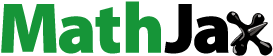
ABSTRACT
In recent years, it has become clear that gut microbiota plays a major role in the human body, both in health and disease. Because of that, the gut microbiome and its impact on human well-being are getting wider and wider attention. Studies focused on the liver are not an exception. However, the majority of the analyses are concentrated on the bacterial part of the gut microbiota, while the fungi living in the human intestines are often omitted or underappreciated. This review is focused on the gut mycobiome as an important factor that should be taken into consideration regarding liver homeostasis and its perturbations. We have collected the findings in this field and we discuss their importance. We aim to emphasize the fungal compositional changes related to liver diseases and, by that, provide novel insights into the directions of liver research and gut microbiota as a therapeutic target for liver diseases.
Introduction
The human gut harbors a flourishing variety of organisms belonging to all kingdoms of life.Citation1 The most numerous and well-studied are bacteria, but the others, less abundant, such as archaea, viruses, protozoa, and fungi, also require attention. In recent years, with the advent of metagenomics studies, it has become clear that this complex internal ecosystem called the gut microbiota is not just a by-passenger but is of extreme importance to human health and maintaining proper homeostasis. Disruption of the normal gut microbiota can have serious health consequences and lead to the development or acceleration of disease progression.Citation2 Noticeably, this concerns not only the pathophysiology of intestinal but also extraintestinal diseases. Taking this into account, it is not surprising that the gut microbiome and its impact on human well-being are getting wider and wider attention.
The human gut microbiota has been extensively studied in relation to many host factors such as ageCitation3,Citation4, genderCitation5,Citation6, dietCitation7,Citation8, lifestyleCitation9,Citation10, and diseases, such as obesityCitation11–13, diabetesCitation14,Citation15, rheumatoid arthritisCitation16,Citation17, autism spectrum disorderCitation18–20, and a variety of cancersCitation21,Citation22. Liver diseases are also on the list, which is not surprising, taking into account the global burden of liver diseases, accounting for approximately 2 million deaths each year.Citation23 Recent interest in the relationship between liver disease and gut microbiota revealed that gut microbiota structure changes in various liver diseases, and there is a crosstalk between gut microbiota and the liver.Citation24,Citation25 The bidirectional communication between them is provided by the biliary system and the portal vein widely known as the gut-liver axis.Citation26
One of the most well-known hypotheses related to the role of the microbiome in liver health and diseases is the so-called leaky gut hypothesis. It assumes that the increased intestinal permeability resulting from an inflamed gut causes the translocation of microbes or microbial components and products (e.g., toxins) to the portal-venous system, further leading to harmful liver inflammation or directly damaging liver structure.Citation27,Citation28
So far, most research, including liver diseases, has focused on the bacterial part of the gut microbiota. Only recently, it has been recognized that gut fungi, known as mycobiome, are also essential members of the gut microbiota.Citation29–31 This fact might seem astonishing, as intestinal fungi constitute only less than 1% of the species that can be found in the human gut.Citation32 Yet, the researchers estimate that of the~1013 microorganisms in the human gastrointestinal tractCitation33, about a billion compose the gut mycobiota.Citation34 The majority of them belong to the Ascomycota and Basidiomycota phylaCitation35,Citation36, and Saccharomyces, Candida, and Malassezia are among the most abundant fungal genera, probably constituting the core mycobiota.Citation35,Citation37,Citation38 Like intestinal bacteria, some gut fungi are beneficial, while others may be harmful. The latter also concerns fungi commonly found in the human gut, e.g., Candida albicans, an opportunistic pathogen that, when out of control, may cause deadly candidiasis.Citation39,Citation40
Regarding the emerging role of the gut mycobiome in liver diseases, this review is focused on the gut mycobiome as a vital factor for maintaining liver homeostasis and understanding liver disease pathogenesis. Our goal is to highlight the compositional changes associated with liver diseases and, by that, provide novel insights into the directions of liver research and gut microbiota as a therapeutic target for liver diseases. We also provide a summary of the alterations of mycobiome in liver diseases ().
Table 1. Summary of the alterations of mycobiome in liver diseases.
Alcohol-associated liver disease
Alcohol-associated liver disease (ALD) is a common disease caused by the overuse of alcohol that leads to liver steatosis, alcohol-associated hepatitis, cirrhosis, and potentially hepatocellular carcinoma (HCC).Citation41 ALD is a severe global problem as it has been estimated that 60–80% of liver-related deaths can be attributed to excessive alcohol consumption. Although alcohol-mediated reactive oxygen species formation and hepatic inflammation are the main drivers of ALD developmentCitation42, ALD has also been recently linked to the changes in the gut mycobiome structure. One of the studies showed that germ-free mice obtaining intestinal microbiota from patients with alcohol hepatitis had more severe liver inflammation and disruption of the intestinal integrity in response to alcohol. Contrary to that, mice receiving microbiota from patients without alcoholic hepatitis were able to reverse the alcohol-caused liver injuries.Citation43 Even though bacteria had been the main point of interest, there are also some interesting reports regarding the gut mycobiota changes and the role of fungi in ALD.
Studies of mice under chronic ethanol administration showed overgrowth of intestinal fungi and increased plasma levels of β-glucan, the main component of the fungal cell walls.Citation44 Reducing an increase in intestinal fungi by antifungal drugs alleviated the symptoms of ethanol-induced liver disease. The possible mechanism of liver damage by fungi includes induction of liver inflammation by β-glucan via the C-type lectin-like receptor CLEC7A on Kupffer cells and possibly other bone marrow-derived cells. This process leads to the upregulation of the inflammatory cytokine IL-1βCitation45, which leads to hepatocyte damage and promotes the development of ethanol-induced liver disease. Moreover, it has been shown that blocking IL-1β prevents alcoholic steatohepatitis in miceCitation46, suggesting possible routes for therapeutic intervention for attenuation of alcohol-related liver disease. However, water-insoluble polysaccharide, a 1,3-β-glucan from Wolfporia cocos, an edible mushroom used in Chinese traditional medicine for over 2000 yearsCitation47, reduces the hepatic inflammatory injury and steatosis in mice with alcoholic hepatic steatosis.Citation48 This observation suggests the protective effect of 1,3-β-glucan in liver diseases, but the mechanism for this action needs further study.
At the gut mycobiome compositional level, patients with alcohol use disorder (AUD) had a significantly increased abundance of the Candida, Debaryomyces, Pichia, Kluyveromyces, Issatchenkia, and Scopulariopsis genera, and C. albicans, Candida zeylanoides, Issatchenkia orientalis, and Scopulariopsis cordiae species compared with a control group.Citation49 Notably, short abstinence periods of 2 weeks were associated with a significantly lower abundance of fungi belonging to Candida, Malassezia, Pichia, Kluyveromyces, Issatchenkia, Claviceps, Cyberlindnera, and Hanseniaspora, as well as C. albicans, C. zeylanoides, I. orientalis, and Cyberlindnera jadinii. These changes were accompanied by significantly higher specific anti-C. albicans immunoglobulin G (IgG) and M (IgM) serum levels in AUD subjects compared to control participants and significantly decreased anti-C. albicans IgG levels in the AUD group after two weeks of abstinence. Moreover, a higher level of Malassezia fungi was observed in AUD with the progressive liver disease compared with non-progressive liver disease. There were also some opposite changes, as an abundance of fungi belonging to the Aspergillus genus and Kazachstania humilis species was significantly decreased in patients with AUD relative to controls.
