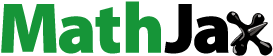
Abstract
In this paper, taking a mine in Xinjiang as the engineering background, through the combination of physical simulation experiment, 3DEC numerical calculation, theoretical analysis and field monitoring, the fracture evolution characteristics of overlying strata and its influence on the stability of support under unbalanced loading conditions are systematically studied. The results show that under the action of gravity-dip effect, the non-uniform filling of gangue leads to the typical cross-layer migration characteristics of overburden caving shape and roof bearing arch. The unstable inclined masonry structure is formed in the middle and upper areas, which is prone to rotation and sliding instability, forming impact pressure. The mining stress of the overlying strata changes significantly due to the mining of the coal seam. The lower the overlying strata, the greater the peak stress, the smaller the stress value in the goaf, and the greater the stress change. The amplitude and instability probability of the support migration increase with the decrease of the working resistance of the support, the increase of the friction between the roof and the support, and the increase of the partial load of the support. Finally, the prevention and control measures of roof caving crushing support in steeply dipping and large mining height working face are put forward.
1. Introduction
The steeply dipping coal seam refers to the coal seam with a buried dip angle of 35°∼ 55°, which is widely distributed in major mining areas in China. The proven reserves are about 180 ∼ 360 billion tons, and the output is about 150 ∼ 300 million tons. More than 50% of the steeply dipping coal seams are high-quality coking coal and anthracite (Wu et al. Citation2020; Wu et al. Citation2023), and most of them are thick and extra-thick coal seams. The full-mechanized mining method can greatly improve the mining efficiency. However, with the increase of mining height, the migration space of surrounding rock increases. The stress state of the support and its interaction with the surrounding rock are more complicated than those of the general mining height and steeply dipping coal seam mining, and it is more difficult to maintain the stability of the support (Wu et al. Citation2014; Wu et al. Citation2017; Xie et al. Citation2016; Luo et al. Citation2023).
In recent years, through theoretical analysis, numerical calculation, physical similar material simulation experiment and field monitoring, many scholars have studied the asymmetric deformation and failure movement law of overlying strata in steeply dipping coal seams (Wang et al. Citation2015; Tu et al. Citation2017; Lang et al. Citation2021; Yang et al. Citation2021; Xie et al. Citation2021), the disaster mechanism of flying gangue in stope space (Wu et al. Citation2021; Hu et al. Citation2021), the distribution characteristics and distribution form of surrounding rock stress (Wu et al. Citation2012; Wang et al. Citation2020; Chi et al. Citation2021; Luo et al. Citation2022b), the stability control mechanism of ‘support-surrounding rock' system (Wang and Jiao Citation2016; Wang et al. Citation2017; Yang et al. Citation2018; Yang et al. Citation2020; Xie et al. Citation2020; Xie et al. Citation2021), the load characteristics of supports (Shi et al. Citation2012; Luo et al. Citation2022a), and the push between supports and its cumulative effect (Wang et al. Citation2015; Yun et al. Citation2016). The judgment conditions for the stability of the support and the calculation method of the stable working resistance of the support are given, which improves the cognition degree of the relationship between the deformation and failure of the overlying strata and the mine pressure behavior in the steeply dipping stope, promotes the continuous progress of the theory and technology of the stability control of the support in the steeply dipping coal seam mining, and lays a foundation for further research in this field. In practical engineering, the roof of the working face with steeply dipping and large mining height will move downward along the inclined direction under the action of self-weight and overlying strata load, which will lead to the rotation of the support, and even lead to the instability of the support and the formation of surrounding rock disaster. The rotation and sliding of this support is inevitable and will not disappear with the increase of the working resistance of the support. At present, there is a lack of in-depth research on this problem.
Therefore, based on the existing research work, this paper takes the steeply dipping and large mining height working face of Xinjiang 2130 coal mine as the engineering background, comprehensively analyzes the deformation, failure and slip filling characteristics of overlying strata through similar material simulation experiments, and analyzes the failure and migration law of overlying strata and its stress path effect through numerical calculation. The mechanical model of support-surrounding rock is constructed to study the influence of roof migration on the load and instability characteristics of support, which provides support for solving the stability control mechanism and prevention and control technology of ' support-surrounding rock ' system in steeply dipping and large mining height coal seam mining.
2. Analysis of deformation and failure of overburden rock
2.1. Engineering background
5 # coal seam is mined in 2130 coal mine of Xinjiang coking coal group. The average dip angle of coal seam is 45°. The designed strike length of working face is 2000 m, and the inclined length is 100 m. The mining method of comprehensive mechanized large mining height is adopted, and the mining height is 4.5 m. According to the special geological conditions of the working face, the rated working resistance of the working face support was finally determined to be 6500 kN, and 60 ZZ6500/22/48 four-column hydraulic support shield supports and 3 ZZG6500/22/48 transition supports were selected. According to the results of field geological survey and rock mechanics test, the characteristics of coal rock in working face are determined, as shown in .
