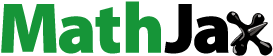
Abstract
The greater Jakarta area is located in west Java and has a high earthquake risk due to the subduction of the Australian Plate beneath the Eurasian Plate. Previous hazard studies have been carried out using various seismic probability approaches. This study presents a new probabilistic seismic hazard analysis (PSHA) by developing an updated seismic model for the crustal active fault database, using a new set of ground motion prediction equations (GMPE), and taking into consideration the effect of site amplification. The logic tree method was used to quantify the epistemic uncertainty of the source parameter components and the attenuation equation was used. We calculate the peak ground acceleration (PGA) and spectral acceleration (SA) for West Java, including metropolitan Jakarta, Bogor and Serang, which have the greatest concentrations of population and business. We found that the mean PGA reaches 1.0 g or a 2% probability of exceedance in 50 years, including site amplification factors. The seismic hazard is dominated by the shallow crustal fault near metropolitan Jakarta. The result of this study may be useful for updating the existing Indonesian hazard map.
1. Introduction
The structural control of Jakarta is dominated by two primary earthquake sources: The Java subduction, which is active due to interactions between the Australian and Eurasian Plates, and the active fault source on the mainland of west Java (Simandjuntak and Barber Citation1996). The tectonic activity has resulted in the formation of active faults in the west Java area, including the Cimandiri Fault (Marliyani et al. Citation2016), the Lembang Fault (Afnimar et al. Citation2015; Daryono et al. Citation2019), the Garut Fault (Supendi et al. Citation2018) and the Baribis Fault (Simandjuntak and Barber Citation1996; Koulali et al. Citation2017; Aribowo et al. Citation2022). West Java has a very high level of population density and is the epicenter of Indonesia’s economy; it includes the cities of Jakarta, Bogor, and Serang. Metropolitan Jakarta is the most densely populated area in Indonesia; however, seismotectonic studies of this region are still lacking. shows the tectonic setting of this study and the population density of West Java. Simandjuntak and Barber (Citation1996) show that the Baribis-Kendeng Fault can be traced from the Sunda Strait eastwards across Java and into the Bali Basin. Aribowo et al. (Citation2022) report that the active Baribis Fault continues to the south of Jakarta extending from the cities of Tangerang, Bekasi, Karawang, and Purwakarta; this was diagnosed during the morphotectonic analysis as well as during an analysis of seismic reflection data and resistive data (). Based on the map of the dilatation level based on results of GPS data processing, Gunawan and Widiyantoro (Citation2019) show that the compression is relatively strong in the western part of the Baribis Fault as compared to the eastern part.
Figure 1. Map of the study area. Colored dots depict population at the village administrative level. Black lines depict active crustal faults used in this study. Faults shown on the map that are numbered 1-5 are segments of the Baribis-Kendeng Fault System (Aribowo et al. Citation2022): 1) Rarata, 2) Salak, 3) Klapanunggal, 4) Citarum, 5) Citarum front; Faults shown on the map that are numbered 6-11 are segments of the Cimandiri Fault (Marliyani et al. Citation2016): 6) Loji, 7) Cidadap, 8) Nyalindung, 9) Cibeber, 10) Saguling, 11) Padalarang; 12) the Lembang Fault (Afnimar et al. Citation2015; Daryono et al. Citation2019); Faults shown on the map that are numbered 13,14 are segments of Ujungkulon A and Ujungkulon B (Irsyam et al. Citation2020). The right inset shows the location of the western part of west Java (red rectangle) with respect to the Indonesian archipelago.

Historically, earthquakes caused by the nearby faults that resulted in damage in Jakarta occurred on January 22, 1780 and October 10, 1834 (Wichmann Citation1918; Musson Citation2012; Nguyen et al. Citation2015). Nguyen et al. (Citation2015) developed a scenario intensity model for the 1780 and 1834 events that closely match the observations (Wichmann Citation1918). Based on the high intensity levels, both events are probably intra-slab earthquakes. Previous research delineated of the Baribis Fault, but evidence of the activity on the fault is still lacking. Using seven borehole seismometers between 2019 to 2021, previous studies recorded 12 shallow earthquakes located along the Baribis Fault line (Damanik et al. Citation2021; Widiyantoro et al. Citation2022). The focal mechanism of these events indicate the existence of a thrust fault of the Baribis Fault.
