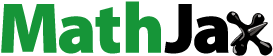
Abstract
The increasing pollution of groundwater resulting from population growth and the evolution of agro-industrial activities have become a poses threat to the socio-economic development of the western Algerian region. This study aims to assess the vulnerability and pollution risks of the plain of M’léta and factors that control the transfer of pollutants, and prepare a report on the basis of a thematic map relating to the DRASTIC and DRIST method which is an improvement of the DRASTIC method. Cartographic analysis of the results of the two methods showed the M'léta Plain is characterized by low to high vulnerability, of which almost the entire plain is characterized by low to medium vulnerability. There was 53.75% for the DRASTIC method, while the second rate was 53.06% for the DRIST method, while the high vulnerability is recorded in the northern part of the plain, which swallowed 46.93% for the DRASTIC method, and for the DRIST method, it amounted to 46.24%. The result of the research shows that the preparation of an objective map avoids many risks of pollution and gives possible solutions for any future decision, and that both methods express approximately the same areas with regard to the degree of vulnerability.
1. Introduction
This study focuses on the Mio-Pliocene aquifer complex, which constitutes an important resource due to the extension of its impluvium. Excessive use of agricultural fertilizers, pesticides and sanitation resulting from demographic growth and modern industries has exposed this this reservoir to many pollutants (Ncibi et al. Citation2020a,Citationb; Nekkoub et al. Citation2020; Brahmi et al. Citation2021; Dib et al. Citation2022), drained without prior treatment and adequate protection measures (Khedidja and Boudoukha Citation2018). The numerical mapping approach to groundwater pollution vulnerability is an effective tool to estimate the degree of pollution vulnerability (Brahmi et al. Citation2021; Hamed et al. Citation2022) and is a standard tool for protecting this aquifer from any potential source of pollution. It is an essential document for land use planning and is valuable for any future decision (Bentekhici et al Citation2018). It mainly helps guide the selection of sites for development projects that may have negative consequences on the quality of groundwater resources (Albinet and Margat Citation1970). Therefore, the purpose of this research is to produce an intrinsic pollution vulnerability map using different methods to estimate this vulnerability, and the main importance of vulnerability maps is that their analysis can provide effective information for making informed decisions for water management (Alam et al. Citation2014; Abdullah et al. Citation2016). There are several ways to estimate vulnerability including Hierarchical Systems Methods (HCS), Mathematical model and analog relations (AR), Parameterized Systems Methods (PCSM, RS, MS) (Hind Es Saouini Citation2015), depending on the environmental context in this study we used criteria weighting and indexing methods by a classification system ‘PCSM’ point count system model is the most used all over the world, although they are subjective and present intrinsic gaps in a qualitative way, these methods are quick and easy to implement. Difficulties that arise in their development stem from the selection of standards and the distribution of ratings and weights, in contrast, we used two methods DRASTIC (Aller et al. Citation1987) and DRIST (Sinan Citation2000), which are the most suitable for the hydrogeological conditions of the M’léta plain, where DRASTIC includes parameters relating to both recharge, to the unsaturated and saturated area of the aquifer in contrast to DRIST which he considers an improvement of the DRASTIC method which is abbreviated to 5 parameters includes the same parameters except for the permeability and the lithological nature (Drias and Toubal Citation2015). Which will allow us to compare the results obtained from the two methods and the parameters involved and responsible in the transmission of pollution from the soil surface to the water table. Although the DRASTIC method usually gives satisfactory results in evaluation of groundwater intrinsic vulnerability to pollution, we have strengthened our research by using another method to substantiate the weaknesses. Finally, all necessary measures must be taken to conserve the precious resource, by establishing a protective perimeter for the borders of the area most vulnerable to pollution.
General setting
The M’léta plain is an East-West oriented depressional platform, formed by terrestrial sediments resulting from the erosion of the frontier mountains of Tessala and wind sediments. belonging to the structural context of the median furrow occupied by the Sebkha, western extension of the vast syncline of the Chief of which she is a part, where important formations dating from the Neogene and Quaternary have accumulated. These formations over comes an jurassico-cretaceous. In terms of hydrogeology, the miocene-pliocene aquifer is of great importance due to its extension, its storage capacity, and its upper cover that protects the aquifer from surface pollution.