Table 1. Characteristics of coal and rock strata.
Field measurement shows that when the working face is under pressure, the caving roof will cause instantaneous impact on the support. Especially under the condition of hard roof, the working face with steeply dipping and large mining height is prone to large area roof caving impact, which directly causes the working face support toppling down and sliding, and the large-scale roof caving in front of the support. As shown in , the stability control of the support is difficult.
2.2. Physical similar material simulation experiment
In order to study the deformation and failure of overlying strata and the instability mechanism of support-surrounding rock in steeply dipping and large mining height, river sand was selected as aggregate, pulverized coal, gypsum and calcium carbonate were used as cementing materials, the physical model similar material ratio is shown in , and mica was used to simulate stratification. The geometric similarity constant was 20, the bulk density similarity constant was 1.6, and the stress similarity constant was 32, at the top of the model, a hydraulic jack is used to apply a compensation load of 0.6 MPa. The model was paved on a three-dimensional loading test bench. The self-developed hydraulic support model is adopted to install high-precision sensors on the columns, top beams, shield beams, and bases to monitor the columns and lateral loads, as shown in .
Table 2. Ratio of similar materials.
The experimental results show that:
When the working face is in the initial mining stage, the immediate roof falls with the advance of the support, and the falling gangue rolls down along the floor, forming a trapezoidal filling shape behind the support. The immediate roof collapses backward, and in the range of the main roof in the middle and upper part of the tendency, the separated are generated, and the rock layer generated by the cracks does not have the bearing capacity. Therefore, a roof bearing arch is formed, the vault is in the middle and upper part of the inclination, the upper arch foot is on the side of the return airway, and the lower arch foot is on the side of the haulage roadway, see .
As the working face continues to advance, the main roof is broken. Due to the non-uniform filling of gangue, the migration-stacking-hinging characteristics of roof broken rock blocks are complex. Along the dip of the working face, from bottom to top, the formation position of the block structure gradually increases, and its stability and supporting effect on the overlying undamaged rock strata gradually weaken, especially in the middle and upper parts of the dip, forming an empty area, the roof structure is unstable, prone to rotation, sliding instability, forming impact pressure, causing surrounding rock disaster. Affected by the unloading effect of roof failure migration, the stress transfer path of roof strata changes, the bearing arch layer of roof also gradually increases, the stability gradually weakens, the vault evolves to the upper layer, and the arch feet on both sides further migrate to both sides of return airway and haulage roadway, as shown in .
In the process of moving the support, the load of the support is asymmetrically distributed along the inclined direction. The average load of the support in the upper, middle and lower regions is 4676, 5363 and 3873 kN, respectively. The working resistance of the support in the middle and upper regions is much larger than that in the lower region. This is due to the characteristics of steeply dipping and large mining height. With the increase of mining height, there is a large scale space in the middle and upper areas. The amplitude and intensity of surrounding rock migration in the middle and upper areas tend to increase, while the filling density of goaf in the lower area tends to increase, and the working resistance is the smallest, as shown in .
2.3. Numerical calculation
The 3DEC software is suitable for the analysis of the static and dynamic response of discrete medium under load. Considering the study of the variation law of overburden movement and fracture structure, roof stress and displacement of the working face, the discrete element numerical analysis software 3DEC is used to simulate the mining process of the working face, and the ideal elastoplastic constitutive model Mohr-Coulomb yield criterion is used to judge the failure of the rock mass.
The Mohr-Coulomb yield criterion is used to judge the failure of rock mass:
(1)
(1)
In the formula, σ1, σ3 are the maximum and minimum principal stresses respectively, and c, φ are the cohesive force and friction angle respectively.
According to the physical and mechanical parameters of coal and rock mass in working face (), a three-dimensional numerical model () was established by using 3DEC discrete element numerical calculation software, and the model size was 180 m × 200m × 200m. The dip angle of coal seam is 45°, the length of working face is 100 m, the mining height is 4.5 m, the advancing distance is 100 m, and the working face advances along the positive direction of y axis. A vertical load of 2 MPa was applied to the top of the model to simulate the formation depth of 80 m, vertical displacement constraints are applied at the bottom of the model, and horizontal displacement constraints are applied on the front, rear, left and right sides of the model. In order to remove the boundary effect, the boundary coal pillar is set at 50 m. The simulation process is: numerical model establishment → initial ground stress calculation balance → working face mining (working face mining height 4.5 m) → excavation calculation balance → until the end of working face mining (working face has completed the initial weighting and periodic weighting).
After the excavation of coal seam, the direction of principal stress has changed greatly. Affected by the dip angle of coal seam, the deflection trajectory of principal stress direction has obvious asymmetric characteristics, which leads to the formation of asymmetric stress arch in roof strata. The arch foot of the stress arch is in the upper and lower coal bodies of the working face, and the vault is in the middle and upper areas. The load of the overlying strata is transmitted to the upper and lower coal bodies of the working face. In the stress arch, an obvious stress release zone is formed, and the principal stress is obviously reduced, especially in the middle and upper parts of the roof strata, which is prone to fracture, as shown in .
