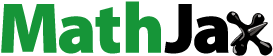
Abstract
Despite their protracted periods of inactivity, long-dormant volcanoes may be highly hazardous, as their reactivation can be characterized by violent explosive eruptions. An example of such volcanoes is the Colli Albani caldera, onto which deposits Rome Capital City is built, Italy. Its last volcanic activity was characterized by voluminous maar-forming phreatomagmatic eruptions dated between 36 and 25ka, but the volcano has produced several maar lake overflows during the Holocene till historical times. Presently, Colli Albani is affected by recurrent seismic events, anomalous heat flow, ground uplifts, hydrothermal circulation and gas emissions. For these reasons, the Italian Civil Protection has recently listed Colli Albani among the ten active volcanoes of Italy, but products for the evaluation of its volcanic hazards lacking. This work presents the first study on vent opening susceptibility mapping at Colli Albani. We explore the potential of an available geological dataset for building, through geographic information system analysis, an index that classifies areas at different vents opening susceptibility (low, moderate and high). Such result highlights as a solid geological mapping is a prerequisite for the volcanic hazard assessment, especially in remote or poorly studied long-dormant volcanoes such as caldera systems where the location of new vents could occur in different volcano sectors.
1. Introduction
The evaluation of volcanic hazard is a challenging scientific task and for this reason it is performed by using different approaches, whose common purpose is to estimate the probability that a particular portion of territory can be affected by a specific volcanic event in reference to a specific time scale (Molist Citation2018). This specific time scale is qualitatively assumed as ‘short’ or ‘long’, depending on the physical state of the volcano itself (Marzocchi et al. Citation2008; Giordano and Caricchi Citation2022). Long dormant volcanoes represent a major challenge for hazard studies. The significance of quiescence since the last eruption, especially at calderas, is not straightforward as it may reflect the end of volcanism or alternatively a recharge period (Giordano and Caricchi Citation2022). Commonly, if the last quiescence is longer than the Holocene the volcanoes are considered extinct (Smithsonian Institution, https://volcano.si.edu). However, an increasing number of long-dormant calderas cannot be simply discarded as such, because of signs of unrest, or based on quiescence periods longer than 10 kyr in their rock record (Szakács Citation1994; Connor et al. Citation2006; Scandone et al. Citation2016). Giordano and Caricchi (Citation2022) have recently questioned the simple statistical approach to the repose time as the longevity of the magma plumbing systems involve the thermal and chemical maturation of the crust, which, for given magma fluxes, becomes progressively prone to the accumulation of larger volumes of eruptible magma across longer quiescence (e.g. Jellinek and De Paolo Citation2003; Annen Citation2009; Karakas et al. Citation2017; Weber et al. Citation2020). Therefore long-dormant calderas should be carefully studied as they may be accumulating magma for a next large volume eruption (e.g. Liu et al. Citation2021).
In case of long dormant volcanoes, the hazard evaluations are made over long time frames and are based on available information referred to the past eruptive activity. Such information, mainly derived by geological records of past eruptions (Calder et al. Citation2015; Verolino et al. Citation2022), is inherently incomplete as the preservation of volcanic deposits depends on many factors such as type of lithology, thickness, erosional processes and volcano-tectonic context (Brown et al. Citation2014). In addition, both burial and selective removal may significantly reduce the completeness of what can be observed by geologists and represented on geological maps (Lucchi et al. Citation2013), which can be obscured also by anthropic activity. All these aspects prevent an accurate reconstruction of the spatio-temporal distribution of eruptive activity and consequently introduce significant uncertainties in quantitative hazard assessment of long dormant volcanoes.
Models used for evaluating volcanic hazards can be distinguished in three main categories: quantitative, semi-quantitative and qualitative. These are based on statistical or heuristic approaches. The data analysed and/or elaborated by the models depend on the physical state of the volcano and are distinguished in three different typologies: (1) data referred to past volcanic phenomena; (2) monitoring data, (3) data obtained by numerical simulations that reproduce and model volcanic phenomena. Regardless of the used approach, the most common product derived from such models is a ‘hazard map’ that classifies the investigated territory in areas at different degrees of hazard. In detail, according to the complexity of the model and type of used approach, the hazards map can be a probability map, a susceptibility map or a zoning map and can be improved associating an estimation of the uncertainty, fundamental requisite to better test the reliability of the results (e.g. Bevilacqua et al. Citation2015).
Nowadays, the hazard maps constitute one of the fundamental tools for the volcanic risk assessment in areas characterized by the presence of both active and long dormant volcanoes (Lirer et al. Citation2010; Martí et al. Citation2012; Selva et al. Citation2012; Bevilacqua et al. Citation2015, Citation2017; Neri et al. Citation2015; Tadini et al. Citation2017, Tierz et al. Citation2018; Marrero et al. Citation2019; Chevrel et al. Citation2021).
In case of hazard maps associated with eruptive vents opening, most works in literature use probabilistic approaches applying different methodologies. For example, the main vent opening maps developed for Campi Flegrei take in account a Bayesian inference procedure that reports the uncertainty ranges for probability values (Selva et al. Citation2012). Bevilacqua et al. Citation2015 have proposed a probabilistic conditioned approach based on selected variables whose uncertainty is estimated through the structured experts elicitation. Another approaches, based on the Kernel Density Estimation (KDE) statistical method are proposed in Verolino et al. (Citation2022), Cappello et al. (Citation2012) and Bartolini et al. (Citation2013) for the Bolaven Volcanic Field, Etna, Lanzarote and La Garrotxa volcanoes, respectively. Finally, more qualitative approaches were used to map the opening of new vents at Ischia volcano. The produced maps (Alberico et al. Citation2008; Sbrana and Toccaceli Citation2011) identify the areas affected by a possible vent opening combining some geological, geochemical and geophysical indicators.
The study here presented is focused on Colli Albani, a long dormant volcano characterized by active degassing of dominantly CO2 associated with impulsive and hazardous gas eruptions (Anzidei et al. Citation2008; Carapezza et al. Citation2010; Vignaroli et al. Citation2015; Giordano et al. Citation2016), periodic seismic swarms and shallow local seismicity (Amato et al. Citation1994), ground deformation (Amato and Chiarabba Citation1995; Anzidei et al. Citation2010; Marra et al. Citation2016) and the presence of an active geothermal system (Giordano et al. Citation2014). The volcano is listed among the ten active volcanoes of Italy by the Italian Civil Protection (https://rischi.protezionecivile.gov.it/it/vulcanico/vulcani-italia/colli-albani) but so far, no hazard maps have been produced for Colli Albani volcano.
