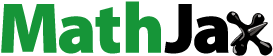
ABSTRACT
Two-thirds of small-bowel transplantation (SBT) recipients develop bacteremia, with the majority of infections occurring within 3 months post-transplant. Sepsis-related mortality occurs in 31% of patients and is commonly caused by bacteria of gut origin, which are thought to translocate across the implanted organ. Serial post-transplant surveillance endoscopies provide an opportunity to study whether the composition of the ileal and colonic microbiota can predict the emergence as well as the pathogen of subsequent clinical infections in the SBT patient population. Five participants serially underwent aspiration of ileal and colonic bowel effluents at transplantation and during follow-up endoscopy either until death or for up to 3 months post-SBT. We performed whole-metagenome sequencing (WMS) of 40 bowel effluent samples and compared the results with clinical infection episodes. Microbiome composition was concordant between participants and timepoint-matched ileal and colonic samples. Four out of five (4/5) participants had clinically significant infections thought to be of gut origin. Bacterial translocation from the gut was observed in 3/5 patients with bacterial infectious etiologies. In all three cases, the pathogens had demonstrably colonized the gut between 1–10 days prior to invasive clinical infection. Recipients with better outcomes received donor grafts with higher alpha diversity. There was an increase in the number of antimicrobial resistance genes associated with longer hospital stay for all participants. This metagenomic study provides preliminary evidence to support the pathogen translocation hypothesis of gut-origin sepsis in the SBT cohort. Ileal and colonic microbiome compositions were concordant; therefore, fecal metagenomic analysis could be a useful surveillance tool for impeding infection with specific gut-residing pathogens.
Introduction
Small Bowel Transplantation (SBT) is a last-resort treatment for intestinal failure.Citation1 SBTs are rarely performed; only 172 surgeries were performed globally in 2021.Citation2 Twenty-seven of these were conducted across four specialist centers in the UK.Citation3 In the UK, 88.2% and 72.6% of non-liver-containing SBT recipients are alive at one and three years respectively (70.7% and 52.0% for liver-containing grafts).Citation4 Nine out of ten patients undergoing SBT develop clinically significant infections; nearly two-thirds of cases present with bacterial sepsis, commonly with enteric bacteria, making it the most frequent cause of morbidity and mortality in the post-transplant period.Citation5–7
Gut-origin sepsis is a long-held hypothesis suggesting that damage to the intestinal barrier allows enteric pathogens or microbial products to translocate into the bloodstream, especially in patients undergoing abdominal surgery.Citation8–10 In SBT patients, the use of empirical antimicrobials and immunosuppressive therapy to mitigate acute graft rejection inadvertently disrupts the gut microbiome, which enters a state of dysbiosis, in addition to damaging the structural integrity of the intestinal epithelium by the surgery itself.Citation7,Citation11 Furthermore, acute graft rejection is a common complication post-SBT, affecting 40% of recipients and accounting for 13% of patient deaths.Citation10,Citation12 Ischemia-reperfusion injury and damage to enterocytes that occurs as a result of acute graft rejection increase intestinal permeability, allowing for bacterial translocation, which can trigger a disseminated sepsis response.Citation13,Citation14 Graft-versus-host disease (GvHD) occurs in 9% of SBT recipients, 77% of whom die of the disease. Most GvHD-associated deaths are also caused by infection.Citation15 Consequently, translocating antimicrobial-resistant (AMR) enteric pathogens are frequent etiologic agents of post-SBT clinical infections, currently posing a major barrier to improving patient outcomes. Prompt diagnosis and treatment of infections in SBT are crucial.
Gut microbiome alterations in SBT patients have previously been recognized as potential predictive markers of infection, sepsis or graft rejection.Citation16 Reduced gut microbiome diversity has also been associated with adverse patient outcomes in other solid and liquid organ transplants; lower microbiome diversity in the post-operative period is associated with graft rejection and bacteremia after liver and kidney transplantation.Citation17,Citation18 In allogeneic stem cell transplantation, domination by Enterococcus and Proteobacteria in the gut is associated with an increased risk of developing Enterococcal or Gram-negative bacteremia.Citation19 Since infection and rejection in SBT can present with similar clinical signs and symptoms, endoscopic biopsies are necessary to distinguish between them.Citation20 Routine surveillance endoscopies, accessed through the stoma created during surgery, offer opportunities to monitor the recovery of the surgically altered bowel and sample the gut microbiome.Citation11
The aim of this study was to perform whole-metagenome sequencing (WMS) to assess the gut microbiomes of five SBT recipients during the post-transplant period. The primary objective was to characterize the temporal shifts in microbiota composition and bacterial diversity with respect to clinical infections and determine whether the infective pathogen can be linked back to the gut microbiome at species or sequence-type (ST) level. We hypothesized that gut colonization precedes invasive infections by the dominant species. Secondary objectives included reporting the changes in 1) AMR carriage and 2) genes encoding functional metabolic pathways in gut bacteria after SBT.
Methods
Experimental design
This prospective cohort study enrolled and followed up with five adult participants undergoing Small Bowel Transplantation between August 2021 and December 2022. This single-center study involved the Multi-Visceral Transplant, Gastrointestinal and Infectious Diseases Departments at Cambridge University Hospital NHS Foundation Trust, UK. This study was approved by the Wales Research Ethics Committee (IRAS Project ID 213,655). Patients and the public were not involved in the design, conduct, reporting, or dissemination plans of the research.
Participant eligibility criteria and consent
Eligible study participants were aged over 17 years, male or female, under the care of the Multi-Visceral Transplant team, and were able to give informed consent. The participants received written information, telephone contact with the study team, and completed an electronic consent form.
Donor organ retrieval
The multi-visceral organ retrieval was performed after confirmation of brain death of donors. In each case, an 80 L cold box was used, which contained multiple 1 L Belzer UW® cold storage transport solution, Soltran bags and Hartmann’s fluid, packed with crushed ice. Heparin and antimicrobials were given during the retrieval operation and the organs were perfused with Belzer solution via the aorta and portal vein. All donors received pre-operative gastrointestinal decontamination (nasogastric antibiotic ciprofloxacin and antifungal fluconazole) and intra-operative intravenous antibiotic meropenem, vancomycin and antifungal amphotericin B liposomal.
Organs were further perfused with room temperature and then cold Hartmann’s infusions until cold and ready to be removed. They were placed in cold ice slush dishes and then packed for transport. The organ block was packed in 3 sterile bags with cold preservation solution, cold saline, and cold fluid respectively. This was placed in the 80 L cold box with ice, sealed with secure tape and labeled.
Clinical data, sample collection and anonymisation
Clinical data on the recipients were collected from electronic medical records and included demographics, antimicrobial and immunosuppressant use, and clinical outcomes (infection or rejection episodes and survival). Data were collected on the day of transplantation and at post-transplant endoscopy appointments. The participants were followed up for 1 year to collect infection and survival data. Any organisms that were identified from clinical samples and reported as significant by a clinical microbiologist were included in the analyses.
Fecal effluent samples were collected from study participants on the date of transplantation (day 0) and at follow-up endoscopies. Samples collected at the time of transplantation included 10 mL of fecal effluent from the recipient’s discarded colon, 10 mL from the recipient’s discarded ileum and 10 mL of donor effluent sample after implantation from the new ileum (which came from the donor and was placed into the recipient). These were transferred on ice from the operating theater to laboratory fridges and processed within 36 hours.
Participants were assigned a unique number.Citation1–5 Samples were assigned a unique code comprising the participant’s unique number, followed by the visit or endoscopy number (V1/E1-E5), and the site from which the sample was taken (ileal-I, colonic-C, donor-D), as shown in Supplementary Data, .
Table 1. Organisms cultured from clinical samples by participant.
