ABSTRACT
Helicobacter pylori (H. pylori) is the main cause of gastric adenocarcinoma. However, the traditional antibiotic treatment of H. pylori is limited due to increased antibiotic resistance and low efficacy; low drug delivery efficiency and difficulties in eradicating H. pylori that is present intracellularly or in biofilms cause further setbacks. Biomaterials that can protect drugs against stomach acid, target lesions, control drug release, destroy biofilms, and exhibit unique antibacterial mechanisms and excellent biocompatibility have emerged as attractive tools for H. pylori eradication, particularly for drug-resistant strains. Herein, we review the virulence mechanisms, current drug treatments, and antibiotic resistance of H. pylori strains. Furthermore, recent advances in the development of biomaterials, including nanoparticles (such as lipid-based nanoparticles, polymeric nanoparticles, and inorganic nanoparticles), microspheres, and hydrogels, for effective and precise therapy of H. pylori and different types of therapeutic mechanisms, as well as future perspectives, have also been summarized.
Introduction
Helicobacter pylori (H. pylori), a gram-negative spiral-shaped bacterium that colonizes the gastric mucus and gastric epithelium, has been estimated to infect nearly 4.4 billion people worldwide.Citation1 It was reported that H. pylori is usually acquired before the age of 10, and by inducing inflammation of the host in the gastric epithelium, H. pylori can cause a series of gastric diseases, including peptic ulcer disease, gastric adenocarcinoma, atrophic gastritis, and mucosa-associated lymphoid tissue lymphoma.Citation2–6 Additionally, H. pylori is closely associated with iron deficiency anemia, idiopathic thrombocytopenic purpura, and vitamin B12 deficiency.Citation7–9 In 2015, the Kyoto Global Consensus Report formally defined H. pylori as an infectious disease and recommended treatment for all patients with it because it plays an important role in the development of gastric adenocarcinoma.Citation10 In addition, a nationwide multicenter study has also indicated H. pylori infection to be a high-risk factor for gastric cancer.Citation6 An updated meta-analysis of randomized controlled trials indicated that its eradication could reduce the incidence and mortality of gastric cancer by 46% and 39%, respectively.Citation11 Furthermore, recent guidelines recommend the treatment of individuals infected with H. pylori to prevent gastric cancer.Citation2,Citation3,Citation12 Therefore, the eradication of H. pylori from the global population is of great significance.
Currently, regimens for H. pylori eradication are based mainly on pharmacological treatments that include antibiotics.Citation3 Early experiments have indicated a high susceptibility of H. pylori to antibiotics in vitro; however, it is difficult to achieve similar effects in vivo.Citation13 This could be attributed to the acidic environment of the stomach (which weakens the bactericidal effects of antibiotics) and the thick gastric mucosa (which makes it difficult for most antibiotics to reach the target effectively).Citation13 Therefore, treatments to efficiently eradicate H. pylori, such as clarithromycin triple therapy and bismuth quadruple therapy, involve the simultaneous administration of several drugs for long periods (usually 7–14 days).Citation3 However, complex drug eradication programs are associated with several limitations, including large pill burdens, adverse effects, and high cost,Citation14 which cause reduced adherence and low cure rates. Additionally, the progressive development of antimicrobial resistance due to the widespread misuse of antibiotics seriously undermines treatment efficacy.Citation14,Citation15 Moreover, persistent and slow-growing infections enable H. pylori to form biofilms or survive inside epithelial cells and macrophages by regulating autophagy, making their eradication by antibiotics extremely challenging.Citation16 Therefore, new treatment strategies that can overcome the acidic gastric environment, increase drug delivery efficiency, and eradicate H. pylori that exists intracellularly or in biofilms are urgently needed for effective alternatives.
Biomaterials formed by synthetic material engineering exhibit several important applications in cancer treatment, antibacterial, immunomodulatory, wound healing processes, and so on.Citation17–20 Biomaterials have also been used for the treatment of H. pylori infection due to their unique properties compared to conventional antibiotic therapy. First, biomaterials can conjugate with or encapsulate conventional antibiotics, which can reduce the administered dose and side effects and enhance bioavailability. In addition, the site-specific targeting of H. pylori and controlled drug release by biomaterials also improve antibacterial efficacy and slow down the emergence of drug-resistant bacteria.Citation21 Additionally, biomaterials (such as inorganic nanoparticles) can enter bacterial cells directly and destroy biofilms, eliminating the H. pylori present there.Citation22,Citation23 Some biomaterials (such as linolenic acid and metal ions) exhibit unique antibacterial mechanisms (they affect the metabolic activities of H. pylori, change its membrane permeability or produce reactive oxygen species (ROS)) and can eradicate H. pylori without any loaded drugs; they hinder the development of drug resistance in H. pylori.Citation24–26 Currently, new H. pylori treatment strategies, including Chinese herbal medicines, probiotics, antimicrobial peptides, and urease inhibitors, are also being studied by researchers to eradicate H. pylori. However, compared with biomaterials, these strategies are either cytotoxic, intolerant to gastric acid and pepsin, or have a lower eradication rate.Citation27–30 Therefore, biomaterials are effective alternatives for efficient H. pylori therapy; liposomes, nanostructured lipid carriers, nanoemulsions, polymeric nanoparticles, inorganic nanoparticles, microspheres, and hydrogels are commonly used.Citation31–33
Herein, the pathogenic mechanisms of H. pylori infection have been described, along with its current drug treatments and drug-resistance mechanisms. Furthermore, recent progress in biomaterials for H. pylori eradication has been summarized. Thus, biomaterials can be used for the effective and precise therapy of H. pylori infections in the future.
H. pylori
H. pylori and its virulence mechanisms
Isolated and cultured by Warren and Marshall for the first time, H. pylori is a spiral microaerobic bacterium with five to seven flagella and demanding growth conditions.Citation34 H. pylori infection will first cause chronic gastritis and then gradually lead to gastric ulcers and gastric atrophy;Citation35 in severe cases, it can lead to gastric cancer.Citation36 It has been reported that there is a parallel relationship between H. pylori and gastric cancer mortality, and eradication of H. pylori is closely related to the prevention of gastric cancer.Citation6,Citation37,Citation38
The pathogenesis of H. pylori infection is shown in . H. pylori produces urease upon entering the stomach, which promotes the hydrolysis of urea, forming ammonia and carbon dioxide. Ammonia neutralizes gastric acid, while carbon dioxide helps bacteria survive in the stomach by resisting gastric acidity. In this way, the bacteria can resist gastric acidity and survive in the stomach. H. pylori, with the help of its helical shape and flagellar movement, easily penetrates the mucus layer to reach the gastric epithelium,Citation39 where it colonizes gastric epithelial cells under the synergistic action of adhesins and outer membrane proteins (such as BabA, SabA, LabA, OipA, AlpA/AlpB, HopQ, HopZ).Citation40–42 Then, the proteins and toxins located in the host cells subsequently cause an inflammatory response.Citation43
Figure 1. Schematic representation of H. pylori pathogenesis and virulence factors. Upon entering the stomach, H. pylori begins to produce urease, which promotes the hydrolysis of urea to synthesize gastric acid. In this way, bacteria can resist gastric acidity and survive in the stomach. With the help of its helical shape and flagellum movement, H. pylori can easily penetrate the mucus layer and reach the gastric epithelium. After reaching the gastric epithelium, H. pylori colonizes gastric epithelial cells under the synergistic action of a variety of adhesins and outer membrane proteins (e.g., BabA, SabA, LabA, OipA, AlpA/AlpB, HopQ, and HopZ). Toxins (e.g., Cag A) are then injected into the host cells through the T4SS, leading to an inflammatory response. In addition, H. pylori can form biofilms composed of bacteria and a self-secreted extracellular matrix that adheres to inert and living surfaces; growth in biofilms facilitates the development of antibiotic resistance in H. pylori.
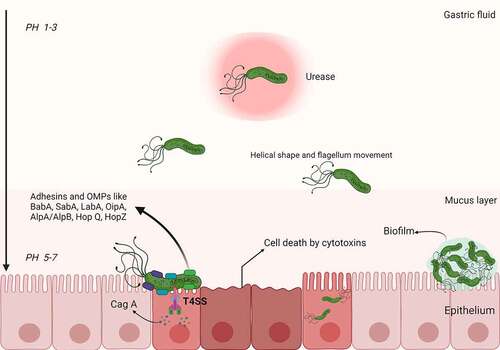
Among the many pathogenic factors of H. pylori, cytotoxin-associated gene A (cagA) and vacuolating cytotoxin A (VacA) are the most extensively investigated virulence factors. Cytotoxin-associated protein A (CagA) is a protein produced by H. pylori, with masses in the range of 120–145 kDa. CagA, along with the type 4 secretion system (T4SS), is encoded by the cag pathogenicity island (cagPAI), which comprises approximately 40 kb of chromosomal DNA.Citation44 According to the presence and absence of CagA, H. pylori can be classified into two subtypes, CagA-positive and CagA-negative. After attachment to gastric cells, the CagA-positive H. pylori injects CagA into the host cells through the T4SS, similar to a needle. Compared to those infected with CagA-negative H. pylori strains, patients with a CagA-positive H. pylori infection have a higher risk of gastric cancer or peptic ulcer disease.Citation45 Xie et al. revealed autophagy was decreased in the duration of H. pylori infection in a CagA-dependent manner, resulted in ubiquitination degradation of RAD51 and genomic instability in gastric cells, which inducing progression of gastric epithelial cells to cancer.Citation45 VacA is a cytotoxin encoded by the vagA gene; its main function is cell vacuolation through pores on the epithelial cell membrane. Additionally, it helps H. pylori resist immune clearance, improves its viability, and enables the host to continue infecting through membrane depolarization, apoptosis stimulation, intercellular adhesion, and T-cell obstruction.Citation40 VacA also interferes with autophagy, enabling the survival of H. pylori in host cells.Citation46
Current drug treatments and antibiotic resistance of H. pylori
The current regimens for H. pylori treatment integrate one PPI with a combination of antimicrobial agents (). According to the Maastricht V/Florence consensus report, in areas of low (<15%) clarithromycin resistance, the standard PPI-clarithromycin-containing regimen is recommended as the first-line treatment, whereas the bismuth-quadruple regimen is recommended in areas of high (>15%) clarithromycin resistance.Citation3 After failure of the first-line treatment (clarithromycin-containing triple therapy or bismuth quadruple therapy), fluoroquinolone-containing (for example, levofloxacin) triple or quadruple therapy is recommended as the second-line treatment. After failure, the patient is recommended to undergo susceptibility testing or molecular determination to guide further treatment.Citation3 In addition to conventional regimens, researchers have modified drug-administration sequences and created sequential, hybrid, and concomitant therapies. However, due to resistance to clarithromycin and metronidazole, sequential therapy gradually fades out of the field of research.Citation12,Citation49 Although hybrid and concomitant therapies exhibit satisfactory H. pylori eradication rates,Citation50,Citation51 they involve complex drug administration, which may affect patient compliance, and the exposure of patients to at least one unnecessary antibiotic, which may promote H. pylori resistance. Thus, the promotion and application of these regimens should be investigated further.Citation49,Citation52 Graham et al. reported a rifabutin-based triple therapy randomized controlled study and indicated that the regimen is unaffected by clarithromycin or metronidazole resistance.Citation53 However, this study excludes individuals of Asian descent; therefore, the applicability of rifabutin-based triple therapy to Asian people, who have a high risk of stomach cancer, remains unknown.Citation53 High-dose PPI-amoxicillin dual therapy and vonoprazan (a new potent acid-inhibitory drug) triple or dual therapy are popular new treatment methods. Numerous studies have confirmed the high H. pylori eradication rates and low side effects of both methods.Citation48,Citation54–56 Interestingly, Horii et al. reported that vonoprazan dual therapy has a low impact on gut microbiota.Citation57 However, vonoprazan is expensive; thus, economic aspects should be considered when using it for treatment. Additionally, probiotics have been poven to improve the eradication effect.Citation58 A study recruiting 234 H. pylori-positive gastritis patients from seven local centers indicated that probiotic administration before or after standard triple therapy improves H. pylori eradication rates.Citation58 In addition, to reduce the chance of reinfection after H. pylori eradication, the strategy of “family-based H. pylori management” could be vital.Citation59
Table 1. The current regimens for H. pylori treatment.