In this paper we discuss the use of available geological data for the definition of the reference period in the hazard assessment and the production, for the first time, of a susceptibility map for the opening of new eruptive vents. This map provides preliminary indications on the areas exposed to studied phenomena. With respect to hazards maps based on more probabilistic approaches, our susceptibility map relates geological datasets recognized in literature and/or by scientific experts as significant predisposing factors to the phenomena in question, providing the initial step for developing probabilistic hazard maps. The Colli Albani volcano represents an excellent case study of long dormant caldera system for which a conspicuous geological dataset is available after the survey and analyses performed for the new geological maps of the area (Giordano, Benedetti, et al. Citation2010), so the results will be discussed in view of their relevance for this kind of volcanoes.
2. Geological background of Colli Albani volcano
The Colli Albani edifice is a caldera volcanic complex located to the SE of Rome City (Giordano, Benedetti, et al. Citation2010; Giordano, Mattei, et al. Citation2010), starting its activity at about 600 ka erupting mafic K-alkaline magmas, ranging from tephrite to tephri-phonolite and foidites (Boari et al. Citation2009; Conticelli et al. Citation2010). The age of the deposits of the most recent preserved eruption is debated to have occurred at 36 ka (Marra et al. Citation2003; Giaccio et al. Citation2009), 25 ka (Villa et al. Citation1999) or even in more recent times (Voltaggio et al. Citation1994; Soligo et al. Citation2003; Soligo and Tuccimei, Citation2010). No historical eruptions are documented, but phreatic activity from Albano maar lake centre produced several lahars associated with the Albano maar lake overflows, grouped in the Tavolato Formation (Funiciello et al. Citation2003, Citation2010; De Benedetti et al. Citation2008) which is well constrained by 14 C dating, archaeological findings and historical accounts between 21.089 ± 100BP ka and the III century B.C. (De Benedetti et al. Citation2008; Anzidei and Carboni Citation2020).
The Colli Albani volcanic stratigraphy shows the superposition over time of different edifices associated with three eruptive periods distinct from each other in terms of eruption style, total volume of erupted magmas and average mass eruption rate (; Giordano, Benedetti, et al. Citation2010).
Figure 1. Schematic Geological map of the Colli Albani volcano derived from the 1:50,000 scale geodatabase of the Italian official Geological Map (ISPRA; Giordano, Benedetti, et al. 2010). Legend: (0) topographic caldera rim; (1a) Holocene alluvial and coastal deposits; (1b) undefined sedimentary rocks; (2) via dei Laghi maar complex (ca. 200 ka–Holocene); (3) Faete intracaldera stratovolcano (< 355 ka–250 ka); 4) Tuscolano-Artemisio post-caldera fissural system (<355 ka–180 ka); (5) Vulcano Laziale caldera and ignimbrite plateau (>600 ka–355 ka). Note that grey lines internal to coloured fields indicate boundaries between individual rock formations.

The first Vulcano Laziale period (ca 600–355 ka) was characterized by intense and voluminous caldera collapse events producing a succession of at least seven ignimbrites of great extent, covering an area of ca. 2000 km2. The related edifice is named Vulcano Laziale caldera complex, formed by the central 10 km × 10 km morphologically wide caldera and the surrounding flat ignimbrite plateau, at the NW edges of which, the ancient city of Rome was built (). The ignimbrites reached the scale of several tens of cubic kilometres (Volcanic Explosivity Index VEI 5-7) and can be classified as intermediate (Giordano and Cas Citation2021), defining the Colli Albani volcano as the most explosive mafic volcano on Earth (Giordano, Benedetti, et al. Citation2010). After the last major caldera-forming event occurred at ca. 355 ka (Villa Senni eruption; Watkins et al. Citation2002; Vinkler et al. Citation2012), the caldera underwent deflation, reduction of average mass eruption rates and became dominated by effusive, strombolian and sub-plinian eruptions from an emerging intracaldera stratovolcano and a series of circumferential and radial fissures. This period is known as Tuscolano-Artemisio and Faete period (<355–180 ka) and several composite edifices, partly overlapping in time and space, were built (): the Tuscolano-Artemisio, Pantano Borghese, S. Maria delle Mole and Monte Due Torri fissure systems, and the Faete intracaldera stratovolcano. The most recent period of activity at Colli Albani volcano is known as via dei Laghi (VdL) period (<200 ka – dormant), when the locus of the activity shifted to the western sector of the caldera producing mostly small volume phreatomagmatic activity from several scattered vents which form the VdL maar field.
The most recent VdL period includes the phreatomagmatic deposits erupted from several monogenetic to polygenetic maars located along the northern and western slopes of Colli Albani edifice (; Giordano, Benedetti, et al. Citation2010). The oldest VdL products are dated at ca 200 ka from the Ariccia maar deposits (Marra et al. Citation2003), while the youngest have been erupted from the Albano maar lake, with debated 40Ar-39Ar ages comprised between 36 ka and 25 ka (Villa et al. Citation1999; Giaccio et al. Citation2009). The eruptive styles were mainly controlled by efficient explosive magma-groundwater interaction that produced intense magma fragmentation and dilute to concentrated pyroclastic density currents radially distributed up to 10 km from the vent (Giordano et al. Citation2002; Porreca et al. Citation2003; Sottili et al. Citation2009). The deposits contain abundant xenolith fragments from the carbonate sedimentary substrate, as well as from intrusive and thermometamorphic rocks, testifying the magma/rock interaction depth within the geothermal reservoir (De Benedetti et al. Citation2010).
Figure 2. Distribution of the via dei Laghi maar complex (TAL, Tavolato formation; UAL, Albalonga unit; SDV, Villa Doria unit; MNN, Peperino Albano; STL, Cantone unit; KRL, Corona del Lago unit; DSN, Coste dei Laghi unit; TGO, Montagnaccio unit), QUARTO LAGHETTO MAAR eruptive centre (PVN, Pavona unit; KLG, Quarto Laghetto unit), ARICCIA MAAR eruptive centre (ICA, Ariccia unit), NEMI MAAR eruptive centre (NEM, Nemi unit). The data derived from Geological map of Colli Albani volcano, Giordano, Mattei, et al. (Citation2010).

The VdL products lie directly on the deposits of the older edifices (Vulcano Laziale, Tuscolano-Artemisio, Faete) and are described and mapped in Giordano, Benedetti, et al. Citation2010; Giordano, Mattei, et al. Citation2010. The distribution of the maar field shows preferential NW-SE and N-S trends (), following the main regional structural trends and suggesting a tectonic control on the location of vents (Giordano et al. Citation2014). Giordano, Benedetti, et al. (Citation2010) interpreted the shift from the Tuscolano-Artemisio and Faete period to the VdL period as related to a major local stress change from deflation to inflation within the caldera which affected the permeability of faults as pathways for magma. This long-term change matches also location and geometry of the recent ground uplift centred in the Albano maar lake (Amato and Chiarabba Citation1995; Riguzzi et al. Citation2009; Marra et al. Citation2016), periodic seismic swarms (Amato et al. Citation1994) and intense degassing from the lake region and its surroundings (Anzidei et al. Citation2008; Carapezza et al. Citation2010). The volcanic-tectonic evolution of the volcano coupled with the geophysical and geochemical parameters recently measured, suggest that the VdL eruptive period appears the most appropriate reference framework to adopt for hazards studies at Colli Albani volcano.