The overgrowth of C. albicans has been observed in ALD subjects compared with nonalcoholic controls also in other studiesCitation44,Citation50,Citation51, suggesting that there might be a real association between C. albicans and ALD. It has been shown that dysbiosis-related exotoxins, such as candidalysin from C. albicans, directly cause hepatocyte death and liver injury, and it is also associated with liver disease severity and mortality in patients with alcoholic hepatitis.Citation50
Another study has shown the increase of the commensal fungus Meyerozyma guilliermondii in the feces of mice with alcoholic hepatic steatosis (AHS).Citation48 Of notice, the inoculation of M. guilliermondii into fungi-free mice worsened the features of AHS. M. guilliermondii has been shown to generate prostaglandin E2 (PGE2) by biotransformation of arachidonic acid and the gut fungi (M. guilliermondii)-induced PGE2 production in the liver was confirmed as one of the mechanisms in the chronic AHS.
It should be emphasized that even considering the reported association between altered gut mycobiota in ALD, determining whether these changes are connected rather to ALD or chronic alcohol uptake is extremely hard. It is also hard to distinguish between the cause and the effects. After all, fungi are present in many types of alcohol as they are widely used in alcohol production, and the altered gut mycobiota in ALD subjects may be just the effect of alcohol consumption and may not be connected directly to ALD. For example, there is an association between the high prevalence of Saccharomyces and the consumption of yeast-containing foods such as beer and bread.Citation11 Furthermore, even focusing on alcoholism, the mechanisms leading to gut mycobiota dysbiosis in chronic alcohol uptake are unclear. It is possible that fungi are a consequence of a bacterial imbalance in the gut and are simply taking over a niche usually colonized by bacteria. This possibility seems to be supported by the fact that fungal bloom often follows bacteria dysbiosis after antibiotic treatment.Citation52 Answer to the question of why fungi from the Candida genus increase in abundance as a result of alcohol usage may also be relatively trivial, as fungi from this genus are also among the most abundant in the human gutCitation39, meaning that they can also be the first and the best adapted to take advantage. Regardless of these hypotheses, further case-designed studies are necessary to confirm or deny the active role of the gut mycobiome in the ALD progression.
Nonalcoholic fatty liver disease and obesity
Nonalcoholic fatty liver disease is one of the most important causes of liver disease worldwide – its prevalence is estimated to be 24–45%, with a predicted increasing trend.Citation53,Citation54 Moreover, it is also characterized by high mortality, accounting for 23% to 29% of total deaths.Citation55 A growing body of evidence suggests that NAFLD is a multisystem disease and is often considered the liver manifestation of metabolic syndrome.Citation56 NAFLD is also associated with obesity and type 2 diabetes. Although recent research indicates that gut microbiota is one of the factors that come into play in NAFLDCitation57,Citation58, the role of gut fungi is not well studied, and the majority of studies are focused rather on obesity than NAFLD itself.
Studies of lean and obese mice suggest that the gut microbiota affects the energy balance by influencing the efficiency of calorie harvest and how this energy is used and stored.Citation59 Obesity was also significantly correlated with a decrease in fungal diversity at a family level, and a tendency toward increased diversity at other taxonomical levels was also found in nonobese individuals.Citation60 More importantly, obese patients exhibited different fungal compositions compared with people with normal BMI. Among these, obese subjects had a higher amount of fungi belonging to the Ascomycota phylum, Saccharomyces and Tremellomycetes classes, and Dipodascaeae and Sachharomycetaceae families. Moreover, increased Candida, Nakaseomyces, and Penicillium populations were observed in obese individualsCitation11–13, and Candida parapsilosis was recently identified as a critical commensal fungus related to diet-induced obesity in mice.Citation61 Additionally, obese individuals display higher yeast counts.Citation13 On the other hand, the number of fungi belonging to the Zygomycota phylum and Mucor genus was decreased in the obese population compared with individuals with normal weight.Citation60 Notably, this negative correlation was reversed after weight loss.
The study of the association of gut fungi composition with anthropometrical and metabolic parameters related to obesity showed that the relative abundance of some fungi was linked to adiposity and related metabolic disorders, including insulin resistance, dyslipidemia, blood pressure, and inflammatory activity.Citation60 Among the most important, the relative abundance of fungi belonging to the Ascomycota phylum, classes Saccharomycetes, Tremellomycetes, and Cystobasidiomycetes, families Erythrobasidiaceae and Dipodascaceae, and genera Aspergillus, Eurotium and Rhodotorula, increased with the occurrence of metabolic abnormalities. Contrary to that, the relative abundance of fungi belonging to the phylum Zygomycota, classes Agaricomycetes and Eurotiomycetes, families Mucoraceae, Nectriaceae, Ceratocystidaceae, Corticiaceae, Debariomycetaceae and Hypocraceae and genera Mucor, Penicillium, Monilliela and Ceratocystis were correlated with protection from these metabolic disorders. However, even if there are some associations between gut mycobiota and obesity, it is still unclear whether changes in fungal intestinal structure contribute to obesity development or are just an effect of different dietary habits leading to excessive weight, such as high carbon or high-fat consumption. Mice fed with a diet rich in fats exhibited a higher abundance of Alternaria, Saccharomyces, Septoriella, and Tilletiopsis genera, as well as Saccharomyces cerevisiae and Tilletiopsis washingtonensis species.Citation62
The finding that obesity is associated with systemic inflammation, and the suggestion that the obesity-related fungal intestinal composition has a proinflammatory effectCitation63, is particularly interesting in the context of the NAFLD. One of the questions that is still unanswered is whether altered microbiota contributes to liver inflammation in NAFLD. Research shows that chronic inflammatory disorders are associated with the disruption of the intestinal epithelial barrier, which, when intact, protects the host from bacterial invasion. Moreover, the gut microbiome may play a critical role in maintaining this intestinal epithelial barrier.Citation64 The proinflammatory effect associated with obesity-related mycobiota might be one of the causes of the leaky intestinal barrier, further leading to liver inflammation.
The research devoted to gut fungal microbiota in NAFLD subjects showed that the relative abundances of Talaromyces, Paraphaeosphaeria, Lycoperdon, Curvularia, Phialemoniopsis, Paraboeremia, Sarcinomyces, Cladophialophora, and Sordaria were higher in patients with NAFLD. In contrast, the abundances of Leptosphaeria, Pseudopithomyces, and Fusicolla were decreased.Citation65 What is more, patients with NAFLD displayed more co-occurring fungal intrakingdom correlations. The richness and evenness of fungal microbiota were increased in patients with nonalcoholic steatohepatitis (NASH) compared with patients without NASH. The same trend was observed for patients with fibrosis.
Furthermore, several fungi genera exhibited higher abundance in liver injury, lipid metabolism, and the development of NAFLD, e.g., Paramycosphaerella, Fusicolla, Arthrinium, Triparticalcar, Trichoderma, and Cladosporium genera were significantly more abundant in patients with NASH; Cladosporium, Staphylotrichum, Paecilomyces, and Thermomyces fungi were increased in patients with significant fibrosis. On the other hand, Pulvinula, genera with decreased abundance in patients with significant fibrosis, was negatively correlated with the fibrosis stage, Alanine aminotransferase (ALT), and Aspartate aminotransferase (AST). Another study showed that patients with NAFLD and more severe disease stages have a specific composition of fecal fungi and an increased systemic immune response to C. albicans.Citation66 Interestingly, these changes could be observed particularly in patients without obesity, underscoring the previously unreported aspects of the nonobese phenotype of NAFLD. Moreover, in a fecal microbiome-humanized mouse model of Western diet-induced steatohepatitis, treatment with antifungal amphotericin B reduced liver damage. This observation suggests that intestinal fungi may be an attractive therapeutic target to minimize NASH and NAFLD progression. Although, it should be pointed out that amphotericin B interacts with cholesterolCitation67 and may affect Toll-like receptor (TLR) signalingCitation68, which may contribute to its beneficial effects on NAFLD and NASH, taking into account that cholesterol and TLR signaling contribute to NASH and NASH fibrosis.