However, rapidly increasing antibiotic resistance has reduced the efficacy of traditional antibiotic therapy against H. pylori.Citation60 At present, the drug resistance of H. pylori mainly refers to resistance against antibiotics used in eradication therapies (such as clarithromycin, fluoroquinolones, metronidazole, amoxicillin, tetracycline, and rifabutin). The mechanism of antibiotic resistance primarily involves structural changes in the gene sequence, the destruction of antibiotic activity by changing drug targets, and the inhibition of intracellular drug activation, affecting drug-efflux mechanisms and causing enzymatic deactivation of the drug ().Citation15 In addition, the acidic environment of the stomach and the presence of thick gastric mucosa (approximately 200 μm) also affect the efficacy of antibiotic delivery, which reduces the cure rate.Citation15 Furthermore, H. pylori can form biofilms composed of bacteria and a self-secreted extracellular matrix that adhere to inert or living surfaces.Citation61 Biofilms play an imperative role in antibiotic resistance by reducing drug penetration, promoting gene mutation, and overexpressing efflux pumps involved in drug resistance.Citation15 The matrix includes an effective and nonspecific barrier, which prevents drug penetration and promotes the overexpression of efflux pumps in the process of drug resistance; thus, encapsulating H. pylori can significantly enhance its viability. Compared to free-floating strains, H. pylori growth in biofilms may occur for a longer time, with a greater susceptibility to developing antibiotic resistance.Citation62,Citation63 Given the aforementioned reasons, investigating alternatives to increase drug-delivery efficiency and eradicate H. pylori present intracellularly or in biofilms may be the key to resolving several issues related to its rapidly increasing drug resistance.
Figure 2. Biological attributes of resistance in H. pylori. The figure shows the biological attributes that drive antibiotic resistance in the H. pylori species (red stars denote the possibility of resistance mutation). It comprises mainly structural changes in genetic sequences that disrupt the cellular activity of antibiotics by altering the drug target (1–3), inhibiting drug activation within the cells (4), biofilm formation (consisting of a matrix of polysaccharides that constitute a multifactorial barrier to drug penetration and activity) (5), reduced drug uptake and increased drug efflux (6), or coccoid formation that generates ultrastructural and metabolic changes that prevent cellular penetration and activity of antibiotic molecules (7). All these attributes are not mutually exclusive but possibly coexist in different strains, jointly leading to three patterns of resistance: single drug resistance (SDR), multidrug resistance (MDR), and heteroresistance (HR). Reproduced with permission from ref,Citation15 © Tshibangu-Kabamba E (2021).
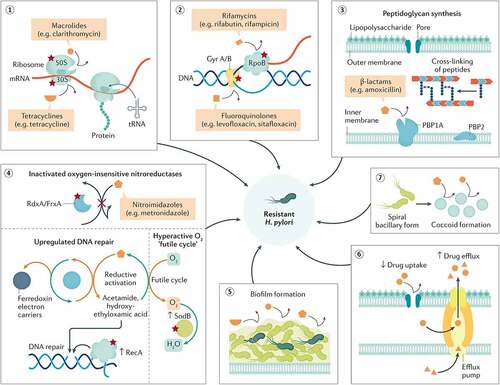
Biomaterials
Biomaterials possess many unique properties, such as a large specific surface area, targeted lesion sites, satisfactory biocompatibility, and excellent stability;Citation64 their application in disease treatment is an important aspect of modern medicine. Owing to their unique antibacterial mechanisms and efficiency in eradicating drug-resistant strains, biomaterials are gradually being used in H. pylori treatment. Currently, biomaterials are mainly used as delivery systems for drugs to eradicate H. pylori, which can increase the drug delivery efficiency. Apart from encapsulating antibacterial agents, biomaterials (such as lipid nanoparticles, chitosan nanoparticles, and inorganic nanoparticles) have been directly applied in H. pylori treatments because of their inherent antibacterial activity. Herein, recent studies on the progress of biomaterials in the treatment of H. pylori infection have been described and summarized.
Nanoparticles
Nanoparticles, defined as nanosized delivery systems that encapsulate drugs, can increase drug solubility and modulate drug-release characteristics and are valuable tools in fighting antibiotic resistance.Citation21 Based on the matrix material, nanoparticles have been classified into several categories, such as lipid-based nanoparticles (including liposomes, nanostructured lipid carriers, and nanoemulsions), polymeric nanoparticles, and inorganic nanoparticles.
Lipid-based nanoparticles
Lipid-based nanoparticles (including liposomes, solid lipid nanoparticles, nanostructured lipid carriers, and nanoemulsions) are among the most widely used drug delivery systems for antimicrobial therapy.Citation65,Citation66 With compositional similarity to cell membranes, lipid-based nanoparticles exhibit high biocompatibility and low toxicity.Citation67,Citation68 Combined with other materials, they can present excellent features, such as target sites and controlled drug release, enabling a high drug-delivery efficiency and low occurrence of antibiotic resistance in H. pylori.Citation69 Notably, lipid-based nanoparticles (such as liposomes) can enter cells and kill H. pylori inside epithelial cells, macrophages, and biofilms.Citation70 In this section, diverse lipid-based nanoparticles (mainly including liposomes and nanostructured lipid carriers) used for the eradication of H. pylori have been outlined, focusing on their stability, cargo transportation, and antimicrobial activity.
Liposomes
Liposomes are defined as smooth, continuous, and bilayered structures that contain mainly phospholipid molecules.Citation71 As delivery systems, they exhibit numerous advantageous properties. First, they are composed of phospholipid bilayers encapsulating water-phase vesicles, and liposomes have high elasticity and good biocompatibility. Additionally, they exhibit high drug-loading and entrapment capacity as the water-soluble and fat-soluble drugs are dissolved in the water phase and lipid membrane, respectively. Furthermore, phospholipids themselves are cell-membrane components; thus, liposomes are nontoxic with high bioavailability.Citation21,Citation72,Citation73 As one of the most widely investigated antibiotic delivery systems, liposomes are often used to deliver antibiotics (such as amoxicillin, furazolidone, doxycycline, and metronidazole) in H. pylori treatments.Citation74–77 In particular, liposomes are ideal vehicles for delivering antimicrobial lipids (such as lauric acid, myristoleic acid, linoleic acid, and linolenic acid (LLA)), making it difficult for H. pylori to develop resistance. For example, Obonyo et al. designed a system named liposomal linolenic acid (LipoLLA) by incorporating lauric acid, myristoleic acid, linoleic acid, and LLA, which solves the problem of poor solubility of LLA in aqueous solutions ().Citation24 After incubation with LipoLLA at a concentration of 67 μg/mL for 30 min, 99.9% of H. pylori were killed. Notably, LipoLLA can also kill the dormant form (the coccoid form) of H. pylori by damaging its membrane ().Citation81 Additionally, H. pylori treated with LipoLLA did not acquire drug resistance during the 10-day period, while drug resistance to LLA was established on day 3.Citation24 Apart from delivering anti-H. pylori drugs alone, liposomes have also been integrated with other materials (such as pectin and monoclonal antibodies against H. pylori) to exhibit target specificity and antiadhesion.Citation76,Citation82 For example, Gottesmann et al. reported the fabrication of pectin-coated liposomes with encapsulated amoxicillin that utilize a specific interaction of pectin with mucins (BabA) and the surface structures of H. pylori.Citation76 Their results showed that pectin-coated liposomes exhibit stronger bactericidal effects than nonpectin-coated liposomes.Citation76
Figure 3. Lipid-based nanoparticles used for H. pylori therapy. a) The schematic drawing shows the molecular structure of LipoLLA, which is composed of phospholipids, cholesterol, and LLA. b) Morphologies of H. pylori SS1 bacteria in the spiral and coccoid forms exposed to different treatments. c) The strategy for lipid polymer nanoparticles to eradicate bacterial biofilms and the eradication of H. pylori plankton in biofilms by nanoparticles. Blank: the blank group with only bacteria; RHL-PC-LPN: amoxicillin-loaded lipid polymer nanoparticles; LPN: using rhamnolipid and phospholipids as mixed lipids; PC-LPN: amoxicillin-loaded LPN: only using phospholipids as the lipid component; PECS + AMX: a mixture of sulfated pectin and amoxicillin; AMX: amoxicillin only; d) The in vitro and in vivo release of NE-P22; a. In vitro release profile of NE-P22; b. In vivo fluorescence imaging of FITC-labeled P22 in mice; c. Quantification of fluorescence intensity; e) Nanostructured lipid carriers kill H. pylori by changing cell-membrane permeability at low concentrations, even without any loaded drug; the eradication does not affect other gut microbiota (such as L. casei and E. coli). (a) and (b) Reproduced with permission from ref,Citation24 © 2012, American Chemical Society; (c) Reproduced with permission from ref,Citation78 © 2015 Elsevier B.V. (d) Reproduced with permission from ref,Citation79 © 2019, The Author(s); (e) Reproduced with permission from ref,Citation80 © 2018 Elsevier B.V.
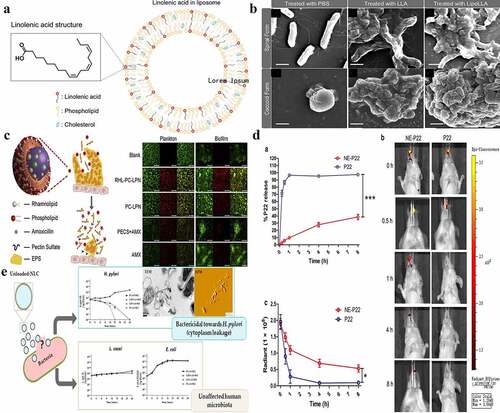
However, there are some challenges associated with the use of liposomes to eradicate H. pylori. First, liposomes alone exhibit low encapsulation efficiencies for antibiotics,Citation83,Citation84 which may be because liposomes are too unstable for gastric antibiotic delivery.Citation32 Adding cholesterol to liposomal formulations can improve liposome stability and increase the interactions between liposomes and H. pylori.Citation76 However, excessive cholesterol may disrupt the linear structure of liposomal membranes, decreasing the encapsulation efficiency and drug release rate.Citation74 Thus, selecting an appropriate proportion of cholesterol is vital for generating stable liposomes. Alam et al. investigated the effects of different cholesterol amounts on the encapsulation efficiency and drug release rate of different liposome formulations. The formulation containing 5 mg of furazolidone, 53 mg of cholesterol, and 106 mg of lipid exhibits optimal encapsulation and drug release efficacy.Citation74 Combining liposomes with polymers is another helpful way to improve their stability. For example, Cai et al. reported amoxicillin-loaded liposome-polymer hybrid nanoparticles with high stability in the acidic environment of the stomach.Citation78 Interestingly, the addition of rhamnolipids imparted a strong biofilm-disrupting capacity to the nanoparticles ().Citation78 Second, the complex manufacturing processes of liposomes increase their cost and lower their production, limiting their use in routine clinical applications.Citation85 Hence, it is important to develop a facile and economical liposome manufacturing process.