2. Materials and methods
2.1. Geography and climatology
The study area is located in the southern part of the endorheic watershed of the great Sebkha of Oran. It is limited to the North by the ridge of Mount Murdjadjo, to the South by the foothills of Tessala, to the East by the plateau of Oran – Es-Senia – Ain El-Beida, and to the West by the town of Hammam Bouhadjar (). Locally, the region is subject to a Mediterranean regime characterized by a specific microclimate and controlled by the phenomenon of the Foehn effect. Despite its proximity to the coast, its semi-arid climate, hot in summer with relatively cold winters, is characterized by an rainfall not exceeding 374 mm at Es-Sénia station, but the rains are very irregular from year to year. The period of precipitation during the year lasts from October to April, with a maximum in December (75 mm) which contributing only very little to the replenishment of the surface water of the M’léta. As for the temperature it is characterized by an average of more than 17 °C and the annual potential evaporation is estimated at about 120 mm.
The climate study was based on data related to the period 1990–2019, at the level of Es-Sénia station provided by the National Office of Meteorology (O.N.M.).
2.2. Geological and hydrogeological setting of the M’léta plain
The geological series of the site according to (Bellaredj Citation2016), presents a Mesozoic substratum formed essentially by schists and quartzites on which rests a Cenozoic cover formed by blue marls (Middle Miocene) in (Hassani Citation1987), Tripoli limestones (Messinian) and reefal limestones which form the crowning of the Tessala mountain and recent formations (colluvium, alluvium) which cover the whole plain of the great Sebkha of Oran (Ahmed Citation2013). Where are attributed to Pleistocene-Holocene formations and correspond to astack of sandy-clay or silty subhorizontal layers (Adda Citation2013).
The fissured limestones of the Upper Miocene, commonly called Messinian Tripoli limestones, are located on the Lower Cretaceous schists and quartzites and form the backbone of the Tessala mountains, which constitute the most important hydrogeological unit of the Oran area. The Hydrogeology section was made along the route of section A-B ( and ) in order to extract the great effects of groundwater flow in the area. It is a carbonate aquifer (Mining Research and Exploitation Company ‘MREC’) where all the forms of the karstic model are represented notably, the presence of dissolution channels, Lapiez, Dolines, Avens, and underground galleries. The geomorphology shows two distinct zones:
South zone is represented by the Tessala mountains (710 m), where the cover represented by limestones is discordant on the shales.
Foothills zone ensures the transit between the contributions from the Tessala mountains and the infiltration regulating the hydrodynamic balance of the aquifers of the Mio-Plio-Quaternary complex. Further north, this aquifer sinks through a system of faults in steps and becomes semi-captive under the alluvium of the Plio-Quaternary.
The Neogene filling is characterized by littoral deposits with varied facies and often reduced thickness, rapidly decreasing in thickness more and more marly downstream of their outcrops. The infiltration waters are stopped downwards either by the intercalations of the Tripoli marly levels or by the blue marls (). The water table is drained by many springs with seasonal variations and very low and irregular flow. Floods overflows sometimes flow for some springs, others have completely dried up (Case of the source of Tangroutah followed for 3 years, according to the Mining Research and Exploitation Company (MREC Citation1984).
The inventory work mainly focused on topographic maps at 1/50,000th scale N°181 of Arbal and N°182 of Sg. According to the piezometric map, established from the measurements taken during the inventory of the water points that we carried out during the month of February 2021 (). The measures well distributed over our region. It turns out that the piezometric curves have low concavity and are almost parallel and the flow is generally from south to north, towards the great Sebkha of Oran where all the waters converge (endoreic basin).
The M'léta aquifer is mainly fed from meteoric waters which infiltrate the entire impluvium and runoff waters from the wadis of the Tessalas which infiltrate at the level of their dejection cones in periods of high waters (Bouazza Citation2014).