Figure 6. Evolution process of overburden failure tendency. (a) Deflection trajectory of principal stress direction; (b) immediate roof caving; (c) main roof caving.

The movement process of roof strata in the tendency of working face is mainly bending subsidence → separation → fracture collapse → sliding filling. When the working face is in the initial mining stage, the caving gangue slips downward under the action of dip angle-gravity field, and the filling tends to the lower area. The length of the gangue support area is 28 m, the caving height is 6.8 m from the coal seam floor, and the main roof strata in the middle and upper areas have cracks, as shown in .
As the working face continues to advance, the roof of the overlying strata further collapses, and the height of the caving zone is 30 m. The gangue stacking is mainly divided into lower filling compaction, middle complete filling and upper partial filling. The angle between gangue stacking and horizontal plane decreases gradually, which is 42°, 39° and 35°, respectively. Due to the reverse support of the filling gangue and the sliding extrusion of the middle and upper strata, the roof of the lower area is in a stable state, and no fracture instability occurs. In the middle and upper areas, the gangue slides and fills along the dip direction and the lateral direction, forming an inverted triangular free surface on the floor. There is a large scale space, the roof migration is active, and the main roof is broken to form a hinged inclined masonry structure, which is prone to rotation and sliding instability, forming a large degree of impact pressure on the area, and the support is vulnerable to impact, which is not conducive to the stability of the ‘support-surrounding rock' system. The vault of the roof bearing arch is located in the upper part of the working face, and the two sides are asymmetrical. The lower arch foot is located on the side of the haulage roadway, and the upper arch foot is located on the side of the return airway.
is the three-dimensional spatial caving-rolling-sliding-accumulating form of overlying rock. It can be seen from the diagram that the spatial caving form of overlying rock in steeply dipping and large mining height stope has obvious asymmetric characteristics. Under the constraint of three-dimensional non-uniform filling of caving gangue, the maximum deformation of the main roof is located in the middle and upper part of the inclination. The initial caving forms an ellipsoid shape in the middle and upper part of the working face, and the periodic caving presents an irregular superposition ‘crescent' shape. The upper boundary of the roof caving is located in the upper part of the inclined area, and the lower boundary is located in the middle and lower part of the inclined area. The upper and lower boundaries of the broken roof are arc triangles, and there is an empty area phenomenon at the upper end. The main roof has obvious initial fracture and two periodic fractures. It can be seen from the collapse form that when the roof breaks periodically, the length of the inclined lower suspended roof > the upper > the middle.
Figure 7. Strike-failure migration characteristics and stress field of overburden strata in different inclination areas. (a) Spatial caving form of overlying strata; (b) upper area; (c) middle area; (d) lower area; (e) stress field.

In the inclined lower area, the gangue filling in the goaf is full, the roof migration space is limited, the broken main roof rock blocks form a stable hinged structure, and the subsidence and rotation are small. In the middle area, the gangue in the goaf is partially filled, and the cutting rotation is obvious. The front rotation angle is 34°, and the rear rotation angle is 55°. The unstable masonry structure is formed after the main roof is broken. In the inclined upper area, there is a free surface in the floor of the goaf, and the amount of gangue filling is small. The roof migration space in this area is large, which is prone to rotary instability and sliding filling to form an empty area. When the gas content of the mine is high, it is easy to accumulate gas, which seriously affects the safe mining of the working face. The cantilever beam structure appears on one side of the working face, and the caving shape of the overburden rock is asymmetric trapezoid. The front rotation angle is 34°, and the rear rotation angle is 65°.
The caving form of overburden rock and the bearing arch of roof in the lower part of the tendency show symmetrical characteristics, while the caving form of overburden rock and the bearing arch of roof in the middle and upper part of the tendency show asymmetric characteristics. The bearing arch vault is close to the side of the goaf, the front arch foot is located in the coal wall in front of the working face, and the rear arch foot is located in the coal body at the open-off cut.
It can be seen that in the mining of steeply dipping and large mining height, the gangue slides and fills along the dip direction and lateral direction under the action of dip angle-gravity field, and presents the characteristics of non-uniform filling in three-dimensional space, which leads to the typical cross-layer migration and transformation characteristics of overburden caving shape and roof bearing arch. Especially in the middle and upper regions, the phenomenon of staggered and cross-layer stacking is obvious, forming an unstable inclined masonry structure, and there are large-scale empty areas. With the advance of the working face, the inclined masonry structure is prone to rotation and sliding instability, which leads to a large range of strata migration in the dip direction and strike direction of the stope, and forms a large impact dynamic load on the middle and upper supports, coal walls and higher strata, resulting in the phenomenon of variable load of supports, toppling and sliding, squeezing and biting between supports and even deformation and failure of return airway. Therefore, the middle and upper regions are the key areas for strata control in steeply dipping and large mining height stopes. This significant difference in the deformation and failure characteristics of overlying strata in different areas of stope space is essentially caused by the difference in the secondary distribution of mining stress in overlying strata in different areas of stope space under mining disturbance. Therefore, it is particularly important to discover and reveal the stress path effect of overlying strata breaking with steeply dipping and large mining height during mining.
