ABSTRACT
Inflammatory bowel disease (IBD) is a chronic and debilitating condition of relapsing and remitting inflammation in the gastrointestinal tract. Conventional therapeutic approaches for IBD have shown limited efficacy and detrimental side effects, leading to the quest for novel and effective treatment options for the disease. Bacterial membrane vesicles (MVs) are nanosized lipid particles secreted by lysis or blebbing processes from both Gram-negative and Gram-positive bacteria. These vesicles, known to carry bioactive components, are facsimiles of the parent bacterium and have been implicated in the onset and progression, as well as in the amelioration of IBD. This review discusses the overview of MVs and their impact in the pathogenesis, diagnosis, and treatment of IBD. We further discuss the technical challenges facing this research area and possible research questions addressing these challenges. We summarize recent advances in the diverse relationship between IBD and MVs, and the application of this knowledge as a viable and potent therapeutic strategy for IBD.
1. Introduction
Inflammatory bowel disease (IBD) is a chronic intestinal inflammation and mucosal immune-associated illness that involves dysbiosis of the intestinal microenvironment ,Citation1–3 The two main subtypes of IBD, ulcerative colitis (UC) and Crohn’s disease (CD), are typified by debilitating and chronic relapsing and remitting inflammation in the colon and gastrointestinal tract (GIT).Citation4 Although the cause of IBD is still unclear, it has been described as multifactorial, involving the combination and interplay of genetic susceptibility, immune dysregulation, microbial factors, and environmental triggers.Citation5,Citation6 While some conventional medications exist for the treatment of IBD with 5-aminosalicylates (5-ASAs), corticosteroids, and immunosuppressive agents as mainstay drugs,Citation7 they have, however, shown limited efficacy and detrimental side effects leading to the quest for new and effective treatment options for the disease.
The relationship between IBD and the gut microbiota has been well established by many studies,Citation8–11 The gut microbiota is vital in maintaining intestinal homeostasis and function, integrity of the epithelial barrier, and health and disease. The biodiversity and number of gut microbiota can be shaped by a variety of factors ranging from exposure to antibiotics, exogenous enzymes, prebiotics, probiotics, fecal microbiota transplantation, diet, and a host of diseases ,Citation12–14 These factors can in turn, cause a disruption of the microbiota, leading to an abnormally composed microbiota referred to as “dysbiosis” as opposed to “eubiosis.” A large number of human disease conditions, including but not limited to diabetes type II, allergies, colorectal cancer, obesity, cardiovascular diseases, and IBD have been linked to an altered composition of the microbiota.Citation13,Citation15,Citation16 Accumulating evidence indicates that bacteria release vesicles that facilitate the actions of the microbiota by transferring and delivering effector chemicals into host cells that modulate host signaling pathways and cell activities. Thus, vesicles secreted by the gut microbiota could have a significant impact on the health and illness of the host.Citation17
Bacterial membrane vesicles (MVs) are nano-sized lipid-bilayered vesicular structures composed of various immunostimulatory components.Citation18 The sizes range from 20 to 400 nm in diameter for Gram-positive bacteriaCitation19 and 20 to 250 nm for Gram-negative bacteria.Citation20,Citation21 MVs, which were originally discovered to be generated through controlled blebbing of the outer membrane of Gram-negative bacteria, and referred to as outer membrane vesicles (OMVs),Citation22 were initially disregarded as bacterial artifacts. Early investigations, conducted in the 1960s depicted OMVs being released from the outer membrane of various Gram-negative bacteria through electron microscopy. Nonetheless, it was not until the detection of OMVs in the spinal fluid of meningococcal patients that curiosity arose in comprehending OMV generation, their roles within the host, and their advantageous attributes for bacteria. Citation23–25 Relative to Gram-negative bacteria, Gram-positive bacteria lack an outer membrane but instead contain a thick peptidoglycan cell wall, resulting in the initial disinterest in MVs research for the bacteria. Although vesicle-like blebbing structures were reported on the surface of Bacillus spp., it was not until 2009 that the first characterization of MVs from the Gram-positive bacteria, Staphylococcus aureus was made with the aid of mass spectrometry.Citation19 MVs from both Gram-negative and Gram-positive bacteria perform functions that influence diverse biological processes, which can either be between bacteria – bacteria or bacteria – host cells.Citation20,Citation22,Citation26 In recent times, studies have revealed that MVs are implicated in the onset and progression, as well as in the treatment of IBD.Citation27–31
In this review, we introduce MVs and give a general overview of their biogenesis, composition, and functions. We focus on the potential involvement of MVs in the onset and progression of IBD, as well as in the diagnosis and treatment of the disease . We discuss specific roles played by MVs in their interactions with the gut microbiota, intestinal epithelial cells (IECs), and immune system, that could trigger and/or exacerbate inflammation. We reveal the potential of MVs as diagnostic biomarkers of IBD and therapeutic agents, either as the active ingredient or as a drug carrier. The present review also explores the possibility of harnessing MVs for IBD vaccines and in genetic engineering to broaden and enhance therapeutic outcomes. Additionally, we present a critical analysis of the present challenges facing MVs-IBD research and propose future research paths that could be explored to tackle these challenges.
2. Overview of MVs
Extracellular vesicles (EVs) are nano-sized particles surrounded by a lipid bilayer. They are excreted by a cell to the extracellular environment. Cells from the three domains of life, Archaea, Bacteria, and Eukarya, produce EVs, and their release follows a common and possibly conserved process within various species,Citation32–34 (). While the EVs from Gram-negative bacteria are called OMVs, vesicles from Gram-positive bacteria are known as membrane vesicles (MVs) or cytoplasmic membrane vesicles (CMVs) due to lack of an outer membrane in the bacteria and their mode of formation.Citation22,Citation35
Figure 1. Formation of MVs from the three domains of life and factors that influence their secretion.
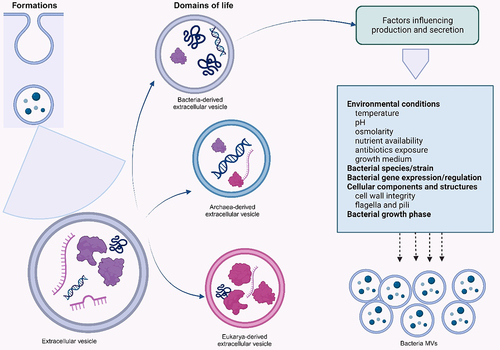
MVs production and secretion are majorly influenced by the expression and regulation of the parent bacterial genes, producing bacterial species, bacterial growth phase, cellular components and structures, and environmental conditions including the bacteria’s growth conditions.Citation36,Citation37
2.1 Biogenesis
Several studies have described two key routes for the generation of MVs in Gram-negative bacteria: blebbing of the outer membrane and endolysin-triggered cell lysis.Citation4,Citation22 Blebbing of the outer membrane in Gram-negative bacteria is reported to occur as a result of a disturbance in the cell envelope due to intercalating of the hydrophobic molecules into the outer membrane or from the unbalanced biosynthesis of the cell membrane.Citation4,Citation22 Three mechanisms that involve membrane blebbing have been described and these include the reduced cross‐linking between the outer membrane and the underlying peptidoglycan,Citation38,Citation39 the accumulation of peptidoglycan fragments or misfolded proteins in the periplasmic space,Citation39 and vesicles derived from bacterium flagellar-sheaths upon rotation of the flagella.Citation39