2.3. Vulnerability assessment methodology
DRASTIC method is one of the approaches used in groundwater for vulnerability estimation due to its capability and ease of use. The Environmental Protection Agency (EPA Citation2003) has developed this method to classify the pollution potential of aquifers (Aller et al. Citation1987). Vulnerability to contamination is a dimensionless index function of hydrogeological factors
In this vulnerability assessment method we have based on the use of statistical techniques for the assignment and distribution of factorial ratings and weightings of all DRASTIC parameters in a GIS environment (Amrani et al. Citation2019).
The elaboration of the maps is carried out in the main structure of a Geographic Information System ‘Arc GIS’, which represents a powerful tool of analysis for environmental studies and spatial modeling of natural phenomena (Nadjla et al. Citation2018), with coordinate system WGS 1984 UTM Zone 30 N.
During this study, vulnerability maps can be developed using criteria weighting and indexing methods. On the other hand, we used two methods (DRASTIC and DRIST) that specify the same parameters, which will allow us to compare the results obtained from the two methods as well as the parameters responsible for the transfer of pollutants from the surface land to aquifer.
2.3.1. DRASTIC method
DRASTIC is a numerical rating method with a classification system ‘PCSM’ point count system mode. This method was developed by the US Environmental Protection Agency (EPA Citation2003; Aller et al. Citation1987), In order to estimate the vulnerability of groundwater to be polluted (Mokili et al. Citation2017). It is identified by the acronym DRASTIC (Bezelgues et al. Citation2002). Each of the seven parameters was assigned a score ranging from 1 to 10 (), defined as value intervals. A weighting factor (p) was then applied to the different parameters to relativize their respective importance in terms of vulnerability (Janipella et al. Citation2020).
Table 1. Standards assigned ratings for DRASTIC method parameters and their weights (Lallemand and Barres Citation1994).
Once the products were defined, and each rating was assigned a weight, the DRASTIC Index (Id) for each hydrogeological unit is obtained by summing these products by EquationEq. (1)(1)
(1) . The index thus calculated represents a measure of the risk of contamination of the hydrogeological unit to which it is attached. This risk increases with the value of the index (Id) (Aller et al. Citation1987; Kehinde Citation2018).
(1)
(1)
where p is the weight of the parameter it is a weighting factor (varies from 1 to 5) and c is the associated rating (varies from 1 to 10) to thematic maps.
The mapping of vulnerability to groundwater pollution allows the study area to be divided into several units with different degrees of relative vulnerability (Aller et al. Citation1987; Engel et al. Citation1996) () A simple classification of vulnerability index values into four classes is proposed, by (Aller et al. Citation1987) the values obtained are grouped into five classes, each of which corresponds to a degree of vulnerability ranging from a value of 23 to a value of 226.
Table 2. Index classification and their degree of vulnerability by the DRASTIC method (Aller et al. Citation1987).
In this regard, we focus on the main objective, which is the development of the map of intrinsic vulnerability to pollution of the water table of the M’léta plain according to the DRASTIC method, based on the analysis of data concerning the physical characteristics of the three parts: Soil, unsaturated zone, saturated zone (Vrba and Zaporozec Citation1994; Baghvand et al. Citation2010).
2.3.2. DRIST method
This method was designed in Morocco and proposed by Sinan (Citation2000). Although it is based on 5 parameters considered in DRASTIC, it can be considered as an improvement of the latter, as it includes both parameters relating to recharging, and land (slope and rocky nature) and unsaturated zone (nature and thickness). This method considers that only these parameters are involved in the vertical transfer of pollution from the soil surface to the water table (Drias and Toubal Citation2015). Expressed that the vulnerability of aquifer can be worked out without using all the parameters.
The weights and scores assigned to the different parameters are the same as those defined in the DRASTIC method. The vertical vulnerability index Iv is obtained by summing the products of each score by its weight as follows by EquationEq. (2)(2)
(2) :
(2)
(2)
where D, R, I, S, and T are the method’s parameters mentioned above; p is the weight of the parameter (it is a weighting factor and varies from 1 to 5); and c is the associated rating (varies from 1 to 10). The weights multiplied by the scores associated with each parameter give DRIST indices between 27 (minimum value) and 166 (maximum value).