3. Stress path effect analysis of overlying strata fracture
The stress path refers to the trajectory of the stress change process of a certain point in the coal and rock mass in the stress coordinate system under the action of external force. The stress path of the overlying strata in different layers of the working face will directly affect the fracture state and the final failure form of the strata.
In order to analyze the mechanical mechanism of roof strata fracture in working face with steeply dipping and large mining height, when the working face advances 100 m (the working face has completed the initial weighting and periodic weighting), in the middle of the strike (y = 100 m), the dip survey lines are arranged for the immediate roof, main roof and high-level roof above the coal seam. The survey lines are arranged along the length direction of the working face to monitor the three-dimensional mining stress state of the overlying strata. Through the secondary development of the result file by Fish language, the stress value of each measuring point is connected, and the stress path change of the roof rock layer can be obtained. The arrangement of measuring points and monitoring results are shown in . In the figure, sig1 is the maximum principal stress, sig2 is the intermediate principal stress, and sig3 is the minimum principal stress.
Figure 8. The stress path of overlying strata in different layers of inclination. (a) Measuring point position; (b) immediate roof; (c) main roof; (d) high-level roof.

From and , it can be seen that the stress path of overlying strata generally shows that along the inclined direction from the lower end of the coal pillar through the working face to the upper end of the coal pillar, the mining stress first decreases sharply and then begins to fluctuate slightly and then increases sharply. The lower the overburden layer, the greater the influence of mining, the greater the peak stress, but the smaller the stress value of the goaf, that is, the greater the amplitude of stress change, the more complex the stress path trajectory.
The immediate roof is closest to the working face and is most affected by mining. The peak values of stress and principal stress difference at the upper and lower ends are the largest. The immediate roof above the goaf has obvious damage and failure under the action of mining stress. At the 50 m position of the working face (50 R marker point in the figure), the mining stress and the principal stress difference are approximately 0, which is most likely to break, and the block size after failure is small.
The main roof is far away from the working face, and the peak value of stress and principal stress difference is smaller than that of the immediate roof. The mining stress and principal stress difference in the lower part of the working face are large. The mining stress and principal stress difference are small at 60 m of the working face (60 R marker point in the figure), indicating that the main roof strata in this area are seriously damaged and prone to fracture.
The high-level roof is the farthest away from the working face and is the least affected by mining. The peak values of stress and principal stress difference are the smallest, and the damage degree is the weakest. It is not easy to break, and the block size after failure is large, and it is easy to form a bearing structure after breaking.
In summary, the coal seam excavation breaks the equilibrium state of the original rock stress field of the surrounding rock, and the mining stress state of the overlying strata has changed significantly. The stress concentration phenomenon occurs in the strata above the side of the coal pillar at the lower end and the upper end, and the principal stress and the principal stress difference increase. Stress release occurs in the rock strata above the goaf, and the principal stress and the principal stress difference decrease significantly. Affected by the dip angle of the coal seam, the mining stress path of the overlying strata is asymmetrically transmitted along the dip direction. With the increase of the horizon, the overlying strata are seriously damaged in the middle and upper regions. In particular, the main roof strata are prone to fracture, sliding and rotary deformation instability, which has a downward impact on the support in the middle and upper regions, resulting in the support prone to dumping and sliding, and the stability of the support-surrounding rock is greatly reduced.
4. Stability analysis of support-surrounding rock
During the experiment, it was found that the lateral load of the lower support of the working face was relatively stable, and the load of the middle and upper support changed greatly. Compared with the mining of steeply dipping coal seam with general mining height, the lateral load of the support increased significantly, and the dumping range of the support also increased. Affected by the increase of mining space, the amplitude and intensity of surrounding rock migration in the middle and upper parts of the working face tend to increase. Roof migration leads to support dumping and sliding, inter-support extrusion, and support stability control is difficult, and dynamic instability is prone to induce surrounding rock disaster. As shown in and .
From the above analysis, it can be seen that in the mining of steeply dipping and large mining height, it is easy to form an empty area between the support and the roof in the middle and upper part of the working face. When the roof moves, the support will move with the movement of the roof. The direction of the friction FR between the roof and the support is downward along the tendency of the working face, and the support rotates reversely. The force of the lower column of the support is greater than that of the upper column. Especially when the main roof in the middle and upper part of the working face is cut off along the direction of gravity, the impact on the support along the tendency will aggravate the instability of the support, as shown in .