Primary sclerosis cholangitis (PSC)
PCS is a chronic liver disease manifested by the biliary tree’s progressive sclerosis (scarring). It is associated with inflammation of the bile ducts that leads to periductal fibrosis, destruction of the bile ducts, and consequently, biliary cirrhosis and liver failure.Citation69 Of notice, approximately 75% of patients with PSC also have inflammatory bowel disease (IBD), while only about 7%–8% of patients with IBD have PSC.Citation70 On the one hand, the strong association of PSC with IBD seems to support the hypothesis that PSC has an autoimmune etiology. On the other hand, some authors indicate that PSC cannot be considered a classical autoimmune disease, as it occurs with a 2:1 male predominance and lacks a characteristic response to immunosuppressants.Citation71,Citation72 Interestingly, the changes in the gut microbiome, including fungi, might contribute to PSC etiology.
One of the general concepts of damaging bile ducts includes the leaky gut hypothesis, but there are also some other more detailed hints that suggest the role of fungi in the pathogenesis of primary sclerosis cholangitis. Genetic variants of CARD9, a protein involved in the innate immunity against fungi, cause susceptibility to PSC and IBD.Citation24 Furthermore, the anti-S. cerevisiae antibodies (ASCA) are highly prevalent in subjects with PSCCitation73, and the presence of fungi belonging to the Candida genus in bile was associated with reduced survival in PSC patients.Citation74 Fungi belonging to Candida genus have the potential to induce Th17 response in T cellsCitation75, and increased Th17 levels have been observed in PSC patients and recently been suggested to be involved in PSC pathogenesis.Citation76
Also, Lemoinne et al.Citation77 indicated that patients with PSC displayed fungal gut dysbiosis at various levels. Although in their study, there were no differences in mycobiota alpha diversity (Shannon and Chao1 indexes) among healthy subjects, patients with IBD only, PSC and IBD, and PSC only, they showed that samples clustered according to groups by beta diversity analysis. Of notice, PSC subjects had an increased proportion of fungi belonging to the Sordariomycetes class, and Exophiala genus, and a decreased proportion of S. cerevisiae. The putative positive effect of S. cerevisiae on the biliary tracts seems to be supported by the fact that S. cerevisiae level was also decreased in patients with active IBD, and it was shown to have anti-inflammatory effects by producing cytokine interleukin 10.Citation78 A similar study performed on the other cohort revealed increased levels of the genera Candida and Humicola (species level annotation suggests H. grisea) in PCS subjects.Citation79 As H. grisea, recently reclassified as Trichocladium griseum, belongs to the Sordiomycetes class, this finding supports the results of Lemoinne et al.Citation77 at increased taxonomic resolution.
Also, the negative impact of Exophiala on the biliary ducts seems to be supported by other studies. Fungi belonging to the Exophiala genus have been connected to infections known as phaeohyphomycosis in hosts with an impaired immune system. Although most of these infections affect the skin, Exophiala is also able to cause systemic infections.Citation80 Particularly, one case report described a systemic infection by Exophiala dermatitidis mimicking PSC in a patient without immunodeficiency.Citation81 Another case report found that E. dermatitidis infection leads to an end-stage liver disease characterized by cholestasis and dilatation of intrahepatic bile ducts.Citation82
Moreover, Lemoinne et al. observed a relative increase in biodiversity, such as patients with PSC had a higher ITS2/16S diversity ratio than IBD-only patients, suggesting an increased fungi-to-bacteria diversity ratio. What is more, the gut microbiota of patients with PSC exhibited a strong disruption in the bacteria-fungi correlation network compared with patients with IBD and healthy subjects, indicating an alteration in the interkingdom crosstalk.
Cirrhosis
Cirrhosis is a late stage of liver scarring caused by chronic liver injury.Citation83 It may be caused by many diseases, e.g., hepatitis, fatty liver disease, and chronic alcohol consumption.Citation84 Injury of the liver leads to the formation of scar tissue called fibrosis. As a result, the liver is progressively damaged and cannot function properly. In advanced stages, cirrhosis leads to a life-threatening condition. In fact, cirrhosis is a leading cause of death worldwide.Citation85 Interestingly, intestinal infections are one of the leading causes of mortality.Citation86
The relationship between intestinal infections and cirrhosis has been investigated mainly for bacteria. Nevertheless, the role of fungi has been gaining attention recently. One study showed that a combined bacterial-fungal dysbiosis metric, Bacteroidetes/Ascomycota, changes with cirrhosis severity.Citation87 Moreover, a lower Bacteroidetes/Ascomycota ratio was associated with lower hospitalizations, and this metric could predict 90-day hospitalizations in patients with cirrhosis. Notably, this prediction was independent of disease severity, encephalopathy, and clinical biomarkers. Fungal diversity was decreased in subjects with cirrhosis compared to healthy controls and inversely correlated with a model for end-stage liver disease (MELD) score. Antibiotics lowered the bacterial and fungal diversity, with the exception of Candida, for which a higher abundance was observed. Omeprazole, a proton pump inhibitor, changed bacterial diversity but did not affect the fungal metrics. C. albicans has also been detected in about 20% of fecal samples of cirrhotic subjects during rifaximin treatment.Citation88
Contrary to the abovementioned results, patients who developed cirrhosis due to chronic hepatitis B virus (HBV) infection had increased fungal abundance and fungal diversity compared with patients with chronic hepatitis B, HBV carriers, and healthy controls.Citation89 Moreover, certain fungal species were detected in higher abundance in HBV-associated cirrhosis, such as Aspergillus, Candida, Galactomyces, Saccharomyces, and Chaetomium. The copies of target DNA for C. albicans, C. parapsilosis, and C. krusei were significantly increased in HBV-associated liver cirrhosis subjects compared to HBV carriers and healthy volunteers.Citation90 What is more, HBV-associated cirrhosis patients had a significantly increased prevalence of C. parapsilosis, C. glabrata, C. tropicalis, and S. cerevisiae than healthy volunteers. The increased abundances of Saccharomyces and Candida in HBV-associated cirrhosis were also confirmed by other studies.Citation91,Citation92 Another study identified liver cirrhosis as an independent risk factor for esophageal candidiasis via multivariate logistic regression analysis.Citation93 However, in our opinion, the question remains whether the cause was not misplaced with the consequence in this case, as it seems equally probable that esophageal candidiasis predisposes to liver cirrhosis, and this type of statistical analysis shows only correlations, not causality.
In light of the abovementioned research, it is worth mentioning that infection with Candida is one of the major threats for patients with cirrhosis, with a reported mortality rate of 55% associated with invasive candidiasis in patients with cirrhosis.Citation94 The odds of mortality in cirrhosis patients having invasive candidiasis are 4.4 times higher than those without invasive candidiasis.
Hepatocellular carcinoma
Hepatocellular carcinoma (HCC) is the most common form of liver cancer. It is also one of the leading causes of cancer-related deaths worldwideCitation95, and its incidence is estimated to cross annually 1 million cases by 2025.Citation96 The pathogenesis of HCC is multifactorialCitation97, and multiple risk factors have been identified, with HBV, HCV, and NASH at the top of them. In general, the gut microbiome of HCC patients differed from that of healthy subjects, and what is more, the intestinal microbiome profile showed a correlation with different etiologies.Citation98,Citation99 However, the contribution of gut fungi to this image has been poorly investigated, concentrated on the role of the fungal metabolites in the development of HCC.