2.4. Evaluation of DRASTIC parameters
2.4.1. Depth to water (D)
Depending on the piezometric level measurement campaigns and the values recorded at the borehole sections capturing the Mio-Pliocene complex, this parameter was evaluated and mapped the depths of the water body ().The depth of the water body of the M’léta plain in its eastern part varies between 1 and 65 m, the shallow areas are located in the north west of the plain and are the most vulnerable to pollution.
2.4.2. Net recharge (R)
The net recharge was evaluated by the results of the water balance of the meteorological stations according to the National Meteorological Office (O.N.M Citation2019) and the National Water Resources Agency (N.W.R.A). It depends on the effective infiltration and its distribution in space and time, therefore on the climate (evaporation and precipitation), but also on the topography and the nature of the formations.
The results of the water balance show that the value of this parameter is estimated at 25 mm and is homogeneous over the entire basin ().
2.4.3. Aquifer media (A)
It is called the lithology of the aquifer, this parameter was resting on existing lithological sections at the level of drillings, wells, geological sections and according to our field investigations through the study area in order to identify the lithological nature of the aquifer. Analysis of these data revealed the existence of two aquifer materials that are most vulnerable to pollution, namely: Beds of sandstone, limestone and shale and Karst limestone ().
2.4.4. Soil media (S)
The soil map of the M’léta aquifer Bureau National d‘étude pour le Développement Rural (B.N.E.D.E.R. 2005) has made it possible to distinguish two zones of different vulnerability ():
Little evolved soils which are mainly present in the southern fringe, in the spread cones of the floods of the wadis coming from the Tessalas mountains.
The calcimagnesic soils which are widely spread in the alluvial plain of the M'léta.
2.4.5. Topography (T)
The map of the slopes is determined from the topographic maps (1/50,000th) and the Digital Terrain Model. It has four classes of slopes ().
The lowest slope class (0–2%) dominates almost the entire study area.
2.4.6. Impact of vadose zone (I)
This parameter is defined according to the drilling logs, this zone has an impact on the potential pollution fate of the aquifer, therefore the study of their nature and thickness determines the factors that can contaminate groundwater, so the evaluation of this parameter is very important.
It shows that there are four classes: Clays, Clays – marls, Sandy clays, with a low to medium vulnerability index between a rating of 1 to 4, sand and Gravel which takes an extremely high vulnerability index with a rating of 8 ().
2.4.7. Hydraulic conductivity (C)
The permeability map of the aquifer () is determined from the measured transmissivity values (Hassani Citation1987; A.N.R.H. Citation2012):
(3)
(3)
K: the hydraulic conductivity of the aquifer (expressed in m.s−1);
T: the transmissivity (expressed in m2.s−1); b: the thickness of the aquifer (m).
In the M’léta plain, the transmissivity varies from 0.38 × 10−3 to 1.5 × 10−3 m2.s−1.
The application of the proposed methodology has shown the effective contribution provided by geographic information systems (GIS) to develop maps of vulnerability and sensitivity to pollution of groundwater levels, particularly those related to regional reach and having a large mass of data (Twana et al. Citation2016) ().
3. Results and discussion
3.1. Pollution vulnerability map according to DRASTIC method
The analysis of the vulnerability map established using the DRASTIC method (Aller et al. Citation1987; Engel et al. Citation1996; El kayassi et al. 2020) which was based mainly on the seven criteria related to the three parts: soil, unsaturated area, saturated area at the level of the M’léta plain (). And, based on the division of Aller et al. (Citation1987), allowed us to distinguish three classes of different degrees of vulnerability.
Class I: Low Vulnerability (87 < Id < 120);
Class II: Medium Vulnerability (121 < Id < 160);
Class III: High Vulnerability (161 < Id < 181).
And through the density of pre-studied data about the geological and hydrogeological horizons about the characteristics of the sector, these classes can be grouped into two areas:
The first zone has a low vulnerability, covers most of the study area (46.24%), and takes the yellow color. This corresponds to fine-textured soils (Silty-Clay) with relatively low permeability. The pollution is only transmitted at a very low speed, the surface of the clay particles allowing significant retention.