The mechanical model of the support along the inclination under general loading is shown in . In Figure13, α is the dip angle of coal seam, °; φ is the rotation angle of the support around the x-axis, °; a is the width of the support, m; b is the height of the support, m; c is the length of the support base, m; LG is the height of the center of gravity of the support, m; G is the weight of the support, kN; Si-1 and Si+1 are the interaction forces between adjacent supports, kN; P is the normal load of the roof to the support (support working resistance), kN; FR is the tangential load of the roof to the support (friction between the roof and the support), kN; FN is the normal load of the floor to the support, kN; FF is the tangential load of the floor to the support (friction between the floor and the support), kN; y0 is the position of load P, m; y1 is the position of load FN, m; k0 is the foundation coefficient of the floor, kN/m3.
During the migration of the support with the roof, the subsidence of the lower edge (O point) of the support base is zO, and the rotation angle around O point is φ. From the mechanical model shown in , the equilibrium condition of the support along the inclination of the working face under any roof load is
(2)
(2)
(3)
(3)
(4)
(4)
According to the theory of elastic foundation, the normal load FN and its action position y1 of the floor on the support can be expressed as
(5)
(5)
(6)
(6)
The inter-support force ΔSi and the subsidence zO and rotation angle φ of the lower edge of the support base under the load state can be expressed as
(7)
(7)
(8)
(8)
(9)
(9)
Combined with the engineering practice, the coal seam dip angle α = 45°, a is the width of the support, 1.6 m; b is the height of the support, 4.5 m; c is the length of the support base, 3 m; LG is the height of the support center of gravity, b/2m; G is the weight of the support, 15 × 9.8 kN; the friction coefficient between the support and the roof and floor is µ1=µ1=0.3; k0 is the foundation coefficient of the floor, 1.0 × 105 kN/m3.
shows the influence of the roof load on the load and instability of the support when y0 = a/2m, ΔSi = 0. It can be seen from the figure:
Figure 14. Influence of roof load on load and instability of support. (a) Influence of roof load on floor load position y1; (b) influence of roof load on support rotation angle φ.

With the increase of the normal load P of the roof, the load position y1 of the floor gradually increases, the partial load degree of the floor to the support load gradually weakens, and the equivalent concentrated load position gradually moves to the middle of the support base, that is, the stability of the support increases.
With the increase of the tangential load FR of the roof, y1 decreases gradually, the degree of partial load of the floor on the support load increases gradually, the equivalent concentrated load position gradually moves downward to the support base, the stability of the support decreases, and the possibility of instability increases.
With the increase of the normal load (working resistance of the support) P of the roof, the tangential load (friction between the roof and the support) FR that keeps the support from rotating instability also increases.
The rotation angle φ of the support decreases with the increase of the normal load P of the roof.
The rotation angle φ increases nonlinearly with the increase of friction FR, but its growth rate decreases with the increase of working resistance P.
shows the influence of the roof load position on the load and instability of the support when p = 5000 kN , ΔSi = 0. It can be seen from the figure:
Figure 15. Influence of roof load position on load and instability of support. (a) Influence of roof load position y0 on floor load position y1; (b) influence of roof load position y0 on support rotation angle φ.

With the increase of the roof load position y0, the floor load position y1 also increases, the degree of support partial load gradually weakens, and the stability of the support increases.
With the increase of the roof load position y0, the friction FR which keeps the support from rotating instability increases.
The support rotation angle φ decreases with the increase of the roof load position y0.
It can be seen from the above analysis that in the mining of coal seam with steeply dipping and large mining height, the dumping and rotation of the support are mainly caused by the migration of the roof of the working face. The amplitude and instability probability of the support migration increase with the decrease of the working resistance of the support, the increase of the friction between the support and the roof, and the increase of the partial load of the support. Therefore, attention should be paid to the roof condition in the middle and upper parts of the working face to prevent a wide range of empty roofs, resulting in continuous and large-scale dumping of the support.
5. Field monitoring
In order to grasp the law of mine pressure in the mining process of steeply dipping and large mining height working face, the KJ377 mine pressure dynamic detector was used to monitor the working resistance of the support for 4 months. The monitoring results of the support in the upper, middle and lower areas are shown in . When the movement state of the roof changes, the working resistance of the support also changes.
The field monitoring results show that:
Along the inclined direction of the working face, the mine pressure law of the working face shows the characteristics of unbalanced sub-regions. The average working resistance of the supports in the upper, middle and lower parts of the working face is 4985, 6422 and 3505 kN respectively. The maximum working resistance during the weighting period is 6481, 7546 and 5400 kN, and the standard deviation of the working resistance of the supports is 1088.07 kN, 1358.17 kN and 767.12 kN respectively. It can be seen that under the unbalanced load and constraint of the roof, the average working resistance and dispersion degree of the working face support show the basic characteristics of the largest in the middle, the second in the upper and the smallest in the lower part, and the pressure strength in the middle and upper parts is large, and the support is vulnerable to the impact load of the roof.
