sources near the Silchar city.
There are different fault lines presented by red colour, and black lines represent defined fault zones near the Fault, and the earthquake event of magnitude sizes of 4–4.9, 5–5.9, 6–6.9, and more than 7 are presented by different colours and sizes as shown in . A detailed description of the fault zone, such as the area considered near the Fault and the distribution of the different sizes of an earthquake in the fault zone, are presented in . This study considers some faults like A.1, A.3 and F.3, but they do not have much effect on the Silchar due to their low seismicity. For the Dauki fault, 13423 km2 area has been considered, this area consists 17 earthquake events of 4–4.9 magnitude, 17 earthquake events of 5–5.9 magnitude, and 2 events of more than 7 magnitudes shown in . The maximum area and number of events are considered for the MCT, and the minimum area and number of events are considered for A1 Fault.
Figure 5. Earthquake data (USGS, IRIS, ISC, NOAA, EMSC), various fault (SEISAT)and fault zone near the city.

Table 3. Earthquake distribution of different magnitude size in each fault zone.
3. Data analysis
Data analysis is the second part of the study, as shown in . In this portion, the collected data have been used to estimate the seismicity parameter and perform DSHA and PSHA. The study consists of estimating the seismicity parameters, selection of ground motion prediction equation, determining the controlling source, and plotting the seismic hazard curve and uniform Hazard spectra.
3.1. Seismicity parameter
3.1.1. a and b
The seismicity of any source region is generally described by two parameters, a & b, and Mmax. The parameters a & b can be estimated from linear regression analysis, which shows the relationship between the moment magnitude and cumulative frequency of occurrence. Gutenberg and Richter (Citation1945) established a relationship between moment magnitude (M) and cumulative frequency of occurrence (λ) of a specific magnitude.
(4)
(4)
(5)
(5)
where α = 2.303*a, β = 2.303*b, M is the magnitude, λ shows the mean annual rate of exceedance for earthquake, magnitude of which are larger than M, and a and a & b are constants in which a is the intercept and b is the slope of the line.
For defining the seismicity of any region, a & b play an important role. The value of a provides an overall earthquake rate, and b provides the ratio of smaller and larger earthquakes. If the b value is higher, it shows that there are more small-size earthquakes than larger-size earthquakes, and if the b value is lower, it indicates many higher-magnitude earthquakes. Three methods are available for calculating seismicity parameters a and b: least squares, maximum likelihood, and modified maximum likelihood. A minor variation in the b value significantly impacts the results. Amongst them, the least squares method has been considered in this study.
For estimation of the seismicity parameter, the number of earthquakes for each zone is classified into the range 4–4.9, 5–5.9, 6–6.9, and above 7 and below 4 magnitude earthquakes are not considered in this study. The Fault, which has few numbers of earthquakes, a and b value can be estimated by reducing the considered range of earthquake magnitude. It will give the a and b values for the Fault, which has few numbers of earthquakes. R2 shows the determination coefficient, which shows how much data fits with the equation and the uncertainty associated with the seismicity parameter. The estimation of seismicity parameters (a and b) by regression analysis for some faults are shown in . The values a, b, and R2 for each Fault have been presented in . The value of b varies between 0.4 and 1.06 for the selected source. It has been observed that Faults such as MCT, EBT&Kabaw, Dapsi, Mishmi Thrust, and MCB have the highest b value (greater than 1). There is a high probability that they will experience earthquakes of a lower magnitude than earthquakes of a larger magnitude. Compared to another fault, fault locations A1 and Sylhet have very low values of b, meaning earthquakes occur very rarely at those locations.
Table 4. Seismicity parameter and Mmax for each fault are presented.
3.1.2. Mmax
There are specific engineering applications to Maximum Magnitude (Mmax). Since higher magnitude values cause high ground motion at fault regions. Thus estimating the maximum magnitude at any fault is necessary.