Sampling considerations
It was impossible to enroll and consent donors into this study (prior to their unexpected deaths) and sample their organs (or collect any personal data) for this research study due to ethical considerations. There is no sampling of donor gut microbiomes performed in the UK health system routinely. However, immediately after surgical implantation, when the donor organ was perfused from the recipient’s blood supply, the donor’s bowel and effluent belonged to the recipient and therefore could be sampled for purposes of this research study. Sampling of the ‘donor’ fecal effluent was performed at this point. Effluent sampling was chosen over gut tissue sampling as it is considered less invasive, is known to contain abundant bacterial DNA and the authors hypothesized that it may translate to bedside stoma-site sampling in the future.
Effluent sample processing
Neat effluent sample (500 µl) was added at a ratio of 1:1 with 500 µl of in-house-produced lysis buffer (4 M guanidine thiocyanate (Sigma-Aldrich®) in 25 mM Tris-HCl, 0.5% β-mercaptoethanol (Sigma-Aldrich®) into a Lysing Matrix E bead tube (Mp Biomedicals™, code 116,914,050.) This underwent bead beating using the FastPrep™ machine (Mp Biomedicals™) for 40 seconds at speed 6,000 rpm and then incubated at room temperature for 10 minutes.
Ethanol (100% (v/v), 1 mL) was added to the bead tube to achieve an ethanol concentration of 50% (v/v) and the tube was incubated at ambient temperature for a further 10 minutes with gentle agitation. A further 860 µl of lysis buffer was added to the tube to bring the final ethanol concentration to 35% (v/v) for optimal column binding. Then 700 µl of this mix was pipetted into a new spin column tube (NBS Biologicals Ltd, code SD5008). The spin columns were placed in the microfuge rotor and spun at 15,000 rpm for 1 minute. The flow-through was discarded.
The column was washed by adding 500 µl of Wash Buffer 1 (1 M guanidine thiocyanate in 25 mM Tris-HCl, with 10% ethanol) and spun for 30 seconds at 15,000 rpm, and the eluate was discarded. The column was washed again, using 500 µl of Wash Buffer 2 (25 mM Tris-HCl buffer, with 70% ethanol) and spun for 30 seconds at 15,000 rpm and the eluate was discarded. The column was washed once more, with 500 µl of Wash Buffer 2 and spun for 2 minutes at 15,000 rpm to remove all the wash solution. A summary of buffer compositions can be found in Supplementary Data.
After ensuring there was no liquid remaining in the tip of the spin column, the spin column was transferred to a clean RNAse free tube (ThermoFisher Scientific Inc., code AM12425.) The nucleic acids were eluted by adding 100 µl of nuclease free water to the column and spun at 15,000 rpm for 1 minute. The spin column was discarded, and the nucleic acid was stored at −70°C.
Sequencing of bowel effluent samples
DNA concentrations were quantified on a Qubit™ 2.0 Fluorometer, and 50 µl of DNA from each sample was transferred into barcoded snap-cap microcentrifuge tubes and shipped to Eurofins Genomics for standard library preparation and sequencing. DNA samples were sequenced on an Illumina NovaSeq 6000 S4 PE150 instrument, with a minimum of 10 million paired-end reads requested for metagenomic sequencing of bowel effluent samples.
Sequencing of selected bacterial isolates
Three recovered clinical bacterial isolates were taken from freezer and passaged twice, on Columbia blood agar (Merck), incubated at 37°C overnight, in aerobic conditions. Enterococcus faecium was grown for 48 hours prior to extraction. Klebsiella michiganensis was grown for 24 hours prior to extraction. Identification was confirmed using the MALDI-TOF Biotyper® (Bruker) platform. The QIAamp® DNA Mini Kit (Qiagen®, code 51,304) was used to extract the bacterial DNA as per the manufacturer’s protocol.
DNA concentrations were quantified on a Qubit™ 2.0 Fluorometer and 100 µl of DNA from each sample was transferred into barcoded snap-cap microcentrifuge tubes and shipped to Eurofins Genomics for standard library preparation and sequencing. Isolate DNA samples underwent whole-genome sequencing (WGS) on an Illumina NovaSeq 6000 S4 PE150 instrument, with a minimum of five million paired-end reads requested for each bacterial isolate.
Bioinformatics analyses
The raw metagenomic sequencing reads were assessed for quality using FastQC v0.11.9.Citation21 After checking QC statistics, kneaddata v0.10.0Citation22 along with the FastQC outputs were used to remove adapters, primer sequences and host reads using the human GCh38 reference genome. Taxonomic assignment on the QC-checked reads was performed using Kraken2 v2.1.2Citation23 using a custom-built database containing archaeal, bacterial, human, fungal, protozoan and viral reference genomes. The “extract_kraken_reads.py” script from the KrakenTools v1.2 suite was used to remove classified human host reads and Kraken2 was run again on the filtered reads for microbial assignment. Bayesian re-estimations at phylum and species levels were performed using Bracken v2.7.0.Citation24 Following assignments, archaeal, viral, and protozoan phyla were disregarded due incomplete draft reference genomes and because our extraction protocol was not optimized to capture organisms belonging to these kingdoms.
Raw metagenomic reads were assembled into contigs using metaSPAdes v3.13.0,Citation25 followed by AMR gene detection using ABRIcate v1.0.1Citation26 with the Comprehensive Antimicrobial Resistance Database (CARD)Citation27 as reference. The ABRIcate pipeline identified 916 AMR genes in the sample collection. We filtered these to only consider genes detected with > 95% identity to the CARD reference gene, producing a subset of 746 AMR genes. In addition, AMR genes conferring resistance to 3 drug classes were reclassified as ‘multidrug’. An unpaired t-test was used to show statistically significant differences (p < 0.05) in the total number of AMR genes between samples pooled by timepoint for the first three timepoints, i.e., day of transplant, first, and second follow-up endoscopies. Functional profiling was carried out to detect genes corresponding to metabolic pathways by running HUMANN3 v3.5 against the Uniref90 database and normalized to reads per kilobase mapped (RPKM) values.Citation22 MaAsLin2 v1.12.0 was used to extract significant associations (if any) between participants and metabolic pathway gene abundances.Citation22 Participant 5 was selected as the reference comparator for gut bacterial functional pathway gene abundance calculations. Participant 5 had the best outcome post-transplant, defined as no post-operative sepsis or rejection episodes, survival at 1 year, and short inpatient hospital stay.
Downstream analyses were performed in RStudio v4.0.4. Kraken2 reports were visualized on the Pavian R package v1.2.0.Citation28 Data cleaning and transformation were carried out using the dplyr v 1.0.8,Citation29 stringr v1.4.0, tidyr v1.2.0 and tidyverse v1.3.2 packages.Citation30 Microbial alpha and beta diversities were computed using vegan v2.5–7.Citation31 Bracken results were combined into a biom table format and rarefied to randomly subsample 20,477 operational taxonomic units (OTUs) from each sample without replacement. PCA was performed using the stats v4.0.4Citation32 and ggbiplot v0.55Citation33 packages to assess total variation in the data.
There was wide variability in the number of raw metagenomic reads per sample among the effluent sample collection (Range: 15956–13071245) and the number of operational taxonomic units (OTUs) detected (Range 931–11811807). We rarefied the collection to randomly subsample at an arbitrary threshold of 20,477 OTUs. Three samples (1V1I, 3E3C and 3E3I) had fewer OTUs than the designated threshold, for which we used all available data on assigned OTUs for individual sample composition analyses, but these samples were excluded from diversity, AMR and metabolomic analyses.