Various disturbances, such as an imbalance of peptidoglycan biosynthesis, could consequently lead to disruption of crosslinking between peptidoglycan and the outer membrane, causing dissociation of the outer membrane from the peptidoglycan layer.Citation40 Studies have shown that certain bacteria, such as Escherichia coli, Vibrio cholerae, and Salmonella spp. mutants deficient in OmpA (an outer membrane porin bearing a periplasmic binding site for diaminopimelic acid, which is a component of peptidoglycan), exhibit increased release of their MVs compared to the wild-type strains.Citation20,Citation40,Citation41 The hypervesiculating nlpI mutant has around 40% less lipoprotein crosslinked to peptidoglycan than wild-type E. coli.Citation42 Nlpl is an outer membrane lipoprotein involved in cell division and in the regulation of the activity of Spr (MepS), a peptidoglycan endopeptidase that breaks down peptide crosslinks in peptidoglycan.Citation20,Citation43 Hence, it is proposed that the altered balance of peptidoglycan synthesis and breakdown in nlpI mutants inhibits the development of appropriate crosslinks between peptidoglycan and lipoprotein and, consequently, increases the release of MVs.Citation20 The buildup of peptidoglycan fragments or misfolded proteins in the periplasmic space is the second mechanism that results in the generation of MVs by blebbing, as displayed by E. coli and P. aeruginosa.Citation39,Citation44,Citation45 Mutants of Porphyromonas gingivalis deficient in autolysin demonstrated an increase in MVs production, and this emanated from the inability of the bacterium to breakdown periplasmic peptidoglycan fragments that accumulated in the periplasm due to the lack of autolysins in P. gingivalis.Citation46,Citation47 Bacteria with mutations in their envelope stress pathways are incapable of protein degradation, and this can result in the accumulation of misfolded proteins, which exert pressure on the membrane of these bacteria, ultimately leading to increased MV secretion. As reported by McBroom and Kuehn in their study, higher growth temperatures cause an increase in the vesiculation of E. coli.Citation48 Lastly, the assembly of bacteria flagella, particularly sheathed flagella, also occasion membrane blebbing of vesicles. The flagella are surrounded by a sheath derived from the outer membrane and, upon rotation, release MVs, and this phenomenon has been reported to occur in members of Vibrio spp.Citation49
Endolysin-triggered cell lysis, on the other hand, involves vesicle formation routes based on the enzymatic actions of endolysins, typically employed by double-stranded DNA phages that utilize these peptidoglycan-hydrolyzing enzymes in the lysis of their hosts for the release of their progeny. Consequently, the cells round up and explode releasing fragments of shattered membrane that round up and self-assemble into E-type MVs.Citation50 This type of MV biogenesis has been observed in P. aeruginosa.Citation51 MVs that arise from Gram-negative bacteria’s explosive cell lysis carry endolysins and have the ability to lyse other cells,Citation52 generating new MVs.Citation34
In Gram-positive bacteria, MVs are released by a process known as “bubbling cell death,” which is somewhat similar to explosive cell death in Gram-negative bacteria. This process of MV biogenesis has been observed in Bacillus subtilis,Citation53 Lacticaseibacillus casei,Citation54 and in other Gram-positive bacteria as well. Citation55–57 A sub-population of cells of B. subtilis express a prophage-encoded endolysin causing holes in the peptidoglycan cell wall. As a result, materials of the cytoplasmic membrane bulges into the extracellular area and is released as MVs.Citation53 Endolysins secreted from dying B. subtilis cells have been demonstrated to cause MV formation in nearby cells by hydrolyzing the cell wall from the outside. In S. aureus, a type of blebbing mechanism has been proposed for MV biogenesis. It involves the disruption of the cytoplasmic membrane by amphipathic, α-helical, phenol-soluble modulins. Subsequently, autolysins, which weaken the crosslinking of the peptidoglycan, modulate MV release through the cell wall.Citation58 Peptidoglycan-hydrolyzing enzymes or β-lactam antibioticsCitation57,Citation59–61 also promote the weakening of the cell envelope, resulting in MV formation in some Gram-positive bacteria.
2.2. Composition
The cargo molecules in MVs are diverse due to variations in the parent bacteria, the biogenesis route, and other environmental factors. This diversity facilitates the roles MVs play in bacteria-bacteria and bacteria-host interactions. The MVs of Gram-negative bacteria have been reported to contain numerous parental components, including enzymes, lipopolysaccharides (LPS), lipooligosaccharides (LOS), proteins, nucleic acids, phospholipids, outer membrane proteins (OMPs), periplasmic and cytoplasmic proteins, cell wall components, ions, metabolites, and signaling molecules.Citation20,Citation62 Unlike MVs from Gram-negative bacteria, Gram-positive bacteria MVs lack LPS and periplasmic components, while other cargo molecules, including peptidoglycan, lipids, lipoproteins, proteins, and nucleic acids, remain the same.Citation19 Lipoteichoic acid (LTA), however, is an exclusive component of the Gram-positive bacteria MVs.Citation19 Pathogens, toxins, and virulent factors are also part of the MVs’ composite of both bacteria Gram-typesCitation17 (). The differences between MVs produced by Gram-positive and Gram-negative bacteria are summarized in .
Figure 2. Overview of bacterial membrane vesicles.
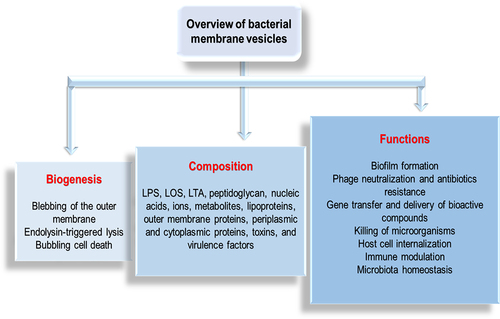
Table 1. Differences between MVs produced by Gram-negative bacteria and Gram-positive bacteria.
Many factors such as bacteria growth stage, conditions of the growth medium, and other environmental factors affect the generation and content of MVs. For instance, The culture of Vibrio vulnificus under optimized conditions of 37°C in an enriched medium of 2 × Luria Bertani in the presence of EDTA significantly increased the production of their MVs by about 70%.Citation66 Again, MVs derived from P. gingivalis at different growth stages not only determined the MV yield but also the protein content and periodontal pathogenicity of these MVs. MVs were extracted in the pre-log, late-log, and stationary growth phases of the bacteria, and it was reported that significantly increased yield, protein composition, and pathogenicity were associated with MVs from the stationary phase of growth.Citation67
The content of MVs has been analyzed using various methods including bicinchoninic acid (BCA) assay, Sodium Dodecyl Sulfate Polyacrylamide Gel Electrophoresis (SDS/PAGE), Western blotting, enzyme-linked immunosorbent assay (ELISA), mass spectrometry (MS), and colorimetric assays.Citation68,Citation69 While the total protein concentration of MVs is quantitatively determined by the BCA assay, SDS/PAGE is a qualitative determination of the total protein content of MVs on a polyacrylamide gel. The presence of a target protein is determined by ELISA and Western blotting. The mass-charge-to-charge ratio (m/z) of gaseous samples can be measured and analyzed in a vacuum environment using the MS technique. With the aid of this high-throughput proteomic analysis, thousands of proteins have been detected and this serves to reveal substantial evidence that supports the biogenesis and functions of MVs.Citation68,Citation69 Colorimetric-based assays are employed to ascertain the quantity of LPS present in the MVs. Some examples are the KDO (2-keto-3-deoxyoctonate) assay, (KDO is an essential sugar component of LPS) and Limulus Amebocyte Lysate (LAL) assay.Citation69–71 Protein assays, including BCA, Bradford, Lowry, or Qubit assays are the most extensively used methods for quantifying MVs for functional assays. MV protein content, however, can be considerably altered by factors such as bacteria growth stage,Citation67,Citation72–74 MV size,Citation75 culture conditions,Citation76–78 bacterial strains,Citation79 and MVs isolation method,Citation73,Citation80,Citation81 indicating that MV protein concentration and the quantity of MVs may not be directly correlated.Citation82 This reveals that the best method(s) to administer MVs for functional assay purposes need to be determined in order to increase the level of objectivity obtainable in comparative studies of MVs.
2.3. Functions
MVs perform important functions (determined by the MV’s structure and composition which are dependent on its biogenesis route), leading to their diverse roles in bacteria and their hosts. These vesicles perform functions that influence diverse biological processes, and which can either be between bacteria – bacteria or bacteria – host cells.Citation20,Citation22,Citation26 These functions, which include biofilm formation, gene transfer, antibiotics and phage neutralization, host cell internalization, disease progression, immune modulation, and microbiota homeostasisCitation68 () are briefly described below:
Figure 3. Composition and functions of MVs from Gram-positive and Gram-negative bacteria.