3. Conceptual framework and datasets
This section describes the conceptual framework and the data used to produce the vent opening susceptibility map related to the long dormant Colli Albani volcano.
The current understanding of the physical state of Colli Albani volcano does not allow us to know whether eruptible magma is present or not in its plumbing system. However, observed ground deformation has been suggested as evidence for magma migration at crustal levels (Chiarabba et al. Citation1997; Trasatti et al. Citation2018). The upward migration of magma through a dyke is described by the following equation (Gudmundsson Citation2012):
(1)
(1)
where Pm is the magmatic pressure, T0 is the tensile strength of rocks and s1–s3 is the tectonic pressure. Magma being a liquid, Pm is isotropic, while any fabric of the rocks and their stress state due to far-field tectonic stresses will either facilitate or oppose to magma upward migration influencing T0 along the dyke path (Geshi et al. Citation2012). For example, a horizontal layering associated with strong rheological contrasts between layers may favour the intrusion of a sill, whereas the presence of vertical fractures/faults/dykes, especially in an extensional tectonic regime, facilitates the upward propagation of a dyke.
Within this framework, the data used in this work are the geo-volcanological elements identified as predisposing factors to the possible opening of new eruptive vents. This approach is in agreement with several previous works (Bevilacqua et al. Citation2015, Citation2017; Tadini et al. Citation2017) where the identification of the predisposing factors is based on expert’s opinions. In this work the geo-volcanological elements are features that identify where: (1) magmatic overpressure Pm had produced the injection of a dyke and eruptions in the geological record, i.e. point source vents and eruptive fractures; (2) the tensile strength of rocks T0 is weakened by the presence of subvertical faults and fractures; (3) the regional tectonics (s1–s3) allows the development of fracture systems; (4) the vertical permeability allows the migration of deep-sourced fluids; (5) the youngest ages of the identified features and evidence of active deformation may reflect the location at depth of the active plumbing system.
The dataset utilized for analysing the susceptibility to vent opening at Colli Albani largely derives from the 1:25,000 scale Geological Database associated with the Geological map of the Colli Albani volcano surveyed at 1:10,000 scale and published at 1:50,000 scale (CARG Project, ISPRA, Giordano, Mattei, et al. Citation2010) ().
Figure 3. The map showing the dataset derived from Giordano, Mattei, et al. (Citation2010). The orange circle (Sector A) identifies the areas enclosing by the most recent activity of the volcano (via dei Laghi maar field).

The elements considered, the motivation for their choice, and the evaluation of the uncertainties about their exact spatial location are the following:
Point source eruptive vents (maars and scoria cones); the location of past eruptive point sources provides evidence both for pathways already used by magma in the past and because it implies the presence of a dyke in the subsurface and associated (sub)vertical fracturing; the younger vents may also be taken as indication for the location of the youngest magma storage regions; their spatial location is certain as it is associated with mapped outcropping edifices.
Eruptive fractures (EF); lavas issued from polygenetic eruptive fractures, along which also point source vents are locally aligned are considered strong evidence for the presence of dykes in the subsurface and associated vertical fractures; their spatial location is considered certain, although it derives from the interpolation of outcrops that define the fissural source region for lavas and scoria cones.
Caldera ring faults (RF); these are the traces of the volcano-tectonic faults along which the polyphased Colli Albani caldera has repeatedly collapsed and also along which many post-caldera monogenetic centres are located; for these reasons they are considered elements that may facilitate magma uprise; these elements are buried and their spatial location is inferred at the base of the morphological inner caldera scarp (Lipman Citation1997) and also constrained by interpreted bore-holes stratigraphic logs (Diano et al Citation2010).
Tectonic faults (F); mapped faults in the Colli Albani region are high angle, extensional and/or transtensional as kinematics (Faccenna et al. Citation1994; Giordano et al Citation2006; Giordano, Mattei, et al. Citation2010), and therefore considered potential weakness zones facilitating the vertical permeability to fluids; as an evidence, most of the active or fossil sites associated with hydrothermal fluids upwelling (e.g. travertine, CO2 vents, zones of hydrothermal mineralization) are locally controlled by faults (Vignaroli et al. Citation2015). The spatial location of faults is defined based on the interpolation of sites where deformation is visible in outcrop, morphostructural interpretation of topography, and, where available, indirect data coming from geophysics or the interpretation of bore-hole stratigraphic logs (e.g. Diano et al Citation2010).
Gas vents (GV); these features represent localized active or fossil CO2 and H2S emission points; they represent a direct hazard (Carapezza et al. Citation2010, Citation2021), and are located in correspondence of structures acting as preferential pathways for the upwelling of hydrothermal fluids from the Colli Albani geothermal system (Giordano et al. Citation2014); their location is certain as it corresponds to measurable points on the surface;
Morpho-structural lineaments (L); alignments of morphological features (ridges, valleys volcanic features, etc) are considered a reliable proxy for tectonic fracturing (Wise et al. Citation1985; Pardo et al. Citation2009) and can be spatially approached in terms of lineament density distribution (km/km2) indicating the area most likely affected by crustal fracturing (e.g. Gabrielsen et al Citation2002; Giordano et al. Citation2013); the uncertainties associated with lineament identification derive in part from the resolution of the analysed aero photogrammetry and satellite images (ca 1 m) and digital elevation models (DEM) (5 m) and partly from the operator bias; details about the methodology of the dataset used for Colli Albani can be found in Giordano et al. Citation2014; the statistical treatment in terms of lineament density distribution alleviates the uncertainties about the exact location of individual lineaments;
A further geo-spatial element here defined and named Sector A (), identifies the area that encloses the point sources of the most recent volcano activity (VdL maar field; Giordano Mattei, et al. Citation2010), the Albano mare lake as the source of several Holocene to historical phreatic/limnic eruptions (Funiciello et al. Citation2003; De Benedetti et al. Citation2008), as well as the area affected by signs of geophysical unrest that are: the 1990–1991 seismic swarm (Amato and Chiarabba Citation1995), the ground uplift centred around the Albano maar lake measured between 1951 and 1994 by precision topographic levelling (Chiarabba et al. Citation1997), and the area identified by InSAR data as currently in uplift (Trasatti et al. Citation2018); the outline of Sector A is qualitative so it must not be considered a well-defined feature in its outer extent, however the overlap within this sector of all the recent volcanic and phreatic activity as well as of present deformation is considered proxy as the most active sector of the volcano and possibly evidence for the most likely area of a future volcanic activity.
All geo-volcanological elements above mentioned are graphically represented by geo-localized geometry entities (points, polylines and polygons) organized in datasets () connected to alfa-numerical databases storing the most important information on the elements themselves.