Raw WGS reads from the three bacterial isolates were processed using Nextflow v21.10.6Citation34 and the GHRU de novo assembly pipeline v2.1.2.Citation35 The inStrain v1.8.0 pipelineCitation36 was used to link isolates from clinical infection episodes back to patients’ gut microbiomes. FASTA files from the three WGS isolates were indexed using bowtie2 v2.4.5.Citation37 Raw read files from the bowel effluent samples containing the highest relative abundance of the corresponding organisms were selected according to the patients and timepoints these infections manifested in. Bowtie2 was then used to map the raw read files against the indexed isolate sequences. The inStrain profile and inStrain compare functions were used to determine average nucleotide identity (ANI) among the regions from the effluent reads which mapped to the indexed isolate genomes (Supplementary data, table s2). The ‘coverage overlap’ column denotes the percentage of bases in the indexed genome which mapped to the effluent read samples. The ‘percent compared’ column depicts the fraction of the reads sequenced at 5× coverage in both effluent samples that were being compared to the indexed genome.Citation36 ANI and relatedness between the two Enterococcus faecium isolates were tested using FastANI v1.33Citation38 and MASH v2.3.Citation39 Finally, the two Enterococcus faecium isolates were sequence-typed using MLST v2.23.0Citation40 on the command line.
Bias and sample size
We reduced bias in our cohort study by utilizing remote, real-time enrollment to all those listed for transplantation at our center, and consequently, none declined participation. We reduced the loss to follow-up to zero by sampling along with routine clinical procedures performed in the hospital setting. A sample size of five was chosen to ensure that time and financial constraints enabled completion over 18 months, recruiting approximately one participant per month and allowing year-long follow-up.
Results
Participant clinical details
Multiple sampling of the five participants over time allowed the collection of 40 bowel effluent samples in total: 5 from post-implantation donor grafts on the date of transplant, 17 from recipient ileums, and 18 from recipient colons. At the time of transplantation, all participants were receiving parenteral nutrition, were ex-smokers or nonsmokers, and had alcohol intake within the recommended limits. None of the participants had serological evidence of previous infection with hepatitis B or C and none had active COVID-19 infection, which was excluded by concurrent PCR testing.
Total microbiota composition
65 unique phyla were detected in the 40 samples. The top nine phyla accounted for over 96% of all operational taxonomic units (OTUs) detected in the collection. Of these, we selected the five most abundant bacterial and/or fungal phyla and assessed their relative abundances longitudinally. shows the most abundant phyla in each sample. The most abundant phyla were Proteobacteria (50.4%), Firmicutes (30.4%), Bacteroidetes (11.2%), Ascomycota (2.3%) and Actinobacteria (1.7%).
Figure 1. Stacked bar charts representing the relative abundances (% of OTUs in sample) of five most prominent phyla by sampling timepoint.

Gut microbiota composition and clinical outcomes
In our study, the gut microbiota composition was predictive of subsequent episodes of clinical infection. Enteric pathogens showed high relative abundances in the gut between 1–10 days prior to causing invasive clinical infection. Four out of the five (4/5) participants had clinically significant organisms identified (). Three out of six (3/6) of these organisms were thought to be of gut origin: Candida glabrata, Enterococcus spp., and Klebsiella michiganensis. Aspergillus fumigatus in participant 3 was suspected to have originated from the lungs (isolated in sputum and later causing an episode of discitis), and Staphylococcus epidermidis in participant 2 was thought to have originated from the skin (later causing a line infection). The origin of the pseudomonal urinary infection in participant 3 was less clear and could include environmental (water) sources. The high Firmicutes abundance was often due to the dominance of Enterococcus faecium.
In our cohort, we noted that higher donor alpha diversities appeared to be associated with better recipient outcomes (Participants 1 and 5). shows the Shannon alpha diversity index at the species level for all available participant samples. Participants whose post-transplant ileal and colonic samples had Shannon alpha diversities increase to over 2.5 at their final endoscopy, i.e., participants 1,3 and 5, survived. Pavian plots containing the metagenomic compositions for each sample are shown in Supplementary Data, .
Figure 2. Shannon α-diversity index, at species level, of participant samples.

Participant 1
Effluent samples were collected at transplant (day 0) and at the first and second endoscopies (days 13 and 34, respectively). Over 40% of the donor sample comprised the Bacteroidetes phylum (42.65% of all assigned OTUs) – the highest composition of Bacteroidetes among all five donors in our cohort. The donor sample also had the second-highest alpha diversity among all donor samples (Shannon = 2.96). At the first follow-up endoscopy, samples from participant 1 showed an increase in the relative abundance of the phylum Ascomycota (dominated by Candida glabrata) in both the ileal (62.95%) and colonic (63.19%) samples. Ten days after the first endoscopy, the patient was diagnosed with a Candida glabrata intra-abdominal infection, requiring surgical drainage and antifungals. They achieved good recovery and alpha diversity of samples obtained at the second endoscopy had increased to 3.55. Participant 1 was discharged 53 days after their transplant.
Participant 2
Effluent samples were collected at transplant (day 0) and during four follow-up endoscopies (days 20, 40, 60, and 81). Samples collected on day 40 showed abundant Enterococcus faecium in the gut. The next day, vancomycin-resistant Enterococcus faecium (VRE) was detected in the urine. The participant was treated with tigecycline, and subsequent endoscopy (day 60) showed a reduction in the taxon in response to the treatment administered. On further endoscopy (day 81), both ileal and colonic samples showed a substantial relapse of VRE, and they developed Enterococcus faecium bacteremia post-procedure on the same day. The alpha diversity of both the ileal and colonic samples at this time point was below 1.5. They had two additional VRE bloodstream infections and sepsis episodes (days 148 and 151) possibly contributing to their death 164 days post-transplant.
Participant 3
Effluent samples were collected at transplant (day 0) and at five follow-up endoscopies (days 8, 15, 43, 84, and 99). At the time of transplantation, the recipient’s samples showed high abundances of Enterococcus faecium (77.04%–80.12%), and this taxon remained dominant at the first (50.59%) and second endoscopies (66.62%–71.02%). Three days after the first endoscopy, VRE was cultured from the body fluid of participant 3 (). Aspergillus fumigatus was detected in the sputum (on day 4 post-transplantation) and bone biopsy (on day 182) but was not observed in the gut microbiome. Notably, the donor sample for participant 3 had the lowest alpha diversity at the species level among all donor samples tested (Shannon index = 1.31), as shown in . Participant 3 was discharged 144 days post-transplantation and was alive at 12 months follow up, despite a readmission at 7 months with fungal (Aspergillus fumigatus) discitis, requiring voriconazole, laminectomy and decompression.
Participant 4
Effluent samples were collected at transplant (day 0) and one follow-up endoscopy (day 10) prior to death on day 18. The donor sample for participant 4 had a Shannon alpha diversity under 2.0, and the recipient samples collected on day 0 showed similar alpha diversity indexes (1.86–2.04). Endoscopy samples had notable relative abundances of Enterococcus faecium (5.66%) and the genus Klebsiella (71.5% − 73.0%) which were classified by Kraken2 as Klebsiella michiganensis. Klebsiella michiganensis and VRE were isolated from the peritoneal fluid and blood clots on day 15, at the time of surgery, prior to death (). Therefore, both organisms that contributed to death were abundant in the gut prior to participant 4’s deterioration.
Participant 5
Participant 5 had the best outcomes: no infection/rejection episodes, survival and a short inpatient hospital stay. Samples were collected on the day of transplantation (day 0) and at the first and second follow-up endoscopies (day 13, 21). The donor sample for participant 5 had the highest alpha diversity index (Shannon = 4.20), and the recipient’s ileal and colonic samples had alpha diversity indices > 3.0, on the date of transplant. During the first endoscopy, although the alpha diversity index of the recipient’s ileal sample dropped to 1.59, the sample contained a large fraction of Bacteroidetes (26.3%) and Firmicutes (65.4%). During the second endoscopy, the alpha diversity indices of the samples increased (to 2.95), with expansions observed in both dominant phyla to include diverse Bacteroidetes and Firmicutes.