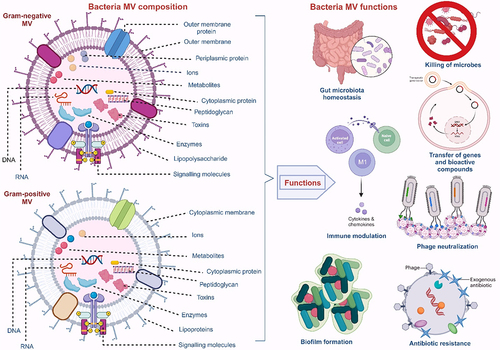
2.3.1. Biofilm formation
MVs in microbial communities are known to be key players in biofilm formation by enhancing the stability of the biofilm matrix, and in the facilitation of bacterial colonization due to their ease of spread on biofilm surfaces.Citation33 Various reports reveal that hydrophobic quorum-sensing molecules that coordinate bacterial growth and behavior, depend on the population density, and are secreted into MVs. Studies have also revealed that MVs are essential components of the biofilm matrix, usually composed of lipids, proteins, nucleic acids, and polysaccharides.Citation83 MVs, thus, transport the necessary molecules that promote biofilm formation. Various studies have elucidated the vital role of extracellular genomic DNA (eDNA) in the onset and stabilization of biofilms. The presence of eDNA in the MVs of S. aureus,Citation84 Acinetobacter baumannii, Francisella spp,Citation85 and P. aeruginosaCitation86 promotes biofilm formation.Citation20,Citation39 Some of these pathogens have been implicated in various nosocomial infections and burn wounds, with an increased incidence of chronic infections due to the formation of biofilms. 84,86 The implications of MVs in biofilm formation and IBD progression are discussed in the next section.
2.3.2. Phages neutralization and antibiotics resistance
Agents that bind to bacterial membranes will be adsorbed to MVs. As a result, MVs neutralize antibiotics such as colistin, daptomycin, and polymyxin that target the bacterial membraneCitation87 as observed in MVs of E. coli.Citation87,Citation88 MVs are also known to release enzymes that confer antibiotic resistance to the parent bacteria and other susceptible bacteria in the microbial communityCitation17,Citation89 (). For instance, S. aureus and Moraxella catarrhalis carry biologically active β-lactamase in their MVs.Citation89,Citation90 MVs can also provide antibiotic protection to both the producer strain and other bacterial populations in a given environmentCitation88 and offer protection against host-defense factors such as antimicrobial peptides from mammalian tissue and complement system factors of the blood.Citation57
Figure 4. MVs in phage neutralization, antibiotics resistance, gene transfer, and delivery of bioactive compounds.
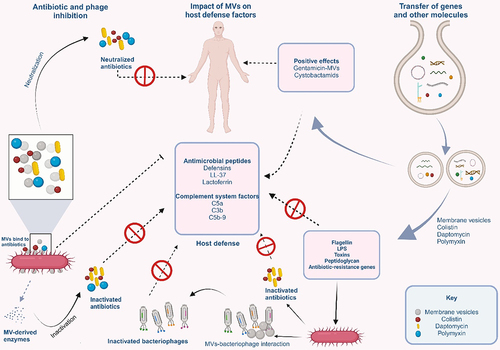
Additionally, MVs serve to prevent adsorption of phages onto bacteria. This is because the attachment of MVs onto the surface of the producer strain occupies the phage receptors, thereby preventing their binding onto the bacterial cell surface. The phages are then made to bind on the surface of the MVs through the phage receptor proteins on the surfaces of the MVsCitation91,Citation92 (). While MVs from E. coli were reported to neutralize T4 phage, those from V. cholerae neutralize ICP1, CIP2, and ICP3 phages.Citation87,Citation93
In summary, MVs sequester phages and antibiotics greatly reducing their availability so that they have no direct interaction with the parent bacteria. These observations show the involvement of MVs in the occurrence of antibiotic-resistant bacteria strains.Citation94
2.3.3. Gene transfer and delivery of bioactive compounds
Until recently, conjugation, transformation, and transduction were the three major gene transfer mechanisms. However, the discovery of MVs defined a new pathway for gene transfer. The genetic material of up to 370 kb has been discovered in the MVs of Gram-positive, Gram-negative, and archaeal microorganisms. All genetic materials, including chromosomal and plasmid-derived DNA, and RNA variants, have been found in MVsCitation95,Citation96 (). The interesting study of Carvalho and collaborators demonstrated that engineered MVs from Bacteroides thetaiotamicron (Bt-MVs) packaged and expressed both Salmonella enterica serovar Typhimurium-derived vaccine antigens and influenza A virus (IAV)-derived vaccine antigens within or on the outer membrane of Bt-MVs.Citation97 These antigens were shown to possess the ability to trigger antibody and antigen-specific immune responses in both mucosal tissues and systemically. This means that MVs can serve as vehicles in the delivery of genetic materials for novel biotechnological applications. Engineered MVs are being developed as new vaccines and adjuvants or as specialized drug delivery vehicles for the treatment of such diseases as cancer.Citation98,Citation99
2.3.4. Killing of microorganisms
MVs have the ability to interact with bacteria and other organisms, including eukaryotes and plants.Citation68,Citation100 Certain bacterial strains belonging to Pseudomonas, Enterobacter, Klebsiella, and Citrobacter genera have been reported to secrete toxin-carrying MVs that can kill other bacteria in a competitive environment.Citation101 Besides the killing of other bacteria, some bioactive compounds and lytic enzymes present in MVs can also kill fungi. This can be observed in the MVs of members of the genera Lysobacter and Myxococcus that lyse and feed on microorganisms. These MVs contain abundant hydrolytic enzymes, which they use to attack their prey. An example is the lytic protease L5 in Lysobacter spp. XL1.Citation57,Citation102
2.3.5. Host cell internalization
Uptake of MVs by host cells and delivery of their cargo into host cells must occur for a successful interaction between host cells and MVs. For instance, the internalization of LPS-containing MVs of E. coli BL21 by human intestinal epithelial cells resulted in the downregulation of E-cadherin expression, and intestinal barrier dysfunction further exacerbating inflammation. Uptake of MVs by non-phagocytic cells has been proposed to occur through five mechanisms, which are macropinocytosis, clathrin-mediated endocytosis, lipid raft-mediated endocytosis, caveolin-mediated endocytosis, and direct membrane fusionCitation103,Citation104 (). Moreover, the mechanism by which MVs enter the host cells depends on the size and cargo of the MVs.Citation47
Figure 5. Internalization of membrane vesicles into host cells and modulation of the immune system.
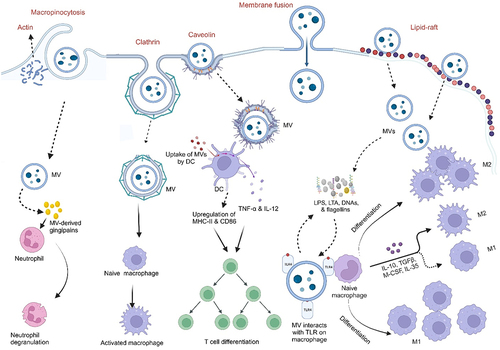
Macropinocytosis is channel utilized by viruses, which are similar in size to MVs, and is proposed to be a possible uptake mechanism of MVs by host cells.Citation105 Macropinocytosis, which is dependent on actin, involves the formation of large, ruffled protrusions from the cell membrane that permit the sampling and internalization of extracellular medium.Citation104,Citation106 The formation of clathrin-coated pits of up to 200 nm in diameter is indicative of clathrin-mediated endocytosis (CME). Here, ligand binding to cell surface receptors can initiate internalization, and dynamin is also needed for the budding off of the vesicle. Unlike macropinocytosis, CME is a well-defined mechanism for invading and pinching off portions of the cell membrane, allowing the entry of such molecules as MVs.Citation104 MVs from Helicobacter pylori,Citation107 Lactiplantibacillus plantarum BGAN8, and nonpathogenic E.coli Nissle 1917 (EcN) and ECOR12Citation108 are taken up by host cells via this mechanism.
Regions of the plasma membrane enriched in sphingolipids, and cholesterol are known as lipid-rafts. The clustering of cholesterol (which is the major component of the lipid raft) and other lipids in these domains allows the curvature of the membrane, driving the formation of invaginations in the host cell and entry of particles such as MVs into the cell.Citation104 MV cargos also aid in facilitating entry into host cells via lipid raft-mediated processes. MVs from Pseudomonas aeruginosaCitation104 and Moraxella catarrhalisCitation68 have been shown to be taken up by the host cell via lipid raft machinery. Caveolin-mediated endocytosis involves the presence and the oligomerization of caveolin in lipid rafts which give rise to the formation of caveolae – cave‐shaped invaginations that are around 80 nm in diameter and are formed on the cell membrane, with cholesterol, caveolins, and sphingolipids in abundance.Citation104,Citation109 Just as in CME, dynamin is also required here.Citation109 MVs from V. choleraeCitation110 and Haemophilus influenzaeCitation111 have been demonstrated to enter the host cell via this mechanism.