The second area of medium and high vulnerability (53.75%) covers the northern surface of the study area (red and brown sectors), and the other part of medium vulnerability is located in the Southeast part. These areas are more closely related to the gravelly nature of the soil and the lithological nature of the unsaturated zone with high permeability. It also coincides with the water table, where the depth of the piezometric surface is very low and sometimes zero. In these areas, most boreholes are reserved for water supply and agricultural needs. These areas must be subjected to rigorous control to define adequate protection measures.
3.2. Pollution vulnerability map according to DRIST method
The map of vulnerabilities generated using the DRIST method made it possible to obtain three different categories of vulnerabilities. Their limitations are as follows:
Class I: Low Vulnerability (51< Iv < 80);
Class II: Medium Vulnerability (81< Iv < 110);
Class III: High Vulnerability (111 < Iv ≤ 139).
Interpretation of the vulnerability map () highlights two areas. The results show the first zone of very low and low vulnerability, which occupies most of the plain (46.93%) and is yellow and dark yellow. Depending on the prior data referring to the applicable parameters related to the geological horizons, this weakness is mainly due to the lithological nature of the vadose zone (clays, marly clays, sandy clays) unfavorable to the transfer of pollutants with the high depth of the water table. The second area of medium and high vulnerability (53.06%) extends mainly in the northern regions of the map (red and brown sectors), specifically in Douar and Touama. Therefore, this result highlights the low depth of the water table and the lithological nature of the unsaturated zone.
4. Conclusion
The hydrogeological context presented in this work highlights that only the Miocene limestones are of great interest due to their large extension and fracturing favorable to storage, where the groundwater table remains protected from any surface contamination, while the water table contained in the Quaternary fill formation.
Based on these results, and based on the data about the geological horizons between the aquifer and the ground surface, we created a water pollution map of the Mio – Pliocene complex using the DRASTIC and DRIST methods. Where the two maps resulting from the DRASTIC and DRIST methods reveal almost the same areas regarding the degree of vulnerability (the most vulnerable areas to pollution are located in the northern part of the plain). The DRASTIC method has proven to be more accurate and clearer in estimating the degree of vulnerability because it depends on seven parameters, which include all parts, starting with the emanation of the pollutant from the surface of the soil until its end and its arrival in the aquifer, passing through all parts, so it has an inevitable and inevitable result in estimating the degree of vulnerability. As for the DRIST method, it almost confirms what the DRASTIC method has proven regarding the areas of vulnerability and degrees of contamination, and it was limited to 5 parameters related to the unsaturated region. The DRIST method’s application shows that only the factors related to the unsaturated zone impact the transfer of the pollutant from the surface to the aquifer. Thus, we conclude from both methods that the unsaturated area has a significant impact on the transmission of pollutants.
It is necessary to put in place a policy to protect drinking water catchments and the water resources they collect. This protection is materialized by the establishment of protection perimeters to prevent or delay the pollution of water catchments.
Control the activities and facilities that could be a source of dangerous pollution.
In principle, conditions should be established for land use plans.
Take strict precautionary measures for the target areas.
Acknowledgments
The authors thank all anonymous reviewers and editors for their constructive and valuable comments and suggestions which have helped to improve this article.
Data availability statement
The data that support the findings of this study are available from the corresponding author, Khedidja Abdelhamid.
Disclosure statement
No potential conflict of interest was reported by the authors.
References
- A.N.R.H. 2012. Agence nationale des ressources hydriques. Étude hydrologique de la plaine de la M’leta.
- Abdullah TO, Ali SS, Al-Ansari NA. 2016. Groundwater assessment of Halabja Saidsadiq Basin, Kurdistan region, NE of Iraq using vulnerability mapping. Arab J Geosci. 9(3):1–16.
- Adda M. 2013. Caractérisation hydrochimique et pollution des eaux souterraines en contexte urbain: cas de l‘agglomération oranaise (ALGERIE). These Magister. Université d’Oran. p. 133.
- Ahmed B. 2013. Le système aquifère de la Grande Sebkha d’Oran: nconsidérations géologiques et hydrogéologiques. Bulletin de l’Institut Scientifique, Rabat, Section Sciences de la Terre, 35:77–92.