Antimicrobial resistance
Post-operatively, all recipients were heavily exposed to antimicrobial agents as shown in and described in supplementary data. shows the temporal variations in the carriage of AMR genes in SBT participants. There was no significant difference between the total number of AMR genes detected when comparing samples taken at time point 1 (day of transplant) and time point 2 (first endoscopy), with a median of 11 AMR genes detected at both timepoints. In contrast, there was a significant increase (p < 0.05) in the number of AMR genes detected between the second (first endoscopy) and third (second endoscopy) time points (). Importantly, we did not detect any AMR genes in donor samples collected on the day of transplantation.
Figure 3. Quantification of antimicrobial resistance genes (ARG) present in participant samples.

Table 2. Clinical summary of participants.
There was a high abundance of the van gene () (known to confer vancomycin resistance in Enterococcus species) detected in participants 2,3,4 and 5, along with mdt and acr genes, which encode multi-drug efflux pumps. The tetracycline efflux pump encoded by the tet gene was detected in all participants. Among the acquired AMR genes, trimethoprim (dfr), aminoglycoside (aac), macrolide, lincosamide, and streptogramin (erm) were detected in all five participants. Trimethoprim-sulfamethoxazole is commonly used as universal prophylaxis after SBT and solid organ transplantation from day 14. Both erm and aac genes are frequently carried by Enterococcus faecium.Citation41 We did not observe a significant negative association between alpha diversity at the species level and AMR carriage in our limited cohort.
Bacterial functional metabolic pathways
In this small SBT cohort, the functional bacterial metabolic pathways were differentially affected (). Significantly higher levels of specific metabolic genes were exclusive to Participant 4. Participant 1, who also had a good clinical outcome, had the least significantly altered functional pathway genes compared to participant 5. Seven pathways, the majority of which are involved in glucose and lipid metabolism, were found in significantly lower abundance across participants 1–4 when compared to participant 5.
Figure 4. Multivariable association matrix depicting the top 50 functional pathway genes with significant alterations.

Ileum and colonic gut microbiome composition
Principal coordinate analysis revealed the microbial composition of the donor, recipient ileum, and recipient colon samples. The ileal and colonic microbial compositions of the transplant recipients were concordant, as shown in .
Linking isolates from clinical infection episodes back to the gut microbiome
Selected bacterial samples (marked with an asterisk in ) underwent whole-genome sequencing. These were: participant 2’s VRE from a bacteremia episode at the end of their hospital stay, participant 3’s VRE isolated from body fluid, and Participant 4’s Klebsiella michiganensis isolated from peritoneal fluid. These organisms were present at high relative abundances in the gut microbiome prior to causing clinical infection.
InStrain analyses showed that all three of these isolates could be linked back to the gut microbiomes of their respective patients (highlighted in Supplementary data, ). Patient 3’s VRE isolate mapped to their effluent samples at the second follow-up endoscopy (3E2I, 3E2C) with a population-level ANI (popANI) of 100% and zero single-nucleotide polymorphisms (SNPs). Patient 4’s Klebsiella michiganensis isolate mapped to their first endoscopy samples (4E1I, 4E1C) with nearly 100% coverage overlap, 99.99% popANI and 1 SNP. Finally, Patient 2’s VRE isolate mapped to their fourth endoscopy samples (2E4I, 2E4C) with 99.99% popANI and 3 SNPs. Moreover, Patient 2 and Patient 3’s VRE isolates appeared to be similar at the strain-level (Supplementary figure s2), since both isolates mapped with gut microbiome samples from patients 2, 3 and 4 with high ANI values (99.9–100%), and 0–12 SNPs. This finding was confirmed with a FastANI value of 99.78 between the two VRE isolates and a MASH distance of 0.0026, indicative of shared sequence-type. MLST was performed in-silico to identify the exact sequence-type, which classified both isolates as ST80 Enterococcus faecium.
Discussion
This prospective cohort study used WMS to assess longitudinal variations in the intestinal microbiota of five SBT recipients at a major UK adult transplantation center. Our primary objective was to show a temporal shift in microbiota composition with respect to clinical infection and confirm our hypothesis that gut colonization can precede invasive infection by the dominant species. To extend this objective, we used WGS on isolates recovered from clinical infection episodes and attempted to map their genomes to the metagenomic bowel effluent data to assess bacterial translocation from the gut. We met our secondary objectives, reporting the changes after SBT of 1) AMR carriage and 2) genes encoding functional metabolic pathways in the gut bacteria. Overall, this study shows ecological shifts of the human microbiome in response to SBT, under the pressure of antimicrobials and immunosuppressive therapy, and establishes a baseline to measure the effects of future therapeutics.
Comparison to previous SBT cohorts
Our cohort was similar to global SBT cohorts in terms of age, indications for transplantation, and percentage of liver-containing transplantation, which are known to be associated with improved graft survival (but lower overall patient survival).Citation4,Citation12,Citation42 Our cohort’s relatively low survival rate at 1 year of 60% likely reflects our small group size. The rejection rate of 20% and sepsis rate of 60% in our cohort were comparable to previously published data.Citation5,Citation12 The organisms causing abdominal infections in our cohort are commonly observed in SBTs.Citation7,Citation43,Citation44
Pathogens outcompete the commensal microbiota before causing clinical infection
Many studies have suggested that the gut microbiome plays a critical role in patient outcomes following SBT. Approximately 90% of the 100 trillion members of the gut microbiome in healthy adults comprise two phyla: Bacteroidetes and Firmicutes,Citation45 which were present at high relative abundance in all five samples from participant 5. During surgery, exposure of the bowel lumen to oxygen alters the microbial community structure from consisting primarily of obligate anaerobes, such as Bacteroides and Clostridia to facultative anaerobes, such as Lactobacilli and Enterobacteriaceae.Citation11
In our small but otherwise typical SBT cohort, we observed an increased abundance of specific pathogens in the gut (Enterococcus faecium, Klebsiella spp., and Candida glabrata) before the onset of clinical infection. Furthermore, we identified that three isolates recovered from clinical infection episodes were either closely genetically related or identical sequence types to the abundant taxa in the gut microbiota. These microorganisms had colonized the gut at clinically meaningful intervals before manifesting as the apparent causes of clinical disease. This interval allows for the possibility of therapeutic intervention. Such interventions could involve targeted antimicrobial treatment or fecal microbiota transplantation (FMT), which have previously been employed in transplant patients colonized with multi-drug resistant (MDR) bacteria.Citation46 FMT has been successfully used in renal transplant patients (colonized with ESBL-producing E.coli) and renal/heart transplant patients (colonized with Clostridium difficile and VRE) to prevent subsequent clinical infections with these MDR organisms.Citation47
A previous study showed that microbiome changes in SBT recipients are temporally associated with the risk of infection as well as rejection episodes.Citation16 VRE, MDR, and extensively drug-resistant (XDR) Enterobacteriaceae and MDR/XDR non-fermenting gram-negative bacteria are increasingly recognized as common causes of infection in solid organ transplant patients.Citation43 Notably, expansions of the Enterococcus and Klebsiella genera and low pre-transplant alpha diversity in the gut have been associated with an increased risk of developing MDR infections in liver transplant recipients,Citation48 which could explain the rapid deterioration post-multi-visceral SBT in participant 4 in our study. Larger longitudinal metagenomic studies in SBT patients are required to ascertain the range of organisms capable of gut translocation, which could enable targeted screening strategies in the future.