Figure 4. The scheme reports the datasets analysed in this work indicating, for each one, the source from which the dataset was derived, the geo-volcanological element of reference, the name of the dataset, and the geometry entity used for the geo-spatial representation.

Point source eruptive vents are represented by point entities with no associated uncertainties: the location of scoria cones is defined as the summit of well exposed conical edifices; maars locations are defined as the centroids of the crater rims or, in the case of poly-phasic Albano maar lake, as multiples vents, defined as exact location by detailed bathymetric and seismic data (Anzidei et al. Citation2008).
The Eruptive fractures, the Caldera ring faults and the Tectonic faults are polylines. The associated uncertainties on their exact location previously described has been accounted for by using a buffer of 250 m on each side of the linear feature (cf. Giordano et al. Citation2014). The width of the buffer takes into account that faults and associated fracturing in the poorly consolidated volcanic rocks at Colli Albani are diffused (see Vignaroli et al. Citation2015 for details).
Gas vents are point entities located at the sites of surveyed active or fossil emission points.
Morpho-structural lineaments derived by aero-photogrammetry, satellite images analyses and the Colli Albani DEM were statistically distinguished in two different populations, characterized by length > 2 km (L1) and < 2 km (L2), as tracers of deep and shallow fracturing, respectively (Giordano et al. Citation2014). These tracers are represented with the density of lineament length per unit area (km/km2) and therefore associated with the polygon feature. Finally, Sector A, as defined above, is represented by a polygon feature ().
4. Vent opening susceptibility map
4.1. Methodological approach
The vent opening susceptibility map was obtained by calculating the Vents Opening Index (VOI) in a study area covering 1296 km2 and centred on a zone involving three volcanic structures Faete and Nemi Maar, Albano maar Lake (). The map is represented by a vector matrix with cell size of 1 km, which is a value consistent with the purpose of the work, the extent of the study area and the spatial scale of the datasets. In particular, such cell size allows to consider the spatial uncertainty of the points more prone to the new eruptive vent opening generated by the potential dike. Not knowing both the depth nd the orientation of the dike, the dispersion of the points on 1 km2 was evaluated reasonable. In our approach, each cell (1 km × 1 km) becomes the spatial reference element that will be mapped through the VOI. The index was calculated by solving an equation that combines, in GIS environment (ESRI platform), the datasets defined for each geo-volcanological element recognized as relevant predisposing factors to the future vent opening. The VOI equation (EquationEquation 2(2)
(2) ) is built with semi-heuristic methodology assigning a specific weight to each dataset (hereafter defined parameter), which reflects the inferred influence on generating the predisposition to vent opening. The vector matrix of 1296 cells are linked to an alphanumeric database that reports for each squared cell the ‘number’ of the datasets features falling or crossing the cell itself. This ‘number’ is individuated through specific spatial analysis tools available on GIS platform. In detail, such tools identify and count the elements falling or crossing each cell following the topological spatial relations between different vector geometries. For dataset with point geometry, the element is counted only if it falls within the cell in analysis (Boolean criteria). For datasets represented with line or polygon geometry, the element is counted if it satisfies the prevalence criteria (more than 50% of cell area have to be covered or crossed by the polygon and line element, respectively) (Burrough et al. Citation2015).
In the VOI equation (EquationEquation 2(2)
(2) ), for all parameters (Pi) except the Sector parameter (P9), the number (n) of geo-volcanological features falling or crossing any analysed cell is multiplied for the weight of the respective parameter (WPi). The obtained values are summed, and the result is furtherly multiplied by the value associated to the Sector parameter (S) in which the geo-volcanological features are located. The weights used in the equation have values equal to 1, 0.5, 0.3, 0.2, 0.1 and represent the percentages reported to the unit indicating how much each parameter Pi weights. Therefore, 1, 0.5, 0.3, 0.2 and 0.1 indicate that the respective parameter weights at 100%, 50%, 30%, 20% and 10%. These weights have been assigned by the authors based on their expert evaluation of both the degree to which each factor effectively predisposes the area to a future vent opening and the degree of their associated spatial certainty.
The Sector parameter (P9) can assume two different values depending on the sector in which the analysed cell is located. If the cell is in the sector affected by the most recent activity (Sector A) the parameter assumes value equal to 2. Instead, when the analysed cell falls outside the Sector A, the parameter assumes a lower value equal to 1. The implemented equation is above reported:
(2)
(2)
where each dataset is indicated with the parameter Pi and the respective weight is indicated with Wpi. In detail, P1, P2, represent the Point source eruptive centres (Maar and Scoria cones respectively); P3 corresponds to the Gas vents; P4, P5 indicate respectively the dataset of Eruptive Fractures and the Caldera Ring Faults, whereas P6 identifies the datasets of Tectonic Faults; P7 and P8 correspond to the dataset of Morpho-structural Lineaments having different characteristics: P7 indicates Lineaments with length ≥ 2 km, whereas P8 defines Lineaments with length < 2 km; finally, P9 identifies the Sector parameter used to distinguish the areas characterized by recent activity from areas affected by oldest activity.
The weight assigned to P1, P2, is the same because both parameters represent the same typology of dataset (eruptive centres with certain spatial location) and assume the value of 1 because both Maars and Scoria Cones represent past eruptive vents. They testifying the presence at depth of the magma plumbing system and the rising of magmas, therefore are recognized as factors with the high predisposition to vent opening in case of reactivation.
The weight assigned to P3 (Gas vents) is equal to 0.5 as it represents the certain presence of vertical pathways of hydrothermal fluids and local weaknesses which connect the active geothermal system, but do not necessarily indicate the connection with the magma plumbing system as geothermal fluids can be laterally advected away from the magmatic source (Giordano et al. Citation2014).
The weights of P4, P5 and P6 are equal to 0.5 because the Eruptive Fractures, the Caldera Ring Faults and the Tectonic Faults are considered equivalent predisposition factors to define vertical permeability, but the 0.5 weight mitigates the effect of the buffer used to account for their special uncertainty.
The weights assigned to parameters P7 and P8 are lower than previous ones and, although both are referred to Morph-structural lineaments detected by remote sensing analyses, they are slightly different. In particular, P7 (lineaments with length ≥ 2 km) weights 0.3 or 0.2 according to the density class as it indicates deep fracturing likely associated with the structural fabric of the substrate, whereas P8 (lineaments with length < 2 km) weights 0.2 or 0.1 according to the density class as it indicates shallow fracturing, likely affected by the highly variable mechanical properties of shallow rocks and sediments (see Giordano et al. Citation2014).
Finally, P9 identifies the Sector A or B. It will assume value equal to 2 if the cell in analysis falls in areas characterized by recent activity (Sector A), and it will assume value equal to 1 if the cell falls in the other Sector (B).