An earthquake sourcing from the active Baribis Fault can trigger a reasonably high-intensity earthquake in the Jakarta area and surroundings. An earthquake of Mw 7 to 8 is capable of producing powerful shaking (MMI 7 to 8) and heavy damage (Nguyen et al. Citation2015). Although the National Centre for Earthquake Studies (PUSGEN) has produced a seismic hazard map for different areas of the island of Java, this seismic hazard map did not consider the Baribis Fault in west Java (Irsyam et al. Citation2020). This study performed a seismic hazard assessment of Jakarta and its surrounding area with additional detailed data regarding the geometry and seismicity of the Baribis Fault. To our knowledge, this is the first study on seismic hazard assessment which includes the effects of the Baribis Fault in west Java. This study combined seismic source observations and ground motion prediction, using a tree logic approach that included multiple branch source parameters to quantify seismic hazards in this area and site locations with site parameters (). The updated seismic hazard map represents the peak ground acceleration (PGA) and spectral acceleration (SA) for different time periods of 475 and 2475-year return periods, which are equivalent to 10% and 2% probability of exceedance in 50 years.
2. Data
2.1. Earthquake monitoring
The micro-earthquake detected by seven borehole seismometers confirmed the activity of the Baribis Fault (Damanik et al. Citation2021). The micro-earthquake activity was recorded with a three-component sensor instrument C100 wideband seismometer, which had a bandwidth ranging from 0.1 Hz–98 Hz and a sensitivity of 1500 V/m/sec on all components; the signal was digitized at a 32 bit resolution. The electronic sensor was installed at depths between eight to 11 meters below the surface. The investigation of seismic activity during the observation period of two years resulted in obtaining data on 12 micro-earthquakes; the focal mechanism analysis of these micro-earthquakes showed reverse faulting on planes. We calculated the average value of strike direction and dip angle of these 12 micro earthquakes and found an average value of 109°E and 57°S (Widiyantoro et al. Citation2022). The analysis of seismic hazard from the Baribis Fault as a seismic source is based on the focal mechanism solutions taken from instrument-recorded microearthquakes; the fault geometry information was then used to create seismic rates.
2.2. Local site effect
The ground motion experienced during an earthquake is influenced by the characteristics of the local soil as a medium for wave propagation from the bedrock source to the ground surface. The amplification factor can be used to transfer earthquake movements from the bedrock to the ground surface. This is done by measuring the characteristics of the subsurface layer in the field, or using a proxy method for mapping the classification of soil types to depths of 30 meters (). The
can be obtained from geomorphological data analysis and slopes from topographic data (Wald and Allen Citation2007). The microtremor array analysis shows that Jakarta is located in a sedimentary basin with a bedrock depth of up to 720 meters that is composed of soft rock and medium rock and which will amplify ground motion (Ridwan et al. Citation2017). The analysis of the regional areas,
soil classification mapping was based on SRTM topographic data, accessed from the United States Geological Survey Global
Server. Most attenuation equations include the
parameter as a variable that describes local soil conditions.
values are then classified into NEHRP site classes (Borcherdt Citation1994) and used to estimate the ground motion at each site.
2.3. Seismic source characterization
2.3.1. Active shallow faults
Seismic source modelling is an important initial stage in attempting a seismic hazard assessment, which depends on knowledge of the geometry and characteristics of seismic sources that affect a particular site. In this study, the seismotectonic was based on detailed earthquake fault sources within a radius of 250 km around the city of Jakarta (Irsyam et al. Citation2015). The Greater Jakarta seismotectonic map contains several main fault lines in west Java and south Sumatra. For the purposes of this analysis, the following faults were included in the west Java area: the Cimandiri (Marliyani et al. Citation2016), Lembang (Afnimar et al. Citation2015; Daryono et al. Citation2019), and Baribis Faults from Aribowo et al. (Citation2022). The Ujung Kulon segment of the Sumatran Fault from Irsyam et al. (Citation2020) was also included.