Due to the low tensile strength of the immediate roof strata, it is easy to be damaged under the action of mining stress after coal seam mining, and the fracture block is small, and the sliding filling is downward along the inclined direction. In the lower area of the tendency, the broken gangue fills the goaf more densely, the roof strata only sink slightly, and the fracture instability is not obvious. Therefore, the mine pressure is not severe, the roof pressure is not obvious, the strength is small, and the step distance is large. The support-surrounding rock system is relatively stable, and the average working resistance of the support and its dispersion are small.
The gangue filling in the middle area is loose and not dense, the migration space of overburden rock is large, the pressure is obvious, the pressure is frequent and the duration is long, and the load of some supports exceeds the rated working resistance (6500 kN) for a long time, which leads to the long-term opening of the safety valve and is not conducive to the safe and normal production of the working face.
There is very little gangue filling in the upper area, and there is a large scale space. The roof has the characteristics of strong pressure, rapid resistance increase, large difference, frequent pressure and short duration. The support changes greatly, and the working resistance of some supports is very small, close to zero, that is, no-load phenomenon, resulting in the lack of roof elements in the ' roof-support-floor (R-S-F) ' system, which is prone to impact collapse of the suspended roof and form impact pressure. At the same time, due to the large range of empty roof, the mutual extrusion between the supports, the support is easy to slide down and fall, and the rotation instability occurs. The load of the ' support-surrounding rock ' system is complex, the average working resistance of the support and its dispersion degree are large, and its instability tendency is much higher than that of the gently inclined coal seam mining.
6. Discussion
Based on the above research on the fracture characteristics of overlying strata on the working face with steeply dipping and large mining height, and the analysis of the stability of support-surrounding rock, combined with the actual production situation of the working face, some ideas are put forward:
The working resistance and initial support force of hydraulic support can be set up in different regions. In practice, by adjusting the output pressure of the hydraulic pump station of the support system and the safety threshold of the support itself, the working resistance of the support in the lower region is set to be 30% ∼ 40% lower than that in the middle region, while that in the upper region is 10% ∼ 20% lower than that in the middle region. The initial support force of the support should reach about 80% of its working resistance to prevent the large-scale empty roof and caving of the roof in the middle and upper regions.
The large working resistance support is selected. The main stress part of the support adopts high-strength plate to meet the strength requirements of the support, and the weight of the support is reduced as much as possible. The semi-unloading sliding advancing of support with pressure is used to realize the coupling contact and load state between the roof and floor of the working face and the support, so as to ensure the stability of the ' support-surrounding rock ' system and reduce the stress of the roof.
The lower area of the working face adopts the ' wedge ' arrangement, which can reduce the height of the lower part of the working face, increase the filling length of the inclined lower part, improve the stress state of the support, reduce the lateral thrust of the inclined basic support to the head support, ensure the load balance of the support system, and eliminate the hidden danger of large-scale impact disaster of the roof, see .
At the end of the working face, multiple supports are connected by cylinders to form an overall anti-dumping and anti-slipping unit, which can not only improve the stability of the support in this range, but also provide a reference for the anti-dumping and anti-slipping of the inclined upper support.
The intelligent mine pressure monitoring instrument is arranged to carry out all-round continuous dynamic monitoring of the mine pressure on the working face, and to predict the roof weighting time, strength and roof caving prone area of the working face. By strengthening the support force of the support, ensuring the stability of the support and strengthening the management, the roof load during the weighting period is effectively controlled, the roof caving disaster is reduced, and the stability of the working face roof is ensured.
At the same time, this study also has some limitations:
The physical simulation three-dimensional test bench is a kind of ' false ' three-dimensional experiment. In the direction of advancing, it can only simulate the advance of short-distance working face, but cannot simulate the advance of long-distance working face. Therefore, the roof breaking characteristics of three-dimensional physical simulation experiments will be the next research goal.
Due to the limitation of monitoring methods, it is difficult to directly monitor the fracture process and evolution characteristics of mining stress paths in different areas of the stope space. Therefore, by monitoring and recording the working resistance of the working face support, the instability and fracture of the overlying strata in different areas of the stope space and the relationship between the support and the surrounding rock are analyzed from the side. Therefore, in the future, more effective on-site monitoring methods are needed to master the three-dimensional overburden fracture morphology, gangue filling, full-cycle support posture evolution, mining stress monitoring and other data in the goaf of the whole space of the working face, so as to provide more solutions for the disaster mechanism of overburden fracture and the stability control of support surrounding rock.