In summary, the anti-H. pylori mechanisms of liposomes exhibit the following features. First, they can protect drugs from stomach acid degradation and enhance their solubility. Second, they can increase membrane permeability and alter the membrane structure of H. pylori. However, the use of liposomes is limited by their complex preparation processes.
Nanoemulsions
Nanoemulsions are kinetically stable colloidal-particulate delivery systems formed by the dispersion of two immiscible liquids (such as oil and water) that are stabilized by surfactants and typically exhibit sizes in the range of 20–200 nm.Citation66 Generally, nanoemulsions are classified into three types: oil-in-water, water-in-oil, and bicontinuous.Citation86 Unlike liposomes, nanoemulsions not only exhibit high biocompatibility but also present long-term stability and are fabricated via simple processes (unlike liposomes), enabling their large-scale production and long-term preservation.Citation66 In regard to antimicrobials, nanoemulsions exhibit antibacterial activity and can destroy bacteria without the addition of any antimicrobial substances.Citation86 Furthermore, they improve the stability, solubility, and bioavailability of loaded (especially lipophilic) drugs.Citation86,Citation87 For instance, Tran et al. developed a nanoemulsion-based delivery system loaded with erythromycin, which exhibits low solubility in water and is easily destroyed in acidic environments, for the eradication of H. pylori. Their results showed that the stability of erythromycin was significantly enhanced by the nanoemulsion system.Citation87 Additionally, nanoemulsions exhibit effective adjuvant activity for vaccines. Yang et al. designed an intranasal HpaA epitope peptide-loaded nanoemulsion delivery system to protect against H. pylori infection.Citation79 They found that this system could extend antigen release and induce Th1 responses, which indicated that nanoemulsions are an ideal delivery system for intranasal vaccines against H. pylori ().Citation79 However, although nanoemulsions are widely used for combating bacterial growth and are easy to produce/preserve,Citation86 there are very few studies on the eradication of H. pylori using them. Therefore, the applicability of nanoemulsions as effective alternatives for H. pylori therapy requires further investigation.
Nanostructured lipid carriers
Nanostructured lipid carriers are the second generation of solid lipid nanoparticles produced from solid and liquid lipids.Citation68 They can be grouped into three classes (imperfect, amorphous, and multiple oil-in-solid fat-in-water) according to their mixture composition and fabrication method.Citation88 Nanostructured lipid carriers exhibit good biocompatibility and stability and can lead to high storage capacities and encapsulation efficiencies.Citation21,Citation88 For example, Seabra et al. fabricated docosahexaenoic acid (DHA)-loaded nanostructured lipid carriers that inhibit H. pylori growth at a very low concentration (25 mM), indicating that the nanoparticles effectively protect the properties of DHA. In addition, they also found that the antibacterial mechanism of DHA against H. pylori primarily involved the destruction of the bacterial cell membrane.Citation89 Interestingly, in a later study by Seabra et al., they found that nanostructured lipid carriers without any loaded drugs can eradicate H. pylori, even at very low concentrations.Citation80 To explore the underlying mechanism, H. pylori was cultured with nanostructured lipid carriers (1.25%) and analyzed by transmission electron microscopy and atomic force microscopy, and they found that most bacterial membranes were disrupted after 12 h (). This indicated that nanostructured lipid carriers can kill H. pylori by destroying their membranes. Furthermore, nanostructured lipid carriers have been demonstrated to have the ability to be specific to H. pylori and do not affect gut microbiota.Citation80
In summary, lipid-based nanoparticles exhibit high biocompatibility and can enhance the solubility and bioavailability of anti-H. pylori drugs. Additionally, they can kill bacteria inside cells or biofilms via endocytosis or fusion with the cell membrane. However, lipid-based nanoparticles exhibit drug leakage (which affects the control of drug release)Citation90 and involve complicated preparation processes (e.g., liposomes), making routine clinical applications challenging. Therefore, developing methods to improve their controlled drug-release capacity and simplifying their synthetic procedures could make lipid-based nanoparticles an effective alternative for H. pylori therapy in the future.
Polymeric nanoparticles
Polymeric nanoparticles are produced using polymeric materials (such as chitosan, ethylcellulose, and poly(lactic-co-glycolic acid)) and colloidal organic compounds in nanosizes.Citation91,Citation92 Compared with lipid-based nanoparticles, polymeric nanoparticles exhibit higher loading capacities and mechanical stabilities.Citation93 Chitosan, the most widely studied natural cationic polysaccharide polymer, exhibits good adhesion and antibacterial properties.Citation94 Interestingly, chitosan nanoparticles can bind to H. pylori through electrostatic interactions and inhibit its growth by changing the permeability of bacterial cell membranes, even without any drug loading. For example, Luo et al. have developed chitosan nanoparticles that exhibit the highest bacteriostatic activity at pH 4. Notably, they found that chitosan nanoparticles with a 95% degree of deacetylation exhibited a higher anti-H. pylori effect than those with an 88.5% degree of deacetylation, indicating that a stronger antibacterial effect is exhibited by chitosans with a higher degree of deacetylation.Citation94 Furthermore, chitosan and its derivatives can be utilized as drug delivery systems because of their special mucoadhesive properties (due to the electrostatic interaction between the positively charged chitosan and negatively charged cells/mucus), which can significantly extend the residence time of drugs in the stomach.Citation95,Citation96 For example, Lin et al. developed pH-responsive chitosan nanoparticles by integrating chitosan and heparin.Citation97 Their results showed that the nanoparticles enhanced the drug-membrane epithelium interaction through the electrostatic interaction between chitosan and mucosa and promoted gastric ulcer healing by the effect of heparin.Citation97 Under acidic conditions (pH in the range of 1.2–6.5), heparin and chitosan form spherical polyelectrolyte complexes that disintegrate upon reaching the infection site (where the pH is approximately neutral).Citation97 In their later study, they fabricated amoxicillin-loaded genipin-cross-linked fucose-conjugated chitosan/heparin nanoparticles (genipin-FCS/Hep nanoparticles).Citation96 Fucose can directly adhere to H. pylori, binding to it, while genipin can slow down the release of amoxicillin. The results showed that the effect of amoxicillin-loaded genipin-FCS/Hep nanoparticles in clearing H. pylori infection was stronger than that of amoxicillin alone ().Citation32,Citation96 Targeting lesions directly is an effective strategy to increase drug delivery efficiency and reduce the occurrence of antibiotic resistance in H. pylori. Based on a similar idea, Lin et al. fabricated fucose-chitosan/heparin nanoparticles to deliver berberine, a traditional Chinese medicine with antibacterial and anti-infective properties.Citation99 Their results demonstrated that targeting H. pylori directly enables more significant H. pylori growth inhibition than other untargeted strategies.Citation99 In addition, chitosan has been combined with other materials to form systems that exhibit unique characteristics with high antibacterial activity. For example, Yang et al. developed superparamagnetic iron oxide nanoparticles coloaded with amoxicillin and chitosan/polyacrylic acid particles.Citation95 Polyacrylic acid particles can compete with amoxicillin to bind to chitosan, enabling the continuous release of amoxicillin in the stomach. What is interesting in this study is the role of superparamagnetic iron oxide in this system, which increased the ability of nanoparticles to penetrate the H. pylori membrane and prolonged the residence time of nanoparticles in the stomach after exposure to the magnetic field ().Citation95 Rhamnolipid-coated chitosan hybrid nanoparticles are advantageous because they integrate the high drug-loading efficiency and stability of chitosan nanoparticles with the biofilm-destruction ability of rhamnolipid layers. For example, Li et al. and Arif et al. have established rhamnolipid-chitosan hybrid nanoparticles loaded with either clarithromycin or amoxicillin and applied them to gastric delivery; both studies indicate a strong ability (89% and 99%, respectively) to eradicate H. pylori biofilms under the mucus layer at minimal inhibitory concentrations of 32 and 132 µg/ml, respectively ()Citation98,Citation100 This further confirms the versatility of biomaterials as anti-H. pylori agents; they exhibit different properties in combination with different materials. In addition to chitosan, other polymers (such as ethyl cellulose) have also been studied.Citation101
Figure 4. a) A representation of amoxicillin-loaded genipin-FCS/Hep NPs, the strategy and observations for H. pylori eradication using them, and the effects of amoxicillin solution alone and with/without amoxicillin-loaded genipin-FCS/Hep NPs in an H. pylori-induced gastric infection mouse model; b) Schematic illustration of SPIO/AMO@PAA/CHI nanoparticles in the gastric acid of gastric lumen; they adhere to and penetrate the mucus layer, become unstable, and release amoxicillin at the site of H. pylori infection. The distribution of the prepared FITC-SPIO/AMO@PAA/CHI in the mucin layer with/without exposure to a magnetic field for 5 and 10 min. c) Schematic illustration of the structure and bacterial biofilm eradication process of lipid polymer nanoparticles. (a) Reproduced with permission from ref,Citation96 © 2013, Elsevier Ltd. (b) Reproduced with permission from ref,Citation95 © 2020, American Chemical Society (c) Reproduced with permission from ref,Citation98 © 2019, Elsevier B.V.
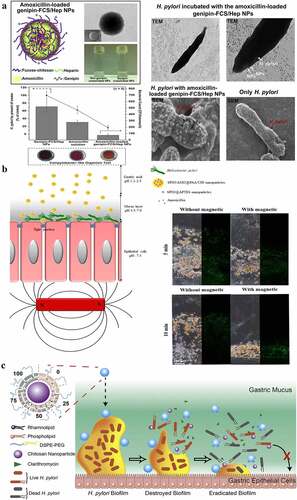
Polymers are also used as delivery vehicles for mRNA, DNA, and/or adjuvants in vaccines in various forms and morphologies because of their excellent stability.Citation102 For example, Chehelgerdi et al. prepared a cagW‑based DNA vaccine loaded with chitosan nanoparticles;Citation103 upon injecting mice with this vaccine, the expression of cagW increased on the 7th day and then gradually decreased, reaching a minimum on the 45th day. Furthermore, they found that vaccination significantly increases cellular immunity. Interestingly, during the H. pylori challenge, the vaccinated mice can clear the H. pylori infection. This suggested that the vaccine exhibited a protective effect against H. pylori in mice.Citation103 In addition to chitosan, polymers such as HP55 (a special type of hydroxypropyl methylcellulose phthalate)/D and poly(lactic-co-glycolic acid) (PLGA) have also been used as delivery systems for vaccines.Citation104 For example, Tan et al. designed an oral dual-antigen epitope and dual-adjuvant vaccine based on the urease of H. pylori and cholera toxin B subunits and delivered it with HP55/PLGA nanoparticles.Citation104 This vaccine exhibits pH-dependent antigen release, with a release rate of less than 5% in acidic environments (pH ≤ 4.5) and 75% in neutral environments (pH = 7.5). In vivo results indicate that vaccine-encapsulated HP55/PLGA nanoparticles elicit high levels of systemic and localized antigen-specific antibodies and Th1/Th17-biased immune responses. During H. pylori challenge, mice injected with this vaccine exhibited 43% complete protection.Citation104,Citation105 Oral vaccines are considered to be the best way to elicit an immune response in the human stomach. However, owing to the acidic environment in the stomach, oral vaccines are easily hydrolyzed, resulting in low antigen uptake efficiency. The use of polymer nanoparticles to deliver oral vaccines is undoubtedly a good way to solve this problem.