For example, aflatoxin B1 (AFB1), a metabolite of several species from the Aspergillus genus, e.g., Aspergillus flavus and Aspergillus parasiticus, has been shown to contribute to HCC development.Citation100,Citation101 Aflatoxin-B1 is converted by members of the cytochrome p450 family into highly reactive intermediates that bind to DNA in hepatic cells, forming promutagenic adducts. The adducts interact with guanine bases of DNA and cause an arginine to serine mutation at codon 249 of the Tp53 tumor suppressor gene, leading to hepatocarcinogenesis.Citation102,Citation103 There is also a high correlation between HBV, another risk factor for HCC, and aflatoxin B1, as both of these factors are frequent in populations with a high incidence of HCC. For example, a prospective China-based population study revealed that the interaction between AFB1 and HBV infection increased the risk of HCC 60-fold.Citation104 These findings may suggest that HBV and aflatoxin B1 interact, increasing the risk of HCC. The observations showed that patients chronically infected with HBV have higher concentrations of AFB1 adducts than uninfected individuals.Citation105,Citation106 The binding of AFB1 metabolites to DNA may increase the risk of integration of viral DNA and malignant transformation as a result.Citation107 Integrating the HBV x gene into the host cells inhibits nuclear excision repair responsible for removing AFB1-DNA adducts, favoring the persistence of mutations.Citation108,Citation109 HBV x, in cooperation with p53, may also contribute to uncontrolled cell proliferation.Citation108,Citation110 Another possibility is that increased hepatocyte necrosis and proliferation caused by HBV infection increase the likelihood of AFB1 mutations, including 249ser, and the subsequent clonal expansion of cells containing these mutations.Citation111
At the level of mycobiome, one research has shown that patients with HCC had significantly decreased gut mycobiome diversity and increased abundance of C. albicans compared to those with liver cirrhosis.Citation112 At the family level, patients with HCC showed a decreased abundance of Myxotrichaceae, Debaryomycetaceae, Trichomonascaceae, and Saccharomycetaceae, and an increased abundance of Saccharomycetales fam Incertae sedis. At the class level, a lower abundance of Leotiomycetes was observed than in liver cirrhosis, and at the genus level, Kazachstania, Debaryomyces, Xeromyces, Amorphotheca, and Blastobotrys were less enriched, whereas Candida was overrepresented. Interestingly, mice fed with C. albicans exhibited increased tumor size and body weight. This observation may suggest that abnormal colonization by C. albicans contributes to the growth of liver tumors.
Moreover, further analysis revealed 46 upregulated and 70 downregulated metabolites in the blood plasma of C. albicans-gavaged mice compared to control groups, suggesting that C. albicans can also change plasma metabolism, involving metabolites and the corresponding signaling pathway. Among the top upregulated metabolites in HCC subjects were L-carnitine and L-acetylcarnitine, which concentrations were previously reported by other studies to differentiate HCC patients from those with liver diseases or health controls.Citation113,Citation114 The link between L-carnitine and L-acetylcarnitine and HCC is not clear. On the one hand, an increase in these metabolites may be the result of the boosted requirement of energy consumption in HCC patients, which results in long-chain acylcarnitines accumulation and activation of the carnitine shuttle system for oxidation of long-chain fatty acids to supply more usable energy.Citation112 On the other hand, it has been shown that an administration of L-carnitine in mice can prevent the progression of nonalcoholic steatohepatitis and inhibit liver carcinogenesis by suppressing oxidative stress and inflammation in the liver.Citation115
Moreover, experiments also showed that NLRP6, which regulates host defense against microbesCitation116,Citation117, is necessary for promoting HCC caused by the abnormal colonization of C. albicans. This finding could be essential for providing new targets for the treatment of HCC.
Fungi as probiotics
S. cerevisiae var. boulardii has been used for treating various gut-related diseases.Citation118 It has been shown to change the gut microbiome and, more importantly, attenuate acute liver injury, hepatic steatosis, and low-grade inflammation.Citation119,Citation120 For example, Saccharomyces boulardii is used in clinical practice as a probiotic, presenting therapeutically important pathways for using gut mycobiota in NAFLD prevention and treatment. Research shows that in mice with diet-induced diabetes and obesity, oral administration of S. boulardii daily for four weeks resulted in body weight, fat mass, hepatic steatosis, and inflammation reduction. This was accompanied by increased cecum weight and changes in gut microbiota composition at the phylum, family, and genus levels.Citation120 In rats fed with a high-fat diet, oral gavage of S. boulardii (7.5×109 CFU/kg/d) for eight weeks resulted in reductions of body weight, liver mass, liver index, hepatic steatosis, endotoxemia, and inflammation. S. boulardii can also adjust the proportion of Escherichia coli and Bacteroides in the intestine of NAFLD rats.Citation121
Agaricus bisporus Citation122–124 and Pleurotus ostreatus Citation125, two types of macrofungi, also possess hepato-protective activity. Polysaccharide components of these fungi, among others, lowered ALT and AST concentrations in serum in a dose-dependent manner, reduced hepatocellular degeneration, necrosis, inflammatory infiltration, and CCl4-induced liver injury, as well as enhanced the antioxidant status, and improved lipid metabolism.
Other possible beneficial fungi such as Hanseniaspora osmophila and Lachancea thermotolerans Citation126 have not been validated in liver diseases. However, it should also be noted that Clostridium difficile colitis and neutropenic patients had S. cerevisiae fungemia after using S. boulardii as a probiotic.Citation127,Citation128 This observation shows that potential fungal probiotics should be treated with caution, especially in the case of immunocompromised patients.
Conclusions
The research provides ample evidence that the intestinal mycobiota may impact the functioning of the liver and may play an essential role in the pathogenesis of the liver disease. Even though it may not always be clear whether the changes in the intestinal mycobiota are the cause of lesions in the liver or the result of other factors that damage the liver, there is no doubt that a balanced gut mycobiome contributes to the maintenance of the host immune homeostasis. Research into intestinal mycobiota and knowledge of the interplay between mycobiota and liver promote a better understanding of the pathogenesis of liver disease and thus allow for the development of new pathways for diagnosis, treatment, and prognosis for patients with liver disease.
From the role of the intestinal barrier, through specific metabolic pathways and mediators, to interactions between bacteria and fungi, the research on the intestinal microbiome shows a complicated picture of the relationship between fungi living in the intestine and liver dysfunction. Given the mounting evidence of bilateral interaction between the gut and liver, there is also no doubt that intestinal mycobiota research is required. However, even more is needed to transfer the scientific findings on the ground of well-planned trials, which then have a chance to turn into effective therapies. It is worth mentioning here that when considering therapies targeting intestinal mycobiome, e.g., antifungal therapies, it would be important to define the specific sub-groups of patients that would mostly benefit from antifungal therapy given the diversity of mycobiome in the heterogeneous human population. This approach fits in with the assumptions of precision medicine – an up-and-coming and extensively developing field of research and healthcare.
Whether we would like to use mycobiome as a diagnostic instrument, prognostic marker, treatment tool, e.g., fecal transplant or probiotics, or direct object of therapy in the case of harmful fungal strains, to make this possible, more large-scale, longer-term, longitudinal studies are necessary. The planned research should also be accompanied by joint analyses on gut mycobiome with other methods, such as metagenomics and metabolomics, as only this kind of comprehensive approach has the potential to deliver as complete picture as possible of the interplay between microbiota and the host.
List of abbreviations
ALD | = | Alcohol-associated liver disease |
ALT | = | Alanine aminotransferase |
AST | = | Aspartate aminotransferase |
AUD | = | Alcohol use disorder |
HCC | = | Hepatocellular carcinoma |
IBD | = | Inflammatory bowel disease |
IL | = | Interleukin |
ITS | = | Internal transcribed spacer |
NAFLD | = | Nonalcoholic fatty liver disease |
NASH | = | Nonalcoholic steatohepatitis |
PSC | = | Primary sclerosis cholangitis |
Author contributions
Szostak N provided the input and wrote the manuscript; Figlerowicz M discussed the content; Philips A designed the outline, discussed the content, coordinated and participated in manuscript writing.
Disclosure statement
No potential conflict of interest was reported by the authors.
Additional information
Funding
References
- Norman JM, Handley SA, Virgin HW. Kingdom-agnostic metagenomics and the importance of complete characterization of enteric microbial communities. Gastroenterology. 2014;146:1459–15.