Lastly, direct membrane fusion has been demonstrated as another mechanism of MVs’ entry into host cells.Citation104 Membrane fusion preferentially take-place at lipid-raft regions and many studies have reported an increased surface area of the host membrane upon the addition of MVs-membrane on a model membrane with dye-labeling procedure.Citation104,Citation112 MVs from P. aeruginosaCitation113 and Legionella pneumophilaCitation112 were taken up via this mechanism. On interaction with eukaryotic cells, the cargo(s) is/are delivered to the host, and appropriate function(s) mediated.Citation64,Citation68
2.3.6. Immune modulation
MVs can influence host immune responses by modulating the expression of immune-related genes and by directly interacting with immune cells. They contain immunomodulatory molecules that can target host innate immune pattern recognition receptors (PRR) such as Toll-like receptors (TLRs) and Nod-like receptors (NLRs) signaling pathways, thereby stimulating the release of pro-inflammatory cytokines and chemokines, which attract immune cells to the site of inflammation.Citation114 As a result of the small sizes of MVs and their immunogenicity, their interaction with innate immune cells (macrophages and neutrophils), antigen-presenting cellsCitation115(dendritic cells), and/or adaptive immune cells (T- and B- cells), leads to the generation of various immune responses as illustrated in .Citation115,Citation116 For instance, the detection of LPS and LOS by TLR-4 results in the activation of nuclear factor-kappa B (NF-κB) and the release of proinflammatory cytokines. MVs of many pathogenic Gram-negative bacteria, including E. coli and P. aeruginosa can activate TLR-4.Citation117,Citation118 Additionally, MVs of Gram-positive bacteria such as S. aureus contain lipoproteins and other components that activate TLR-2 signaling in epithelial cells and macrophages, eliciting pro-inflammatory cytokine responses.Citation57,Citation59,Citation119 Internalized MVs can also activate host cytosolic PRRs. Almost all peptidoglycans from Gram-negative bacteria have a conserved structural motif that is recognized by NOD1. Entry of MV-associated peptidoglycan into epithelial cells activates NOD1, leading to the activation of NF-κB and the upregulation of human β-defensins 2 and 3.Citation120 NOD2, which detects a conserved peptidoglycan motif exclusive to both Gram-positive and Gram-negative bacteria, is also activated.Citation119,Citation121 Nucleic acids contained in MVs also activate NOD2, resulting in NF-κB activation, as seen in S. aureus-derived MVs.Citation119 MVs from the probiotic EcN and the commensal ECOR12 indirectly activate the innate immune response in IECs. These MVs activated NOD1 signaling pathways in IECs and subsequently triggered NF-κB signaling through the NOD1-RIP2 pathway.Citation108 Another study reported that MVs from EcN directly activated DCs, and these activated DCs induced the differentiation of Treg cells (FOXP3+).Citation122 These studies show that MVs are effective in modulating intestinal immune responses and can be strategically applied as novel therapeutic agents in IBD.
Additionally, MVs have been implicated in bacterial pathogenesis as they can serve as long-distance delivery vehicles to stimulate the immune system, promote host colonization, and enhance immune evasion. Certain immunogenic molecules such as flagellin, peptidoglycan, toxins, and LPS, which stimulate the host immune system through TLRsCitation123 and/or NLRsCitation108 are contained in MVs. These molecules are also linked to some virulence factors of the bacteria, including adherence, invasion, immune system modulation, and antimicrobial resistance. MVs can also contain more than one virulence factor simultaneously.Citation20,Citation47 Detailed exploration of immune modulation by MVs with respect to IBD is found in succeeding sections.
2.3.7. Microbiota homeostasis
Studies have shown that MVs are intimately involved in the communication between the gut microbiota and the host via a complex network of signaling pathways. These MVs play critical roles in the modulation of the gut microbiota homeostasis as they shape the immune responses of the host. MVs from Clostridium butyricum,Citation124 Lactobacillus rhamnosus,Citation31 Lactobacillus plantarum,Citation30 Akkermansia muciniphila,Citation29 Faecalibacterium prausnitzii,Citation125 among others have been reported to efficiently modulate the gut microbiota balance via various mechanisms which are further explored in subsequent sections. MVs from many pathogenic bacteria such as Fusobacterium nucleatum (Fn) and E. coli can contribute to dysbiosis of the gut causing an imbalance in the gut microbiota homeostasis.
3. MVs and their potential role in IBD
3.1. MVs and the gut-microbiota
Crosstalk between epithelial and immune cells is crucial for maintaining intestinal homeostasis in the human gut. MVs secreted by intestinal bacteria can diffuse in the intestinal microenvironment or enter the bloodstream. After the internalization and cargo delivery of MVs into their target cells, specific signaling pathways for further processes are activated.Citation126 It is interesting to note that MVs produced by a species of bacteria can impact the growth, reproduction, and colonization of members of the producing species differently. The MVs in the gut can be beneficial or harmful to the microflora and the host cell. In the host, for instance, MVs can regulate immunity (via interactions between epithelial and host cells) and promote the growth and colonization of probiotics, thereby maintaining microbial homeostasis. These are favorable to the host. MVs produced by commensal and probiotic bacteria in the human GIT can facilitate interactions amongst the host’s epithelial and immune cells, maintain microbiota homeostasis, and offer protection against diseases.Citation97,Citation127 On the other hand, MVs from pathogenic bacteria can damage the host’s mucosal barrier, causing harmful inflammatory storms to the host.Citation128
Dysbiosis, an imbalance in the gut microbiota, plays a crucial role in the onset and progression of IBD. This imbalance contributes to the development of IBD through various mechanisms, including changes in the production and release of MVs. An important characteristic of IBD is a shift in the composition of the gut microbiota, typified by a decrease in beneficial bacteria and an increase in harmful bacteria.Citation30,Citation31,Citation129 This dysbiotic state of the gut leads to alterations in the production and release of MVs, which results in the inhibition of colonization by probiotics and an increase in the growth and colonization of gut pathogens, culminating in inflammatory processes, a marked symptom of IBD.
3.2. MVs in the pathogenesis of IBD
As summarized in , several lines of evidence suggest that MVs play a crucial role in the development and progression of IBD, a chronic inflammatory condition affecting the gastrointestinal tract.
Table 2. Bacterial MVs in the pathogenesis of IBD.
3.2.1. MV-induced disruption of intestinal epithelial barrier integrity
MVs from certain pathogenic bacteria in the GIT can lead to intestinal barrier dysfunction, a major symptom of IBD. MVs can disrupt the integrity of the intestinal epithelial barrier, allowing bacteria and their products to translocate into the lamina propria, the layer of connective tissue beneath the epithelium. This translocation further stimulates the immune system and contributes to chronic inflammation. Internalization of MVs from E. coli BL21 by intestinal epithelial cells occasions a cascade that involves sorting nexin 10 (SNX10) and LPS release from the MVs into the cytosol. The presence of cytosolic LPS leads to further downstream processing that culminates in intestinal barrier dysfunction, promoting inflammation in the gut.Citation71 Fn-MVs significantly reduced the levels of tight junction proteins ZO-1, claudin-1, and occludin, as well as MUC-1 and −2, dysregulating the epithelial barrier integrity in colitis mice.Citation28 Another study reported that Fn-derived MVs downregulated tight junction proteins ZO-1 and occludin, resulting in epithelial barrier dysfunction both in vitro and in vivo. The exacerbation of colitis by the MVs was linked with Fn-MVs facilitated downregulation of miR-574-5p expression and activation of autophagyCitation134 ().
Figure 6. Bacterial membrane vesicles in the pathogenesis of IBD.
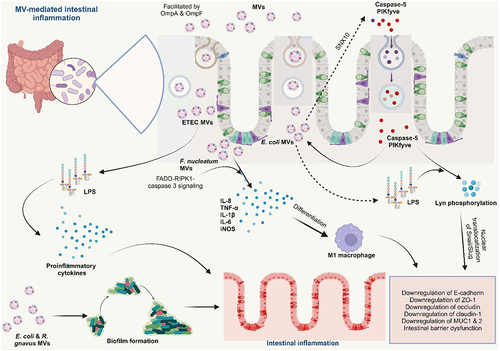
3.2.2. MV-induced modulation of host immune responses and delivery of virulence factors
MVs can influence host immune responses in IBD by modulating the expression of immune-related genes and/or by directly interacting with immune cells. These interactions can lead to an imbalance in immune responses, contributing to the chronic inflammatory characteristic of IBD. MVs from IBD-associated bacteria contain pro-inflammatory molecules, such as LPS and flagellin, which can activate TLRs on host cells. TLR activation triggers inflammatory signaling pathways that lead to the production of pro-inflammatory cytokines, contributing to the inflammatory state of IBD.