The Cimandiri Fault is a left lateral reverse (oblique) slip fault that consists of six major segments with a dip of 45oE and a slip rate at each segment between 0.1-0.5 mm/year (Marliyani et al. Citation2016). On the other hand, the Lembang Fault is a strike-slip fault with a dip angle of 90° and a slip rate of ∼2 mm/year (Daryono et al. Citation2019), and the Baribis Fault has an oblique thrust with a dip angle of 57oS. The Baribis Fault slip rate estimated using GPS data is ∼1.2 mm/year (Koulali et al. Citation2017). The Ujungkulon segment is located in the transition between the subduction of the Sumatra and Java segments. This fault is a strike slip fault with a dip angle of 90° and a slip rate of 10 mm/year (Irsyam et al. Citation2020). Detailed information, including sense of faulting, dimension (length, depth), slip rate, dip and maximum magnitude, is listed in .
Table 1. Source parameters for seismogenic structures.
2.3.2. Subduction zone
The tectonic setting of Java Island is controlled by the Australian and Eurasian Plates (Bock et al. Citation2003). The development of continuous GPS installations on the island of Java was carried out to determine the plate velocity and the interplate coupling model (Hanifa et al. Citation2014; Gunawan et al. Citation2016; Koulali et al. Citation2017). In addition, Widiyantoro et al. (Citation2022) suggest that the Java megathrust is capable of generating earthquakes with Mw 9.1. The modelling of earthquake subduction sources in the study region is divided into two subduction segments: the Selat Sunda megathrust and the west-central Java megathrust. The parameters of the subduction source model, including segment, dimension, dip, maximum magnitude and Gutenberg–Richter b-values, are shown in .
3. Method and result
In this study, a seismic hazard analysis was carried out using an event-based probabilistic seismic hazard analysis (PSHA); the ground motion was calculated from stochastic event sets, and the results were aggregated separately to form a probabilistic estimation. The ground motion model was divided into three tectonic regions: active shallow crust, subduction interface, and subduction intra-slab, each using a logic tree weight set of GMPEs (). The total combination of the ground-shaking intensity model is nine realizations. The magnitude-frequency distributions (MFDs) for a source, as shown in , were produced with occurrence rates using a hybrid approach (Youngs and Coppersmith Citation1986); combining Gutenberg and Richter (Citation1944) and characteristic earthquakes of a single magnitude (Schwartz and Coppersmith Citation1984). The magnitude-frequency distributions produced based on the moment rate that calculated from the parameters of Baribis Fault. The statistical parameters of Guttenberg-Richter is taken from the database of the PUSGEN (Irsyam et al. Citation2020). Moment rate across a fault source was used to derive the MFDs, the statistical approach for GR a and b-values from the database of the PUSGEN (Irsyam et al. Citation2020). The site characterization of the seismic hazard, using average shear wave velocity from the surface to 30 m depth ( was obtained from both microtremor array and the topography proxy. The GMPEs require input regarding the basin structure to determine the local depth to a fixed shear wave velocity above 1.0 km/sec (Chiou and Youngs Citation2013) and 2.5 km/sec (Campbell and Bozorgnia Citation2014) based on (
Figure 2. Magnitude frequency distribution of the Baribis Fault segment. The plotted numbers show that the Guttenberg-Richter (GR) MFD (line of blue crosses) yields many more occurrences for most magnitudes; however, these are magnitudes near the characteristic magnitude (M = 7.22), while the Young and Coppersmith (YC) MFD (line of orange crosses) rates are higher.

Table 2. Ground motion prediction equations used in seismic hazard analysis and associated logic tree weight.
The level of ground motion that would occur was calculated based on the GMPEs due to the characteristics of the seismic source. The GMPEs used are in accordance with the Indonesian region’s seismic characteristics and earthquake source model, but due to limited strong-motion recording data, currently there is no specific attenuation equation developed for the Indonesian region. The selection of the GMPEs in this study was based on the similarity of the geological and tectonic conditions of the region where the equation was constructed. The attenuation was adopted by previous research conducted by Irsyam et al. (Citation2020). In this study, we used the GMPEs used to update the Indonesia’s 2017 national seismic hazard maps (Irsyam et al. Citation2020). The GMPEs proposed by Boore et al. (Citation2014), Campbell and Bozorgnia (Citation2014), and Chiou and Youngs (Citation2013) were used for the active shallow crustal source zone. GMPEs for the tectonic region of the subduction interface combined with those by Atkinson and Boore (Citation2003), Zhao et al. (Citation2006), and Abrahamson et al. (Citation2016). For the intra-slab earthquakes, GMPEs were updated from Atkinson and Boore (Citation2003) with the Cascadia term from Atkinson and Boore (Citation2003), and with data region B/C rock site conditions and the Geomatrix 1993 SSlab. As with the Irsyam et al. (Citation2020) we use equal weights when combining the GMPEs. The weighted GMPE's is the sum of the three ground motion models for each tectonic region using equal weights of one-third ().