- Wang B, Yao M, Lv L, Ling Z, Li L. The human microbiota in health and disease. Engineering. 2017;3:71–82.
- Koenig JE, Spor A, Scalfone N, Fricker AD, Stombaugh J, Knight R, Angenent LT, Ley RE. Succession of microbial consortia in the developing infant gut microbiome. Proc Natl Acad Sci U S A. 2011;108:4578–4585.
- Yatsunenko T, Rey FE, Manary MJ, Trehan I, Dominguez-Bello MG, Contreras M, Magris M, Hidalgo G, Baldassano RN, Anokhin AP, et al. Human gut microbiome viewed across age and geography. Nature. 2012;486(7402):222–227.
- Kim YS, Unno T, Kim B-Y, Park M-S. Sex differences in gut microbiota. World J Mens Health. 2020;38:48.
- Valeri F, Endres K. How biological sex of the host shapes its gut microbiota. Front Neuroendocrinol. 2021;61:100912.
- Wu GD, Chen J, Hoffmann C, Bittinger K, Chen YY, Keilbaugh SA, Bewtra M, Knights D, Walters WA, Knight R, et al. Linking long-term dietary patterns with gut microbial enterotypes. Science. 2011;334(6052):105–108.
- Claesson MJ, Jeffery IB, Conde S, Power SE, O’Connor EM, Cusack S, Harris HMB, Coakley M, Lakshminarayanan B, O’Sullivan O, et al. Gut microbiota composition correlates with diet and health in the elderly. Nature. 2012;488(7410):178–184.
- Walker RL, Vlamakis H, Lee JWJ, Besse LA, Xanthakis V, Vasan RS, Shaw SY, Xavier RJ. Population study of the gut microbiome: associations with diet, lifestyle, and cardiometabolic disease. Genome Med. 2021;13:188.
- Zhong H, Penders J, Shi Z, Ren H, Cai K, Fang C, Ding Q, Thijs C, Blaak EE, Stehouwer CDA, et al. Impact of early events and lifestyle on the gut microbiota and metabolic phenotypes in young school-age children. Microbiome. 2019;7(1):2.
- Hoffmann C, Dollive S, Grunberg S, Chen J, Li H, Wu GD, Lewis JD, Bushman FD. Archaea and fungi of the human gut microbiome: correlations with diet and bacterial residents. PLos One. 2013;8:e66019.
- Morales P, Brignardello J, Gotteland M. The association of intestinal microbiota with obesity. Rev Med Chil. 2010;138:1020–1027.
- Borges FM, de Paula TO, Sarmiento MRA, de Oliveira MG, Pereira MLM, Toledo IV, Nascimento TC, Ferreira-Machado AB, Silva VL, Diniz CG. Fungal diversity of human gut microbiota among eutrophic, overweight, and obese individuals based on aerobic culture-dependent approach. Curr Microbiol. 2018;75:726–735.
- Li WZ, Stirling K, Yang JJ, Zhang L. Gut microbiota and diabetes: from correlation to causality and mechanism. World J Diabetes. 2020;11:293–308.
- Chen Z, Radjabzadeh D, Chen L, Kurilshikov A, Kavousi M, Ahmadizar F, Ikram MA, Uitterlinden AG, Zhernakova A, Fu J, et al. Association of insulin resistance and type 2 diabetes with gut microbial diversity: a microbiome-wide analysis from population studies. JAMA Netw Open. 2021;4(7):e2118811.
- Gupta VK, Cunningham KY, Hur B, Bakshi U, Huang H, Warrington KJ, Taneja V, Myasoedova E, Davis JM, Sung J. Gut microbial determinants of clinically important improvement in patients with rheumatoid arthritis. Genome Med. 2021;13:149.
- Manasson J, Blank RB, Scher JU. The microbiome in rheumatology: where are we and where should we go? Ann Rheum Dis. 2020;79:727–733.
- Fattorusso A, Di Genova L, Dell’isola GB, Mencaroni E, Esposito S. Autism spectrum disorders and the gut microbiota. Nutrients. 2019;11:E521.
- Lou M, Cao A, Jin C, Mi K, Xiong X, Zeng Z, Pan X, Qie J, Qiu S, Niu Y, et al. Deviated and early unsustainable stunted development of gut microbiota in children with autism spectrum disorder. Gut. 2022;71:1588–1599. gutjnl-2021-325115.
- Mulle JG, Sharp WG, Cubells JF. The gut microbiome: a new frontier in autism research. Curr Psychiatry Rep. 2013;15:337.
- Akbar N, Khan NA, Muhammad JS, Siddiqui R. The role of gut microbiome in cancer genesis and cancer prevention. Health Sci Rev. 2022;2:100010.
- Lee KA, Luong MK, Shaw H, Nathan P, Bataille V, Spector TD. The gut microbiome: what the oncologist ought to know. Br J Cancer. 2021;125:1197–1209.
- Asrani SK, Devarbhavi H, Eaton J, Kamath PS. Burden of liver diseases in the world. J Hepatol. 2019;70:151–171.
- Li X, Shen J, Ran Z. Crosstalk between the gut and the liver via susceptibility loci: novel advances in inflammatory bowel disease and autoimmune liver disease. Clin Immunol Orlando Fla. 2017;175:115–123.
- Atif M, Warner S, Oo YH. Linking the gut and liver: crosstalk between regulatory T cells and mucosa-associated invariant T cells. Hepatol Int. 2018;12:305–314.
- Albillos A, de Gottardi A, Rescigno M. The gut-liver axis in liver disease: pathophysiological basis for therapy. J Hepatol. 2020;72:558–577.
- Llorente C, Schnabl B. The Gut Microbiota and Liver Disease. Cell Mol Gastroenterol Hepatol. 2015;1:275–284.
- Ilan Y. Leaky gut and the liver: a role for bacterial translocation in nonalcoholic steatohepatitis. World J Gastroenterol: WJG. 2012;18:2609–2618.
- Zhang L, Zhan H, Xu W, Yan S, Ng SC. The role of gut mycobiome in health and diseases. Ther Adv Gastroenterol. 2021;14:17562848211047130.
- Pérez JC. Fungi of the human gut microbiota: roles and significance. Int J Med Microbiol. 2021;311:151490.
- Wu X, Xia Y, He F, Zhu C, Ren W. Intestinal mycobiota in health and diseases: from a disrupted equilibrium to clinical opportunities. Microbiome. 2021;9:60.
- Nash AK, Auchtung TA, Wong MC, Smith DP, Gesell JR, Ross MC, Stewart CJ, Metcalf GA, Muzny DM, Gibbs RA, et al. The gut mycobiome of the human microbiome project healthy cohort. Microbiome. 2017;5(1):153.
- Sender R, Fuchs S, Milo R. Revised estimates for the number of human and bacteria cells in the body. PLoS Biol. 2016;14:e1002533.
- Auchtung TA, Fofanova TY, Stewart CJ, Nash AK, Wong MC, Gesell JR, Auchtung JM, Ajami NJ, Petrosino JF. Investigating colonization of the healthy adult gastrointestinal tract by fungi. mSphere. 2018;3(2): e00092-18.
- Shuai M, Fu Y, Zhong H-L, Gou W, Jiang Z, Liang Y, Miao Z, Xu J-J, Huynh T, Wahlqvist ML, et al. Mapping the human gut mycobiome in middle-aged and elderly adults: multiomics insights and implications for host metabolic health. Gut. 2022 January;71(9): gutjnl-2021-326298.
- Richard ML, Sokol H. The gut mycobiota: insights into analysis, environmental interactions and role in gastrointestinal diseases. Nat Rev Gastroenterol Hepatol. 2019;16:331–345.
- Hallen-Adams HE, Suhr MJ. Fungi in the healthy human gastrointestinal tract. Virulence. 2017;8:352–358.