Interaction of LPS from bacterial-associated MVs with PBMCs resulted in the strong production of proinflammatory cytokines IL-6, IL-8, MCP-1, and MIP-1α.Citation136 MVs from Fn promoted the secretion of proinflammatory cytokines IL-8 and TNF-α in vitro in colonic epithelial cells.Citation27 Fn-MVs triggered an upregulation of the proinflammatory cytokines IL-1β, IL-6, TNF-α, and iNOSCitation28,Citation134 and downregulation of anti-inflammatory IL-10 in vitro in intestinal epithelial cells and in vivo in colitis mice.Citation134 Increased levels of F4/80+ iNOS+M1-like macrophages were also reported; thus, Fn-MVs enhanced apoptosis of intestinal epithelial cells in vivo by inducing the pro-inflammatory M1 phenotype resulting in intestinal barrier dysfunction in UC (). The FADD-RIPK1-caspase 3 signaling mediated this action of Fn-MVs and serves as a basis for further studies.Citation28 In their study, Tulkens and colleagues also demonstrated a significant increase in the bacterial MVs associated with LPS activity in patients with intestinal barrier dysfunction such as IBD.Citation136
A study by Durant and fellow researchers, found the possibility that the presence of IBD could most likely affect the responses of immune cells to otherwise beneficial MVs from commensal bacteria. In their study, they demonstrated that DCs are important APCs that can produce and respond to IL-10 to regulate immune responses and microbial tolerance. However, DC subsets are altered in IBD, and a decline in the numbers of CD103+ DCs in the colon of both UC and CD patients compared to healthy controls was reported, supporting a loss of regulatory DCs in IBD.Citation137 Compared to healthy controls, Bt-MVs were unable to induce the expression of IL-10 in colonic DCs of UC patients and elicited a significantly lower proportion of DCs that expressed IL-10 in the blood of both CD and UC patients.Citation137
The localization of Bt-associated antigens to host immune cells (macrophages) through the MVs of Bt with sulfatase activity was shown to be the primary cause of the fulminant colitis observed in genetically-susceptible dnKO mice treated with the bacterium. However, upon deletion of the anaerobic sulfatase maturating enzyme (anSME) from the bacterium, its ability to stimulate colitis in dnKO mice was remarkably abolished. This would mean that access of Bt-MVs to host immune cells was sulfatase-dependent and that the MVs of this bacterium and associated enzymes promote inflammatory immune stimulation in genetically susceptible hosts.Citation130 The colonic macrophages of dnKO mice gavaged with wild-type, WT-Bt revealed a significant upregulation in the levels of pro-inflammatory markers COX-2, TNF-α, and IL-1β as compared to mice treated with PBS and ΔanSME Bt.
Many pathogenic E. coli have been reported to significantly promote the progression of IBD. The association between adherent invasive E. coli (AIEC) and IBD progression has been reviewed by several studies,Citation138–140 AIEC strain LF82 recovered from a chronic lesion of a CD patient demonstrated great invasive ability in intestinal epithelial cells in vitro. The outer membrane proteins, OmpA and OmpC found in their MVs were identified as the virulence factors responsible for their invasiveness.Citation133 Moreover, some other harmful E. coli associated with IBD, enterotoxigenic E. coli (ETEC) and enterohemorrhagic E. coli (EHEC)Citation141 release MVs containing toxins (such as EHEC cytolysin ClyA and cytolethal distending toxin V, ETEC heat-labile enterotoxin (LT)) that can damage host cells, exacerbate inflammation, promote bacterial colonization, and consequently, disease progression.Citation104,Citation141 The strong induction of the proinflammatory cytokine IL-8 by ETEC MVs was also reported to occur via the internalization of their MVs and subsequent delivery of their LPS (contained in the MVs) to intestinal epithelial cells, which is then recognized by novel caspase- and RIPK2-dependent pathways.Citation131 Underacylated LPS-derived ETEC OMVs showed similar uptake dynamics but less proinflammatory potency than MVs derived from WT ETEC, suggesting that this identification is likely due to the detection of the lipid A moiety.
3.2.3. Promotion of bacterial colonization
MVs play significant roles in IBD development and progression by facilitating the colonization of harmful bacteria in the gut of IBD patients. They can achieve this by the various mechanisms described below.
Some MVs contain adherence factors that facilitate the entry of harmful bacteria into host cells, increasing their chances of colonizing the gut. The MVs of Francisella tularensis were reported to be involved in the entry of the bacteria into macrophages.Citation135 Furthermore, dysbiosis enables the overgrowth of harmful bacteria in the gut, resulting in the increased secretion of their MVs in the gut lumen. Studies have also shown that IBD patients exhibited elevated levels of MVs in their feces compared to healthy individuals,Citation142 which has been attributed to the dysbiotic gut microbiota associated with IBD. As reported above, MVs from pathogenic E. coli release toxins that damage the host cells facilitating their colonization in host cells.Citation104,Citation141 Again, the disruption of the integrity of the intestinal epithelial barrier by MVs allows the influx of harmful bacteria into the lamina propria, the layer of connective tissue beneath the epithelium. This translocation provides harmful bacteria access to nutrients and a protected environment for colonization.Citation28,Citation143
Another mechanism is via biofilm formation – a community of harmful bacteria embedded in a matrix of extracellular polymeric substances (EPS). Biofilms provide a protective environment for bacteria, making them more difficult for the immune system to eliminate. Additionally, biofilms release toxins and other inflammatory mediators that can contribute to the chronic inflammatory characteristic of IBD.Citation144 MVs have the capacity to protect biofilms from host immune attack and antimicrobial agents, thereby promoting their persistence in the gutCitation145 (). Many pathogenic MVs-producing microorganisms such as enterotoxigenic Bacteroides fragilis, E. coli, Ruminococcus gnavus among others, which are known to be increased in the dysbiotic gut of IBD patients, have been found to form biofilms in the ileum and right-sided colon of the gut.Citation146,Citation147 Additionally, as MVs interact with host cells and modulate their signaling pathways, they can suppress immune responses, ultimately creating a more favorable environment for colonization by harmful bacteria.Citation28,Citation134
4. Bacterial MVs as diagnostic biomarkers of IBD
MVs can be analyzed for their content of specific molecules or signatures that could serve as biomarkers for IBD diagnosis and disease monitoring. Metagenomic profiling of patients with CD (active and remission) showed that the microbial community structure of stool-derived MVs was significantly different from the stool-derived microbiome in relation to healthy controls. Consequently, 16S rRNA sequencing of fecal-derived MVs was reported as more suitable as a diagnostic biomarker for IBD than just 16S rRNA sequencing of the bacterial population in the feces of IBD patients.Citation148 Another metagenomic profiling study corroborated the above. Heo et al.. (2023) revealed that the analysis of gut microbe-derived MVs was more effective than stool microbiome analysis at differentiating patients with IBD from healthy controls.Citation149 Yet, Kang reported that even though colitis induction resulted in a change in the gut-bacterial composition, a more drastic change was observed in the composition of bacterial-derived fecal MVs. Metagenomics of MVs composition in stool samples of dextran sulfate sodium (DSS)-induced colitis in mice revealed a decrease in the MVs of Akk and Bacteroides acidifaciens and an increase in MVs from TM7 phylum, particularly in DQ777900_s and AJ400239_s species.Citation150
While several miRNAs are associated with disease origin and development, some have been found to be pathology-specific.Citation151 Accumulating evidence reveals that significant levels of miR-21, miR-155, and miR-223 presented by IBD patients could be potential biomarkers for IBD.Citation152 As a result, changes in miRNA expression profiles have been addressed for applications in the classification of early detection, prognosis, and diagnosis of IBD.Citation152 The 2015 study by Polytarchou et al. revealed the association of miR-214 with the progression of IBD and how reducing its expression slowed the development of colitis and colitis-associated cancer in mice.Citation153 Interestingly, recent studies have also shown that MVs affect the expression of miRNAs in IBD. The downregulation or upregulation of certain miRNAs in the presence of MVs tends to monitor not only the progression of IBD but also the alleviation of inflammation. For instance, the downregulation of miR-574-5p expression by Fn-MVsCitation134 and restoration of miR-199a-3p expression by Cb-MVsCitation154 demonstrate that miRNAs are intricately associated with MV exposure in IBD cases. With this knowledge, some specific miRNAs could serve as both diagnostic and potential targets for IBD treatment.