The resulting hazard map was constructed from the earthquake source model, using an assumption of the attenuation equation and the application of a logic tree (). The seismic source logic tree uses branches for each tectonic region type to consider all possible ground motions for all modelled earthquakes. In our model, some epistemic uncertainty of the source parameter components remains unaccounted for example; the current model employs a single MFD and single Mmax for each source.
Figure 3. The structure of the seismic source logic tree consists of three tectonic regions: active shallow crust, subduction interface, and subduction intra-slab.

The ground motion and spectral acceleration values for Jakarta and the surrounding area were estimated at a probability of exceeding of 10% and 2% in 50 years. The hazard computation was performed for the bedrock site (∼ 760 m/s) and local site conditions. The value of mean PGA for Jakarta in bedrock sites range from 0.2–0.4 g and between 0.3–0.5 g for a probability of exceeding 10% and 2% in 50 years (). PGA values also include local site conditions, ranging from 0.3–0.5 g and 0.4– 0.6 g for a probability of exceeding 10% and 2% in 50 years (). In contrast, the value of the SA for a period of 0.2 s at rock sites range between 0.5–0.7 g and 0.6–0.8 g for a probability of exceeding 10% and 2% in 50 years (). SA for the period 0.2 s include local site conditions in the range of 0.6–0.8 g and 1.0–1.2 g for possible exceedances of 10% and 2% in 50 years (). The hazard curves for metropolitan cities in west Java show that the influence of ground motion parameters is higher due to the dominant influence of local faults and soft rock ().
Figure 4. The seismic hazard map for mean PGA at bedrock corresponds to (a) 475-year return periods and (b) 2475-year return periods (equivalent to a 10% and 2% probability of exceedance (PoE) in 50 years). The same colour scale is used for both PoE. The black lines depict active crustal faults. The plotted cities are the areas evaluated in terms of hazard curves and disaggregation.

Figure 5. The seismic hazard map for mean PGA, including local site conditions that correspond to (a) 475-year return periods and (b) 2475-year return periods (equivalent to a 10% and 2% probability of exceedance (PoE) in 50 years).

Figure 6. The seismic hazard map for mean SA (0.2s) at bedrock corresponds to (a) 475-year return periods and (b) 2475-year return periods (equivalent to a 10% and 2% probability of exceedance (PoE) in 50 years). The same colour scale is used for both PoE. The black lines depict active crustal faults. The plotted cities are the areas evaluated in terms of hazard curves and disaggregation.

Figure 7. The seismic hazard map for mean SA (0.2s) including local site conditions that correspond to (a) 475-year return periods and (b) 2475-year return periods (equivalent of a 10% and 2% probability of exceedance (PoE) in 50 years).

Figure 8. Probabilistic seismic hazard curves of each city (dark green square for Bogor, purple triangle for Jakarta, and green circle for Serang). PoE is shown for an investigative period of one year. The X-axis represents peak ground acceleration in units g (gravity, 980 cm/s2). The Y-axis associated probabilities (rates) of exceedance. The red line is at 0.000404 and 0.002105, corresponding to 2% and 10% PoE in 50 years.

4. Discussion
Previous seismic hazard assessments for Jakarta have been done by Irsyam et al. (Citation2015) and Delfebriyadi (Citation2020). In comparing the previous seismic hazard map, this study has made several updates: (1) an updated seismic model for crustal active fault database (2) an updated soil condition database for site amplification, and (3) a new set of GMPE to calculate strong motion. In comparing the ground acceleration value determined in this study with that of previous studies, it was seen that there is a high hazard at ground level in the northern part of west Java for both short and long periods. The differences in the contour pattern are due to the updated geometry model used.