4.2. The vents opening susceptibility map
The vent opening susceptibility map resulting from EquationEquation (2)(2)
(2) () identifies zones characterized by a different predisposition to potential vents opening in case of resumption of Colli Albani volcanic activity. The values of each cell calculated by VOI index were visualized in three different intervals through the Natural Break algorithm, as considered one of more suitable methods to obtain a reliable data clustering. These intervals identify areas with different susceptibility: high, moderate, low. The red zones, classified at high susceptibility (VOI > 2.50), involve mainly the central zone of the study area, in particular the south-west portion dominated by the presence of the volcanic structures of Albano maar lake, Nemi and Ariccia maars. Further areas at high susceptibility are visible inside the caldera along the Faete structure and in the north-eastern external sector of the caldera margin characterized by the presence of significant volcano-tectonic structures, as eruptive fissure, and scoria cones alignments. The zones at high susceptibility cover 71 km2 and correspond to the 5.5% of the entire study area. The zones at moderate susceptibility (1.24 < VOI < 2.50), coloured in orange (), interest all the study area but their distribution is not homogenous. In fact, they are strongly concentrated in the central area where the caldera volcanic structures are present and, in the west and south portions that result mainly characterized by the presence of the structural lineaments. The moderate susceptibility zones cover around 576 km2 and combined with 71 km2 of red zones identify an area of 647 km2 (around 50% of the entire study area). The zones classified low susceptibility (VOI < 1.24), coloured in yellow, cover the remaining area of 604 km2, whereas a total of 45 km2 results classified as no data and is localized in the North-West corner of the study area.
5. Discussion
The Colli Albani volcano represents an excellent long-dormant caldera case study, where signs of geophysical and geochemical unrest, along with evidence of an active geothermal system, suggest that it must be considered potentially active (Chiarabba et al. Citation1997; Funiciello et al. Citation2003; Salvi et al. Citation2004; De Benedetti et al. Citation2008; Riguzzi et al. Citation2009; Carapezza et al. Citation2010, Citation2021; Giordano et al. Citation2014; Marra et al. Citation2016, Citation2020; Trasatti et al. Citation2018; Giordano and Caricchi Citation2022). In this regard, the proximity of the caldera to the metropolitan area of Roma, with more than 2.5 million people, requires the implementation of hazard studies. In this work, the methodology applied to evaluate the hazard by vents opening at Colli Albani volcano uses a semi-quantitative heuristic approach based on the spatial analysis of past eruptive centres combined with the most relevant volcano-tectonic features, and also considering the location of the areas involved by the geophysical unrest recorded in the last twenty years. In detail, the review, classification, and analyses of a conspicuous geological data set available for the Colli Albani caldera system allowed for the first time to produce a susceptibility map of new vents opening in case of renewal of volcanism. Our results identify sectors characterized by highest values of susceptibility which are mainly associated with the most recent volcanic structures. The location and age of the past eruptive vents, reflecting the volcanic evolution of the caldera, largely controls the results, although some outliers exist associated especially with areas where high density of tectonic lineaments suggest the presence of weakness zone favourable for magma rise. The obtained map shows that the area affected by the recent maar structures correspond to the zone in which the predisposition to a potential opening of new eruptive vents is high, whereas more than 50% of zones affected by older scoria cones are identified at moderate susceptibility, due their location along older and likely less active volcano-tectonic structures. The map highlights an area of particular interest positioned to west/south-west with respect to the Faete intra caldera stratovolcano, where several zones are classified at high susceptibility. Looking at the whole analysed area, the high susceptibility zones are concentrated within and along the caldera structure, with a slightly NE-SW elongated trend that could be related to the presence of regional trending faults along which the most recent eruptive and gas vents occurred. Even if we did not analyse monitoring data, many of these high susceptibility zones correspond to areas where recently ground deformation and seismic events have been recorded (Chiarabba et al. Citation1997, Citation2010; Riguzzi et al. Citation2009; Trasatti et al. Citation2018), likely in correspondence of the most active volcano-tectonic structures of the caldera. The areas characterized by the high susceptibility values, in addition to the caldera structure, appear to be concentrated along volcano-tectonic structures that follow regional trends and exert the main control on the recent evolution of the volcano and its manifestations. Finally, the areas with a lower degree of susceptibility, classified as moderate, cover the 50% of the entire study area and particularly affect the western and southern sectors outside the caldera. These zones are mainly characterized by the presence of tectonic lineaments, which can be assumed as proxy for their re-activation by volcano-tectonic processes representing areas of upper crustal weakness in the caldera that could affect magma intrusion in a case of unrest. The methodological approach proposed here highlights that geological dataset, generally related to geological maps, can be used to obtain useful basic products for volcanic hazard assessment in areas usually not provided by a monitoring network and also since often considered ‘non-active’ volcanic areas. In particular, the example of the long dormant Colli Albani caldera shows how the volcanological and structural data properly processed allowed the production of a susceptibility map for eruptive vent openings. This outcome represents a fundamental step for a subsequent development of probabilistic hazard maps, especially in approaching volcanic hazard assessment for long dormant volcanoes.
6. Conclusive remarks
This study represents the first attempt to map the hazard from vents opening at Colli Albani, a long dormant volcano. The approach proposed here is based on a semi-quantitative heuristic model applied to a conspicuous geological dataset coming from CARG Project. The model, developed on a GIS environment, produces a numerical index (VOI) able to map the susceptibility to potential opening of new eruptive vents in the Colli Albani area. Combining a set of geo-volcanological elements (faults, ring faults, eruptive fractures, lineaments, scoria cones, maars, degassing vents) through an equation attributing to each element specific weights previously discussed by the experts, the model calculates the VOI index for each km2 of the study area assigning one of the three classes of susceptibility (low, moderate and high) defined by the natural break algorithm.
The resulting map identifies the zones at high susceptibility (around 70 km2) mainly localized in the central zone of the study area and involving: (1) the south-west portion dominated by the presence of the volcanic structures of Albano maar lake, Nemi and Ariccia maars, (2) an area of about 3 km2 inside the Faete, (3) some small portions positioned in north-east caldera ring and characterized by the presence of eruptive fractures or scoria cones. The areas at high susceptibility correspond to the 5.5% of the entire study area, whereas the areas at moderate susceptibility (576 km2) involve almost half of the entire study area, in particular the western and southern sectors outside the caldera where the tectonic lineament are present. The obtained susceptibility map appears consistent with the location of the ground deformations and seismic events recorded recently at Colli Albani volcano. The proposed model can also be the basis for the generation of a short-term vent opening map, once monitoring information becomes available. This suggests that the approach proposed in this study can represent a valid starting point for investigating some typologies of hazards related to the long dormant volcanoes where a resumption of the eruptive activity cannot be ruled out and volcanic risk mitigation strategies lacking.
Acknowledgements
We thank Karoly Nemeth and an anonymous reviewer whose suggestions and comments have improved the quality of the manuscript. This research was performed in the project titled ‘Volcanic hazard maps for the Colli Albani caldera based on geo-volcanological data: construction, testing and elaboration’ funded in the framework of MUR-INGV agreement FISR 2019.