Gut microbiota composition and acute rejection
A historic cohort study of 19 SBT patients showed differences in the microbiome of patients with and without acute graft rejection using 16S sequencing data, and suggested that a low abundance of Firmicutes and a high abundance of Proteobacteria could be associated with rejection.Citation49 Our study adds to the existing literature by offering insight into the impact of SBT on the microbiome and subsequent rejection and sepsis episodes in the immediate post-operative period with species-level resolution using metagenomic data. While we also found a high abundance of Proteobacteria in participants with complications post-SBT, we only described the clinical course of one participant with recurrent rejection episodes (Participant 2).
Carriage of enterococcus faecium and risk of infection
An increase in the order Lactobacillales has previously been proposed as a reliable marker for acute rejection.Citation49 However, our findings are in contrast, with four out of five participants in our study showing notable abundance of Enterococcus faecium, belonging to the order Lactobacillliales. Three of four VRE-colonized participants in our study developed clinically significant infections. Here, colonization status (both pre- and post-transplant) appeared to be a stronger predictor of post-transplant bacteremia, which aligns with previous reports of VRE infections in solid organ transplant recipients colonized with VRE.Citation50,Citation51 While this discrepancy could be attributed to our small sample size, the two studies recruiting patients of different age groups (predominantly pediatric vs. adults) or distinct geographical regions (USA vs. UK), with frequent reports of VRE in our institution,Citation52 it could also signal a transition toward an increase in nosocomial VRE in the UK.Citation53 The two VRE isolates we recovered and sequenced belonged to ST80, a highly-prevalent MDR strain which has been reported across Europe.Citation52,Citation54,Citation55
A previous study on SBT showed that post-transplant colonization with VRE is associated with a nine-fold increased risk of developing bacteremia compared to non-colonized hosts (36% versus 4%), but this did not translate into differences in one-year survival.Citation51 Collectively, these findings highlight the challenges of generalizing at higher taxonomic levels, limited by the resolution of the 16S microbiome data used in previous microbiome studies in SBT cohorts. As sequencing technologies become increasingly affordable, we emphasize the need for more comprehensive genus/species-level observations using whole-metagenome data for SBT patients across demographic strata.
Higher alpha diversities are associated with better outcomes
In this study, we noted that recipients with better outcomes received transplanted donor samples with higher alpha diversity. Through its competitive exclusion ability, a diverse gut microbiome can outcompete existing or introduced pathobionts and prevent them from colonizing the host.Citation56 In addition, mixed microbial populations in the gut create redundancies in key metabolic pathways, thereby permitting compensatory means of producing essential metabolic intermediates for healthy gut function.Citation57 We also observed that non-survivors in our cohort had alpha diversities below 2.5 at their final time point. These findings need to be replicated in larger studies to explore the possibility of donor selection based on alpha diversity in addition to the potential for post-operative surveillance of recipient gut microbiomes to monitor recovery. Beta-diversity was highly varied between samples taken from the same participant at different time points (data not shown), which contrasts findings in healthy subjectsCitation58 and potentially reflects the effects of the antimicrobial and immunosuppressive therapy patients received in the post-operative period.
AMR correlates with duration of inpatient stay
AMR is an emerging problem in transplanted patients.Citation59 Intensive antimicrobial use alters the gut microbiome and promotes colonization by MDR bacteria.Citation19,Citation60,Citation61 Recent guidelines for the management of MDR infections in transplant recipients call for studies to assess colonization using WGS to understand antimicrobial impact and the association between colonization and infection.Citation62 Our study provides preliminary metagenomic data in SBT recipients that could help inform strategies to tailor antimicrobial plans for individuals to lengthen the time to first bloodstream infection without encouraging the development of further AMR.Citation63
In the present study, there was an increase in the abundance and diversity of AMR genes over time. We suggest that this could be partially due to the timing of our sampling, which was conducted during a period of heavy immunosuppression and initiation of broad-spectrum antimicrobials in a hospital setting. The association with the length of hospitalization underlines the importance of early discharge and removal from a potentially contaminated hospital setting when possible. In this small study, we saw no AMR gene carriage in the donor grafts, despite previous studies suggesting that a healthy gut environment can be a reservoir for AMR genes.Citation64 We suspect that the absence of AMR gene detection despite adequate sequencing quality in donor samples can be attributed to the characteristics of individual donors. In the UK, deceased donors often suffer from cerebral vascular accidents and traumatic brain injuryCitation65, making them unlikely to be exposed to long courses of antimicrobials; therefore, there is limited selection pressure for AMR development in donor gut microbiomes.
Metabolomic exploration
The gut microbiota is known to modulate intestinal homeostasis by aiding energy metabolism through the exportation of key microbial metabolic products, such as short-chain fatty acids (SCFAs), amino acids, and vitamins.Citation66,Citation67 SCFAs such as acetate, propionate, and succinate constitute 10% of the daily caloric requirements of humans as important energy substrates for colonocytes and regulators of lipid and carbohydrate metabolism.Citation68 For example, Gram-negative Veillonella (Firmicutes) and Parabacteroides (Bacteroides), found in high abundance in participants 1 and 5 respectively (), are involved in the production of propionate and succinate which activate gluconeogenesis in the liver, and have been implicated in gut recovery.Citation69 The genes associated with the histidine degradation pathway, which produces histamine, were also found to be more abundant in participant 5. Microbiota-derived histamine from Lactobacillus spp. modulates the immune response by suppressing the pro-inflammatory effects of TNF-α in a host receptor-dependent manner.Citation70,Citation71 It is also noteworthy that participants 1, 3, and 5 had a high abundance of Lactobacillus spp. (Supplementary Data, Figure s1) and were all alive at 1-year. In addition, genes involved in the fatty acid beta-oxidation pathway (found in high abundance in participant 4) encode a restorative mechanism to maintain anaerobic conditions and prevent facultative anaerobes from proliferating and infiltrating the gut lumen upon exposure to oxygen.Citation72 As an emerging field, the true relevance of these and the remaining pathways detected in patient outcomes needs to be further validated in a larger study cohort with interdisciplinary collaborations to identify potential targets for intervention.
Limitations
Our study has several limitations. Primarily because of the small sample size, our findings are descriptive. Since the study was conducted in the post-pandemic period, we received relatively fewer follow-up endoscopy samples as post-transplant endoscopy sampling was streamlined. Future studies with larger sample sizes are needed to overcome this limitation.
We accept this study cannot begin to evaluate donor-related factors known to impact gut microbiome. It was not possible to collect donor data (age, gender, smoking status, weight, diet, known carriage of resistant bacteria) due to ethical constraints. With the scarcity of organs for transplant, clinicians presently may not be able to await an organ block with optimal microbiome alpha biodiversity, even if this analysis could be performed in a timely manner. However, there may be some utility in performing this if it could be used to correlate with clinical outcomes or predict patients more at risk of adverse outcomes. Further studies with the necessary ethical approvals could be considered.
Sample size also impacts sequencing analyses due to variability in the number of raw reads per sample during sample collection. We set our sequencing threshold at 30,000 reads per sample and excluded three samples from alpha diversity, AMR, and metabolomic analyses due to inadequate sequencing depth (see Methods). A larger cohort would enable the sequencing threshold to be set at 45,000 reads per sample for community-level analyses without excluding significant sample numbers. The Kraken2 analysis pipeline also misclassified Mycobacterium canetti and Toxoplasma gondii due to recognized gaps in reference genomes and a tendency for the program to misclassify human DNA as Toxoplasma gondii.Citation73 Additionally, the IgG pre-screen was negative for all five participants, which suggested false positive OTU assignments for the taxon. Therefore, these reads were removed from the downstream analyses. We were also unable to recover the isolate which caused VRE infection in participant 4, and therefore cannot validate the genetic similarity observed with the isolates recovered from participants 2 and 3, despite the data suggesting high ANI between these isolates and participant 4’s gut samples.