Wells and Coppersmith (Citation1994) mentioned the relation between the magnitude of an earthquake and the source characteristics. The empirical relationships were developed between moment magnitude and surface rupture length, subsurface rupture length, downward rupture width, rupture area, and maximum and average displacement per event for a different source type, i.e. normal slip, reverse slip, and strike-slip. It was found that the relationship between magnitude and surface rupture area was well correlated compared to the relationship between magnitude, displacement, and rupture length.
Kijko(Citation2004) developed the equation to evaluate the maximum earthquake magnitude for different regions. Different cases were considered viz. (1) when earthquake magnitude was distributed according to the doubly-truncated Gutenberg-Richter relationship, (2) when the empirical magnitude distribution deviated moderately from the Gutenberg –Ritcher relationship, (3) when no specific type of the magnitude distribution was assumed.
Several popular methods are available for calculating the max magnitude such as Gupta (Citation2002), Mueller et al. (Citation2015), Gutenberg and Richter (Citation1945), Kijko and Sellevoll (Citation1989), Raghu Kanth and Iyengar (Citation2007), and Kijko (Citation2004). For this study, Gupta (Citation2002) and Kijko (Citation2004) methods are used. Maximum magnitude for various faults is presented in .
4. Selection of ground motion prediction equation (GMPE)
In the study of seismic hazard analysis, GMPE plays an important role. Ground motion is the function of magnitude (Mw) of the earthquakes and source-to-site distance (Epicentral distance and Hypocentral distance). These equations are generated by regression analysis on a set of strong ground motion data.
Many researchers have given the ground motion attenuation equation of different regions of India. Bajaj and Anbazhagan (Citation2019) developed a new ground motion prediction equation for the Himalayan region of magnitude range 4–9 and distance range 10–750 km. For that purpose, a data set of 4940 events has been considered. In this study, the whole Himalayan region has been divided into four parts, i.e. Kashmir Himalayan, Kumaon–Garhwal Himalayan, Bihar-Nepal Himalayan, and North-eastern Himalayan region. One GMPE equation has been developed for the Himalayan region by adding uncertainty in the seismological model parameter for different parts of the Himalayas.
Gupta (Citation2010) developed the ground motion attenuation equation for the Indo-Burmese subduction zone. Atkinson and Boore’s equation was used, and the coefficients were found based on regression analysis with observed data. The equation was valid for a magnitude range of 6.3–7.2, a distance range of 150–375 km, and a period range of 0.02–4 sec for different size classes.
Nath et al. (Citation2012) generated a ground motion prediction equation by considering the 13 earthquake magnitudes ranging from Mw 4.8 to Mw 8.1 and rupture distance varying up to 100 km. Youngs et al. (Citation1997) equation has been considered, and the coefficient has been estimated based on recorded ground motion data from a different station. The equation is valid for PGA and PGV, up to 4 secs, and can be used for North-East India. Other equation such as Aman et al. (Citation1995), Singh et al. (Citation1996), Das et al. (Citation2002) Kanno et al. (Citation2006), Lin and Lee (Citation2008), Sharma et al. (Citation2011), NDMA (Citation2011), Anbazhagan et al. (Citation2013) Raghukanth and Kavitha (Citation2014), Shayanfar and Jahani (Citation2015), Ahmed and Singh (Citation2016), and Bahuguna and Sil (Citation2018) are also available to estimate the ground motion value. A detailed description of the equation available for northeast India is presented in . For the study of hazard analysis, it is essential to select the appropriate ground motion equation to estimate the correct variation of ground motion.
Table 5. Past available ground motion attenuation equations are presented.