5. Potential therapeutic applications of MVs in IBD
Several studies have shown strong evidence for the possible application of MVs for therapeutic purposes in IBD. The findings from these studies are discussed below and have also been summarized in .
Table 3. Application of MVs in IBD therapy.
5.1. Modulation of intestinal epithelial barrier integrity
The integrity of the intestinal epithelial layer protects against invading pathogens and toxins. The formation of tight junctions (TJs) between adjacent IECs is very crucial in the maintenance of epithelial barrier function. Disruption of this epithelial barrier enhances intestinal permeability, a key predisposing factor to allergy, inflammation, and other metabolic diseases.Citation165 The gut bacteria have been shown to strengthen the epithelial layer, and interactions of the immune systemCitation166 and MVs from the gut microbiota are crucial in the modulation of epithelial barrier integrity. Administration of MVs derived from EcN to DSS-treated mice significantly improved epithelial barrier function in these mice (Fábrega et al., 2017). MVs from Lactobacillus kefirgranum PRCC-1301 (PRCC-1301-MVs) significantly inhibited the loss of tight junction proteins occludin, claudin-1, and ZO-1 thereby limiting epithelial permeability in the colon tissues of DSS-colitis mice.Citation156 MVs from Clostridium butyricum, Cb-MVs significantly upregulated the secretion of colonic mucus (MUC-2) and tight junction proteins (ZO-1) compared to DSS-colitis mice.Citation157 A report of Cb from another study indicated that their MVs significantly enhanced the secretion of higher amounts of mucins (MUC-1, −2, −3, and −4) as well as tight junction proteins claudin-1, 3, and ZO-1, improving DSS-damaged epithelial barrier.Citation124 MVs from fecal fermentation exposed to miR-200b-3p restored intestinal barrier function via upregulation of tight junction molecules, claudin-3 and colonic MUC-1, and MUC-4 in DSS-colitis mice.Citation167 Regulation of microbial tryptophan metabolites by Cb-MVs enhanced intestinal barrier integrity and reduced inflammatory activities in colitis mice.Citation154 MVs derived from Akkermansia municiphila, Akk maintain the integrity of the intestinal barrier by the stimulation of the expressions of tight junction molecules, ZO-1 and occludin, as well as mucus in the intestinal lumen of the colon by entering the intestinal epithelial cells.Citation29 MVs from F. prausnitzii, Fp significantly upregulated tight junction molecules ZO-1 and occludin in DSS-treated mice, significantly improving the epithelial barrier integrity.Citation125
These studies entail that MVs from probiotics have the capacity to repair the integrity of the intestinal epithelial barrier, subsequently eliminating the influx of bacteria and other agents into the lamina propria, thereby reducing inflammation and engendering IBD treatment ().
Figure 7. Bacterial membrane vesicles (MVs) repair the intestinal epithelial integrity and restore gut microbiota homeostasis.
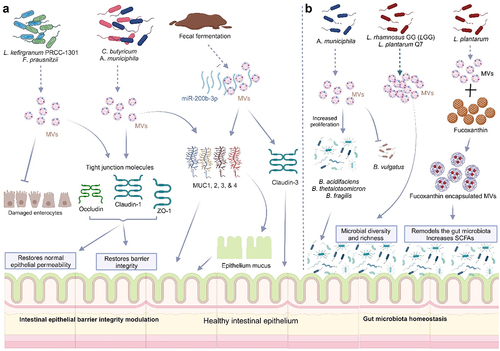
5.2. Restoration of gut microbiota homeostasis
Several studies have revealed that commensal and probiotic-derived MVs play fundamental roles in maintaining the stability of the intestinal microbiota. Not only do these MVs support the growth and colonization of beneficial microorganisms, but they also inhibit the growth and colonization of opportunistic and pathogenic microorganisms. The cargo delivered by MVs to the intestinal microflora, including enzymes, functional genes, and essential nutrients, enable them to thrive in the constantly changing microenvironment of the intestine.Citation128 Although the intestines are host to a great diversity of bacteria, not all these bacteria have the capacity to produce MVs.
A recent study revealed that Akk-derived MVs restored the balance of the gut microbiota through membrane fusion by selectively promoting the proliferation of beneficial bacteria B. acidifaciens, B. thetaiotaomicron, and B. fragilis, by fusion but did not fuse with B. vulgatus, thus, had no growth benefit for it. This reveals the ineffectiveness of Akk-MVs in promoting the proliferation of potentially opportunistic Bacteroides species in DSS-induced gut disorder.Citation29 MVs from L. rhamnosus GG (LGG)Citation31 and L. plantarum Q7Citation30 also ameliorated DSS-induced colitis and enhanced gut-microbiota balance by promoting the microbial diversity present. Oral gavage of LGG and Q7 MVs increased bacterial α-diversity and restored the taxonomic imbalance of gut microbiota induced by DSS. An increase in the number of Bifidobacteria and Muribaculaceae with a reduction in the Proteobacteria population was observed with oral administration of Q7-MVs, while Helicobacter, Odoribacter, Desulfovibrio were increased in DSS-treated mice, Odoribacter, Alistipes, Muribaculaceae, Lachnospiraceae_NK4A136_group, and Akkermansia were enriched in LGG-MVs treated mice. A greater abundance of Odoribacter was, however present in the LGG-MVs treated group compared to DSS-treated mice.Citation31 Cb-MVs re-modeled the gut microbiota composition thereby improving DSS-induced colitis in mice.Citation157 The relative abundances of Lactobacillus, Bacteroidales_S24-7_group, Akkermansia and Bacteroides, were significantly downregulated in response to DSS treatment, while MV treatment reversed these decreases.Citation157 Cb-MVs also attenuated colitis in mice and modulated the gut microbiota by significantly reducing levels of pathogenic bacteria, including Escherichia/Shigella, and promoting a relative abundance of butyrate-producing Clostridium sensu stricto-1 and Butyricicoccus.Citation124,Citation154 Another study reported that MVs from normal feces of mice effectively reversed the composition of the intestinal microbiota, restored the intestinal barrier, and rescued colitis. Remarkably, MVs from fecal samples of colitis mice had similar effects after treatment with miR-200b-3pp.Citation167 Akk-MVs treated group exhibited marked improvements in both richness and diversity of the gut microbiota compared to DSS-PBS-treated mice by promoting an increase in the relative abundances of several probiotic or commensal bacterial genera, including Bacteroides, Lactobacillus, and Alistipes, together with Lachnospiraceae_NK4A136_group and bacterium f Lachnospiraceae. The beneficial members of the Bacteroides genera in the MV-treated group were significantly upregulated. Additionally, Akk-MVs reduced the relative abundances of bacteria belonging to the phylum Proteobacteria, the largest phylum comprised of many pathogenic bacteria and regarded as a microbial signature of dysbiosis in the gut microbiotaCitation29 ().
5.3. Immune system modulation
MVs from commensals and probiotic bacteria could elicit mucosal immunomodulatory responses by modulating the expression of immune-related genes and/or by directly interacting with immune cells in order to restore the immunological profile, alleviating colitis.