Disclosure statement
The authors report there are no competing interests to declare.
Data availability statement
The resulting data presented in the paper are freely available upon request.
References
- Alberico I, Lirer L, Petrosino P, Scandone R. 2008. Volcanic hazard and risk assessment from pyroclastic flows at Ischia island (southern Italy). J Volcanol Geotherm Res. 171(1–2):118–136.
- Amato A, Chiarabba C, Cocco M, di Bona M, Selvaggi G. 1994. The 1989–1990 seismic swarm in the Alban Hills volcanic area, central Italy. J Volcanol Geotherm Res. 61(3–4):225–237.
- Amato A, Chiarabba C. 1995. Recent uplift of the Alban Hills Volcano (Italy): evidence for magmatic inflation? Geophys Res Lett. 22(15):1985–1988.
- Annen C. 2009. From plutons to magma chambers: thermal constraints on the accumulation of eruptible silicic magma in the upper crust. Earth Planet Sci Lett. 284(3–4):409–416.
- Anzidei M, Carapezza ML, Esposito A, Giordano G, Lelli M, Tarchini L. 2008. The Albano Maar Lake high resolution bathymetry and dissolved CO2 budget (Colli Albani volcano, Italy): constrains to hazard evaluation. J Volcanol Geotherm Res. 171(3–4):258–268.
- Anzidei M, Riguzzi F, Stramondo S. 2010. Current geodetic deformation of the Colli Albani volcano: a review. In: The Colli Albani volcano: Special Publication of IAVCEI. Vol. 3. London: Geological Society of London; p. 299–310.
- Anzidei, A., Carboni, G., editors. 2020. Roma prima del mito: abitati e necropoli dal neolitico alla prima eta’ dei metalli nel territorio di roma (VI-III millennio a.C.). Summertown: Archaeopress.
- Bartolini S, Cappello A, Martí J, Del Negro C. 2013. QVAST: a new Quantum GIS plugin for estimating volcanic susceptibility. Nat Hazards Earth Syst Sci. 13(11):3031–3042.
- Bevilacqua A, Isaia R, Neri A, Vitale S, Aspinall WP, Bisson M, Flandoli F, Baxter PT, Bertagnini A, Esposti Ongaro T, et al. 2015. Quantifying volcanic hazard at Campi Flegrei caldera (Italy) with uncertainty assessment: 1. Vent opening maps. J Geophys Res Solid Earth. 120(4):2309–2329.
- Bevilacqua A, Neri A, Bisson M, Esposti Ongaro T, Flandoli F, Isaia R, Rosi M, Vitale S. 2017. The effects of vent location, event scale, and time forecasts on pyroclastic density current hazard maps at Campi Flegrei caldera (Italy). Frontiers Earth Science. 5:72.
- Boari E, Avanzinelli R, Melluso L, Giordano G, Mattei M, De Benedetti AA, Morra V, Conticelli S. 2009. Isotope geochemistry (Sr–Nd–Pb) and petrogenesis of leucite-bearing volcanic rocks from “Colli Albani” volcano, Roman Magmatic Province, Central Italy: inferences on volcano evolution and magma genesis. Bull Volcanol. 71(9):977–1005.
- Burrough PA, McDonnel A, Lloyd CD. 2015. Principles of geographical information systems. Oxford: Oxford University Press.
- Brown SK, Crosweller HS, Sparks RSJ, Cottrell E, Deligne NI, Guerrero NO, Hobbs L, Kiyosugi K, Loughlin SC, Siebert L, et al. 2014. Characterisation of the Quaternary eruption record: analysis of the Large Magnitude Explosive Volcanic Eruptions (LaMEVE) database. J Appl Volcanol. 3(1):1–22.
- Calder E, Wagner K, Ogburn S. 2015. Volcanic hazard maps. In: Global volcanic hazards and risk. Cambridge: Cambridge University Press; p. 335–342.
- Cappello A, Neri M, Acocella V, Gallo G, Vicari A, Del Negro C. 2012. Spatial vent opening probability map of Etna volcano (Sicily, Italy). Bull Volcanol. 74(9):2083–2094.
- Carapezza ML, Barberi F, Tarchini L, Ranaldi M, Ricci T. 2010. Volcanic hazards of the Colli Albani. In: The Colli Albani volcano: Special Publication of IAVCEI. Vol. 3. London: Geological Society of London; p. 279–297.
- Carapezza ML, Barberi F, Tarchini L, Ranaldi M. 2021. Hazard from endogenous gas emissions and phreatic explosions in Rome city (Italy). Geophys Res Lett. 48(5):e2020GL089797.
- Chevrel MO, Favalli M, Villeneuve N, Harris AJ, Fornaciai A, Richter N, Derrien A, Boissier P, Di Muro A, Peltier A. 2021. Lava flow hazard map of Piton de la Fournaise volcano. Nat Hazards Earth Syst Sci. 21(8):2355–2377.
- Chiarabba C, Amato A, Delaney PT. 1997. Crustal structure, evolution, and volcanic unrest of the Alban Hills, Central Italy. Bull Volcanol. 59(3):161–170.
- Chiarabba C, Giordano G, Mattei M, Funiciello R. 2010. The three-dimensional structure of the Colli Albani volcano. London: IAVCEI Special Series; p. 29–39.
- Connor CB, McBirney AR, Furlan C, 2006. What is the probability of explosive eruption at a long-dormant volcano? In: Mader HM, Coles SG, Connor CB, Connor LJ, editors. Statistics in volcanology: Special Publication of IAVCEI. Vol. 1. London: Geological Society of London ; p. 39–46.
- Conticelli S, Boari E, Avanzinelli R, De Benedetti AA, Giordano G, Mattei M, Melluso L, Morra V. 2010. Geochemistry, isotopes and mineral chemistry of the Colli Albani volcanic rocks: constraints on magma genesis and evolution. In: The Colli Albani Volcano: Special Publication of IAVCEI. Vol. 3. London: Geological Society of London; p. 107–139.
- De Benedetti AA, Funiciello R, Giordano G, Diano G, Caprilli E, Paterne M. 2008. Volcanology, history and myths of the Lake Albano maar (Colli Albani volcano, Italy). J Volcanol Geotherm Res. 176(3):387–406.
- De Benedetti AA, Caprilli E, Rossetti F, Giordano G. 2010. Metamorphic, metasomatic and intrusive xenoliths of the Colli Albani volcano and their significance for the reconstruction of the volcano plumbing system. In: R Funiciello, G. Giordano, editors. The Colli Albani volcano. Special Publications of IAVCEI. Vol. 3. London: Geological Society of London ; p. 153–176.
- Diano G, Bonamico A, Giordano G. 2010. Three-dimensional reconstruction of the main unconformities of the Colli Albani stratigraphy and deposit volume calculations. In: The Colli Albani Volcano. Vol. 3. London: Geological Society of London; p. 177–188.