Although efforts to develop an H. pylori vaccine began in the early 1990s,Citation106 effective vaccines are currently lacking. The main reason for this could be their inability to induce a completely protective immune response against H. pylori.Citation107 Moreover, the necessity for developing an H. pylori vaccine remains controversial. Nevertheless, rapidly increasing drug resistance rates, the high prevalence of H. pylori infection, and frequent reinfections due to ineffective antibiotic treatment indicate that childhood vaccination to prevent H. pylori infection could be an effective and economical strategy. This is especially true for countries with a high incidence of gastric cancer (such as China and Japan).Citation108 At present, identifying suitable antigens and adjuvants is vital to facilitate the development of H. pylori vaccines. The delivery of H. pylori vaccines using nanotechnology could improve vaccine delivery effectiveness and prevent its degradation, thereby improving vaccine efficacy. With the declining success rate of current H. pylori treatment strategies, designing an effective vaccine could be a cost-effective way to control H. pylori epidemics.
To summarize, polymer-based polymeric nanoparticles are mainly investigated as drug delivery systems in H. pylori eradication regimens, particularly for hydrophobic drugs. They cause high drug stability and controlled release, leading to a high drug delivery efficiency and low H. pylori drug resistance. Some polymer-based polymeric nanoparticles, such as chitosan, exhibit excellent adhesion and the ability to change the permeability of H. pylori membranes, which enables chitosan nanoparticles to inhibit the growth of H. pylori, even without any drug loading.
Inorganic nanoparticles
Inorganic nanoparticles are defined as inorganic salts (such as silver, gold, and zinc) or oxides with nanoscale sizes (1–100 nm); they exhibit small particle sizes, large specific surface areas, numerous surface-active centers, and strong catalytic abilities.Citation33,Citation109 As an effective strategy to eradicate H. pylori, anti-H. pylori mechanisms of inorganic nanoparticles mainly involve metal-ion release, ROS production, and disruption of the H. pylori cell membrane and biofilm.Citation32,Citation110 Owing to inorganic nanoparticles’ unique antimicrobial mechanism, it is difficult for H. pylori to develop resistance.
Among the inorganic nanoparticles, silver nanoparticles, which inhibit the urease activity of H. pylori and reduce biofilm formation, are the most representative.Citation22,Citation111 Gopalakrishnan et al. designed N-acylhomoserine lactonase-stabilized silver nanoparticles (AiiAAgnanoparticles) that inhibit biofilm formation by degrading quorum-sensing molecules and inhibit urea production in H. pylori.Citation22 Interestingly, AiiAAgNPs exhibit no toxic side effects at effective concentrations (1–5 μM), but they exhibit cytotoxic effects against tumor cells at high concentrations (80–100 μM).Citation22 Similarly, Camargo et al. also synthesized a silver ion-based complex (Ag(PhTSC∙HCl)2](NO3)∙H2O) and transported it using polymeric nanoparticles.Citation111 Their results showed that these complexes exhibited high efficacy against floating H. pylori and H. pylori present in biofilms, with no in vivo toxicity for Galleria mellonella.Citation111
Gold nanoparticles are also commonly used inorganic nanoparticles and have also been used for H. pylori eradication.Citation25,Citation112–114 Gold nanoparticles can deposit on the surface of H. pylori, which kills H. pylori by membrane disruption. In addition, gold nanoparticles can also enter the cell and produce ROS, which kill H. pylori by affecting its metabolism.Citation25 For example, Zhi et al. synthesized pH-sensitive acid-sensitive cis-aconitic anhydride-modified anti-H. pylori-conjugated gold nanostars (GNS@Ab).Citation25 Notably, they improved the bactericidal effect using near-infrared laser photothermal treatment, which reduced the emergence of H. pylori resistance and even eradicated H. pylori-resistant strains isolated from clinical patients ().Citation25 Additionally, most of the GNS@Abs are eliminated from the body after the complete eradication of H. pylori in vivo without disrupting the gut-microbiota balance.Citation25
Figure 5. a) Schematic preparation of pH-sensitive GNS@Ab and their application in targeted imaging and photothermal therapy of H. pylori in vivo with antibiotic resistance; b) (a) Schematic diagram of Pd(h)@ZIF-8 and Pd(H)@ZIF-8@AP synthesis and (b) the targeting (i), degradation (II, III), sterilization (IV), regulation of immunity (v), and restoration of gastric mucosa (VI) in the stomach of H. pylori-infected mice, and the influence of intestinal flora homeostasis; c) Schematic illustration of the fabrication and antibacterial process of Janus Ga/Zn micromotors. (a) Reproduced with permission from ref,Citation25 © 2019 Elsevier Inc. (b) Reproduced with permission from ref,Citation23 © 2021 Wiley‐VCH GmbH. (c) Reproduced with permission from ref,Citation115 © 2021 Wiley‐VCH GmbH.
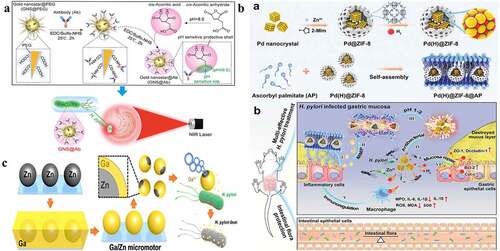
Zn and zinc oxide nanoparticles have also been used in anti-H. pylori regimens because of their ability to inhibit urease activity and good biocompatibility.Citation23,Citation116,Citation117 For example, Zhang et al. integrated Pd(H)@ZIF nanoparticles with ascorbate palmitate (AP, with a negative charge and enzymatic hydrolysis properties) ().Citation23 AP can precisely locate H. pylori, palladium (Pd) nanoparticles can absorb and release hydrogen (which changes the permeability of the cell membrane and interferes with H. pylori metabolism), and the zinc-based zeolitic-imidazolate framework (ZIF-8) can generate Zn2+ (which destroys the survival environment of H. pylori by inhibiting urease activity).Citation23 Notably, Pd(H)@ZIF-8@AP not only kills H. pylori but also inhibits the inflammatory response and repairs damaged mucosa by inhibiting the secretion of inflammatory factors in macrophages and removing ROS. In addition, the effect of Pd(H)@ZIF-8@AP on intestinal flora is minimal.Citation23 The hydrogen-generating ability of Zn-based micromotors in an acidic environment has been used to design mobile antibacterial platforms. For example, Lin et al. have integrated Zn-based micromotors with gallium ions. The gallium ions present a good ability to eradicate H. pylori, and the Ga/Zn micromotors exhibit good locomotivity in a simulated gastric acid environment (pH = 1.5). Moreover, Zn and Ga can degrade completely after 15 min ().Citation115
In summary, anti-H. pylori mechanisms of inorganic nanoparticles mainly involve oxidative stress, nonoxidative stress, and metal-ion release.Citation32,Citation112 Inorganic nanomaterials have gradually gained importance in the field of anti-H. pylori, particularly for eradicating drug-resistant bacteria, due to their anti-multidrug resistance and good antibacterial effects. They can effectively eradicate H. pylori by multiple antibacterial mechanisms, making it difficult for H. pylori to develop resistance, and they can be integrated with other bioactive materials to form multifunctional anti-H. pylori platforms. However, their refractory degradation and potential nanotoxicity require further investigation before use in clinical H. pylori therapy.
Microspheres
Microspheres are homogeneous monolithic particles ranging from 0.1–1000 µm in size that are widely used as controlled-release drug carriers.Citation118 Compared with nanoparticles, microspheres exhibit better loading capacities and controlled-release capabilities, facilitating the reduction of drug doses and long-term maintenance of therapeutic effects.Citation119,Citation120 Microspheres are often combined with other materials to form multifunctional anti-H. pylori platforms. For example, Tripathi et al. designed amoxicillin-loaded pectin/gellan gum-blended sodium alginate microspheres. The results showed that these delivery systems exhibit targeted drug delivery to gastric sites for the treatment of H. pylori, along with a suitable drug-release pattern.Citation121 Chitosan exhibits excellent adhesion and antibacterial properties,Citation122 and chitosan microspheres impart a good loading capacity and controlled-release capability to delivery systems, along with specific adhesion to H. pylori.Citation123,Citation124 Gonçalves et al. reported that chitosan microspheres exhibit the highest adherence to H. pylori at pH 6, and the microspheres are significantly more effective against BabA/SabA+H. pylori (76% reduction) strain than the BabA+/SabA H. pylori strain (50% reduction).Citation123,Citation125 Moreover, their results showed that chitosan microspheres can eradicate H. pylori through adhesion and are subsequently eliminated from the gastrointestinal tract.Citation126 Based on this idea, Adebisi et al. also designed a clarithromycin-loaded chitosan hybrid microsphere and found that chitosan causes a significantly high adhesion of the microsphere to H. pylori.Citation124
Collectively, microspheres exhibit high loading capacities and can sustain drug release over time, increasing the anti-H. pylori drug-delivery efficiency while maintaining the bactericidal concentration. In addition, combining with other materials makes microspheres a strong multifunctional anti-H. pylori platforms.
Hydrogels
Hydrogels are hydrophilic three-dimensional network-structured gels formed by water-soluble or hydrophilic polymers through chemical or physical cross-linking.Citation127 As another efficient delivery system for H. pylori therapeutic agents, hydrogels exhibit good biocompatibility, degradability, and controlled drug-release capacity.Citation128–130 Chitosan and its derivatives are often used as base materials to prepare hydrogels because of their antibacterial and adhesion properties with H. pylori.Citation129,Citation131,Citation132 For example, El-Mahrouk et al. reported metronidazole-loaded pH-sensitive chitosan hydrogels for H. pylori eradication therapy.Citation131 The results showed that the swelling and drug-release ability of the hydrogels were highly dependent on pH; they were significant at pH 1.2 but low in phosphate buffers at pH 7.4. In vivo experiments indicated that the system can exist in a dog’s stomach for at least 48 h, and its ability to kill H. pylori is stronger than that of oral metronidazole alone.Citation131 Similarly, Mohamed et al. have developed a novel chitosan hydrogel. Notably, they linked the chitosan hydrogel with benzophenone tetracarboxylimide benzoyl thiourea, which selectively inhibited cyclooxygenase-2 activity.Citation129 This means that hydrogels can also function as multifaceted platforms for H. pylori eradication. Additionally, hydrogels also exhibit high drug-loading capacity and the ability to load multiple anti-H. pylori agents.Citation133 For example, Silva et al. fabricated cross-linked sodium alginate-carboxymethyl cellulose hydrogels loaded with furazolidone (encapsulation efficiency: 71–76%) and bismuth(III) (encapsulation efficiency: 88%).Citation133 The hydrogels swelled to approximately 150% in the first 2 h, remaining for at least 6 days in simulated gastric tissue (pH = 1.2), indicating that the hydrogels can exhibit sustained drug release. Although this study did not show the role of hydrogels in eradicating H. pylori, it reported their drug-loading capacity and therapeutic potential toward H. pylori.