The hazard map was computed using an OpenQuake engine (Pagani et al.. 2014) for the mean PGA and SA at periods of 0.2 and 1.0 s with reference to the soil conditions. The map of the highest PGA hazard values was in the crustal fault line, peaking at 0.7 g and 1.0 g for 10% and 2% probability of exceedance (PoE) in 50 years. While the contribution of the subduction interface is less clear, it could be seen along the southern coastline of the island of Java, as in the western part of Ujung Kulon, where the hazard values are parallel and increase towards the coastline (). This study considered soil conditions that significantly impacted high-hazard levels. The site conditions in this study were based on the microtremor array observation (Ridwan et al. Citation2017) for the Jakarta area, while the global SRTM topography from the United States Geological Survey’s global server was used for the regional area.
In the Jakarta area, the mean PGA is 0.40 g for a 500-year return period at ground level. The hazard is dominated by the Baribis Fault which is indicated to pass through the southern part of Jakarta with the influence of a megathrust. The other factor is the depth of the engineering bedrock beneath Jakarta, which is quite deep and capable of amplifying seismic waves, making a significant contribution to the distribution of hazard values.
A hazard curve describes the earthquake hazard function associated with one of the ground motion parameters against the mean annual rate of exceedance at a location. Based on the hazard curve in several cities that have large populations, the hazard in Bogor is the highest, followed by Jakarta and Serang (). These hazard curve values are also reflected on the hazard map. Jakarta has 10% PoE of ∼0.37 g in 50 years and 2% PoE of ∼0.6 g; Serang has 10% PoE of ∼0.26 g in 50 years and 2% PoE of ∼0.44 g; Bogor has PGA with 10% PoE of ∼0.83 g in 50 years and 2% PoE of ∼1.15 g.
We compared our hazard curve results to see how the new model differs from the previous model (Irsyam et al. Citation2020). For Jakarta, the previous model has a mean PGA of ∼0.22 g with a 10% PoE in 50 years. The current model ground motion hazard is greater for ∼0.15 g. This may be due to considering some active crustal faults near Jakarta that did not contribute to the previous model. We also calculate PGA using the 12 segments of the Baribis-Kendeng Fault System from Aribowo et al. (Citation2022). The PGA result using these 12 segments is shown in . The difference between PGA modeled using 12 segments and 5 segments is shown in . High PGA difference occurred in 107.8°E, which is not part of our study area of interest. The metropolitan city area, Jakarta, Bogor, and Serang, is not affected by the Baribis segment east of 107.8°E.
Figure 9. The seismic hazard map for mean PGA at bedrock corresponds to 475-year return periods: (a) including the entire Baribis Fault segment, (b) PGA difference between the result from (a) and .

Disaggregation for the 10% probability of exceedance in 50 years shows that earthquake events at different locations significantly influence ground motion in the Jakarta area (). From the identification of the source of the earthquake due to fault activity used in the modeling, a Mw 7 at a distance of up to 50 km from the city of Jakarta can have a significant influence on ground motion; the earthquake originates from the subduction activity of the Australian and Eurasian Plates, while a Mw 8 earthquake at a distance 150-160 km is most likely to cause significant ground shaking. illustrates that a Mw 6 with a hypocenter less than 10 km has the most significant influence on the hazard in Bogor. Meanwhile, for Serang (), most incidences of ground motion are due to large earthquakes (Mw > 7) at a distance of ∼130 km. These results may be useful to analyze seismic risk on a micro-scale to assist disaster risk mitigation efforts in Jakarta and its surroundings.
5. Conclusions
We conducted a seismic hazard assessment for Jakarta and its surrounding area. The OpenQuake engine was used to calculate one of the ground motion parameters; i.e. peak ground acceleration and the response spectrum, which includes the effect of site amplification. Using microearthquake monitoring data and considering the site conditions allowed us to develop a new map of the seismic risk of Jakarta and its surrounding area. The earthquake hazard map produces a concentration trend with ground motion values in areas close to the earthquake source zone and considers soft rock types. The hazard curve in Bogor is the highest, followed by those in Jakarta and Serang. Seismic source disaggregation shows the source contribution at each location: i.e. Jakarta, Bogor, and Serang. The hazard is dominated by active faults, particularly the Baribis Fault, which is in close proximity to these cities. Overall, the acceleration values in this study are relatively higher than those in the existing Indonesian hazard maps that were published in 2017.