MVs derived from L. paracasei, reduced the activation of inflammation-associated proteins such as COX-2, iNOS, and NF-κB, as well as nitric oxide in vitro. Oral administration of these MVs in vivo offered protection against DSS-induced colitis. Upregulation of endoplasmic reticulum (ER) stress-associated proteins by these MVs was reported to be responsible for the anti-inflammatory effects observed.Citation159 Cb-MVs restored the expression miR-199a-3p, which targets map3k4, thereby suppressing proinflammatory mitogen-activated protein kinase (MAPK) and NF-κB signaling pathways, ultimately contributing to Cb-MVs mediated anti-inflammatory effect.Citation154 Pretreatment with Akk-derived MVs in vitro mitigated the production of the proinflammatory cytokine IL-6 from colonic epithelial cells upon stimulation by pathogenic E. coli MVs.Citation150 Administration of MVs derived from EcN to DSS-treated mice significantly reduced levels of proinflammatory cytokines IL-1β, TNF-α, and IL-17 in DSS-treated mice.Citation158 Treatment of the macrophage cell line RAW 264.7 with EcN-MVs improved the immune-related enzymatic and phagocytic activities of macrophages. Acid phosphatase which is associated with phagocytosis and clearance of exogenous substances by macrophages, was significantly improved upon stimulation with EcN-MVs.Citation168 Capsular Polysaccharide A (PSA) which is contained in B. fragilis-derived MVs has an immunomodulatory function and can prevent experimental colitis. Treatment of DCs with PSA-containing MVs prevented trinitrobenzene sulfonic acid (TNBS)-induced colitis in mice via suppression of the proinflammatory cytokines, TNF-α and IL-17, and increased secretion of IL-10. These MVs also enhanced the anti-inflammatory capacity of regulatory T-cells (CD4+CD25+Foxp3+Tregs) and stimulated increased production of IL-10 from them. This study reported that the DCs’ action depends on Growth Arrest and DNA-Damage Inducible protein (Gadd45α) and that DCs recognize MV-associated PSA via TLR-2.Citation161
There was a significant reduction in NF-κB activation and IL-8 expression in LPS-treated HT-29 human IECs upon pretreatment with Propionibacterium freudenreichii-derived MVs indicating their potent anti-inflammatory property, which partly depended on the activity of immunomodulatory proteins such as SlpB.Citation162 MVs derived from L. paracasei inhibited LPS-induced proinflammatory cytokines and increased the expression of anti-inflammatory cytokines in HT-29 cells.Citation159 PRCC-1301-MVs showed effective reduction in the levels of proinflammatory cytokines IL-2, IL-8, and TNF-α in DSS-treated Caco-2 cells as well as inhibition of the NF-κB signaling pathway in mice models of colitis.Citation156 Kuhn and colleagues demonstrated that MVs from L. casei and L. plantarum strongly increased IL-10 anti-inflammatory cytokine. Another report also showed a significant reduction of TNF-α and increased IL-10 levels in macrophage inflammation models in vitro upon treatment with MVs from L. plantarum and L. casei.Citation169 Pediococcus pentosaceus-derived MVs reportedly suppressed antigen-specific humoral and cellular responses and promoted M2-like macrophage polarization and myeloid-derived suppressor cell differentiation in bone marrow-derived macrophages and bone marrow progenitors, respectively, presumably in a TLR-2-dependent manner. Consistent with their immunomodulatory activity, MV-differentiated cells upregulated expressions of IL-10, arginase-1, and PD-L1 and suppressed the proliferation of activated T cells.Citation163 Cb-derived MVs polarized macrophages to M2 phenotypeCitation157 and significantly reduced the levels of plasma LPS, IL-6, and TNF-α,Citation124 ameliorating DSS-induced colitis in mice. MVs from fecal fermentation exposed to miR-200b-3p reduced levels of inflammatory markers IL-6, and TNF-α and increased the levels of IL-10 in DSS-induced colitis.Citation167 Bt-MVs demonstrated upregulation of IL-10 production in colonic tissue and in splenocytes, ameliorating colitis in mice. Further interactions of Bt-MVs with the monocytic cell line THP-1 were shown to be mediated primarily by TLR-2.Citation164
In their studies, Hao et. al. (2021) and Tong et. al. (2021) demonstrated that the increased genera in dysbiotic-colitis mice positively correlated with inflammatory cytokines. However, treatment with MVs from Lp-Q7 and LGG promoted the growth of anti-inflammatory bacteria genera, strongly alleviating colitis. They also reported that the expression of the pro-inflammatory cytokines TNF-α, IL-1β, IL-6, and IL-2 in these mice models of DSS-induced colitis were significantly downregulated by oral administration of the MVs.Citation30,Citation31 Akk-MVs elicited mucosal immunoglobulin A response by translocating into Peyer’s patches and then activating DCs and B cells, thereby enhancing the intestinal immune barrier function in order to prevent invasion by pathogens.Citation29 Fp-MVs increased the ratio of T-reg cells in the colon tissue of colitis mice, downregulated the expression of the proinflammatory cytokines IL-1β, IL-2, IL-6, IL-12a, IFN-γ, TNF-α, and granulocyte-macrophage colony-stimulating factor (GM-CSF), and upregulated the anti-inflammatory cytokines IL-4, IL-10, and TGF-β in DSS-treated miceCitation125 ().
Figure 8. Bacterial membrane vesicles modulate the immune system under IBD conditions to enhance intestinal immune barrier function.
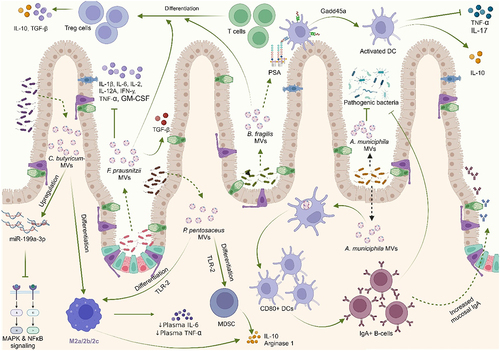
5.4. Inhibition of MV release and interaction with their targets
Some studies have shown that certain agents could either prevent (block) the release of pathogenic MVs or inhibit their interaction with target cells/genes, mitigating inflammation in IBD patients. Wang and colleagues reported that deletion of sorting nexin 10 (SNX10) or treatment with its inhibitor DC-SX029 restored MV-induced intestinal barrier dysfunction and alleviated colitis in mice by blocking cytosolic MV-LPS release and further downstream signaling.Citation71 The blockade of autophagy using chloroquine and inhibition of miR-574-5p/CARD3 axis ameliorated epithelial barrier dysfunction, autophagy activation, and subsequently, colitis severity mediated by Fn-MVs in vitro and in vivo.Citation134 Hickey et al. reported that deletion of the anaerobic sulfatase maturating enzyme (anSME) from the wild-type Bt remarkably eliminated the ability of the MVs to stimulate colitis in dnKO mice. This revealed that access of Bt-MVs to host immune cells was sulfatase-dependent. The MVs of the bacteria and associated enzymes promote inflammatory immune stimulation in genetically susceptible hosts.Citation130 The deletion of the yfgL gene in AIEC strain LF82 led to the release of fewer MVs by the bacteria and a gross reduction in their capacity to strongly invade intestinal epithelial cells.Citation133
5.5. Genetically engineered MVs for targeted drug delivery
Due to MVs’ ability to penetrate physiological barriers that many synthetic delivery carriers cannot penetrate, they can finely serve as carriers of active components, such as anti-inflammatory drugs, or therapeutic nucleic acids.Citation160 Moreso, the lipid bilayer of MVs offers stability and protection to the cargo, especially in the harsh environment of the GIT, leading to increased bioavailability of both the MVs and their encapsulated therapeutic agent. In addition, MVs can be engineered to display specific ligands on their surface, allowing for targeted drug delivery to sites of inflammation in the gut, thereby reducing off-target effects and improving efficacy. Unfortunately, their low secretion limits their widespread use, coupled with the lower yield of MVs loaded with active components.Citation160
Liang and colleagues, however, successfully engineered MVs from the probiotic, L. plantarum on a large scale and even incorporated fucoxanthin (a dietary intervention for colitis) in these MVs. These FX-MVs gave a 150-fold yield and greater protein content compared with the naturally secreted MVs of the probiotic. Additionally, FX-MVs promoted the gastrointestinal stability of fucoxanthin and inhibited H2O2-induced oxidative damage by scavenging free radicals effectively, greatly ameliorating colitis.Citation160 FX-MVs offered significant protection to colitis-mice, mitigating colonic inflammatory response. Interestingly, one mechanism by which FX-MVs attenuated colonic inflammatory response was by re-modeling the gut microbiota communities with a subsequent increase in the abundance of short-chain fatty acids in the colon ().Citation160 FX-MVs also promoted polarization of macrophages to M2 type and effectively suppressed levels of proinflammatory cytokines, improving colonic inflammation.Citation160 Probiomimetics obtained from individually coupling MVs from L. casei and L. plantarum onto microparticles alleviated inflammation-induced loss of intestinal barrier function. They were reported to improve transepithelial electric resistance (an in vitro measure of barrier integrity function) caused by LPS-induced inflammation in Caco-2 monolayers, whereas native MVs could not.Citation155 Probiomimetics also greatly ameliorated LPS-induced TNF-α secretion in colonic epithelial cells in vitro compared to native MVs. Reduction in IL-8 cytokine and significant stimulation of IL-10 secretion in these inflammatory environments were also observed, although L. plantarum MVs and L. plantarum MV-coated microparticles showed a higher anti-inflammatory effect than L. casei MVs and L. casei MV-coated microparticles.Citation155
Nanoprobiotics, prepared from EcN-1917 probiotic derived-MVs encapsulating manganese dioxide nanozymes demonstrated increased therapeutic ability of these MVs. These nanoprobiotics showed effective adherence to inflamed colonic epithelium and eliminated excess reactive oxygen species in the intestinal lumen of the murine IBD model. It is fascinating to note that these nanoprobiotics, in combination with the anti-inflammatory medicine, metformin, improved the overall richness and diversity of the gut microbiota, remodeled the pro-inflammatory state of the microenvironment, and displayed better therapeutic efficacy than commercially available IBD chemotherapeutics.Citation170
MVs derived from Bt were engineered to express and stably deliver keratinocyte growth factor-2 (KGF-2), a human-derived therapeutic protein into the GIT of mice for protection against tissue inflammation and injury. These engineered Bt-MVs reduced disease severity and promoted epithelial repair and recovery in the DSS-induced colitis in mice.Citation97
These studies illustrate that despite the challenges associated with MV secretion, their excellent plasticity allows for further manipulations for greater efficacy in IBD therapy.