Hydrogels, with high drug-carrying capacity, are efficient anti-H. pylori drug-delivery systems. They exhibit numerous advantageous properties; their three-dimensional network structure improves drug stability, and they exhibit good biocompatibility and sustained drug-release ability.Citation134 Targeting the low drug utilization rate of anti-H. pylori drugs, hydrogels can effectively improve medication utilization by maintaining a sustained release of drugs in the stomach, effectively eradicating H. pylori and hindering the development of drug resistance.Citation135
Conclusion and future perspective
The increasing prevalence of antibiotic-resistant strains and high failure rate of treatment are severe challenges to the current H. pylori treatment strategies. Thus, extensive research has been carried out to find effective strategies to combat H. pylori infections and antibiotic resistance, including designing new drug combinations, extending the treatment periods, and performing susceptibility testing before treatment. However, the existence of gastric acid milieud and pepsin, which can destroy most of the antibiotics in the stomach, and gastric mucosa, which is approximately 200 μm thick with a pH gradient from pH 1–2 to 7,Citation32 cripple effective antibiotic delivery efficiency. This makes drug eradication programs extremely complicated, which not only significantly affects patient compliance but also increases eradication costs and consequently limits the applicability of H. pylori therapy. Therefore, improving drug delivery efficiencies and developing effective antibiotic-independent antimicrobial moieties could be promising emerging approaches to resolve the challenges associated with anti-H. pylori processes. As a new strategy to eradicate H. pylori, biomaterials have the following advantages (): (1) Protected drugs against stomach acid, targeted lesions and controlled drug release. With increasing antimicrobial resistance, the optimization of dosing and delivery efficiency can produce higher eradication rates. The biomaterials summarized in this review, such as nanoparticles, microspheres, and hydrogels, can protect drugs against the harsh acidic environment of the stomach and exhibit controlled drug-release capacity with high delivery efficiency. Among them, microspheres and hydrogels exhibit particularly high drug loading and controlled drug-release capacities and can deliver multiple anti-H. pylori drugs.Citation136,Citation137 Therefore, if we want to deliver multiple anti-H. pylori drugs, microspheres and hydrogels may be a good choice. For example, it may be interesting to see the eradication effects of vonoprazan dual therapy (a novel eradication regimen for H. pylori) delivered by microspheres or hydrogels in the future. Targeted lesions are another strategy for improving delivery efficiency, thereby increasing eradication rates and reducing the development of H. pylori resistance. Biomaterials (such as chitosan and ascorbate palmitate) can adhere to H. pylori through electrostatic interactions, targeting lesions, and indirectly prolonging the duration of drug action. However, Gottesmann et al. indicated that electrostatic interactions alone are not sufficient for specific interactions with H. pylori, and direct interactions with the H. pylori surface (such as specific binding with the mucin of H. pylori) are critical.Citation76 Hence, this should be considered when developing bacteria-targeting biomaterials. (2) Destroys the biofilm. Biofilms play an important role in the emergence of H. pylori antibiotic resistance, and the antimicrobial resistance of bacteria growing inside biofilms can be 1000 times higher than that of external bacteria.Citation100 Biomaterials, such as rhamnolipids and silver, can bind to the extracellular substance of biofilms and disrupt them by mediating signals or by other means. Therefore, strategies involving these biomaterials could be powerful “weapons” against the antibiotic-resistance crisis. (3) Changes in the permeability of the H. pylori membrane. Biomaterials such as linolenic acid, docosahexaenoic acid, and nanostructured lipid carriers can change the permeability of the H. pylori membrane, disrupting its integrity. Additionally, owing to the presence of components similar to those of cell membranes, these biomaterials could also have sterilizing effects on intracellular H. pylori compared with other biomaterials. Hence, these biomaterials could be used for the development of antibiotic-free anti-H. pylori agents. In addition, chitosan can also change the permeability of the H. pylori membrane; unlike the aforementioned biomaterials, its mechanism could involve its adhesion to H. pylori, followed by membrane destruction by the physiochemical properties of the nanomaterial. (4) Produced reactive oxygen species (ROS) or affected the metabolic activities of H. pylori. Apart from mechanically disrupting its membrane through the direct contact of nanomaterials with H. pylori, some biomaterials (such as gold nanoparticles, liquid metal gallium, Zn, and zinc oxide nanoparticles) eradicate H. pylori by generating ROS or by disrupting its metabolic activities. A large amount of ROS causes changes in bacterial morphology and DNA damage. Owing to their unique anti-H. pylori mechanisms, these biomaterials could also be suitable for developing antibiotic-free anti-H. pylori agents ().
Table 2. The current anti-H. pylori mechanism of biomaterials.
Figure 6. Schematic representation of the main mechanism of H. pylori eradication by biomaterials. (a) Acting as a physical barrier against stomach acids and enzymes, biomaterials provide better stability to the contained drugs. Furthermore, biomaterials delay drug release and target lesions, increasing the contact between H. pylori and the drug, thereby improving drug utilization. (b) Biomaterials directly destroy biofilms (one of the causative factors for H. pylori antibiotic resistance), eradicating H. pylori from biofilms. (c) Biomaterials damaging H. pylori membranes. (d) Biomaterials (such as inorganic metal materials) themselves exhibit antibacterial effects that kill H. pylori directly.
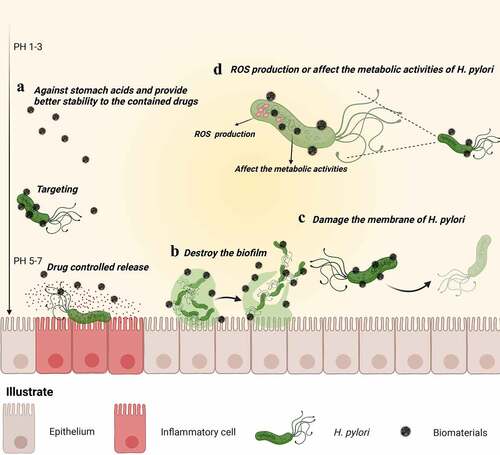
From bench to bedside, numerous challenges need to be addressed before designing biomaterials for the efficient treatment of H. pylori infections in the future. First, the designed biomaterials should exhibit high stability in the harsh acidic environment of the stomach to improve the bioavailability of drugs. Second, to ensure good anti-H. pylori activity by biomaterials, bacterial targeting should be applied in the formulation design. Third, rapidly increasing H. pylori resistance is largely attributable to the misuse of antibiotics. Delivering antibiotics with low resistance rates (such as amoxicillin), improving delivery efficiency, and developing antibiotic-independent antimicrobial moieties could be the focus of future studies. Fourth, the persistent survival of H. pylori inside cells and biofilms is also an important reason for therapeutic failure and the emergence of H. pylori resistance. Therefore, fabricating biomaterials that can eradicate H. pylori present intracellularly and inside biofilms is crucial for improving the cure rate. Additionally, it is also vital to maintain the ecological homeostasis of the gut ecosystem and develop biodegradable biomaterials for H. pylori treatment. Most importantly, clinical translation and widespread applications are the ultimate goals of biomaterial design, making cost effectiveness and large-scale production important considerations.
In summary, biomaterials can be used to encapsulate and deliver conventional antibiotics, antimicrobial lipids, vaccines, or phytomedicines with antibacterial effects to increase the drug delivery efficiency and hinder the development of drug resistance in H. pylori. Their unique multiple antimicrobial mechanisms eradicate H. pylori that is present intracellularly and inside biofilms and could solve the current problem of high drug resistance. This review discusses recent progress in biomaterials for the eradication of H. pylori and summarizes the main anti-H. pylori mechanisms. In conclusion, biomaterials exhibit high potential to be integrated into standard treatments in the future because of their safety and H. pylori eradication efficiency. The application of biomaterials could help achieve the ultimate goal of complete elimination of H. pylori in the future.
Author contributions and disclosures
Yongkang Lai, Wei Wei and Yiqi Du contributed equally to the review and writing of this manuscript. Jie Gao and Zhaoshen Li revised the review.
Disclosure statement
No potential conflict of interest was reported by the author(s).
Additional information
Funding
References
- Hooi JKY, Lai WY, Ng WK, Suen MMY, Underwood FE, Tanyingoh D, Malfertheiner P, Graham DY, Wong VWS, Wu JCY, et al. Global prevalence of Helicobacter pylori infection: systematic review and meta-analysis. Gastroenterology. 2017;153(2):420–22. doi:10.1053/j.gastro.2017.04.022
- Liou JM, Malfertheiner P, Lee YC, Sheu BS, Sugano K, Cheng HC, Yeoh KG, Hsu PI, Goh KL, Mahachai V, et al. Screening and eradication of Helicobacter pylori for gastric cancer prevention: the Taipei global consensus. Gut. 2020;69(12):2093–2112. doi:10.1136/gutjnl-2020-322368. PMCID: 33004546.
- Malfertheiner P, Megraud F, O’Morain CA, Gisbert JP, Kuipers EJ, Axon AT, Bazzoli F, Gasbarrini A, Atherton J, Graham DY, et al. Management of Helicobacter pylori infection-the Maastricht V/Florence Consensus Report. Gut. 2017;66(1):6–30. doi:10.1136/gutjnl-2016-312288. PMCID: 27707777.
- Ying L, Ferrero RL. Role of NOD1 and ALPK1/TIFA Signalling in Innate Immunity Against Helicobacter pylori Infection. Curr Top Microbiol Immunol. 2019;421:159–177.
- Robinson K, Atherton JC. The Spectrum of Helicobacter-Mediated Diseases. Annu Rev Pathol. 2021;16:123–144.
- Cai Q, Zhu C, Yuan Y, Feng Q, Feng Y, Hao Y, Li J, Zhang K, Ye G, Ye L, et al. Development and validation of a prediction rule for estimating gastric cancer risk in the Chinese high-risk population: a nationwide multicentre study. Gut. 2019;68(9):1576–1587. doi:10.1136/gutjnl-2018-317556. PMCID: 30926654.
- Queiroz DM, Harris PR, Sanderson IR, Windle HJ, Walker MM, Rocha AM, Rocha GA, Carvalho SD, Bittencourt PF, de Castro LP, et al. Iron status and Helicobacter pylori infection in symptomatic children: an international multi-centered study. PloS one. 2013;8(7):e68833. doi:10.1371/journal.pone.0068833. PMCID: 23861946.
- Sato R, Murakami K, Okimoto T, Watanabe K, Kodama M, Fujioka T. Development of corpus atrophic gastritis may be associated with Helicobacter pylori-related idiopathic thrombocytopenic purpura. J Gastroenterol. 2011;46:991–997.
- Green R, Allen LH, Bjørke-Monsen AL, Brito A, Guéant JL, Miller JW, Molloy AM, Nexo E, Stabler S, Toh BH, et al. Vitamin B(12) deficiency. Nat Rev Dis Primers. 2017;3:17040 doi:10.1038/nrdp.2017.40. PMCID: 28660890.