7. Conclusion
In this paper, physical simulation experiment, numerical calculation, theoretical analysis and field monitoring are combined to study the fracture characteristics of overlying strata and the stability analysis of support in steeply dipping and large mining height stope. The results are as follows:
In the mining of steeply dipping and large mining height, influenced by the three-dimensional unbalanced constraint effect of caving gangue, the caving form of overburden rock and the bearing arch of roof show typical cross-layer migration and transformation characteristics. The phenomenon of cross-layer stacking in the middle and upper areas is obvious, forming an unstable inclined masonry structure, and there is a large-scale empty area, which is prone to rotation and sliding instability, forming impact pressure and causing surrounding rock disaster.
The stress path of the overlying strata on the working face generally shows the characteristics that the mining stress first decreases sharply, then fluctuates slightly and then increases sharply. The lower the overburden layer, the greater the stress peak, the smaller the stress value of the goaf, the greater the stress change, the more serious the damage, and the smaller the damage block, the bearing structure cannot be formed.
When the roof moves, the support will move with the movement of the roof. Along the inclined direction of the working face, the working resistance of the support is the largest in the middle (6422 kN), followed by the upper part (4985 kN), and the lowest in the lower part (3505 kN). The upper and middle parts of the working face are prone to no-load, dumping and rotational instability of the support, and the stability control of the support is difficult.
The amplitude and instability probability of support migration increase with the decrease of support working resistance, the increase of friction between roof and support, and the increase of support partial load. When P = 6000 kN, y0 = 1.2 m, the stability of the support is the highest. In the actual mining process, it is necessary to focus on the full-time monitoring of the support in the middle and upper areas of the working face, and adopt the roof tendency partition control method and the ' wedge ' arrangement of the working face, set up the overall anti-dumping and anti-slipping unit, and adopt the measures such as the semi-unloading sliding advancing of support with pressure to ensure the stability of the ' support-surrounding rock ' system.
Acknowledgments
The author thanks the editors and the staff for their efforts. The authors thank the anonymous reviewers for their comments and suggestions to improve the manuscript. The authors acknowledge and appreciate the technical support provided by Coal Mine No. 2130 of Xinjiang Coking Coal (Group) Co., Ltd.
Data availability
Our data are collated from field studies. The numerical parameters are based on the geological data of a coal reservoir in Xinjiang Province, China, which are listed in .
Disclosure statement
No potential conflict of interest was reported by the authors.
Additional information
Funding
References
- Chi XL, Y K, Wei Z. 2021. Breaking and mining-induced stress evolution of overlying strata in the working face of a steeply dipping coal seam. Int J Coal Sci Technol. 8(4):614–625. doi: 10.1007/s40789-020-00392-3.
- Hu BS, Wu YP, Wang HW, Tang YP, Wang CR. 2021. Risk mitigation for rockfall hazards in steeply dipping coal seam: a case study in Xinjiang, northwestern China. Geomatics Nat Hazards Risk. 12(1):988–1014. doi: 10.1080/19475705.2021.1909147.
- Lang D, Wu XB, Wu YP, Lin HF, Luo SH. 2021. Boundary distribution of top-coal limit-equilibrium zone in fully mechanized caving in steeply dipping coal seams. Geomat Nat Hazards Risk. 12(1):2561–2589. doi: 10.1080/19475705.2021.1969450.
- Luo SH, Wang T, Wu YP, Huangfu JY. 2022a. Stability analysis of “support-surrounding rock” system for fully mechanized longwall mining in steeply dipping coal seams. Shock Vib. 2022:1–15. doi: 10.1155/2022/5376718.
- Luo SH, Wang T, Wu YP, Tian CY, Lang D, Zhao HT. 2022b. Space-time evolution characteristics of stress transfer path of surrounding rock in longwall mining of steeply dipping seam. J Chin Coal Soc. 47(07):2534–2545. doi: 10.13225/j.cnki.jccs.2021.1134.
- Luo SH, Wang T, Wu YP, Xie PS, Shi H. 2023. Experiment on space-time evolution characteristics of deformation and failure of overlying strata in longwall mining of steeply dipping coal seam. Energy Explor Exploit. 41(2):656–676. doi: 10.1177/01445987221136886.
- Shi JJ, Shi HY, Bao SS, Liu HW. 2012. The hydraulic support parameter design in lean coal seam and numerical simulation about the relation hydraulic support and surround rock. J Chin Coal Soc. 37(S2):313–318. doi: 10.13225/j.cnki.jccs.2012.s2.037.
- Tu HS, Tu SH, Zhang C, Zhang L, Zhang XG. 2017. Characteristics of the roof behaviors and mine pressure manifestation during the mining of steep coal seam. Arch Min Sci. 62(4):871–891. doi: 10.1515/amsc-2017-0060.
- Wang JA, Jiao JL. 2016. Criteria of support stability in mining of steeply inclined thick coal seam. Int J Rock Mech Min Sci. 82:22–35. doi: 10.1016/j.ijrmms.2015.11.008.