- Szóstak N, Handschuh L, Samelak-Czajka A, Tomela K, Schmidt M, Pruss Ł, Milanowska-Zabel K, Kozlowski P, Philips A. Host factors associated with gut mycobiome structure. mSystems. 2023 February;8(2):e0098622.
- Odds FC. Candida infections: an overview. Crit Rev Microbiol. 1987;15:1–5.
- Clancy CJ, Nguyen MH. Systemic Candidiasis: Candidemia and Deep-Organ Infections. In: Calderone RA, Clancy CJ, editors. Candida and Candidiasis. Washington, DC, US: ASM Press; 2012. p. 429–441. doi:10.1128/9781555817176.
- Seitz HK, Bataller R, Cortez-Pinto H, Gao B, Gual A, Lackner C, Mathurin P, Mueller S, Szabo G, Tsukamoto H. Alcoholic liver disease. Nat Rev Dis Primer. 2018;4:16.
- Sheron N. Alcohol and liver disease in Europe–simple measures have the potential to prevent tens of thousands of premature deaths. J Hepatol. 2016;64:957–967.
- Llopis M, Cassard AM, Wrzosek L, Boschat L, Bruneau A, Ferrere G, Puchois V, Martin JC, Lepage P, Le Roy T, et al. Intestinal microbiota contributes to individual susceptibility to alcoholic liver disease. Gut. 2016;65(5):830–839.
- Yang A-M, Inamine T, Hochrath K, Chen P, Wang L, Llorente C, Bluemel S, Hartmann P, Xu J, Koyama Y, et al. Intestinal fungi contribute to development of alcoholic liver disease. J Clin Invest. 2017;127(7):2829–2841.
- Kankkunen P, Teirilä L, Rintahaka J, Alenius H, Wolff H, Matikainen S. (1,3)-β-glucans activate both dectin-1 and NLRP3 inflammasome in human macrophages. J Immunol. 2010;184:6335–6342.
- Petrasek J, Bala S, Csak T, Lippai D, Kodys K, Menashy V, Barrieau M, Min SY, Kurt-Jones EA, Szabo G. IL-1 receptor antagonist ameliorates inflammasome-dependent alcoholic steatohepatitis in mice. J Clin Invest. 2012;122:3476–3489.
- Li X, Ma L, Zhang L. Chapter twelve - molecular basis for poria cocos mushroom polysaccharide used as an antitumor drug in China. In: Zhang L, editor. Progress in molecular biology and translational science. Vol 163. Glycans and glycosaminoglycans as clinical biomarkers and therapeutics - part B. Academic Press; 2019 [Accessed June 27, 2022]. pp. 263–296. https://www.sciencedirect.com/science/article/pii/S1877117319300328.
- Sun S, Wang K, Sun L, Cheng B, Qiao S, Dai H, Shi W, Ma J, Liu H. Therapeutic manipulation of gut microbiota by polysaccharides of wolfiporia cocos reveals the contribution of the gut fungi-induced PGE2 to alcoholic hepatic steatosis. Gut Microbes. 2020;12:1830693.
- Hartmann P, Lang S, Zeng S, Duan Y, Zhang X, Wang Y, Bondareva M, Kruglov A, Fouts DE, Stärkel P, et al. Dynamic changes of the fungal microbiome in alcohol use disorder. Front Physiol. 2021;12:699253.
- Chu H, Duan Y, Lang S, Jiang L, Wang Y, Llorente C, Liu J, Mogavero S, Bosques-Padilla F, Abraldes JG, et al. The candida albicans exotoxin candidalysin promotes alcohol-associated liver disease. J Hepatol. 2020;72(3):391–400.
- Lang S, Duan Y, Liu J, Torralba MG, Kuelbs C, Ventura‐cots M, Abraldes JG, Bosques‐padilla F, Verna EC, Brown RS, et al. Intestinal fungal dysbiosis and systemic immune response to fungi in patients with alcoholic hepatitis. Hepatol Baltim Md. 2020;71(2):522–538.
- Drummond RA, Desai JV, Ricotta EE, Swamydas M, Deming C, Conlan S, Quinones M, Matei-Rascu V, Sherif L, Lecky D, et al. Long-term antibiotic exposure promotes mortality after systemic fungal infection by driving lymphocyte dysfunction and systemic escape of commensal bacteria. Cell Host & Microbe. 2022 May;30(7):1020–1033.e6.
- Younossi Z, Anstee QM, Marietti M, Hardy T, Henry L, Eslam M, George J, Bugianesi E. Global burden of NAFLD and NASH: trends, predictions, risk factors and prevention. Nat Rev Gastroenterol Hepatol. 2018;15:11–20.
- Ma X, Liu S, Zhang J, Dong M, Wang Y, Wang M, Xin Y. Proportion of NAFLD patients with normal ALT value in overall NAFLD patients: a systematic review and meta-analysis. BMC Gastroenterol. 2020;20:10.
- Estes C, Razavi H, Loomba R, Younossi Z, Sanyal AJ. Modeling the epidemic of nonalcoholic fatty liver disease demonstrates an exponential increase in burden of disease. Hepatol Baltim Md. 2018;67:123–133.
- Byrne CD, Targher G. NAFLD: a multisystem disease. J Hepatol. 2015;62:S47–64.
- Puri P, Sanyal AJ. The intestinal microbiome in nonalcoholic fatty liver disease. Clin Liver Dis. 2018;22:121–132.
- Bibbò S, Ianiro G, Dore MP, Simonelli C, Newton EE, Cammarota G. Gut microbiota as a driver of inflammation in nonalcoholic fatty liver disease. Mediators Inflamm. 2018;2018:9321643.
- Turnbaugh PJ, Hamady M, Yatsunenko T, Cantarel BL, Duncan A, Ley RE, Sogin ML, Jones WJ, Roe BA, Affourtit JP, et al. A core gut microbiome in obese and lean twins. Nature. 2009;457:480–484.
- Mar Rodríguez M, Pérez D, Javier Chaves F, Esteve E, Marin-Garcia P, Xifra G, Vendrell J, Jové M, Pamplona R, Ricart W, et al. Obesity changes the human gut mycobiome. Sci Rep. 2015;5:14600.
- Sun S, Sun L, Wang K, Qiao S, Zhao X, Hu X, Chen W, Zhang S, Li H, Dai H, et al. The gut commensal fungus, Candida parapsilosis, promotes high fat-diet induced obesity in mice. Commun Biol. 2021;4:1–11.
- Heisel T, Montassier E, Johnson A, Al-Ghalith G, Lin YW, Wei LN, Knights D, Gale CA. High-fat diet changes fungal microbiomes and interkingdom relationships in the murine gut. M Sphere. 2017;2: e00351-17.
- Verdam FJ, Fuentes S, de Jonge C, Zoetendal EG, Erbil R, Greve JW, Buurman WA, de Vos WM, Rensen SS. Human intestinal microbiota composition is associated with local and systemic inflammation in obesity. Obesity. 2013;21:E607–615.
- Chopyk DM, Grakoui A. Contribution of the intestinal microbiome and gut barrier to hepatic disorders. Gastroenterology. 2020;159:849–863.
- You N, Xu J, Wang L, Zhuo L, Zhou J, Song Y, Ali A, Luo Y, Yang J, Yang W, et al. Fecal fungi dysbiosis in nonalcoholic fatty liver disease. Obes Silver Spring Md. 2021;29:350–358.
- Demir M, Lang S, Hartmann P, Duan Y, Martin A, Miyamoto Y, Bondareva M, Zhang X, Wang Y, Kasper P, et al. The fecal mycobiome in non-alcoholic fatty liver disease. J Hepatol. 2022;76:788–799.
- Kamiński DM. Recent progress in the study of the interactions of amphotericin B with cholesterol and ergosterol in lipid environments. Eur Biophys J. 2014;43:453–467.