Finally, a larger sample size is required to thoroughly explore the impact of shifts in the metabolic potential of the microbiome on patient outcomes. Further steps would involve the investigation of correlations between metabolic pathway gene abundance and gene expression using RNA-seq. Thus, dysregulated pathways may be targets for future clinical trials that can translate knowledge in this area to promote the development of novel vaccines, monoclonal antibodies, FMT, targeted bacterial metabolic pathway alterations, and help generate microbiota-driven therapeutics.Citation47,Citation74 Despite these limitations, our study pioneers the use of WMS on longitudinal gut microbiota samples from SBT patients. We contribute new data to support the bacterial translocation hypothesis of gut-origin sepsis in patients undergoing intestinal transplantation and offer preliminary insights into AMR gene carriage and metabolic potential in the SBT cohort.
Conclusion
This metagenomic study adds to the body of evidence supporting the gut-origin sepsis theory in a rare cohort of SBT recipients, providing a rationale for initiating a similar, full-scale clinical study. We found preliminary evidence linking colonization by specific gut pathogens with episodes of sepsis. Ileal and colonic samples, matched by time point and participant, were concordant, which suggests that bedside fecal sampling from the stoma site could be an effective surveillance tool for impeding sepsis with specific bowel-residing pathogens. We found an increase in AMR gene abundance and diversity with an increase in inpatient stay duration. Finally, we showed that higher alpha diversities (in both donor and recipient microbiomes) were predictive of improved patient outcome. To aid future efforts to combat the threat of AMR and mortality associated with infections in SBT, this study is the first to capture the complete metagenomic landscape of the gut microbiome in patients post-SBT.
Authors contributions
Conception and design: EGK, JBS, SB, JPD
Recruitment of participants: KA, TD, RB
Collection of samples and clinical data: RB, HS, DM, LS, CR, JW, NR, IA, AB
Processing of samples: CC, RB, JPD, SF, LK
Analysis and interpretation of data: AM, EH, RB, JK, EGK
Drafting initial paper: RB, AM, EGK
Revising the final paper: RB, AM, CC, JPD, SF, JK, LK, EH, GD, HS, DM, LS, CR, JW, NR, IA, AB, KA, TD, JBS, SB, EGK
Revised_SBT_supplementary_data_18122023.docx
Download MS Word (14.7 MB)Acknowledgments
We thank the transplant nursing team for their assistance in this study. Most importantly, heart-felt thanks were expressed to the participants for selflessly supporting this study.
Disclosure statement
No potential conflict of interest was reported by the author(s).
Data availability statement
The authors confirm that the data supporting the findings of this study are available in the article and its supplementary materials. The microbial WMS reads for 40 patient samples and WGS reads for 3 bacterial isolates have been deposited in the NCBI Sequence Read Archive under BioProject accession PRJNA1004534.
Supplementary material
Supplemental data for this article can be accessed online at https://doi.org/10.1080/19490976.2024.2323232
Additional information
Funding
References
- Garcia J, Selvaggi G, Tekin A, Ruiz P, Tzakis A. Long-term outcomes after intestinal and multi-visceral transplantation. In: Kirk A, Knechtle S, Larsen C, Madsen J, Pearson T Webber S, editors. Textbook of organ transplantation [internet]. Oxford, UK: John Wiley & Sons, Ltd; 2014 [accessed 2023 Jul 12]. p. 1334–19. doi:10.1002/9781118873434.ch109.
- Global Observatory on Donation and Transplantation. International report on organ donation and transplantation activities [internet]. 2021:46. https://www.transplant-observatory.org/wp-content/uploads/2022/12/2021-data-global-report-1.pdf.
- NHS Blood and Transplant. Organ and tissue donation and transplantation: activity report 2021/22. UK: UK Transplant Registry; 2022.
- NHS Blood and Transplant. Annual report on intestine transplantation [internet]. England: NHS; 2022 Sep. p. 29. https://nhsbtdbe.blob.core.windows.net/umbraco-assets-corp/27817/nhsbt-annual-report-on-intestine-transplantation-202122.pdf.
- Florescu DF, Qiu F, Langnas AN, Mercer DF, Chambers H, Hill LA, Qaragholi N, Kalil AC. Bloodstream infections during the first year after pediatric small bowel transplantation. Pediatr Infect Dis J. 2012 Jul;31(7):700–704. doi:10.1097/INF.0b013e318256f9c3.
- Singh N, Limaye AP. Infections in solid-organ transplant recipients. In: Mandell, Douglas, and Bennett’s principles and practice of infectious diseases [internet]. Elsevier;2015 [acccessed 2023 Jul 12]. p. 3440–3452. https://linkinghub.elsevier.com/retrieve/pii/B9781455748013003131.
- Soltys KA, Reyes JD, Green M. Risks and epidemiology of infections after intestinal transplantation. In: Ljungman P, Snydman D Boeckh M, editors. Transplant infections [internet]. Cham: Springer International Publishing; 2016[accessed 2023 Jul 12]. p. 235–248. doi:10.1007/978-3-319-28797-3_15.
- MacFie J. Current status of bacterial translocation as a cause of surgical sepsis. Br Med Bull. 2005 Jan 1;71(1):1–11. doi:10.1093/bmb/ldh029.
- Deitch EA. Gut-origin sepsis: evolution of a concept. The Surgeon. 2012 Dec;10(6):350–356. doi:10.1016/j.surge.2012.03.003.
- Elsabbagh AM, Hawksworth J, Khan KM, Kaufman SS, Yazigi NA, Kroemer A, Smith C, Fishbein TM, Matsumoto CS. Long-term survival in visceral transplant recipients in the new era: a single-center experience. Am J Transplant. 2019 Jul;19(7):2077–2091. doi:10.1111/ajt.15269.
- Hartman AL, Lough DM, Barupal DK, Fiehn O, Fishbein T, Zasloff M, Eisen JA. Human gut microbiome adopts an alternative state following small bowel transplantation. Proc Natl Acad Sci U S A. 2009 Oct 6;106(40):17187–17192. doi:10.1073/pnas.0904847106.
- Grant D, Abu-Elmagd K, Mazariegos G, Vianna R, Langnas A, Mangus R, Farmer DG, Lacaille F, Iyer K, Fishbein T. et al. Intestinal transplant registry report: global activity and trends. Am J Transplant. 2015 Feb;15(1):210–219. doi:10.1111/ajt.12979.
- Grant D, Hurlbut D, Zhong R, Wang P, Chen H, Garcia B, Behme R, Stiller C, Duff J. Intestinal permeability and bacterial translocation following small bowel transplantation in the rat. Transplant. 1991 Aug;52(2):221–224. doi:10.1097/00007890-199108000-00007.
- Pollard S. Small bowel transplantation. Clin Colon Rectal Surg. 2004 May;17(2):119–124.
- Wu G, Selvaggi G, Nishida S, Moon J, Island E, Ruiz P, Tzakis AG. Graft-versus-host disease after intestinal and multivisceral transplantation. Transplant. 2011 Jan 27;91(2):219–224. doi:10.1097/TP.0b013e3181ff86ec.
- Wang C, Li Q, Li J. Gut microbiota and its implications in small bowel transplantation. Front Med. 2018 Jun;12(3):239–248. doi:10.1007/s11684-018-0617-0.
- Kato K, Nagao M, Miyamoto K, Oka K, Takahashi M, Yamamoto M, Matsumura Y, Kaido T, Uemoto S, Ichiyama S, et al. Longitudinal analysis of the intestinal microbiota in liver transplantation. Transplant Dir. 2017 Apr;3(4):e144. doi:10.1097/TXD.0000000000000661.
- Lee JR, Muthukumar T, Dadhania D, Toussaint NC, Ling L, Pamer E, Suthanthiran M. Gut microbial community structure and complications after kidney transplantation: a pilot study. Transplant. 2014 Oct 15;98(7):697–705. doi:10.1097/TP.0000000000000370.