- Alam F, Umar R, Ahmed S, Dar FA. 2014. A new model (DRASTIC-LU) for evaluating groundwater vulnerability in parts of central Ganga Plain, India. Arab J Geosci. 7(3):927–937.
- Albinet M, Margat J. 1970. Groundwater pollution vulnerability mapping. Bulletin du Bureau de Researches Geologicques et Minieres Bull BRGM 2nd Series, 3, 13–22.
- Aller L, Bennett T, Lehr J, Petty RJ, Hackett G. 1987. DRASTIC: a standardized system for evaluating ground water pollution potential using hydrogeologic settings. Washington, DC: US Environmental Protection Agency. p. 455.
- Amrani S, Hinaje S, Gharmane Y. 2019. Application des methods paramétriques (DRASTIC et SI) pour l’étude de la vulnérabilité à la pollution potentielle par les nitrates de la nappe d’eau superficielle de timahdite-almis guigou (moyen Atlas, MAROC). J Water Sci. 32(3):237–252.
- B.N.E.D.E.R. 2005. Bureau National d‘étude pour le Développement Rural. Etude d’assainissement et de luttes contre les inondations dans la plaine de la M’léta. Première phase: état des lieux.
- Baghvand A, Nasrabadi T, Bidhendi GN, Vosoogh A, Karbassi A, Mehrdadi N. 2010. Groundwater quality degradation of an aquifer in Iran central desert. Desalination. 260(1–3):264–275.
- Bellaredj E. 2016. Les facies gypso-salins dans la région d’Oran et leurs conséquences sur les ressources. J Appl Biosci. 104(1):9976–9984.
- Bentekhici N, Benkesmia Y, Berrichi F, Bellal S. 2018. Évaluation des risques de la pollution des eaux et vulnérabilité de la nappe alluviale à l’aide des données spatiales. Cas de la plaine de Sidi Bel Abbès (Nord-Ouest Algérien). Rev Sci Eau. 31(1):43–59.
- Bezelgues S, Des Garets E, Mardhel V, Dörfliger N. 2002. Cartographie de la vulnérabilité de Grand-Terre et de Marie-Galatie (Guadeloupe).
- Bouazza S. 2014. Actualisation de l’étude hydrogéologique de la plaine de la M’léta (Algérie-Nord-Ouest). Mémoire d'ingéniorat. Université d’Oran. p. 166.
- Brahmi S, Baali F, Hadji R, Brahmi S, Hamad A, Rahal O, Besser H, Dhaouadi L, Hadji R, Hamed Y, Jemmali H. 2021. Ecologic and economic perspectives for sustainable irrigated agriculture under arid climate conditions: An analysis based on environmental indicators for southern Tunisia. Journal of African Earth Sciences, 177, 104134.
- Dib I, Khedidja A, Chattah W, Hadji R. 2022. Multivariate statistical-based approach to the physical-chemical behavior of shallow groundwater in a semiarid dry climate: the case study of the Gadaïne-Ain Yaghout plain NE Algeria. Min Miner Depos. 16(3):38–47.
- Drias T, Toubal AC. 2015. Cartographie de la vulnérabilité a la pollution de la nappe alluviale de Tebessa-Morsott (bassin versant de l’Oued Ksob) extrême est algérien. LARHYSS J. 22:35–48.
- Engel B, Navulur K, Cooper B, Hahn L. 1996. Estimating groundwater vulnerability to non point source pollution from nitrates and pesticides on a regional scale.
- EPA. 2003. Contaminated sediment transport and fate modeling. Athens, GA: United States Environmental Protection Agency. p. 30605. /http://www.epa.gov/nerl/research/2003/g5-4.htmlS.
- Es Saouini H. 2015. Vulnerabilite a la pollution des eaux souterraines: aspect dynamique et application a l’aquifere de R’mel (Larache, MAROC) [Doctorat en sciences et techniques de l’ingenieur]. Universite Abdelmalek Essaadi, TANGER.