For selecting a suitable ground motion equation, past earthquake seismic data were collected from various seismological stations in North East India, and these data were compared with the available GMPE of North East Indian region. The magnitudes 4.2, 5.2, 6, and 6.9 magnitudes of the earthquake and 70 seismic records are considered for validating GMPE. The comparison of seismic data for the magnitude of Mw 6.9 (06/08/1988, Mw 6.9, depth 90 km, latitude 25.15, longitude 95.13) and Mw 5.2 (10/09/1986, Ms 5.2, depth 43 km, latitude 25.38, longitude 98.07) with various GMPE are shown in and . As shown in the figure, the dotted point represents the observed ground motion data collected from the various seismic stations in the northeast of India, while the various lines represent the various GMPE available for North East India. After Comparing the recorded data with previously available GMPE for Northeast India, it has been found that NDMA (Citation2011) and Bajaj and Anbazhagan (Citation2019) GMPE are the best fittings for higher and lower magnitudes of different periods at some extent. Therefore, these two GMPE’s are used for seismic hazards analysis of Silchar City, and a weighted factor of 0.5 has been considered for both equations.
NDMA (Citation2011) equation has been proposed based on 1600 simulated ground motion earthquake data collected from various regions. The equation validates the magnitude range of 4–8.5 for a distance of less than 500 km.
(6)
(6)
where
Sa is spectral acceleration in g; M is moment magnitude; r is hypocentral distance; C1, C2, C3, C4, C5, C6, C7, C8 and ε are constants.
Bajaj and Anbazhagan’s (Citation2019) equation has been proposed based on 10000 seismic data collection from North East India, and the same is valid for a magnitude range of 4.0–9.0 and distances less than 750 km.
(7)
(7)
where y is spectral acceleration in g, M is moment magnitude, R is hypocentral distance, and a1, a2, a3, a4, a5, a6, a7, and σ are constants.
EquationEquations 6(6)
(6) and Equation7
(7)
(7) can be used to estimate spectral acceleration and PGA. Spectral acceleration and PGA can be estimated by considering coefficient values for different periods. If t = 0 sec, these equations will give the PGA value, otherwise, it will give spectral acceleration.
5. Results and discussion
Seismic Hazard Analysis is a quantitative estimation of ground motion hazard at a site. Two methodologies are widely used for Seismic Hazard Analysis; one is Deterministic Hazard analysis (DSHA). Another is probabilistic Hazard analysis (PSHA), as shown in .
5.1. DSHA method
In the DSHA method, whole seismic hazard study is based on a particular earthquake scenario Kramer (Citation1996). The steps for estimation of ground motion value for deterministic seismic hazard method are shown in .
Steps
Identify and classify all possible seismic sources capable of producing higher ground motion value at the site.
Estimating a minimum source-to-site distance for each seismic source zone and Maximum magnitude Max.
Controlling sources are identified based on the sources that produce the strongest level of ground shaking by using the ground motion attenuation equation.
Hazard at the site is usually represented in terms of ground motion (PGA) due to the controlling source or earthquake.
The seismic hazard of the site commonly characterizes peak acceleration, peak velocity, and response spectrum ordinates. This method is a simple and quick method for Hazard analysis and has been used for many years in designing large dams, power plants, and other critical facilities. This method provides a scenario for evaluating the worst-case ground motions, and this method does not provide any information regarding the occurrence of that controlling earthquake. It does not consider the uncertainty in size, location, and time.
PGA values at Silchar city due to the different faults in the DSHA method are shown in . The controlling source will be responsible for generating maximum ground motion at Silchar city. In this study, it has been found that the Dauki fault can generate the PGA up to 0.65 g at the site. So, the Dauki fault will be the controlling source for Silchar City. Dauki fault is in the Shillong–Mikkir plateau region, whose length is 320 km, and the maximum magnitude observed in the past is 7.7.
5.2. PSHA method
PSHA method considers all possible earthquake scenarios that can occur in the seismic source zone, and this method has also allowed using uncertainty in size, location, and rate of earthquake recurrence. PSHA method provides a structure in which all the uncertainty can be identified, computed, and combined to give a complete seismic hazard scenario Kramer (Citation1996). The steps for generating the seismic hazard curve in the probabilistic seismic hazard method are shown in .
Steps
Identification and categorization of seismic sources near the site.
Determination of the seismicity parameter (a and b) for each source.
Estimating ground motion (PGA) value produced at the site due to any possible size of an earthquake by using the ground motion attenuation equation.