- Salyer ACD, Caruso G, Khetani KK, Fox LM, Malladi SS, David SA. Identification of adjuvantic activity of amphotericin B in a novel, multiplexed, poly-TLR/NLR high-throughput screen. PLos One. 2016;11:e0149848.
- Hirschfield GM, Karlsen TH, Lindor KD, Adams DH. Primary sclerosing cholangitis. Lancet. 2013;382:1587–1599.
- Loftus EV, Harewood GC, Loftus CG, Tremaine WJ, Harmsen WS, Zinsmeister AR, Jewell DA, Sandborn WJ. PSC-IBD: a unique form of inflammatory bowel disease associated with primary sclerosing cholangitis. Gut. 2005;54:91–96.
- Pollheimer MJ, Halilbasic E, Fickert P, Trauner M. Pathogenesis of primary sclerosing cholangitis. Best Pract Res Clin Gastroenterol. 2011;25:727–739.
- Chapman R, Cullen S. Etiopathogenesis of primary sclerosing cholangitis. World J Gastroenterol. 2008;14:3350–3359.
- Muratori P, Muratori L, Guidi M, Maccariello S, Pappas G, Ferrari R, Gionchetti P, Campieri M, Bianchi FB. Anti-Saccharomyces cerevisiae antibodies (ASCA) and autoimmune liver diseases. Clin Exp Immunol. 2003;132:473–476.
- Rudolph G, Gotthardt D, Klöters-Plachky P, Kulaksiz H, Rost D, Stiehl A. Influence of dominant bile duct stenoses and biliary infections on outcome in primary sclerosing cholangitis. J Hepatol. 2009;51:149–155.
- Katt J, Schwinge D, Schoknecht T, Quaas A, Sobottka I, Burandt E, Becker C, Neurath MF, Lohse AW, Herkel J, et al. Increased T helper type 17 response to pathogen stimulation in patients with primary sclerosing cholangitis. Hepatol Baltim Md. 2013;58:1084–1093.
- Nakamoto N, Sasaki N, Aoki R, Miyamoto K, Suda W, Teratani T, Suzuki T, Koda Y, Chu PS, Taniki N, et al. Gut pathobionts underlie intestinal barrier dysfunction and liver T helper 17 cell immune response in primary sclerosing cholangitis. Nat Microbiol. 2019;4:492–503.
- Lemoinne S, Kemgang A, Belkacem KB, Straube M, Jegou S, Corpechot C, Network SA, Chazouillères O, Housset C, Sokol H. Fungi participate in the dysbiosis of gut microbiota in patients with primary sclerosing cholangitis. Gut. 2020;69:92–102.
- Sokol H, Leducq V, Aschard H, Pham HP, Jegou S, Landman C, Cohen D, Liguori G, Bourrier A, Nion-Larmurier I, et al. Fungal microbiota dysbiosis in IBD. Gut. 2017;66:1039–1048.
- Rühlemann MC, Solovjeva MEL, Zenouzi R, Liwinski T, Kummen M, Lieb W, Hov JR, Schramm C, Franke A, Bang C. Gut mycobiome of primary sclerosing cholangitis patients is characterised by an increase of trichocladium griseum and candida species. Gut. 2020;69:1890–1892.
- Vasquez A, Zavasky D, Chow NA, Gade L, Zlatanic E, Elkind S, Litvintseva AP, Pappas PG, Perfect JR, Revankar S, et al. Management of an outbreak of exophiala dermatitidis bloodstream infections at an outpatient oncology clinic. Clin Infect Dis off Publ Infect Dis Soc Am. 2018;66:959–962.
- Oztas E, Odemis B, Kekilli M, Kurt M, Dinc BM, Parlak E, Kalkanci A, Sasmaz N. Systemic phaeohyphomycosis resembling primary sclerosing cholangitis caused by exophiala dermatitidis. J Med Microbiol. 2009;58:1243–1246.
- Hong KH, Kim JW, Jang SJ, Yu E, Kim EC. Liver cirrhosis caused by exophiala dermatitidis. J Med Microbiol. 2009;58:674–677.
- Anthony PP, Ishak KG, Nayak NC, Poulsen HE, Scheuer PJ, Sobin LH. The morphology of cirrhosis. Recommendations on definition, nomenclature, and classification by a working group sponsored by the world health organization. J Clin Pathol. 1978;31:395–414.
- Schuppan D, Afdhal NH. Liver cirrhosis. Lancet. 2008;371:838–851.
- GBD 2017 Cirrhosis Collaborators. The global, regional, and national burden of cirrhosis by cause in 195 countries and territories, 1990-2017: a systematic analysis for the global burden of disease study 2017. Lancet Gastroenterol Hepatol. 2020;5:245–266.
- Tandon P, Garcia-Tsao G. Bacterial infections, sepsis, and multiorgan failure in cirrhosis. Semin Liver Dis. 2008;28:26–42.
- Bajaj JS, Liu EJ, Kheradman R, Fagan A, Heuman DM, White M, Gavis EA, Hylemon P, Sikaroodi M, Gillevet PM. Fungal dysbiosis in cirrhosis. Gut. 2018;67:1146–1154.
- Zullo A, Hassan C, Ridola L, Lorenzetti R, Campo SM, Riggio O. Rifaximin therapy and hepatic encephalopathy: pros and cons. World J Gastrointest Pharmacol Ther. 2012;3:62–67.
- Chen Y, Chen Z, Guo R, Chen N, Lu H, Huang S, Wang J, Li L. Correlation between gastrointestinal fungi and varying degrees of chronic hepatitis B virus infection. Diagn Microbiol Infect Dis. 2011;70:492–498.
- Guo R, Chen Z, Chen N, Chen Y. Quantitative real-time PCR analysis of intestinal regular fungal species in fecal samples from patients with chronic hepatitis B virus infection. Lab Med. 2010;41:591–596.
- Mou H, Yang F, Zhou J, Bao C. Correlation of liver function with intestinal flora, vitamin deficiency and IL-17A in patients with liver cirrhosis. Exp Ther Med. 2018;16:4082–4088.
- Cui L, Morris A, Ghedin E. The human mycobiome in health and disease. Genome Med. 2013;5:63.
- Ou TM, Huang HH, Hsieh TY, Chang WK, Chu HC, Hsu CH, Shih YL, Huang TY, Chen PJ, Lin HH. Liver cirrhosis as a predisposing factor for esophageal candidiasis. Adv Dig Med. 2014;1:86–91.
- Verma N, Roy A, Singh S, Pradhan P, Garg P, Singh M. Factors determining the mortality in cirrhosis patients with invasive candidiasis: a systematic review and meta-analysis. Med Mycol. 2022;60:myab069.
- Llovet JM, Kelley RK, Villanueva A, Maini MK, Mazzaferro V, Pinato DJ, Pikarsky E, Zhu AX, Finn RS. Hepatocellular carcinoma. Nat Rev Dis Primer. 2021;7:6.
- IARC International Agency for Research on Cancer. GLOBOCAN 2018. 2020 [Accessed 2022 July 7]. http://gco.iarc.fr/today/home.
- Chidambaranathan-Reghupaty S, Fisher PB, Sarkar D. Hepatocellular carcinoma (HCC): epidemiology, etiology and molecular classification. Adv Cancer Res. 2021;149:1–61.
- Ponziani FR, Bhoori S, Castelli C, Putignani L, Rivoltini L, Del Chierico F, Sanguinetti M, Morelli D, Paroni Sterbini F, Petito V, et al. Hepatocellular carcinoma is associated with gut microbiota profile and inflammation in nonalcoholic fatty liver disease. Hepatol Baltim Md. 2019;69:107–120.
- Ren Z, Li A, Jiang J, Zhou L, Yu Z, Lu H, Xie H, Chen X, Shao L, Zhang R, et al. Gut microbiome analysis as a tool towards targeted non-invasive biomarkers for early hepatocellular carcinoma. Gut. 2019;68:1014–1023.