- Hamed Y, Hadji R, Ncibi K, Hamad A, Ben Saad A, Melki A, Mustafa E. 2022. Modelling of potential groundwater artificial recharge in the transboundary Algero-Tunisian Basin (Tebessa-Gafsa): The application of stable isotopes and hydroinformatics tools. Irrigation and Drainage, 71(1), 137–156.
- Hassani MI. 1987. Hydrogéologie d‘un bassin endoréique semi-aride: le bassin versant de la grande Sebkha d‘Oran (Algérie) [Doctoral dissertation]. Université Scientifique et Médicale de Grenoble.
- Janipella R, Quamar R, Sanam R, Jangam C, Pandurang, Balwant, Jyothi V, Padmakar C, Pujari PR. 2020. Evaluation of groundwater vulnerability to pollution using GIS based DRASTIC method in Koradi, India - a case study. J Geol Soc India. 96(3):292–297.
- Kehinde AM. 2018. Application of vulnerability modeling techniques in groundwater resources management: a comparative study. Appl Water Sci. 8:1–24.
- Khedidja AK, Boudoukha A. 2018. Study of the physical and chemical qualities of surface water and groundwater in the upper valley of wadi Rhumel (Eastern Algeria). Arabian J Geosci. 11(5):1–8.
- Lallemand-Barrès A. 1994. Normalisation des critères d'établissement des cartes de vulnérabilité aux pollutions. Etude documentaire préliminaire. Rapport BRGM R, 37928.
- Mokili M, Faidance M, Patrick M. 2017. Cartographie de la vulnérabilité à la pollution de la nappe souterraine d’Isiro (Nord-est de la RD Congo) à partir de la méthode DRASTIC. Afr Sci. 13(3):125–139.
- MREC. 1984. Mining Research and Exploitation Company. Hydrogeological study in the region of the Arbal salt deposit. Sidi Bel Abbes: Sidi Bel Abbes University.
- Nadjla B, Yamina B, Faouzi B, Sid Ahmed B. 2018. Évaluation des risques de la pollution des eaux et vulnérabilité de la nappe alluviale à l’aide des données spatiales. Cas de la plaine de Sidi Bel Abbès (nord-ouest algérien) Assessing water pollution risks and groundwater vulnerability using spatial data. Case of the Sidi Bel Abbes plain (North-West Algeria). J Water Sci. 31(1):43–59.
- Ncibi K, Chaar H, Hadji R, Baccari N, Sebei A, Khelifi F, Abbes M, Hamed Y. 2020a. A GIS-based statistical model for assessing groundwater susceptibility index in shallow aquifer in Central Tunisia (Sidi Bouzid basin). Arab J Geosci. 13(2):1–21.
- Ncibi K, Hadji R, Hamdi M, Mokadem N, Abbes M, Khelifi F, Zighmi K, Hamed Y. 2020b. Application of the analytic hierarchy process to weight the criteria used to determine the Water Quality Index of groundwater in the northeastern basin of the Sidi Bouzid region, Central Tunisia. Euro-Mediterr J Environ Integr. 5(1).
- Nekkoub A, Baali F, Hadji R, Hamed Y. 2020. The EPIK multi-attribute method for intrinsic vulnerability assessment of karstic aquifer under semi-arid climatic conditions, case of Cheria Plateau, NE Algeria. Arab J Geosci. 13(15):1–15.
- O.N.M. 2019. Office National de Météorologie. Données climatologies de station d‘Es-Sénia périmètreTafraoui.
- Sinan M. 2000. Méthodologie d’identification, d’évaluation et de protection des ressources en eau des aquifères régionaux par la combinaison des SIG, de la géophysique et de la géostatistique: application à l’aquifère du Haouz de Marrakech (Maroc). Ecole Mohammadia d’Ingénieurs, Maroc.
- Twana OA, Salahalddin SA, Nadhir AA, Sven K. 2016. Groundwater Vulnerability Using DRASTIC and COP Models: case Study of Halabja Saidsadiq Basin, Iraq. Engineering. 8(11):741–760.
- Vrba J, Zaporozec A. 1994. Guidebook on mapping groundwater vulnerability. Heise.