Estimating the uncertainties in the form of probability function for earthquake size, location, time, and ground motion parameter prediction. Then, the probability that the ground motion parameter will be exceeded during a time period has been determined. Using the PSHA method, the seismic hazard curve and uniform hazard spectra have been obtained. This method is more popular than the DSHA method because the results of PSHA are just more flexible than those of DSHA.
5.2.1. Seismic hazard curve
For estimating the various uncertainty, these equations are used to plot the Seismic hazard curve
Probability distribution of source-to-site distance from fault geometry and site location:
Probability distribution of Earthquake Magnitude from G-R relationship:
Where m0 is the lower threshold magnitude.
Probability of exceeding the specified value of the ground motion parameter:
For the time uncertainty, Poisson distribution model is used, and the probability of at least one exceedance in a period of t years:
λ is mean annual rate of exceedance
Finally, after the combination of all uncertainty:
(12)
(12)
NS, NM, and NR are nos. of segments divided respectively for source, magnitude, and distance range.
λy* is the mean annual rate of exceedance of particular value y*.
The probability distribution of epicentral distance and the Earthquake magnitude for the Oldham Fault are shown in the . It can be observed from the figure that as source to site distance and magnitude increases, the probability density function (fRr & fMm) decreases.
Figure 12. Probability distribution in source to site distance(a) and magnitude (b) for Oldham Fault.

The seismic hazard Curve is the plot between the mean annual exceedance rate and peak ground acceleration or spectral acceleration. shows the seismic hazard curve for each Fault for Silchar city, representing the variation between the mean annual exceedance rate and ground motion (PGA). With increasing PGA value, the rate of exceedance decreases, which means that higher ground motion values have a low chance of occurring. The above curve shows a high probability of occurrence compared to the one below. The value of λ and PGA can be estimated from the hazard curve. For example, for a 2% probability of exceedance in 50 years, the λ value can be estimated from EquationEquation (4)(4)
(4) , λ= -ln (1–0.02)/50 is equal to 0.000404 and the return period will be 1/λ, which is 2475 years. From this value of λ, the PGA value can be estimated from the hazard curve.
Comparison of PGA value estimated for the Silchar city due to each Fault at 2%, 5%, and 10% probabilities of exceedance in 50 and 100 years are shown in . For Silchar city, 2%, 5%, and 10% probability of exceedance in 50 years PGA values are 0.35 g, 0.28 g, and 0.25 g, respectively. PGA values for 2%, 5%, and 10% probability of exceedance in 100 years are 0.45 g, 0.3 g, and 0.28 g, respectively. In this study MATLAB software has been used to estimate the seismic hazard analysis.
Figure 14. PGA value estimated for the Silchar city due to each Fault at 2%, 5%, and 10% probabilities of exceedance in 50 and 100 years.

For a 2% probability of exceedance in 50 years, PGA values for Dauki and Sylhet are 0.34 g and 0.34 g, respectively and for a 10% probability of exceedance for 50 years, are 0.28 g and 0.27 g, respectively, which is nearly equal. But in the DSHA method, PGA values for Dauki and Sylhet are 0.65 g and 0.48 g. here In the PSHA method, the PGA value obtained from Dauki and Sylhet are identical, so it is difficult to identify the controlling source from PSHA Method, but in the DSHA method PGA value obtained from the Dauki fault is high as compared Sylhet fault. Hence by considering the DSHA method, the Dauki Fault has been considered the Controlling Source. Finally, PGA value estimated from DSHA method and PSHA method are present in . The PGA value estimated from the DSHA method is 0.65 and from the PSHA method is 0.25–0.45 for various return period. It is cleared from the result that PGA value estimated from the DSHA is higher than the PSHA method.
Table 6. Comparison of PGA results in DSHA and PSHA method.