- Sugano K, Tack J, Kuipers EJ, Graham DY, El-Omar EM, Miura S, Haruma K, Asaka M, Uemura N, Malfertheiner P, et al. Kyoto global consensus report on Helicobacter pylori gastritis. Gut. 2015;64(9):1353–1367. doi:10.1136/gutjnl-2015-309252
- Ford AC, Yuan Y, Moayyedi P. Helicobacter pylori eradication therapy to prevent gastric cancer: systematic review and meta-analysis. Gut. 2020;69(12):2113–2121. doi:10.1136/gutjnl-2020-320839
- Chey WD, Leontiadis GI, Howden CW, Moss SF. ACG Clinical Guideline: treatment of Helicobacter pylori Infection. Am J Gastroenterol. 2017;112(2):212–239. doi:10.1038/ajg.2016.563
- Graham DY, Dore MP, Lu H. Understanding treatment guidelines with bismuth and non-bismuth quadruple Helicobacter pylori eradication therapies. Expert Rev Anti Infect Ther. 2018;16(9):1353–1367. doi:10.1080/14787210.2018.1511427
- Howden CW, Graham DY. Recent Developments Pertaining to H. pylori Infection. Am J Gastroenterol. 2021;116:1–3.
- Tshibangu-Kabamba E, Yamaoka Y. Helicobacter pylori infection and antibiotic resistance - from biology to clinical implications. Nat Rev Gastroenterol Hepatol. 2021;18:613–629.
- Capurro MI, Greenfield LK, Prashar A, Xia S, Abdullah M, Wong H, Zhong XZ, Bertaux-Skeirik N, Chakrabarti J, Siddiqui I, et al. VacA generates a protective intracellular reservoir for Helicobacter pylori that is eliminated by activation of the lysosomal calcium channel TRPML1. Natu microbiol. 2019;4(8):1411–1423. doi:10.1038/s41564-019-0441-6. PMCID: 31110360.
- Chouhan D, Mandal BB. Silk biomaterials in wound healing and skin regeneration therapeutics: from bench to bedside. Acta biomaterialia. 2020;103:24–51.
- Kharaziha M, Baidya A, Annabi N. Rational design of immunomodulatory hydrogels for chronic wound healing. Adv Mater. 2021;33:e2100176.
- Xu W, Dong S, Han Y, Li S, Liu Y. Hydrogels as antibacterial biomaterials. Curr Pharm Des. 2018;24:843–854.
- Pan H, Zheng M, Ma A, Liu L, Cai L. Cell/bacteria-based bioactive materials for cancer immune modulation and precision therapy. Adv Mater. 2021;33:e2100241.
- de Souza MPC, de Camargo BAF, Spósito L, Fortunato GC, Carvalho GC, Marena GD, Meneguin AB, Bauab TM, Chorilli M, et al. Highlighting the use of micro and nanoparticles based-drug delivery systems for the treatment of Helicobacter pylori infections. Crit Rev Microbiol. 2021;47(4):435–460. doi:10.1080/1040841x.2021.1895721. PMCID: 33725462.
- Gopalakrishnan V, Masanam E, Ramkumar VS, Baskaraligam V, Selvaraj G. Influence of N-acylhomoserine lactonase silver nanoparticles on the quorum sensing system of Helicobacter pylori: a potential strategy to combat biofilm formation. J Basic Microbiol. 2020;60:207–215.
- Zhang W, Zhou Y, Fan Y, Cao R, Xu Y, Weng Z, Ye J, He C, Zhu Y, Wang X. Metal-organic-framework-based hydrogen-release platform for multieffective Helicobacter pylori targeting therapy and intestinal flora protective capabilities. Adv Mater. 2022;34(2):e2105738. doi:10.1002/adma.202105738. PMCID: 34655499.
- Obonyo M, Zhang L, Thamphiwatana S, Pornpattananangkul D, Fu V, Zhang L. Antibacterial activities of liposomal linolenic acids against antibiotic-resistant Helicobacter pylori. Mol Pharm. 2012;9:2677–2685.
- Zhi X, Liu Y, Lin L, Yang M, Zhang L, Zhang L, Liu Y, Alfranca G, Ma L, Zhang Q, et al. Oral pH sensitive GNS@ab nanoprobes for targeted therapy of Helicobacter pylori without disturbance gut microbiome. Nanomedicine. 2019;20:102019. doi:10.1016/j.nano.2019.102019. PMCID: 31125676.
- Saravanakumar K, Chelliah R, MubarakAli D, Oh DH, Kathiresan K, Wang MH. Unveiling the potentials of biocompatible silver nanoparticles on human lung carcinoma A549 cells and Helicobacter pylori. Sci Rep. 2019;9:5787.
- Lin J, Huang WW. A systematic review of treating Helicobacter pylori infection with Traditional Chinese Medicine. World J Gastroenterol. 2009;15:4715–4719.
- Homan M, Orel R. Are probiotics useful in Helicobacter pylori eradication? World J Gastroenterol. 2015;21:10644–10653.
- Zhang L, Wu WK, Gallo RL, Fang EF, Hu W, Ling TK, Shen J, Chan RL, Lu L, Luo XM, et al. Critical role of antimicrobial peptide cathelicidin for controlling Helicobacter pylori survival and infection. J Immunol. 2016;196(4):1799–1809. doi:10.4049/jimmunol.1500021. PMCID: 26800870.
- Ni WW, Liu Q, Ren SZ, Li WY, Yi LL, Jing H, Sheng LX, Wan Q, Zhong PF, Fang HL, et al. The synthesis and evaluation of phenoxyacylhydroxamic acids as potential agents for Helicobacter pylori infections. Bioorg Med Chem. 2018;26(14):4145–4152. doi:10.1016/j.bmc.2018.07.003. PMCID: 29983280.
- Robinson K, Lehours P. Review - Helicobacter, inflammation, immunology and vaccines. Helicobacter. 2020;25:e12737.
- Zhang Q, Wu W, Zhang J, Xia X. Eradication of Helicobacter pylori: the power of nanosized formulations. Nanomedicine. 2020;15:527–542.
- Safarov T, Kiran B, Bagirova M, Allahverdiyev AM, Abamor ES. An overview of nanotechnology-based treatment approaches against Helicobacter Pylori. Expert Rev Anti Infect Ther. 2019;17:829–840.
- Marshall BJ, Warren JR. Unidentified curved bacilli in the stomach of patients with gastritis and peptic ulceration. Lancet. 1984;1:1311–1315.
- Fang JY, Du YQ, Liu WZ, Ren JL, Li YQ, Chen XY, Lv NH, Chen YX, Lv B. Chinese consensus on chronic gastritis (2017, Shanghai). J Dig Dis. 2018;19(4):182–203. doi:10.1111/1751-2980.12593. PMCID: 29573173.
- Hanada K, Graham DY. Helicobacter pylori and the molecular pathogenesis of intestinal-type gastric carcinoma. Expert Rev Anticancer Ther. 2014;14:947–954.
- Sukri A, Hanafiah A, Mohamad Zin N, Kosai NR. Epidemiology and role of Helicobacter pylori virulence factors in gastric cancer carcinogenesis. APMIS. 2020;128:150–161.
- Chiang TH, Chang WJ, Chen SL, Yen AM, Fann JC, Chiu SY, Chen YR, ChuangSL, Shieh CF, Liu CY, et al. Mass eradication of Helicobacter pylori to reduce gastric cancer incidence and mortality: a long-term cohort study on Matsu Islands. Gut. 2021;70(2):243–250. doi:10.1136/gutjnl-2020-322200. PMCID: 32792335.
- Ansari S, Yamaoka Y. Helicobacter pylori Virulence factors exploiting gastric colonization and its pathogenicity. Toxins. 2019;11(11):14–23.
- Baj J, Forma A, Sitarz M, et al. Helicobacter pylori virulence factors-mechanisms of bacterial pathogenicity in the gastric microenvironment. Cells. 2020;10(1):e00001
- Kao CY, Sheu BS, Wu JJ. Helicobacter pylori infection: an overview of bacterial virulence factors and pathogenesis. Biomed J. 2016;39:14–23.
- Xu C, Soyfoo DM, Wu Y, Xu S. Virulence of Helicobacter pylori outer membrane proteins: an updated review. EurJ Clin Microbiol Infect Dis. 2020;39:1821–1830.
- Sharndama HC, Mba IE. Helicobacter pylori: an up-to-date overview on the virulence and pathogenesis mechanisms. Braz J Microbiol. 2022;53:33–50.
- Cover TL, Lacy DB, Ohi MD. The Helicobacter pylori cag type IV secretion system. Trends Microbiol. 2020;28:682–695.
- Xie C, Li N, Wang H, He C, Hu Y, Peng C, Ouyang Y, Wang D, Xie Y, Chen J, et al. Inhibition of autophagy aggravates DNA damage response and gastric tumorigenesis via Rad51 ubiquitination in response to H. pylori infection. Gut Microbes. 2020;11(6):1567–1589. doi:10.1080/19490976.2020.1774311. PMCID: 32588736.
- Raju D, Hussey S, Ang M, Terebiznik MR, Sibony M, Galindo-Mata E, Gupta V, Blanke SR, Delgado A, Romero-Gallo J, et al. Vacuolating cytotoxin and variants in Atg16L1 that disrupt autophagy promote Helicobacter pylori infection in humans. Gastroenterology. 2012;142(5):1160–1171. doi:10.1053/j.gastro.2012.01.043. PMCID: 22333951.
- Shiotani A, Roy P, Lu H, Graham DY. Helicobacter pylori diagnosis and therapy in the era of antimicrobial stewardship. Therap Adv Gastroenterol. 2021;14:17562848211064080.
- Suzuki S, Gotoda T, Kusano C, Ikehara H, Ichijima R, Ohyauchi M, Ito H, Kawamura M, Ogata Y, Ohtaka M, et al. Seven-day vonoprazan and low-dose amoxicillin dual therapy as first-line Helicobacter pylori treatment: a multicentre randomised trial in Japan. Gut. 2020;69(6):1019–1026. doi:10.1136/gutjnl-2019-319954. PMCID: 31915235.
- Hu Y, Zhu Y, Lu NH. Recent progress in Helicobacter pylori treatment. Chin Med J. 2020;133:335–343.
- Kim BJ, Lee H, Lee YC, Jeon SW, Kim JH, Kim HS, Sung JK, Lee DH, Kim HU, Park MI, et al. Ten-day concomitant, 10-day sequential, and 7-day triple therapy as first-line treatment for Helicobacter pylori Infection: a nationwide randomized Trial in Korea. Gut Liver. 2019;13(5):531–540. doi:10.5009/gnl19136. PMCID: 31505907.
- Hsu PI, Lin PC, Graham DY. Hybrid therapy for Helicobacter pylori infection: a systemic review and meta-analysis. World J Gastroenterol. 2015;21:12954–12962.
- Graham DY, Hernaez R, Rokkas T. Cross-roads for meta-analysis and network meta-analysis of H. pylori therapy. Gut. 2022;71:643–650.
- Graham DY, Canaan Y, Maher J, Wiener G, Hulten KG, Kalfus IN. Rifabutin-Based Triple Therapy (RHB-105) for Helicobacter pylori Eradication: a Double-Blind, Randomized, Controlled Trial. Ann Intern Med. 2020;172:795–802.
- Graham DY, Lu H, Shiotani A. Vonoprazan-containing Helicobacter pylori triple therapies contribution to global antimicrobial resistance. J Gastroenterol Hepatol. 2021;36:1159–1163.