- Faccenna C, Funiciello R, Mattei M. 1994. Late Pleistocene NS shear zones along the Latium Thyrrhenian margin: structural characters and volcanological implications. Boll Geofis Teor ed Appl. 36(141–44):507–522.
- Funiciello R, Giordano G, De Rita D. 2003. The Albano maar lake (Colli Albani Volcano, Italy): recent volcanic activity and evidence of pre-Roman Age catastrophic lahar events. J Volcanol Geotherm Res. 123(1–2):43–61.
- Funiciello R, Heiken G, D, Benedetti AA, Giordano G. 2010. Volcanic activity of the Lake Albano Maar in Roman history. In: The Colli Albani volcano: Special Publication of IAVCEI. Vol. 3. London: Geological Society of London; p. 331.
- Gabrielsen RH, Braathen A, Dehls J, Roberts D. 2002. Tectonic lineaments of Norway. Nor Geol Tidsskr. 82(3):153–174.
- Geshi N, Kusumoto S, Gudmundsson A. 2012. Effects of mechanical layering of host rocks on dike growth and arrest. J Volcanol Geotherm Res. 223–224:74–82.
- Giaccio B, Marra F, Hajdas I, Karner DB, Renne PR, Sposato A. 2009. 40Ar/39Ar and 14C geochronology of the Albano maar deposits: implications for defining the age and eruptive style of the most recent explosive activity at Colli Albani Volcanic District, Central Italy. J Volcanol Geotherm Res. 185(3):203–213.
- Giordano G, De Benedetti AA, Diana A, Diano G, Gaudioso F, Marasco F, Miceli M, Mollo S, Cas RAF, Funiciello R. 2006. The Colli Albani mafic caldera (Roma, Italy): stratigraphy, structure and petrology. J Volcanol Geotherm Res. 155(1-2):49–80.
- Giordano G, De Rita D, Cas R, Rodani S. 2002. Valley pond and ignimbrite veneer deposits in the small-volume phreatomagmatic ‘Peperino Albano’basic ignimbrite, Lago Albano maar, Colli Albani volcano, Italy: influence of topography. J Volcanol Geotherm Res. 118(1-2):131–144.
- Giordano G, De Benedetti AA, Diana A, Diano G, Esposito A, Fabbri M, Gaudioso F, Marasco F, Mazzini I. Miceli V, et al. 2010. Stratigraphy, volcano tectonics and evolution of the Colli Albani volcanic field. In: The Colli Albani volcano: Special Publication of IAVCEI. Vol. 3. London: Geological Society of London; p. 43–47.
- Giordano G, Mattei M, Funiciello R. 2010. Geological map of the Colli Albani volcano. In: The Colli Albani volcano: Special Publication of IAVCEI. Vol. 3. London: Geological Society London.
- Giordano G, Pinton A, Cianfarra P, Baez W, Chiodi A, Viramonte J, Norini G, Groppelli G. 2013. Structural control on geothermal circulation in the Cerro Tuzgle–Tocomar geothermal volcanic area (Puna plateau, Argentina). J Volcanol Geotherm Res. 249:77–94.
- Giordano G, De Benedetti AA, Bonamico A, Ramazzotti P, Mattei M. 2014. Incorporating surface indicators of reservoir permeability into reservoir volume calculations: application to the Colli Albani caldera and the Central Italy Geothermal Province. Earth Sci Rev. 128:75–92.
- Giordano G, Carapezza ML, Monica GD, Todesco M, Tuccimei P, Carlucci G, De Benedetti AA, Gattuso A, Lucchetti C, Piersanti M, et al. 2016. Conditions for long-lasting gas eruptions: the 2013 event at Fiumicino International Airport (Rome, Italy). J Volcanol Geotherm Res. 325:119–134.
- Giordano G, Cas RA. 2021. Classification of ignimbrites and their eruptions. Earth Sci Rev. 220:103697.
- Giordano G, Caricchi L. 2022. Determining the state of activity of transcrustal magmatic systems and their volcanoes. Annu Rev Earth Planet Sci. 50(1):231–259.
- Gudmundsson A. 2012. Magma chambers: formation, local stresses, excess pressures, and compartments. J Volcanol Geotherm Res. 237–238:19–41.
- Jellinek AM, De Paolo DJ. 2003. A model for the origin of large silicic magma chambers: precursors of caldera-forming eruptions. Bull Volcanol. 65(5):363–381.
- Karakas O, Degruyter W, Bachmann O, Dufek J. 2017. Lifetime and size of shallow magma bodies controlled by crustal-scale magmatism. Nature Geosci. 10(6):446–450.
- Lipman PW. 1997. Subsidence of ash-flow calderas: relation to caldera size and magma-chamber geometry. Bull Volcanol. 59(3):198–218.
- Lirer L, Petrosino P, Alberico I. 2010. Hazard and risk assessment in a complex multi-source volcanic area: the example of the Campania Region, Italy. Bull Volcanol. 72(4):411–429.
- Liu P-P, Caricchi L, Chung S-L, Li X-H, Li Q-L, Zhou M-F, Lai Y-M, Ghani AA, Sihotang T, Sheldrake TE, et al. 2021. Growth and thermal maturation of the Toba magma reservoir. Proc Natl Acad Sci USA. 118(45):e2101695118.
- Lucchi F, Peccerillo A, Keller J, Tranne CA, Rossi PL, editors. 2013. The Aeolian islands volcanoes. London: Geological Society of London.
- Marra F, Freda C, Scarlato P, Taddeucci J, Karner DB, Renne PR, Gaeta M, Palladino DM, Trigila R, Cavarretta G. 2003. Post-caldera activity in the Alban Hills volcanic district (Italy): 40Ar/39Ar geochronology and insights into magma evolution. Bull Volcanol. 65(4):227–247.
- Marra F, Gaeta M, Giaccio B, Jicha BR, Palladino DM, Polcari M, Sottili G, Taddeucci J, Florindo F, Stramondo S. 2016. Assessing the volcanic hazard for Rome: 40Ar/39Ar and In‐SAR constraints on the most recent eruptive activity and present‐day uplift at Colli Albani Volcanic District. Geophys Res Lett. 43(13):6898–6906.
- Marra F, Castellano C, Cucci L, Florindo F, Gaeta M, Jicha BR, Palladino DM, Sottili G, Tertulliani A, Tolomei C. 2020. Monti Sabatini and Colli Albani: the dormant twin volcanoes at the gates of Rome. Sci Rep. 10(1):1–17.
- Marrero JM, García A, Berrocoso M, Llinares Á, Rodríguez-Losada A, Ortiz R. 2019. Strategies for the development of volcanic hazard maps in monogenetic volcanic fields: the example of La Palma (Canary Islands). J Appl Volcanol. 8(1):1–21.