- Kew MC. Aflatoxins as a cause of hepatocellular carcinoma. J Gastrointest Liver Dis JGLD. 2013;22:305–310.
- Hamid AS, Tesfamariam IG, Zhang Y, Zhang ZG. Aflatoxin B1-induced hepatocellular carcinoma in developing countries: geographical distribution, mechanism of action and prevention. Oncol Lett. 2013;5:1087–1092.
- Hussain SP, Schwank J, Staib F, Wang XW, Harris CC. TP53 mutations and hepatocellular carcinoma: insights into the etiology and pathogenesis of liver cancer. Oncogene. 2007;26:2166–2176.
- Bressac B, Kew M, Wands J, Ozturk M. Selective G to T mutations of p53 gene in hepatocellular carcinoma from southern Africa. Nature. 1991;350:429–431.
- Qian GS, Ross RK, Yu MC, Yuan JM, Gao YT, Henderson BE, Wogan GN, Groopman JD. A follow-up study of urinary markers of aflatoxin exposure and liver cancer risk in Shanghai, people’s republic of China. Cancer Epidemiol Biomark Prev Publ Am Assoc Cancer Res Cosponsored Am Soc Prev Oncol. 1994;3:3–10.
- Allen SJ, Wild CP, Wheeler JG, Riley EM, Montesano R, Bennett S, Whittle HC, Hall AJ, Greenwood BM. Aflatoxin exposure, malaria and hepatitis B infection in rural Gambian children. Trans R Soc Trop Med Hyg. 1992;86:426–430.
- Turner PC, Mendy M, Whittle H, Fortuin M, Hall AJ, Wild CP. Hepatitis B infection and aflatoxin biomarker levels in Gambian children. Trop Med Int Health TM IH. 2000;5:837–841.
- Groopman JD, Wang JS, Scholl P. Molecular biomarkers for aflatoxins: from adducts to gene mutations to human liver cancer. Can J Physiol Pharmacol. 1996;74:203–209.
- Kew MC. Hepatitis B virus x protein in the pathogenesis of hepatitis B virus-induced hepatocellular carcinoma. J Gastroenterol Hepatol. 2011;26:144–152.
- Ahn JY, Jung EY, Kwun HJ, Lee CW, Sung YC, Jang KL. Dual effects of hepatitis B virus X protein on the regulation of cell-cycle control depending on the status of cellular p53. J Gen Virol. 2002;83:2765–2772.
- Madden CR, Finegold MJ, Slagle BL. Altered DNA mutation spectrum in aflatoxin b1-treated transgenic mice that express the hepatitis B virus x protein. J Virol. 2002;76:11770–11774.
- Chisari FV, Klopchin K, Moriyama T, Pasquinelli C, Dunsford HA, Sell S, Pinkert CA, Brinster RL, Palmiter RD. Molecular pathogenesis of hepatocellular carcinoma in hepatitis B virus transgenic mice. Cell. 1989;59:1145–1156.
- Liu Z, Li Y, Li C, Lei G, Zhou L, Chen X, Jia X, Lu Y. Intestinal Candida albicans promotes hepatocarcinogenesis by up-regulating NLRP6. Front Microbiol. 2022;13:812771.
- Zhou L, Ding L, Yin P, Lu X, Wang X, Niu J, Gao P, Xu G. Serum metabolic profiling study of hepatocellular carcinoma infected with hepatitis B or hepatitis C virus by using liquid chromatography-mass spectrometry. J Proteome Res. 2012;11:5433–5442.
- Fujiwara N, Nakagawa H, Enooku K, Kudo Y, Hayata Y, Nakatsuka T, Tanaka Y, Tateishi R, Hikiba Y, Misumi K, et al. CPT2 downregulation adapts HCC to lipid-rich environment and promotes carcinogenesis via acylcarnitine accumulation in obesity. Gut. 2018;67:1493–1504.
- Ishikawa H, Takaki A, Tsuzaki R, Yasunaka T, Koike K, Shimomura Y, Seki H, Matsushita H, Miyake Y, Ikeda F, et al. L-carnitine prevents progression of non-alcoholic steatohepatitis in a mouse model with upregulation of mitochondrial pathway. PLos One. 2014;9:e100627.
- Levy M, Thaiss CA, Zeevi D, Dohnalova L, Zilberman-Schapira G, Mahdi JA, David E, Savidor A, Korem T, Herzig Y, et al. Microbiota-modulated metabolites shape the intestinal microenvironment by regulating NLRP6 inflammasome signaling. Cell. 2015;163:1428–1443.
- Li R, Zhu S. NLRP6 inflammasome. Mol Aspects Med. 2020;76:100859.
- Ansari F, Alian Samakkhah S, Bahadori A, Jafari SM, Ziaee M, Khodayari MT, Pourjafar H. Health-promoting properties of Saccharomyces cerevisiae var. boulardii as a probiotic; characteristics, isolation, and applications in dairy products. Crit Rev Food Sci Nutr. 2021;0:1–29.
- Yu L, Zhao X, Cheng M, Yang GZ, Wang B, Liu HJ, Hu YX, Zhu LL, Zhang S, Xiao ZW, et al. Saccharomyces boulardii administration changes gut microbiota and attenuates D-Galactosamine-induced liver injury. Sci Rep. 2017;7:1359.
- Everard A, Matamoros S, Geurts L, Delzenne NM, Cani PD. Saccharomyces boulardii administration changes gut microbiota and reduces hepatic steatosis, low-grade inflammation, and fat mass in obese and type 2 diabetic db/db mice. mBio. 2014;5:e01011–01014.
- Liu YT, Li YQ, Wang YZ. Protective effect of saccharomyces boulardii against intestinal mucosal barrier injury in rats with nonalcoholic fatty liver disease. Zhonghua Gan Zang Bing Za Zhi Zhonghua Ganzangbing Zazhi Chin J Hepatol. 2016;24:921–926.
- Huang J, Ou Y, Yew TWD, Liu J, Leng B, Lin Z, Su Y, Zhuang Y, Lin J, Li X, et al. Hepatoprotective effects of polysaccharide isolated from Agaricus bisporus industrial wastewater against CCl₄-induced hepatic injury in mice. Int J Biol Macromol. 2016;82:678–686.
- Liu Y, Zheng D, Su L, Wang Q. Protective effect of polysaccharide from Agaricus bisporus in Tibet area of China against tetrachloride-induced acute liver injury in mice. Int J Biol Macromol. 2018;118:1488–1493.
- Li S, Li J, Zhang J, Wang W, Wang X, Jing H, Ren Z, Gao Z, Song X, Gong Z, et al. The antioxidative, antiaging, and hepatoprotective effects of alkali-extractable polysaccharides by Agaricus bisporus. Evid-Based Complement Altern Med ECAM. 2017;2017:7298683.
- Duan Z, Zhang Y, Zhu C, Wu Y, Du B, Ji H. Structural characterization of phosphorylated pleurotus ostreatus polysaccharide and its hepatoprotective effect on carbon tetrachloride-induced liver injury in mice. Int J Biol Macromol. 2020;162:533–547.
- Fernández-Pacheco P, Pintado C, Briones Pérez A, Arévalo-Villena M. Potential probiotic strains of saccharomyces and non-saccharomyces: functional and biotechnological characteristics. J Fungi. 2021;7:177.
- Santino I, Alari A, Bono S, Teti E, Marangi M, Bernardini A, Magrini L, Di Somma S, Teggi A. Saccharomyces cerevisiae fungemia, a possible consequence of the treatment of clostridium difficile colitis with a probioticum. Int J Immunopathol Pharmacol. 2014;27:143–146.
- Cesaro S, Chinello P, Rossi L, Zanesco L. Saccharomyces cerevisiae fungemia in a neutropenic patient treated with saccharomyces boulardii. Support Care Cancer off J Multinatl Assoc Support Care Cancer. 2000;8:504–505.