- Murakami K, Sakurai Y, Shiino M, Funao N, Nishimura A, Asaka M. Vonoprazan, a novel potassium-competitive acid blocker, as a component of first-line and second-line triple therapy for Helicobacter pylori eradication: a phase III, randomised, double-blind study. Gut. 2016;65:1439–1446.
- Tai WC, Liang CM, Kuo CM, Huang PY, Wu CK, Yang SC, Kuo YH, Lin MT, Lee CH, Hsu CN, et al. A 14 day esomeprazole- and amoxicillin-containing high-dose dual therapy regimen achieves a high eradication rate as first-line anti-Helicobacter pylori treatment in Taiwan: a prospective randomized trial. J Antimicrob Chemother. 2019;74(6):1718–1724. doi:10.1093/jac/dkz046. PMCID: 30768161.
- Horii T, Suzuki S, Takano C, Shibuya H, Ichijima R, Kusano C, Ikehara H, Gotoda T. Lower impact of vonoprazan-amoxicillin dual therapy on gut microbiota for Helicobacter pylori eradication. J Gastroenterol Hepatol. 2021;36(12):3314–3321. doi:10.1111/jgh.15572. PMCID: 34107551.
- Du YQ, Su T, Fan JG, Lu YH, Zheng P, Li XH, Guo CY, Xu P, Gong YF, Li ZS. Adjuvant probiotics improve the eradication effect of triple therapy for Helicobacter pylori infection. World J Gastroenterol. 2012;18(43):6302–6307. doi:10.3748/wjg.v18.i43.6302. PMCID: 23180952.
- Ding SZ, Du YQ, Lu H, Wang WH, Cheng H, Chen SY, Chen MH, Chen WC, Chen Y, Fang JY, et al. Chinese consensus report on family-based Helicobacter pylori infection control and management (2021 Edition). Gut. 2022;71(2):238–253. doi:10.1136/gutjnl-2021-325630. PMCID: 34836916.
- Li BZ, Threapleton DE, Wang JY, Xu JM, Yuan JQ, Zhang C, Li P, Ye QL, Guo B, Mao C, et al. Comparative effectiveness and tolerance of treatments for Helicobacter pylori: systematic review and network meta-analysis. BMJ. 2015;351:h4052. doi:10.1136/bmj.h4052. PMCID: 26290044.
- Yonezawa H, Osaki T, Kamiya S. Biofilm Formation by Helicobacter pylori and Its Involvement for Antibiotic Resistance. Biomed Res Int. 2015;2015:914791.
- Hathroubi S, Servetas SL, Windham I, Merrell DS, Ottemann KM. Helicobacter pylori biofilm formation and its potential role in pathogenesis. Microbiol Mol Biol Rev. 2018;82(2):e00001–18
- Moghadam MT, Chegini Z, Khoshbayan A, Farahani I, Shariati A. Helicobacter pylori Biofilm and New Strategies to Combat it. Curr Mol Med. 2021;21:549–561.
- Patil-Sen Y. Advances in nano-biomaterials and their applications in biomedicine. Emerg Top Life Sci. 2021;5:169–176.
- Pinilla CMB, Lopes NA, Brandelli A. Lipid-based nanostructures for the delivery of natural antimicrobials. Molecules. 2021;26(12):3587
- Yousefi M, Ehsani A, Jafari SM. Lipid-based nano delivery of antimicrobials to control food-borne bacteria. Adv Colloid Interface Sci. 2019;270:263–277.
- Naseri N, Valizadeh H, Zakeri-Milani P. Solid lipid nanoparticles and nanostructured lipid carriers: structure, preparation and application. Adv Pharm Bull. 2015;5:305–313.
- Zhang Y, Xie F, Yin Y, Zhang Q, Jin H, Wu Y, Pang L, Li J, Gao J. Immunotherapy of tumor RNA-loaded lipid nanoparticles against hepatocellular carcinoma. Int J Nanomedicine. 2021;16:1553–1564. doi:10.2147/ijn.s291421. PMCID:33658783.
- Li M, Du C, Guo N, Teng Y, Meng X, Sun H, Li S, Yu P, Galons H. Composition design and medical application of liposomes. Eur J Med Chem. 2019;164:640–653. doi:10.1016/j.ejmech.2019.01.007. PMCID: 30640028.
- Eleraky NE, Allam A, Hassan SB, Omar MM. Nanomedicine Fight against Antibacterial Resistance: an Overview of the Recent Pharmaceutical Innovations. Pharmaceutics. 2020;12(2):8271–85.
- Dai D, Yin Y, Hu Y, Lu Y, Zou H, Lu G, WangLian QJ, Gao J, Shen X. Tumor RNA-loaded nanoliposomes increases the anti-tumor immune response in colorectal cancer. Drug Deliv. 2021;28(1):1548–1561. doi:10.1080/10717544.2021.1954727. PMCID: 34286631.
- Xing H, Hwang K, Lu Y. Recent developments of liposomes as nanocarriers for theranostic applications. Theranostics. 2016;6:1336–1352.
- Plaza-Oliver M, Santander-Ortega MJ, Lozano MV. Current approaches in lipid-based nanocarriers for oral drug delivery. Drug Deliv Transl Res. 2021;11:471–497.
- Alam MI, Paget T, Elkordy AA. Formulation and advantages of furazolidone in liposomal drug delivery systems. Europ j pharm sci. 2016;84:139–145.
- Thamphiwatana S, Gao W, Pornpattananangkul D, Zhang Q, Fu V, Li J, Li J, Obonyo M, Zhang L. Phospholipase A2-responsive antibiotic delivery via nanoparticle-stabilized liposomes for the treatment of bacterial infection. J Mater Chem B. 2014;2(46):8201–8207. doi:10.1039/c4tb01110d. PMCID: 25544886.
- Gottesmann M, Goycoolea FM, Steinbacher T, Menogni T, Hensel A. Smart drug delivery against Helicobacter pylori: pectin-coated, mucoadhesive liposomes with antiadhesive activity and antibiotic cargo. Appl Microbiol Biotechnol. 2020;104:5943–5957.
- Bardonnet PL, Faivre V, Boullanger P, Piffaretti JC, Falson F. Pre-formulation of liposomes against Helicobacter pylori: characterization and interaction with the bacteria. Eur J Pharm Biopharm. 2008;69:908–922.
- Cai J, Huang H, Song W, Hu H, Chen J, Zhang L, Li P, Wu R, Wu C. Preparation and evaluation of lipid polymer nanoparticles for eradicating H. pylori biofilm and impairing antibacterial resistance in vitro. Int J Pharm. 2015;495(2):728–737. doi:10.1016/j.ijpharm.2015.09.055. PMCID: 26417849.
- Yang Y, Chen L, Sun HW, Guo H, Song Z, You Y, Yang LY, Tong YN, Gao JN, Zeng H, et al. Epitope-loaded nanoemulsion delivery system with ability of extending antigen release elicits potent Th1 response for intranasal vaccine against Helicobacter pylori. J Nanobiotechnology. 2019;17(1):6. doi:10.1186/s12951-019-0441-y. PMCID: 30660182.
- Seabra CL, Nunes C, Brás M, Gomez-Lazaro M, Reis CA, Gonçalves IC, Reis S, Martins MCL. Lipid nanoparticles to counteract gastric infection without affecting gut microbiota. Eur J Pharm Biopharm. 2018;127:378–386. doi:10.1016/j.ejpb.2018.02.030. PMCID: 29524597.
- Thamphiwatana S, Gao W, Obonyo M, Zhang L. In vivo treatment of Helicobacter pylori infection with liposomal linolenic acid reduces colonization and ameliorates inflammation. Proc Natl Acad Sci U S A. 2014;111:17600–17605.
- Wang R, Song C, Gao A, Liu Q, Guan W, Mei J, Ma L, Cui D. Antibody-conjugated liposomes loaded with indocyanine green for oral targeted photoacoustic imaging-guided sonodynamic therapy of Helicobacter pylori infection. Acta biomaterialia. 2022. doi:10.1016/j.actbio.2022.02.031.
- Singh DY, Prasad NK. Double liposomes mediated dual drug targeting for treatment of Helicobacter pylori infections. Die Pharmazie. 2011;66:368–373.
- Jain P, Jain S, Prasad KN, Jain SK, Vyas SP. Polyelectrolyte coated multilayered liposomes (nanocapsules) for the treatment of Helicobacter pylori infection. Mol Pharm. 2009;6:593–603.
- Shah S, Dhawan V, Holm R, Nagarsenker MS, Perrie Y. Liposomes: advancements and innovation in the manufacturing process. Adv Drug Deliv Rev. 2020;154-155:102–122.
- Vargas KM, Shon YS. Hybrid lipid-nanoparticle complexes for biomedical applications. J Mater Chem B. 2019;7:695–708.
- Tran LTC, Gueutin C, Frebourg G, Burucoa C, Faivre V. Erythromycin encapsulation in nanoemulsion-based delivery systems for treatment of Helicobacter pylori infection: protection and synergy. Biochem Biophys Res Commun. 2017;493:146–151.
- Khosa A, Reddi S, Saha RN. Nanostructured lipid carriers for site-specific drug delivery. Biomed Pharmacother. 2018;103:598–613.
- Seabra CL, Nunes C, Gomez-Lazaro M, Correia M, Machado JC, Gonçalves IC, Reis CA, Reis S, Martins MCL. Docosahexaenoic acid loaded lipid nanoparticles with bactericidal activity against Helicobacter pylori. Int J Pharm. 2017;519(1–2):128–137 doi:10.1016/j.ijpharm.2017.01.014. PMCID: 28088639.
- Mirchandani Y, Patravale VB, B S. Solid lipid nanoparticles for hydrophilic drugs. J Control Release. 2021;335:457–464.
- Borandeh S, van Bochove B, Teotia A, Seppälä J. Polymeric drug delivery systems by additive manufacturing. Adv Drug Deliv Rev. 2021;173:349–373.
- Zhang S, Li Y, Qiu X, Jiao A, Luo W, Lin X, Zhang X, Zhang Z, Hong J, Cai P, et al. Incorporating redox-sensitive nanogels into bioabsorbable nanofibrous membrane to acquire ROS-balance capacity for skin regeneration. Bioact Mater. 2021;6(10):3461–3472. doi:10.1016/j.bioactmat.2021.03.009. PMCID: 33817421.
- Wilczewska AZ, Niemirowicz K, Markiewicz KH, Car H. Nanoparticles as drug delivery systems. Pharmacoll Rep. 2012;64:1020–1037.
- Luo D, Guo J, Wang F, Sun J, Li J, Cheng X, Chang M, Yan X. Preparation and evaluation of anti-Helicobacter pylori efficacy of chitosan nanoparticles in vitro and in vivo. J Biomater Sci Polym Ed. 2009;20(11):1587–1596. doi:10.1163/092050609x12464345137685. PMCID: 19619399.
- Yang SJ, Huang CH, Yang JC, Wang CH, Shieh MJ. Residence time-extended nanoparticles by magnetic field improve the eradication efficiency of Helicobacter pylori. ACS Appl Mater Interfaces. 2020;12:54316–54327.
- Lin YH, Tsai SC, Lai CH, Lee CH, He ZS, Tseng GC. Genipin-cross-linked fucose-chitosan/heparin nanoparticles for the eradication of Helicobacter pylori. Biomaterials. 2013;34:4466–4479.
- Lin YH, Chang CH, Wu YS, Hsu YM, Chiou SF, Chen YJ. Development of pH-responsive chitosan/heparin nanoparticles for stomach-specific anti-Helicobacter pylori therapy. Biomaterials. 2009;30:3332–3342.