5.2.2. Uniform hazard spectra
From the PSHA, uniform hazard spectra are obtained, which estimates the likelihood that all earthquakes at a site will exceed the spectral acceleration (Sa) as visualized by the previous hazard scenario. The UHS is just one way to use PSHA's output. Uniform Hazard spectra is a plot between the spectral acceleration versus the oscillator period. UHS is the response spectrum, spectral ordinates of which for considered oscillator periods are selected for the same annual frequency of exceedance or probability of exceedance. Thus, every ordinate of the curve has an equal rate of being exceeded. The plotting of uniform Hazard Spectra from the Seismic Hazard curve has been shown in .
Steps-
Calculation of hazard curves on a site in terms of spectral acceleration (SA) for different oscillator periods (for example, from 0.1 sec to 5 sec).
Selection of annual frequency of exceedance, AFE, (return period, RP) corresponding to accepted probability of exceedance, POE, during particular time period (e.g. 50 yrs)
Determination of values of spectral acceleration SA for selected AFE, or RP, for all considered oscillator periods.
The plot of SA determined in step 3 versus oscillator period is the Uniform Hazard Spectrum
For example, for a 2% probability of exceedance in 50 years, λ value can be calculated from the Poisson distribution EquationEquation (11)(11)
(11)
(13)
(13)
In the following example, P equals 2%, and T equals 50 years, so that λ will be equal to 0.000404 (Return period 2475 years). For this value of λ, Sa can be estimated from the hazard curve of different periods, where y-axis and x-axis are represented by spectral acceleration(g) and oscillator period respectively of uniform hazard spectra, thus, the plotted curve will be the UHS for a 2% probability of exceedance in 50 years (2475 years of return period). Uniform hazard spectra for different recurrence interval of specific ground motion due to possible earthquake for Silchar has been shown in . In the figure, UHS curve initially increases when period is low, but it decreases as period increases, so for low period like 0.2 sec, spectral acceleration is maximum.
The importance factor(I) is used to estimate the seismic design forces depending upon the functional use of the structure, characterized by hazardous consequences of its failure, post-earthquake functional need, historical value, or economic importance. Three I values are considered in the Indian code 1.5 for important service and community building (critical governance building, schools), 1.2 for Residential and commercial buildings, and 1 for all other buildings as per IS 1893: 2016.
For a lateral design force, the base shear force generated by an elastic structure during the response to a Design Basis Earthquake (DBE) shall be reduced by the response reduction factor (R). Design codes often recommend different values of 'R' based on the structure’s ductility, strength, and redundancy. Response reduction factor (R) depends on various structures, such as 3 for RC buildings with ordinary moment resisting frames and 5 for RC buildings with special moment resisting frames. In the design basis earthquake (DBE), the peak horizontal accelerations are defined by a 10% probability of exceeding in 50 years (475 years Return period) and the maximum considered earthquake (MCE), the peak horizontal accelerations are defined by a 2% probability of exceeding in 50 years (2475 years Return period) as per the Indian standard.
shows a comparison of uniform hazard spectra for various return periods (RP) with the response spectra of DBE (Design Based Earthquake) as per IS 1893: 2016. compares uniform hazard spectra for 475, 950, 2475, and 4950 years return periods with the response spectrum of MCE (Maximum Considered Earthquake) as per IS 1893: 2016. It has been observed from the figures that for Silchar city, uniform hazard spectra for various earthquake recurrence intervals have exceeded the spectra as mentioned in the IS 1893: 2016. for DBE levels with all probable combinations of I and R values. It has also been observed that for higher level R factor, the difference between site-specific uniform hazard spectra and IS 1893: 2016, codal provisions is maximum, while for lower R factor, the difference is minimum. Similarly, the difference is maximum for the lower level I factor of 1.0, while the difference is minimum for the higher level, I factor of 1.5. Also, the difference has been observed for both the stiff structure as well as for flexible structure. So, considering the significant range of differences between the site-specific uniform hazard spectra and IS 1893: 2016 spectra for all the combinations of I and R for specific zone factor (Z) of Silchar city, there is a need to study the site-specific hazard spectra in detail before construction of any structures over there. Site-specific uniform hazard spectra are also important as these will provide information about the location of the Fault, soil condition, and type of structure to be constructed with utmost safety .