- Martí J, Sobradelo R, Felpeto A, García O. 2012. Eruptive scenarios of phonolitic volcanism at Teide–Pico Viejo volcanic complex (Tenerife, Canary Islands). Bull Volcanol. 74(3):767–782.
- Marzocchi W, Sandri L, Selva J. 2008. BET_EF: a probabilistic tool for long-and short-term eruption forecasting. Bull Volcanol. 70(5):623–632.
- Molist JM. 2018. Assessing volcanic hazard: a Review. Oxford Handbooks.
- Neri A, Bevilacqua A, Esposti Ongaro T, Isaia R, Aspinall WP, Bisson M, Flandoli F, Baxter PJ, Bertagnini A, Iannuzzi E, et al. 2015. Quantifying volcanic hazard at Campi Flegrei caldera (Italy) with uncertainty assessment: 2. Pyroclastic density current invasion maps. J Geophys Res Solid Earth. 120(4):2330–2349.
- Pardo N, Macias JL, Giordano G, Cianfarra P, Avellán DR, Bellatreccia F. 2009. The ∼ 1245 yr BP Asososca maar eruption: the youngest event along the Nejapa–Miraflores volcanic fault, Western Managua, Nicaragua. J Volcanol Geotherm Res. 184(3-4):292–312.
- Porreca M, Mattei M, Giordano G, D, Rita D, Funiciello R. 2003. Magnetic fabric and implications for pyroclastic flow and lahar emplacement, Albano maar, Italy. Journal of Geophysical Research Solid Earth. 108(B5)
- Riguzzi F, Pietrantonio G, Devoti R, Atzori S, Anzidei M. 2009. Volcanic unrest of the Colli Albani (central Italy) detected by GPS monitoring test. Phys Earth Planet Inter. 177(1–2):79–87.
- Salvi S, Atzori S, Tolomei C, Allievi J, Ferretti A, Rocca F, Prati C, Stramondo S, Feuillet N. 2004. Inflation rate of the Colli Albani volcanic complex retrieved by the permanent scatterers SAR interferometry technique. Geophys Res Lett. 31(12):n/a–n/a.
- Sbrana A, Toccaceli RM. 2011. Carta Geologica della Regione Campania. - Foglio 464 - Isola di Ischia, Progetto GARG Regione Campania - Assessorato Difesa del Suolo, Litografia Artistica Cartografica, Firenze. Vol. 216; pp + 1 carta: 10.000.
- Scandone R, Bartolini S, Martí J. 2016. A scale for ranking volcanoes by risk. Bull Volcanol. 78(1):1–8.
- Selva J, Orsi G, Di Vito MA, Marzocchi W, Sandri L. 2012. Probability hazard map for future vent opening at the Campi Flegrei caldera, Italy. Bull Volcanol. 74(2):497–510.
- Soligo M, Tuccimei P. 2010. Geochronology of Colli Albani volcano. In: Funiciello R, Giordano G. Editors, The Colli Albani volcano, IAVCEI series. Vol. 3. London: Geological Society of London; p. 99–106.
- Soligo M, Tuccimei P, Giordano G, Funiciello R D, Rita D. 2003. New U-series dating of a carbonate level underlying the Peperino Albano phreatomagmatic ignimbrite (Colli Albani, Italy). Quaternary. 16:115–120.
- Soligo M, Tuccimei P, Funiciello R, Giordano G. 2010. Geochronology of Colli Albano volcano. In: The Colli Albani volcano: Special Publication of IAVCEI. Vol. 3. London: Geological Society of London; p. 99–106.
- Sottili G, Taddeucci J, Palladino DM, Gaeta M, Scarlato P, Ventura G. 2009. Sub-surface dynamics and eruptive styles of maars in the Colli Albani Volcanic District, Central Italy. J Volcanol Geotherm. Res. 180(2–4):189–202.
- Szakács A. 1994. Redefining active volcanoes: a discussion. Bull Volcanol. 56(5):321–325.
- Tadini A, Bevilacqua A, Neri A, Cioni R, Aspinall WP, Bisson M, Isaia R, Mazzarini F, Valentine GA, Vitale S, et al. 2017. Assessing future vent opening locations at the Somma‐Vesuvius volcanic complex: 2. Probability maps of the caldera for a future Plinian/sub‐Plinian event with uncertainty quantification. J Geophys Res Solid Earth. 122(6):4357–4376.
- Tierz P, Stefanescu ER, Sandri L, Sulpizio R, Valentine GA, Marzocchi W, Patra AK. 2018. Towards quantitative volcanic risk of pyroclastic density currents: probabilistic hazard curves and maps around Somma‐Vesuvius (Italy). J Geophys Res Solid Earth. 123(8):6299–6317.
- Trasatti E, Marra F, Polcari M, Etiope G, Ciotoli G, Darrah TH, Tedesco D, Stramondo S, Florindo F, Ventura G. 2018. Coeval uplift and subsidence reveal magma recharging near Rome (Italy). Geochem Geophys Geosyst. 19(5):1484–1498.
- Verolino A, Jenkins SF, Sieh K, Herrin JS, Schonwalder-Angel D, Sihavong V, Oh JH. 2022. Assessing volcanic hazard and exposure to lava flows at remote volcanic fields: a case study from the Bolaven Volcanic Field, Laos. J Appl Volcanol. 11(1):6.
- Vignaroli G, Aldega L, Balsamo F, Billi A, De Benedetti AA, De Filippis L, Giordano G, Rossetti F. 2015. A way to hydrothermal paroxysm, Colli Albani volcano, Italy. Bulletin. 127(5-6):672–687.
- Villa IM, Calanchi I, Dinelli E, Lucchini F. 1999. Age and Evolution of the Albano Crater Lake (Roman Volcanic Province). Acta Vulcanol. 11:305–310.
- Vinkler AP, Cashman K, Giordano G, Groppelli G. 2012. Evolution of the mafic Villa Senni caldera-forming eruption at Colli Albani volcano, Italy, indicated by textural analysis of juvenile fragments. J Volcanol Geotherm Res. 235-236:37–54.
- Voltaggio M, Andretta D, Taddeucci A. 1994. 230Th-238U data in conflict with 40Ar/39Ar leucite ages for quaternary volcanic rocks of the Alban Hills, Italy. Eur J Mineral. 6(2):209–216.
- Watkins SD, Giordano G, Cas RA, De Rita D. 2002. Emplacement processes of the mafic Villa Senni Eruption Unit (VSEU) ignimbrite succession, Colli Albani volcano, Italy. J Volcanol Geotherm Res. 118(1–2):173–203.
- Weber G, Caricchi L, Arce JL, Schmitt AK. 2020. Determining the current size and state of subvolcanic magma reservoirs. Nat Commun. 11(1):1–14.
- Wise DU, Funiciello R, Parotto M, Salvini F. 1985. Topographic lineament swarms: clues to their origin from domain analysis of Italy. Geol Soc America Bull. 96(7):952–967.