Acknowledgements
We thank the two anonymous reviewers for their constructive and positive comments on the manuscript. This study was supported by the Institut Teknologi Bandung (Riset Unggulan ITB, 2020) and PT. Reasuransi Maipark, Jakarta awarded to SW.
Disclosure statement
The authors declare no potential conflict of interest in the conduct and documentation of this study.
Data availability statement
Data available on request from the authors.
References
- Abrahamson N, Gregor N, Addo K. 2016. BC Hydro ground motion prediction equations for subduction earthquakes. Earthquake Spectra. 32(1):23–44.
- Afnimar, Yulianto E, Rasmid. 2015. Geological and tectonic implications obtained from first seismic activity investigation around Lembang fault. Geosci Lett. 2(1):4.
- Aribowo S, Husson L, Natawidjaja DH, Authemayou C, Daryono MR, Puji AR, Valla PG, Pamumpuni A, Wardhana DD, de Gelder G, et al. 2022. Active back‐arc thrust in north west Java, Indonesia. Tectonics. 41(7):e2021TC007120.
- Atkinson GM, Boore DM. 2003. Empirical groundmotion relations for subduction-zone earthquakes and their application to cascadia and other regions. Bull Seismol Soc Am. 93(4):1703–1729.
- Atkinson GM, Boore DM. 2008. Erratum to empirical ground-motion relations for subduction zone earthquakes and their application to Cascadia and other regions. Bull Seismol Soc Am. 98(5):2567–2569.
- Bock Y, Prawirodirdjo L, Genrich JF, Stevens CW, McCaffrey R, Subarya C, Puntodewo SSO, Calais E. 2003. Crustal motion in Indonesia from Global Positioning System measurements. J Geophys Res. 108(B8)
- Boore DM, Stewart JP, Seyhan E, Atkinson GM. 2014. NGA-West2 equations for predicting PGA, PGV, and 5% damped PSA for shallow crustal earthquakes. Earthquake Spectra. 30(3):1057–1085.
- Borcherdt RD. 1994. Estimates of site-dependent response spectra for design (methodology and justification). Earthquake Spectra. 10(4):617–653.
- Campbell KW, Bozorgnia Y. 2014. NGA-West2 ground motion model for the average horizontal components of PGA, PGV, and 5% damped linear acceleration response spectra. Earthquake Spectra. 30(3):1087–1115.
- Chiou BSJ, Youngs RR. 2013. Update of the Chiou and Youngs NGA ground motion model for average horizontal component of peak ground motion and response spectra, PEER Report 2013/07.
- Damanik R, Supendi P, Widiyantoro S, Rawlinson N, Ardianto A, Gunawan E, Husni YM, Zulfakriza Z, Sahara DP, Shiddiqi HA. 2021. Earthquake monitoring of the Baribis Fault near Jakarta, Indonesia, using borehole seismometers. Geosci Lett. 8(1):38.
- Daryono MR, Natawidjaja DH, Sapiie B, Cummins P. 2019. Earthquake Geology of the Lembang Fault, West Java, Indonesia. Tectonophysics. 751:180–191.
- Delfebriyadi. 2020. Risiko Bencana Gempabumi untuk DKI Jakarta. Bandung: Institut Teknologi Bandung.
- Geomatrix Consultants. 1993. Seismic margin earthquake for the Trojan site: final unpublished report prepared for Portland General Electric Trojan Nuclear Plant, Ranier, Oregon.
- Gunawan E, Meilano I, Abidin HZ, Hanifa, NR, Susilo. 2016. Investigation of the best coseismic fault model of the 2006 Java tsunami earthquake based on mechanisms of postseismic deformation. J Asian Earth Sci. 117:64–72.,
- Gunawan E, Widiyantoro S. 2019. Active tectonic deformation in Java, Indonesia inferred from a GPS-derived strain rate. J Geodyn. 123:49–54.
- Gutenberg B, Richter C. 1944. Frequency of earthquakes in California. Bull Seismol Soc Am. 34(4):185–188.