- Taur Y, Xavier JB, Lipuma L, Ubeda C, Goldberg J, Gobourne A, Lee YJ, Dubin KA, Socci ND, Viale A. et al. Intestinal domination and the risk of bacteremia in patients undergoing allogeneic hematopoietic stem cell transplantation. Clin Infect Dis. 2012 Oct 1;55(7):905–914. doi:10.1093/cid/cis580.
- Wu T, Abu-Elmagd K, Bond G, Nalesnik MA, Randhawa P, Demetris AJ. A schema for histologic grading of small intestine allograft acute rejection. Transplant. 2003 Apr;75(8):1241–1248. doi:10.1097/01.TP.0000062840.49159.2F.
- Andrews S. FastQC: a quality control tool for high throughput sequence data [internet]. 2010. https://www.bioinformatics.babraham.ac.uk/projects/fastqc/.
- Beghini F, McIver LJ, Blanco-Míguez A, Dubois L, Asnicar F, Maharjan S, Mailyan A, Manghi P, Scholz M, Thomas AM, et al. Integrating taxonomic, functional, and strain-level profiling of diverse microbial communities with bioBakery 3. eLife. 2021 May 4;10:e65088. doi:10.7554/eLife.65088.
- Wood DE, Lu J, Langmead B. Improved metagenomic analysis with kraken 2. Genome Biol. 2019 Dec;20(1):257. doi:10.1186/s13059-019-1891-0.
- Lu J, Rincon N, Wood DE, Breitwieser FP, Pockrandt C, Langmead B, Salzberg SL, Steinegger M. Metagenome analysis using the kraken software suite. Nat Protoc. 2022 Dec;17(12):2815–2839. doi:10.1038/s41596-022-00738-y.
- Nurk S, Meleshko D, Korobeynikov A, Pevzner PA. metaSPAdes: a new versatile metagenomic assembler. Genome Res. 2017 May;27(5):824–834. doi:10.1101/gr.213959.116.
- Seemann T. ABRIcate [Internet]. 2015. https://github.com/tseemann/abricate.
- Alcock BP, Raphenya AR, Lau TTY, Tsang KK, Bouchard M, Edalatmand A, Huynh W, Nguyen ALV, Cheng AA, Liu S, et al. CARD 2020: antibiotic resistome surveillance with the comprehensive antibiotic resistance database. Nucleic Acids Res. 2019 Oct 29;gkz935. doi:10.1093/nar/gkz935.
- Breitwieser FP, Salzberg SL, Schwartz R. Pavian: interactive analysis of metagenomics data for microbiome studies and pathogen identification Schwartz R, editor. Bioinformat. 2020 Feb 15;36(4):1303–1304. doi:10.1093/bioinformatics/btz715.
- Wickham H, Francois R, Henry L, Müller K, Vaughan D. Dplyr: a grammar of data manipulation [internet]. 2023. https://CRAN.R-project.org/package=dplyr.
- Wickham H, Averick M, Bryan J, Chang W, McGowan L, François R, Grolemund G, Hayes A, Henry L, Hester J, et al. Welcome to the tidyverse. J Open Source Softw. 2019 Nov 21;4(43):1686. doi:10.21105/joss.01686.
- Oksanen J, Blanchet GF, Kindt R, Legendre P, Minchin PR, O’Hara RB. Vegan: community ecology package [internet]. 2015. https://cran.r-project.org/web/packages/vegan/index.html.
- R Core Team. R: a language and environment for statistical computing [internet]. Vienna, Austria: R Foundation for Statistical Computing; 2023. https://www.R-project.org/.
- Vu V, Marwick B, Hester J, Held M. Ggbiplot: an implementation of the biplot using ggplot2 [internet]. 2015. https://github.com/vqv/ggbiplot.
- Di Tommaso P, Chatzou M, Floden EW, Barja PP, Palumbo E, Notredame C. Nextflow enables reproducible computational workflows. Nat Biotechnol. 2017 Apr;35(4):316–319. doi:10.1038/nbt.3820.
- Underwood A. ghru-assembly [Internet]. Centre for genomic pathogen surveillance; 2020. https://gitlab.com/cgps/ghru/pipelines/dsl2/pipelines/assembly.
- Olm MR, Crits-Christoph A, Bouma-Gregson K, Firek BA, Morowitz MJ, Banfield JF. inStrain profiles population microdiversity from metagenomic data and sensitively detects shared microbial strains. Nat Biotechnol. 2021 Jun;39(6):727–736. doi:10.1038/s41587-020-00797-0.
- Langmead B, Salzberg SL. Fast gapped-read alignment with bowtie 2. Nat Methods. 2012 Apr;9(4):357–359. doi:10.1038/nmeth.1923.
- Jain C, Rodriguez RLM, Phillippy AM, Konstantinidis KT, Aluru S. High throughput ANI analysis of 90K prokaryotic genomes reveals clear species boundaries. Nat Commun. 2018 Nov 30;9(1):5114. doi:10.1038/s41467-018-07641-9.
- Ondov BD, Treangen TJ, Melsted P, Mallonee AB, Bergman NH, Koren S, Phillippy AM. Mash: fast genome and metagenome distance estimation using MinHash. Genome Biol. 2016 Dec;17(1):132. doi:10.1186/s13059-016-0997-x.
- Page AJ, Alikhan NF, Carleton HA, Seemann T, Keane JA, Katz LS. Comparison of classical multi-locus sequence typing software for next-generation sequencing data. Microb Genomic [Internet]. 2017 Jun 13[accessed 2023 Oct 26];3(8). doi:10.1099/mgen.0.000124.
- Miller WR, Munita JM, Arias CA. Mechanisms of antibiotic resistance in enterococci. Expert Rev Anti Infect Ther. 2014 Oct;12(10):1221–1236. doi:10.1586/14787210.2014.956092.
- Middleton SJ. The current status of small bowel transplantation in the UK and internationally. Gut. 2005 Nov 1;54(11):1650–1657. doi:10.1136/gut.2004.062612.
- Cervera C, van DC, Gavaldà J, Welte T, Akova M, Carratalà J. Multidrug-resistant bacteria in solid organ transplant recipients. Clin Microbiol Infect. 2014;20:49–73. doi:10.1111/1469-0691.12687.
- Spence AB, Natarajan M, Fogleman S, Biswas R, Girlanda R, Timpone J. Intra‐abdominal infections among adult intestinal and multivisceral transplant recipients in the 2‐year post‐operative period. Transpl Infect Dis [Internet]. 2020 Feb[accessed 2023 Jul 13];22(1). doi:10.1111/tid.13219.
- Rinninella E, Raoul P, Cintoni M, Franceschi F, Miggiano G, Gasbarrini A, Mele M. What is the healthy gut microbiota composition? A changing ecosystem across age, environment, diet, and diseases. Microorgan. 2019 Jan 10;7(1):14. doi:10.3390/microorganisms7010014.
- Saha S, Tariq R, Tosh PK, Pardi DS, Khanna S. Faecal microbiota transplantation for eradicating carriage of multidrug-resistant organisms: a systematic review. Clin Microbiol Infect. 2019 Aug;25(8):958–963. doi:10.1016/j.cmi.2019.04.006.
- Manges AR, Steiner TS, Wright AJ. Fecal microbiota transplantation for the intestinal decolonization of extensively antimicrobial-resistant opportunistic pathogens: a review. Infect Dis. 2016 Aug 2;48(8):587–592. doi:10.1080/23744235.2016.1177199.
- Annavajhala MK, Gomez-Simmonds A, Macesic N, Sullivan SB, Kress A, Khan SD, Giddins MJ, Stump S, Kim GI, Narain R. et al. Colonizing multidrug-resistant bacteria and the longitudinal evolution of the intestinal microbiome after liver transplantation. Nat Commun. 2019 Oct 17;10(1):4715. doi:10.1038/s41467-019-12633-4.