Figure 17. Comparison of the Uniform Hazard Spectrum of Silchar with the response spectrum of DBE according to IS 1893:2016.

Figure 18. Comparison of the uniform hazard spectrum of Silchar with the response spectrum of MCE according to IS 1893:2016.

In the DSHA method, the worst-case scenario has been considered with the assumption that the maximum magnitude can occur in that source with a minimum distance from the source to the site. However, in the PSHA, all possible scenarios were considered for hazard analysis. Generally, the DSHA method provides the maximum ground acceleration value that is unlikely to occur during the structure’s life. The large uncertainties are generally associated with the considered database and at every analysis step. Therefore, selecting the most pessimistic scenario is neither likely to represent a real scenario nor a reasonable engineering decision. Further, everyone might be ready to face at least the same risk in case of earthquakes, to which he is prone in the regular walk of life. However, the DSHA method does not provide a means to quantify the amount of risk (Gupta et al. Citation2022). The PSHA approach assigns a finite probability of occurrence in the maximum possible during a specified time interval. Also, the probabilistic approach can incorporate the effects of all the controlling factors in a balanced way and can thus be considered more reliable.
A comparison of PGA value with the past study in DSHA and PSHA method are presented in . There is some variance between the PGA values of the present and the past studies. Bahuguna and Sil (Citation2018) have provided higher PGA values, and the present results are pretty similar to those of NDMA (Citation2011), Das et al. (Citation2016), and IS 1893:2016, Citation2016 . The PGA variations in different studies depend mainly upon the seismicity parameter and the equations considered for the study. The current study is based on updated earthquake data and the best-matched equations of ground motions which have resulted in more accurate results about the seismicity of the nearby fault region of Silchar city.
Table 7. Comparison of results with past study.
6. Conclusions
A seismic hazard study of the Silchar district headquarter has been conducted in this paper. For the analysis purpose, the earthquake data have been collected from different agencies during the period from 1762 to 2020, and the fault data has been obtained from the seismotectonic atlas (SEISAT). Afterwards, earthquake and fault data are superimposed on Northeast India map, and fault zones are defined based upon their density. Seismic parameters and maximum magnitude have been estimated by using Gutenberg-Richter (G-R) relationship. The best-fitted ground motion equations are selected for the study based upon comparison with available ground motion equations. The final results are represented as controlling source, seismic hazard curve, and uniform hazard spectra for 475 years, 950 years, 2475 years, and 4950 years of recurrence interval of specific ground motions due to possible earthquake at Silchar headquarter location. Following conclusions can be drawn based upon the present study.
From the seismic design consideration, the present uniform hazard spectra are more accurate and this represents a real picture of the vulnerable locations of the Silchar city. The current result is advanced and more region-specific than past studies and can be used further for estimating micro-zonation parameters of Silchar city.
The study has resulted that out of 28 faults, 17 faults are responsible for the ground motion with more than PGA of 0.1 g at Silchar city. The top 5 faults viz. Dauki, Naga & Dishang, Sylhet, Kaladan, and Lohiti are found to be responsible for higher ground motion at the Silchar location.
Seismicity parameter b value ranges from 0.36 to 1.06, and the maximum observed magnitude Mw earthquake ranges from 6 to 8.6 due to various sources near the city.
NDMA (Citation2011) and Bajaj and Anbazhagan (Citation2019) GMPE are the best-fitted equation for the study region, which are found after comparison of various available GMPEs with the observed Seismic data.
As per the estimated values obtained from various sources, PGA values lies between 0.1 and 0.65 in the DSHA method. Furthermore, the Dauki fault is the controlling source which is responsible for highest PGA (0.65g) value at the Silchar.
PGA values estimated in the PSHA method from the various source are found to be within 0.05–0.35 and 0.04–0.25 for 2% and 10% probability of exceedance, respectively, in 50 years while for 100 years, the values are within 0.05–0.45 and 0.05–0.28 for 2% and 10% probability of exceedance, respectively. The PSHA method has been given more preference over the DSHA method as the former is more rational in terms of scientific basis focusing on real scenarios considering uncertainty and risk.