- Hanifa NR, Sagiya T, Kimata F, Efendi J, Abidin HZ, Meilano I. 2014. Interplate coupling model off the southwestern coast of Java, Indonesia, based on continuous GPS data in 2008–2010. Earth Planet Sci Lett. 401:159–171.
- Irsyam M, Cummins PR, Asrurifak M, Faizal L, Natawidjaja DH, Widiyantoro S, Meilano I, Triyoso W, Rudiyanto A, Hidayati S, et al. 2020. Development of the 2017 national seismic hazard maps of Indonesia. Earthquake Spectra. 36(1_suppl):112–136.
- Irsyam M, Hutabarat D, Asrurifak M, Imran I, Widiyantoro S, Hendriyawan Sadisun I, Hutapea B, Pindratno H, Afriansyah T. 2015. Development of seismic risk microzonation maps of Jakarta City. London: Taylor & Francis Group.
- Koulali A, McClusky S, Susilo S, Leonard Y, Cummins P, Tregoning P, Meilano I, Efendi J, Wijanarto AB. 2017. The kinematics of crustal deformation in Java from GPS observations: implications for fault slip partitioning. Earth Planet Sci Lett. 458:69–79.
- Marliyani GI, Arrowsmith JR, Whipple KX. 2016. Characterization of slow slip rate faults in humid areas: cimandiri fault zone, Indonesia. J Geophys Res Earth Surf. 121(12):2287–2308.
- Musson RMW. 2012. A provisional catalogue of historical earthquakes in Indonesia. Edinburgh, British: Geological Survey. Open Report OR/12/073.
- Nguyen N, Griffin J, Cipta A, Cummins PR. 2015. Indonesia’s Historical Earthquakes: modelled examples for improving the national hazard map. Canberra: Geoscience Australia. Report No: GA2015/23.
- Pagani M, Monelli D, Weatherill G, Danciu L, Crowley H, Silva V, Henshaw P, Butler L, Nastasi M, Panzeri L, et al. 2014. OpenQuake engine: an open hazard (and risk) software for the global earthquake model. Seismol Res Lett. 85(3):692–702.
- Ridwan M, Widiyantoro S, Irsyam M, Yamanaka, H, Afnimar. 2017. Development of an engineering bedrock map beneath Jakarta based on microtremor array measurements. SP. 441(1):153–165.,
- Schwartz DP, Coppersmith KJ. 1984. Fault behaviour and characteristic earthquakes: examples from the Wasatch and San Andreas fault zones. J Geophys Res. 89(B7):5681–5698. (cited on page 26).
- Simandjuntak TO, Barber AJ. 1996. Contrasting tectonic styles in the Neogene orogenic belts of Indonesia. Geol Soc London Spec Publ. 106(1):185–201.
- Supendi P, Nugraha A, Puspito N, Widiyantoro S, Daryono D. 2018. Identification of active faults in West Java, Indonesia, based on earthquake hypocenter determination, relocation, and focal mechanism analysis. Geosci Lett. 5(1).
- Wald DJ, Allen TI. 2007. Topographic slope as a proxy for seismic site conditions and amplification. Bull Seismol Soc Am. 97(5):1379–1395.
- Wichmann A. 1918. Die Erdbeben des Indischen Archipels bis zum Jahre 1857, Verh. der K. Akad. van Wet. Te Amsterdam. 20(4):193. (in German).
- Widiyantoro S, Supendi P, Ardianto A, Baskara AW, Bacon CA, Damanik R, Rawlinson N, Gunawan E, Sahara DP, Zulfakriza Z, et al. 2022. Implications for fault locking south of Jakarta from an investigation of seismic activity along the Baribis fault, northwestern Java, Indonesia. Sci Rep. 12(1):10143.
- Youngs RR, Coppersmith KJ. 1986. Implications of fault slip rates and earthquake recurrence models to probabilistic seismic hazard estimates. Int J Rock Mech Mining Sci Geomech Abstr. 23(4):125.
- Zhao JX, Irikura K, Zhang J, Fukushima Y, Somerville PG, Asano A, Ohno Y, Oouchi T, Takahashi T, Ogawa H. 2006. An empirical site-classification method for strong-motion stations in Japan using H/V response spectral ratio. Bull Seismol Soc Am. 96(3):914–925.