- Oh PL, Martínez I, Sun Y, Walter J, Peterson DA, Mercer DF. Characterization of the ileal microbiota in rejecting and nonrejecting recipients of small bowel transplants. Am J Transplant. 2012 Mar;12(3):753–762. doi:10.1111/j.1600-6143.2011.03860.x.
- Ziakas PD, Pliakos EE, Zervou FN, Knoll BM, Rice LB, Mylonakis E. MRSA and VRE colonization in solid organ transplantation: a meta-analysis of published studies. Am J Transplant. 2014 Aug;14(8):1887–1894. doi:10.1111/ajt.12784.
- Simkins J, Morris MI, Camargo JF, Vianna R, Beduschi T, Abbo LM. Clinical outcomes of intestinal transplant recipients colonized with multidrug-resistant organisms: a retrospective study. Transpl Int. 2017 Sep;30(9):924–931. doi:10.1111/tri.12987.
- Gouliouris T, Coll F, Ludden C, Blane B, Raven KE, Naydenova P, Crawley C, Török ME, Enoch DA, Brown NM. et al. Quantifying acquisition and transmission of enterococcus faecium using genomic surveillance. Nat Microbiol. 2020 Oct 26;6(1):103–111. doi:10.1038/s41564-020-00806-7.
- Guy R, Higgins H, Rudman J, Fountain H, Bennet KF, Hopkins KL, Demirjian A, Gerver SM, Mirfenderesky M, Henderson KL, et al. Antimicrobial resistance in England 2017 to 2021 (ESPAUR report 2021–22). In: ESPAUR 2022 [Internet] MDPI. 2023 [accessed 2023 Jul 13];3. https://www.mdpi.com/2673-9992/15/1/3.
- Egan SA, Kavanagh NL, Shore AC, Mollerup S, Samaniego Castruita JA, O’Connell B, McManus BA, Brennan GI, Pinholt M, Westh H. et al. Genomic analysis of 600 vancomycin-resistant enterococcus faecium reveals a high prevalence of ST80 and spread of similar vanA regions via is 1216E and plasmid transfer in diverse genetic lineages in Ireland. J Antimicrob Chemother. 2022 Feb 2;77(2):320–330. doi:10.1093/jac/dkab393.
- Fang H, Fröding I, Ullberg M, Giske CG. Genomic analysis revealed distinct transmission clusters of vancomycin-resistant enterococcus faecium ST80 in Stockholm, Sweden. J Hosp Infect. 2021 Jan;107:12–15. doi:10.1016/j.jhin.2020.10.019.
- Lawley TD, Walker AW. Intestinal colonization resistance. Immunol. 2013 Jan;138(1):1–11. doi:10.1111/j.1365-2567.2012.03616.x.
- Lozupone CA, Stombaugh JI, Gordon JI, Jansson JK, Knight R. Diversity, stability and resilience of the human gut microbiota. Nature. 2012 Sep;489(7415):220–230. doi:10.1038/nature11550.
- Priya S, Blekhman R. Population dynamics of the human gut microbiome: change is the only constant. Genome Biol. 2019 Dec;20(1):150. doi:10.1186/s13059-019-1775-3.
- Oriol I, Sabé N, Simonetti AF, Lladó L, Manonelles A, González J, Tubau F, Carratalà J. Changing trends in the aetiology, treatment and outcomes of bloodstream infection occurring in the first year after solid organ transplantation: a single-centre prospective cohort study. Transpl Int. 2017 Sep;30(9):903–913. doi:10.1111/tri.12984.
- Pérez-Cobas AE, Gosalbes MJ, Friedrichs A, Knecht H, Artacho A, Eismann K, Otto W, Rojo D, Bargiela R, von Bergen M. et al. Gut microbiota disturbance during antibiotic therapy: a multi-omic approach. Gut. 2013 Nov;62(11):1591–1601. doi:10.1136/gutjnl-2012-303184.
- Montassier E, Gastinne T, Vangay P, Al-Ghalith GA, Bruley Des Varannes S, Massart S, Moreau P, Potel G, de La Cochetière MF, Batard E. et al. Chemotherapy-driven dysbiosis in the intestinal microbiome. Aliment Pharmacol Ther. 2015 Sep;42(5):515–528. doi:10.1111/apt.13302.
- Pouch SM, Patel G. Multidrug-resistant gram-negative bacterial infections in solid organ transplant recipients—guidelines from the American society of transplantation infectious diseases community of practice. Clin Transplant. 2019 Sep[accessed 2023 Jul 13];33(9). doi:10.1111/ctr.13594.
- Chatani B, Garcia J, Biaggi C, Beduschi T, Tekin A, Vianna R, Arheart K, Gonzalez IA. Comparison in outcome with tailored antibiotic prophylaxis postoperatively in pediatric intestinal transplant population. Pediatr Transplant. 2018 Nov;22(7):e13277. doi:10.1111/petr.13277.
- Brinkac L, Voorhies A, Gomez A, Nelson KE. The threat of antimicrobial resistance on the human microbiome. Microb Ecol. 2017 Nov;74(4):1001–1008. doi:10.1007/s00248-017-0985-z.
- Rosen RD, Singh A, Burns B. Trauma organ procurement [Internet]. 2023 Feb. https://www.ncbi.nlm.nih.gov/books/NBK555947/.
- Jandhyala SM. Role of the normal gut microbiota. World J Gastroenterol. 2015;21(29):8787. doi:10.3748/wjg.v21.i29.8787.
- Rowland I, Gibson G, Heinken A, Scott K, Swann J, Thiele I, Tuohy K. Gut microbiota functions: metabolism of nutrients and other food components. Eur J Nutr. 2018 Feb;57(1):1–24. doi:10.1007/s00394-017-1445-8.
- Carding S, Verbeke K, Vipond DT, Corfe BM, Owen LJ. Dysbiosis of the gut microbiota in disease. Microb Ecol Health Dis [Internet]. 2015 Feb 2 [accessed 2023 Feb 9]; 26(8). 10.3402/mehd.v26.26191.
- De Vadder F, Kovatcheva-Datchary P, Goncalves D, Vinera J, Zitoun C, Duchampt A, Bäckhed F, Mithieux G. Microbiota-generated metabolites promote metabolic benefits via gut-brain neural circuits. Cell. 2014 Jan;156(1–2):84–96. doi:10.1016/j.cell.2013.12.016.
- Thomas CM, Hong T, Van Pijkeren JP, Hemarajata P, Trinh DV, Hu W, Britton RA, Kalkum M, Versalovic J. Histamine derived from probiotic lactobacillus reuteri suppresses TNF via modulation of PKA and ERK signaling. PloS One. 2012 Feb 22;7(2):e31951. doi:10.1371/journal.pone.0031951.
- Moro J, Tomé D, Schmidely P, Demersay TC, Azzout-Marniche D. Histidine: a systematic review on metabolism and physiological effects in human and different animal species. Nutr. 2020 May 14;12(5):1414. doi:10.3390/nu12051414.
- Cani PD. Human gut microbiome: hopes, threats and promises. Gut. 2018 Sep;67(9):1716–1725. doi:10.1136/gutjnl-2018-316723.
- Lu J, Salzberg SL, Sun F. Removing contaminants from databases of draft genomes. PLoS Comput Biol. 2018 Jun 25;14(6):e1006277. doi:10.1371/journal.pcbi.1006277.
- Wood NJ. Potential of intestinal microbiota profile as a diagnostic biomarker of small bowel transplant rejection. Nat Rev Gastroenterol Hepatol. 2012 Feb;9(2):61–61. doi:10.1038/nrgastro.2011.261.