- Li P, Chen X, Shen Y, Li H, Zou Y, Yuan G, Hu P, Hu H. Mucus penetration enhanced lipid polymer nanoparticles improve the eradication rate of Helicobacter pylori biofilm. J Control Release. 2019;300:52–63. doi:10.1016/j.jconrel.2019.02.039. PMCID: 30825476.
- Lin YH, Lin JH, Chou SC, Chang SJ, Chung CC, Chen YS, Chang CH. Berberine-loaded targeted nanoparticles as specific Helicobacter pylori eradication therapy: in vitro and in vivo study. Nanomedicine. 2015;10(1):57–71. doi:10.2217/nnm.14.76. PMCID: 25177920.
- Arif M, Sharaf M, Samreen K, Chi S, Liu Z. Chitosan-based nanoparticles as delivery-carrier for promising antimicrobial glycolipid biosurfactant to improve the eradication rate of Helicobacter pylori biofilm. J Biomater Sci Polym Ed. 2021;32:813–832.
- Pan-In P, Banlunara W, Chaichanawongsaroj N, Wanichwecharungruang S. Ethyl cellulose nanoparticles: clarithomycin encapsulation and eradication of H. pylori. Carbohydr Polym. 2014;109:22–27. doi:10.1016/j.carbpol.2014.03.025
- Pippa N, Gazouli M, Pispas S. Recent advances and future perspectives in polymer-based nanovaccines. Vaccines. 2021;9(6):558. doi:10.3390/vaccines9060558
- Chehelgerdi M, Doosti A. Effect of the cagW-based gene vaccine on the immunologic properties of BALB/c mouse: an efficient candidate for Helicobacter pylori DNA vaccine. J Nanobiotechnology. 2020;18(1):63. doi:10.1186/s12951-020-00618-1
- Tan Z, Liu W, Liu H, Li C, Zhang Y, Meng X, Tang T, Xi T, Xing Y. Oral Helicobacter pylori vaccine-encapsulated acid-resistant HP55/PLGA nanoparticles promote immune protection. Eur J Pharm Biopharm. 2017;111:33–43. doi:10.1016/j.ejpb.2016.11.007
- Song H, Lv X, Yang J, Liu W, Yang H, Xi T, Xing Y. A novel chimeric flagellum fused with the multi-epitope vaccine CTB-UE prevents Helicobacter pylori-induced gastric cancer in a BALB/c mouse model. Appl Microbiol Biotechnol. 2015;99(22):9495–9502. doi:10.1007/s00253-015-6705-z
- Fallen MJ, Clayton CL. Vaccination against Helicobacter pylori urease. Lancet. 1990;336(8708):186–187. doi:10.1016/0140-6736(90)91716-N
- Sutton P, Chionh YT. Why can’t we make an effective vaccine against Helicobacter pylori ? Expert Rev Vaccines. 2013;12(4):433–441. doi:10.1586/erv.13.20
- Milani M, Sharifi Y, Rahmati-Yamchi M, Somi MH, Akbarzadeh A. Immunology and vaccines and nanovaccines for Helicobacter pylori infection. Expert Rev Vaccines. 2015;14(6):833–840. doi:10.1586/14760584.2015.1008460
- Arif M. Complete life of cobalt nanoparticles loaded into cross-linked organic polymers: a review. RSC Adv. 2022;12(24):15447–15460. doi:10.1039/D2RA01058E
- Zaidi S, Misba L, Khan AU. Nano-therapeutics: a revolution in infection control in post antibiotic era. Nanomedicine: Nanotechnology, Biology and Medicine. 2017;13(7):2281–2301. doi:10.1016/j.nano.2017.06.015
- Almeida Furquim de Camargo B, Soares Silva DE, Noronha da Silva A, Campos DL, Machado Ribeiro TR, Mieli MJ, Borges Teixeira Zanatta M, Bento da Silva P, Pavan FR, Gallina Moreira C, et al. New Silver(I) coordination compound loaded into polymeric nanoparticles as a strategy to improve in vitro Anti- Helicobacter pylori Activity. Mol Pharm. 2020;17(7):2287–2298. doi:10.1021/acs.molpharmaceut.9b01264
- Thamphiwatana S, Fu V, Zhu J, Lu D, Gao W, Zhang L. Nanoparticle-stabilized liposomes for pH-responsive gastric drug delivery. Langmuir. 2013;29(39):12228–12233. doi:10.1021/la402695c
- Shahid M, Farooqi ZH, Begum R, Arif M, Wu W, Irfan A. Hybrid microgels for catalytic and photocatalytic removal of nitroarenes and organic dyes from aqueous medium: a review. Crit Rev Anal Chem. 2020;50(6):513–537. doi:10.1080/10408347.2019.1663148
- Arif M, Farooqi ZH, Irfan A, Begum R. Gold nanoparticles and polymer microgels: last five years of their happy and successful marriage. J Mol Liq. 2021;336:116270. doi:10.1016/j.molliq.2021.116270
- Lin Z, Gao C, Wang D, He Q. Bubble-Propelled janus gallium/zinc micromotors for the active treatment of bacterial infections. Angewandte Chemie International Edition. 2021;60(16):8750–8754. doi:10.1002/anie.202016260
- Saravanan M, Gopinath V, Chaurasia MK, Syed A, Ameen F, Purushothaman N. Green synthesis of anisotropic zinc oxide nanoparticles with antibacterial and cytofriendly properties. Microb Pathog. 2018;115:57–63. doi:10.1016/j.micpath.2017.12.039
- Chakraborti S, Bhattacharya S, Chowdhury R, Chakrabarti P, Webber MA. The molecular basis of inactivation of metronidazole-resistant Helicobacter pylori using polyethyleneimine functionalized zinc oxide nanoparticles. PloS one. 2013;8(8):e70776. doi:10.1371/journal.pone.0070776
- Bhoyar PK, Morani DO, Biyani DM, Umekar MJ, Mahure JG, Amgaonkar YM. Encapsulation of naproxen in lipid-based matrix microspheres: characterization and release kinetics. J youn pharmac. 2011;3(2):105–111. doi:10.4103/0975-1483.80293
- Jóhannesson G, Stefánsson E, Loftsson T. Microspheres and nanotechnology for drug delivery. Dev Ophthalmol. 2016;55:93–103.
- Uyen NTT, Hamid ZAA, Tram NXT, Ahmad N. Fabrication of alginate microspheres for drug delivery: a review. Int J Biol Macromol. 2020;153:1035–1046. doi:10.1016/j.ijbiomac.2019.10.233
- Tripathi GK, Singh S, Nath G, Dubey RK. Evaluation of pH triggers in situ porous controlled release micro balloon delivery of amoxicillin for eradication of Helicobacter pylori. <![CDATA[Current Drug Delivery]]>. 2011;8(6):667–677. doi:10.2174/156720111797635531
- Zheng KK, Tong Y, Zhang SH, He R, Xiao L, Iqbal Z, Zhang Y, Gao J, Zhang L, Jiang L, et al. Flexible bicolorimetric polyacrylamide/chitosan hydrogels for smart real-time monitoring and promotion of wound healing. Adv Funct Mater. 2021;31(34):2102599. doi:10.1002/adfm.202102599
- Gonçalves IC, Magalhães A, Fernandes M, Rodrigues IV, Reis CA, Martins MC. Bacterial-binding chitosan microspheres for gastric infection treatment and prevention. Acta biomaterialia. 2013;9(12):9370–9378. doi:10.1016/j.actbio.2013.07.034
- Adebisi AO, Conway BR. Lectin-conjugated microspheres for eradication of Helicobacter pylori infection and interaction with mucus. Int J Pharm. 2014;470(1–2):28–40. doi:10.1016/j.ijpharm.2014.04.070
- Gonçalves IC, Henriques PC, Seabra CL, Martins MC. The potential utility of chitosan micro/nanoparticles in the treatment of gastric infection. Expert Rev Anti Infect Ther. 2014;12(8):981–992. doi:10.1586/14787210.2014.930663
- Henriques PC, Costa LM, Seabra CL, Antunes B, Silva-Carvalho R, Junqueira-Neto S, Maia AF, Oliveira P, Magalhães A, Reis CA, et al. Orally administrated chitosan microspheres bind Helicobacter pylori and decrease gastric infection in mice. Acta biomaterialia. 2020;114:206–220. doi:10.1016/j.actbio.2020.06.035
- Cui L, Yao Y, Yim EKF. The effects of surface topography modification on hydrogel properties. APL Bioengineering. 2021;5(3):031509. doi:10.1063/5.0046076
- Park J, Kim D. Release behavior of amoxicillin from glycol chitosan superporous hydrogels. Journal of Biomaterials Science, Polymer Edition. 2009;20(5–6):853–862. doi:10.1163/156856209X415855
- Mohamed NA, Abd El-Ghany NA, Abdel-Aziz MM. Synthesis, characterization, anti-inflammatory and anti-Helicobacter pylori activities of novel benzophenone tetracarboxylimide benzoyl thiourea cross-linked chitosan hydrogels. Int J Biol Macromol. 2021;181:420–429. doi:10.1016/j.ijbiomac.2021.04.095
- Moogooee M, Ramezanzadeh H, Jasoori S, Omidi Y, Davaran S. Synthesis and in vitro studies of cross-linked hydrogel nanoparticles containing amoxicillin. J Pharm Sci. 2011;100(3):1353–1367. doi:10.1002/jps.22351
- El-Mahrouk GM, Aboul-Einien MH, Makhlouf AID. Design, optimization, and evaluation of a novel metronidazole-loaded gastro-retentive pH-Sensitive Hydrogel. AAPS PharmSciTech. 2016;17(6):1285–1297. doi:10.1208/s12249-015-0467-x
- Chang C-H, Lin Y-H, Yeh C-L, Chen Y-C, Chiou S-F, Hsu Y-M, Chen Y-S, Wang -C-C. Nanoparticles incorporated in pH-sensitive hydrogels as amoxicillin delivery for eradication of Helicobacter pylori. Biomacromolecules. 2010;11(1):133–142. doi:10.1021/bm900985h
- Silva K, de Carvalho DÉL, Valente VMM, Campos Rubio JC, Faria PE, Silva-Caldeira PP. Concomitant and controlled release of furazolidone and bismuth(III) incorporated in a cross-linked sodium alginate-carboxymethyl cellulose hydrogel. Int J Biol Macromol. 2019;126:359–366. doi:10.1016/j.ijbiomac.2018.12.136
- Merino S, Martín C, Kostarelos K, Prato M, Vázquez E. Nanocomposite hydrogels: 3D polymer-nanoparticle synergies for on-demand drug delivery. ACS nano. 2015;9:4686–4697.
- Zhao X, Chen X, Yuk H, Lin S, Liu X, Parada G. Soft materials by design: unconventional polymer networks give extreme properties. Chem Rev. 2021;121:4309–4372.
- Narayanaswamy R, Torchilin VP. Hydrogels and their applications in targeted drug delivery. Molecules. 2019;24(3):603
- Huang P, Wang X, Liang X, Yang J, Zhang C, KongWang D, Wang W. Nano-, micro-, and macroscale drug delivery systems for cancer immunotherapy. Acta biomaterialia. 2019;85:1–26. doi:10.1016/j.actbio.2018.12.028. PMCID: 30579043.