It has been observed that for many combinations of Response reduction factor (R) and importance factor (I) for a specific zone factor for Silchar city, the site-specific hazard spectra has been exceeded the response spectra as per IS 1893:2016 code. The difference is maximum for the higher R factor and lowers I factor. These are crucial factors that can control the seismic design and construction of various types of regional structure.
The results of the present seismic hazard study will be helpful in seismic zonation studies and earthquake-resistant design of various types of structures like bridges, buildings, dams, and buried lifeline structures like a tunnel, drainage, and sewage lines. Further, the study will be beneficial for city planning from seismic vulnerability and design consideration.
Disclosure statement
The authors declare that there are no conflicts of interest
Data availability statement
The data used to support the findings of this study are included within the article and are available from the corresponding author upon request.
References
- Ahmed R, Singh RP. 2016. Attenuation relation predicted observed ground motion of Gorkha Nepal earthquake of April 25, 2015. Nat Haz. 80:311–328.
- Aman A, Singh UK, Singh RP. 1995. A new empirical relation for strong seismic ground motion for the Himalayan region. Current Sci. 69(9):772–776.
- Anbazhagan P, Kumar A, Sitharam TG. 2013. Ground Motion prediction equation considering combined dataset of recorded and simulated sound motions. Soil Dyn Earthq Eng. 53:92–108.
- Bahuguna A, Sil A. 2018. Comprehensive seismicity, seismic sources and seismic hazard assessment of Assam, North East India. J Earthq Eng. 24(2):254–297.
- Bajaj K, Anbazhagan P. 2019. Regional stochastic GMPE with available recorded data for active region – application to the Himalayan region. Soil Dyn Earthq Eng. 126:1–13.
- Baro O, Kumar A, Zadeh AI. 2020. Seismic hazard assessment of the Shillong Plateau using a probabilistic approach. Geomat Nat Haz Risk. 11(1):2210–2238.
- Das R, Sharma ML, Wason HR. 2016. Probabilistic seismic hazard assessment for Northeast India region. Pure App Geophysics. 173:2653–2670.
- Das S, Gupta ID, Gupta VK. 2002. A new attenuation model for North-East India. 12th Symposium on Earthquake Engineering, IIT Roorkee, India.
- Devi A, Sarbeswar K. 2014. Seismic hazard analysis of Northeast India and its adjoining region. Int J Environ Sci. 4(5):859–874.
- Gardner JK, Knopoff L. 1974. Is the sequence of earthquakes in Southern California, with aftershocks removed, Poissonian? Bull Seis Soc America. 64(5):1363–1367.
- Gupta ID. 2002. The state of art in sesimic hazard analysis. Indian Soc Earthq Tech. 39(4):311–346.
- Gupta ID. 2010. Response spectral attenuation relations for in-slab earthquakes in Indo- Burmese subduction zone. Soil Dyn Earthq Eng. 30(5):368–377.
- Gupta PK, Ghosh G, Kumar V, Paramasivam P, Dhanasekaran S. 2022. Effectiveness of LRB in curved bridge isolation: a numerical study. Appl Sci. 12(21):11289.
- Gutenberg B, Richter CF. 1945. Frequency of earthquakes in California. Nature. 156(3960):371–371.
- Haloi J, Sil A. 2015. Seismic Site Classification of a Bridge Site over River Barak on Silchar Bypass Road. Int J Adv Earth Sci Eng. 4(1), :275–282.
- IS 1893:2016. 2016. Part 1: general provisions and buildings, criteria for earthquake resistant design of structures. Bureau of Indian Standards. New Delhi, India.
- Joshi A, Mohan K, Patel R. 2007. A deterministic approach for preparation of seismic hazard maps in North East India. Nat Haz. 43(1):129–146.
- Kanno T, Narita A, Morikawa N, Fujiwara H, Fukushima Y. 2006. A New Attenuation Relation for Strong Ground Motion in Japan Based on Recorded Data. Bull Seis Soc America. 96(3):879–897.