- Wang JA, Jiao JL, Cheng WD, Yun DF, Xie JW, Shangguan KF. 2015. Roles of arc segment in controlling the support stability in longwall fully mechanized top coal caving mining face of steeply inclined coal seam. J Chin Coal Soc. 40(10):2361–2369. doi: 10.13225/j.cnki.jccs.2015.0036.
- Wang JC, Wei WJ, Zhang JW, Xie F. 2017. Stability analysis of support around the longwall top-coal caving mining in steeply thick coal seam. J Chin Coal Soc. 42(11):2783–2791. doi: 10.13225/j.cnki.jccs.2017.0914.
- Wang HW, Wu YP, Liu MF, Jiao JQ, Luo SH. 2020. Roof-breaking mechanism and stress-evolution characteristics in partial backfill mining of steeply inclined seams. Geomat Nat Hazards Risk. 11(1):2006–2035. doi: 10.1080/19475705.2020.1823491.
- Wang JA, Zhang JW, Gao XM, Wen JD, Gu YD. 2015. Fracture mode and evolution of main roof stratum above longwall fully mechanized top coal caving in steeply inclined thick coal seam (I)—initial fracture. J Chin Coal Soc. 40(6):1353–1360. doi: 10.13225/j.cnki.jccs.2015.0407.
- Wu YP, Hu BS, Lang D, Tang YP. 2021. Risk assessment approach for rockfall hazards in steeply dipping coal seams. Int J Rock Mech Min Sci. 138:104626. doi: 10.1016/j.ijrmms.2021.104626.
- Wu YP, Liu KZ, Yun DF, Xie PS, Wang HW. 2014. Research progress on the safe and efficient mining technology of steeply dipping seam. J Chin Coal Soc. 39(8):1611–1618. doi: 10.13225/j.cnki.jccs.2014.9039.
- Wu YP, Wang HW, Xie PS. 2012. Analysis of surrounding rock macro stress arch-shell of longwall face in steeply dipping seam mining. J Chin Coal Soc. 37(4):559–564. doi: 10.13225/j.cnki.jccs.2012.04.014.
- Wu YP, Xie PS, Yun DF, Wang HW, Luo SH, Gao XC, Lang D, Hu BS, Yan ZZ, Wang T. 2023. Gravity-dip effect and strata control in mining of the steeply dipping coal seam. J Chin Coal Soc. 48(01):100–113. doi: 10.13225/j.cnki.jccs.2022.0648.
- Wu YP, Yun DF, Xie PS, Wang HW, Lang D, Hu BS. 2020. Progress, practice and scientific issues in steeply dipping coal seams fully-mechanized mining. J Chin Coal Soc. 45(01):24–34. doi: 10.13225/j.cnki.jccs.YG19.0494.
- Wu YP, Yun DF, Xie PS, Wang HW, Luo SH. 2017. Theory and technology of fully mechanized longwall mining in steeply inclined seam. Beijing: Science Press.
- Xie PS, Wu YP, Zhang H, Liu ZK, Wang HW. 2016. Changeful load feature analysis of support in longwall and large mining height fully-mechanized face if steeply dipping seam. Coal Sci Technol. 44(4):101–105. doi: 10.13199/j.cnki.cst.2016.04.020.
- Xie PS, Zhang YY, Luo SH, Duan JJ. 2020. Instability mechanism of a multi-layer gangue roof and determination of support resistance under inclination and gravity. Min Metall Explor. 37(5):1487–1498. doi: 10.1007/s42461-020-00252-3.
- Xie PS, Zhang YY, Zhang YL, Chen JJ, Zhang XB. 2021. Instability law of the coal-rock interbedded roof and its influence on supports in large mining height working face with steeply dipping coal seam. J Chin Coal Soc. 46(02):344–356. doi: 10.13225/j.cnki.jccs.xr20.1866.
- Yang K, Chi XL, Liu S. 2018. Instability mechanism and control of hydraulic support in fully mechanized longwall mining with large dip. J Chin Coal Soc. 43(07):1821–1828. doi: 10.13225/j.cnki.jccs.2017.0949.
- Yang K, Chi XL, Liu QJ, Liu WJ, Liu S. 2020. Cataclastic regenerated roof and instability mechanism of support in fully mechanized mining face of steeply dipping seam. J Chin Coal Soc. 45(09):3045–3053. doi: 10.13225/j.cnki.jccs.2019.0854.
- Yang K, Wei Z, Chi XL, Gao AS, Fu Q. 2021. Fracture criterion of basic roof deformation in fully mechanized mining with large dip angle. Energy Explor Exploit. 39(3):886–902. doi: 10.1177/0144598720986628.
- Yun DF, Liu Z, Cheng WD, Fan ZD, Wang DF, Su PZ, Meng XJ, Zhang YH. 2016. Study on loading monitoring of side guard plate powered support of fully mechanized top coal caving mining in high inclined thick seam. Coal Sci Technol. 44(9):145–149. doi: 10.13199/j.cnki.cst.2016.09.026.