- Kijko A, Sellevoll MA. 1989. Estimation of Earthquake Hazard Parameters from Incomplete Data Files. Part I. Utilization of Extreme and Complete Catalogs with Different Threshold Magnitudes. Bull Seis Soc America. 79(3):645–654.
- Kijko A. 2004. Estimation of the maximum earthquake magnitude, Mmax. Pure Appl Geophysics. 161:1655–1681.
- Kramer SL. 1996. Geotechnical earthquake engineering. New Jersey: Prentice-Hall international series in civil Engineering and Engineering mechanics.
- Lin PS, Lee CT. 2008. Ground-motion attenuation relationships for subduction-zone earthquakes in Northeastern Taiwan. Bull Seis Soc America. 98(1):220–240.
- Menon A, Ornthammarath T, Corigliano M, Lai CG. 2010. Probabilistic Seismic Hazard Macrozonation of Tamil Nadu in Southern India. Bull Seis Soc India. 100(3):1320–1341.
- Mueller C, Boyd OS, Petersen MD, Moschetti MP, Rezaeian S, Shumway A. 2015. Seismic hazard in the eastern United States. Earthq Spec. 31(S1):85–107.
- Nath SK, Thingbaijam KKS, Maiti SK, Nayak A. 2012. Ground-motion predictions in Shillong Region, northeast India. J Seismo. 16:475–488.
- Nath SK, Thingbaijam K. 2012. Probabilistic seismic hazard assessment of India. Seismo Res Letters. 83(1):135–149.
- National Disaster Management Authority (NDMA). 2011. Development of probabilistic seismic hazard map of India. New Delhi: Government of India.
- Raghu Kanth ST, Iyengar RN. 2007. Estimation of seismic spectral acceleration in peninsular India. J Ear Sys Sci. 116(3):199–214.
- Raghu Kanth STG, Iyengar RN. 2006. Seismic hazard estimation for Mumbai City. Curr. Sci. 91(11):1486–1494.
- Raghu Kanth STG, Singh KD, Pallav K. 2009. Deterministic seismic scenarios for Imphal City. Pure Appl Geophysics. 166(4):641–672.
- Raghukanth STG, Kavitha B. 2014. Ground motion relations for active regions in India. Pure Appl. Geophy. 171(9):2241–2275.
- Sharma M, Malik S. 2006. “Probabilistic Seismic Hazard Analysis and Estimation of Spectral Strong Ground Motion”. 4th International Conference on Earthquake Engineering, Taipei, Taiwan.
- Sharma ML, Douglas J, Bungum H, Kotadia J. 2011. Ground-motion prediction equations based on data from the Himalayan and Zagros regions. J Earthq Eng. 13(8):1191–1210.
- Shayanfar M, Jahani E. 2015. New fuzzy method in choosing Ground Motion Prediction Equation (GMPE) in probabilistic seismic hazard analysis. Earthq Struc. 10(2):389–408.
- Sil A, Sitharam TG, Kolathaya S. 2013. Probabilistic seismic hazard analysis of Tripura and Mizoram States. Nat Haz. 68:1089–1108.
- Singh RP, Aman A, Prasad YJ. 1996. Attenuation, relations for strong ground motion in the Himalayan region. Pure Appl Geophys. 147(1):161–180.
- Sitharam TG, Sil A. 2014. Comprehensive seismic hazard assessment of Tripura and Mizoram states. J Earth Sys Sci. 123:837–857.
- Verma M, Bansal BK. 2013. Seismic hazard assessment and mitigation in India: an overview. Int. J. Earth Sci. 102(5):1203–1218.
- Wells D, Coppersmith K. 1994. New empirical relationships among magnitude, rupture length, rupture width, rupture area, and surface displacement. Bull Seis Soc America. 84(4):974–1002.
- Youngs RR, Chiou SJ, Silva WJ, Humphrey JR. 1997. Strong ground motion attenuation relationships for subduction zone earthquakes. Seismol Res Lett. 68(1):58–73.