5.6. MVs in IBD vaccines
Recent advances in immunological research present therapeutic vaccinations as an alternative in the treatment of many diseases. By stimulating the production of particular antibodies by the immune system, these vaccines may provide a means of treating IBD. Therapeutic vaccinations are a better option for managing IBD patients because of their safety and efficacy, as well as their ability to lessen the financial and healthcare burden associated with illness management. Studies on gut microbiota vaccines have upscaled in recent times. This entails using vaccines to induce the production of antibodies in the gut that target and act more specifically on the relevant pathogenic microorganisms – for the treatment of IBD.Citation171 For instance, the involvement of different E. coli strains in the pathogenesis of IBD reveals that anti-E. coli vaccines could significantly mitigate intestinal inflammation. Daley and team found that a genetically attenuated ETEC vaccine, which was proven to be safe, improves E. coli flora dysbiosis by inducing a significant mucosal IgA response in the gut.Citation172 Tran and colleagues also found that colitis-related characteristics such as inflammation, damage to epithelial cells, and constricted gut passageways improved in mice immunized with flagellin. This shows that chronic inflammatory illnesses could be treated with naturally occurring antibodies against flagellin or other pathogenic bacteria associated with IBD. Thus, stimulating the production of these particular antibodies with flagellin vaccination may be a useful strategy for treating intestinal inflammation in IBD patients. Furthermore, vaccines for IBD that target cytokinesCitation171 could also be formulated with an appropriate immune-stimulating bioparticle.
Although the intricate pathophysiology of IBD makes vaccination against a single pathogen insufficient to protect against the disease, MVs have several characteristics that make developing vaccines from them appealing. These include their capacity to display proteins from many sources, their inherent possession of pathogen-associated molecular patterns (PAMPs) that trigger potent immune responses, their nanoscale size for effective antigen processing and delivery, and their adaptability to be further altered, such as the combination of MVs with other nanomaterials that can help to improve vaccination efficacy by integrating the advantages of each individual component.Citation173 MVs have been formulated into vaccines against viruses including human immunodeficiency virus, coronaviruses, human papilloma virus, hepatitis viruses, and influenza, and a wide variety of bacteria such as Neisseria meningitidis, Neisseria gonorrhoeae, Acinetobacter baumannii, Streptococcus pneumoniae, S. aureus, Bordetella pertussis, Burkholderia mallei, Burkholderia pseudomallei, Edwardsiella tarda, E. coli, Klebsiella pneumoniae, P. aeruginosa, and Salmonella enterica.Citation173 CPS14+MVs vaccine prepared from the MVs of a probiotic E. coli strongly provoked an IgG class-switch combination with a Th1/Th2-balanced IgG subclass distribution without any adjuvant. This vaccine was also structurally stable with heat treatment. Mice of various ages showed broad efficacy for the CPS14+MV vaccination, and the humoral immune responses provoked by the vaccine remained in both the lungs and blood for a period of one year. The study revealed that the probiotic E. coli MVs-based vaccine platform provides a viable, broadly applicable defense against encapsulated pathogens.Citation174 Although there are still debates on the possible involvement of Mycobacterium avium subspecies paratuberculosis (MAP) in the onset of CD, Aitken et al. successfully identified this organism in excised tissues of 18 IBD patients, with none detected in the 15 samples of non-IBD control.Citation175 A recent, in silico vaccine design from MVs derived from MAP, showed that the multi-epitope vaccine obtained by stitching antigenic, immunogenic, and IFN-γ-inducing B-cell, MHC-I, and MHC-II epitopes through linkers could be a promising vaccine candidate against MAP, although both in vitro and in vivo experiments are required for solid confirmation.Citation176
Although there are currently no direct studies on the development of MV-based IBD vaccines, these studies strongly show that the intrinsic characteristics of MVs and their ease of manipulation make their development and application in IBD vaccines feasible and imminent.
6. Challenges and future research directions
Despite the promising potential of MVs as therapeutic agents, several challenges need to be addressed before they can be widely used in clinical practice. These challenges include:
6.1. Development of efficient production process and administration of MVs in functional assays
Effective methods are needed to produce large quantities of MVs with consistent and desired characteristics. Many methodsCitation69 have been published for the extraction and purification of MVs, each presenting a range of advantages and disadvantages. For uniformity of MV studies across the globe and ease of comparative analysis, the best methods for the extraction and purification of MVs, which will also ensure they are produced in large quantities, need to be established.
Additionally, the study of Müller and colleagues revealed the significant effect of different culture conditions on the anti-inflammatory properties of vesicles derived from L. casei and L. plantarum.Citation169 This entails that manipulation of vesicle-producing bacteria’s growth conditions could significantly affect the biological functions of these vesicles. As such, besides bioengineering, MVs from probiotic and commensal bacteria with proven anti-inflammatory activity could be manipulated in other ways to yield an even more excellent and efficient anti-inflammatory activity for the treatment of inflammatory-related diseases.
Many studies in MVs research quantify MVs for administration in functional assays using protein content characteristics only. The different protein assays (BCA, Lowry, Bradford, and Qubit assays) employed by Bitto and colleagues in their study showed significant variation in both the quantification and sensitivity of MVs produced by different species. Normalizing MVs by protein content lessened the ability to separate strain differences in the immunological functions of MVs.Citation82 However, species-, strain-, and growth stage-dependent differences in MV cargo content were evident upon MV characterization by particle number. Performing immunological assays using an equivalent amount of MVs from P. aeruginosa, H. pylori, and S. aureus quantified based on their protein concentration masks the disparities in the amount of immunogenic cargo carried by MVs, and this impacts analyses of their immunostimulatory properties substantially. On the other hand, performing the same assays using an equivalent amount of MVs quantified by particle number revealed significant differences in their ability to be detected by PRRs, activate NF-κB, and induce an IL-8 proinflammatory response.Citation82 Consequently, a standardized method for MV quantification in which a variety of factors that affect MV function, such as the bacterial growth conditions, growth stage, MVs extraction method, sample purity, MV size, particle number, and cargo content, are also reported, is strongly recommended.Citation82 Biological comparisons of the functional differences between MVs across various bacterial genera, species, and strains will be made easier by this standardization. This will eventually produce consistency and comparability in the area of MV research.Citation82
6.2. Deeper insights into the mechanisms of MV interactions with host cells
A deeper understanding of the complex interactions between MVs and host cells (intestinal epithelial cells and immune cells) is crucial for optimizing MV-based therapies.
6.3. Safety and efficacy evaluation
MVs are produced from either pathogenic bacteria, probiotics, or host commensals, and consequently, rigorous validations in the laboratory are still necessary for adequate evaluation of the appropriate dosage, safety, and efficacy of these MV-based therapies in IBD patients. These uncertainties hinder the translation of MV-based therapies from the laboratory to clinical trials, and specific studies that address them are urgently needed. Further studies targeted at MVs from particular bacteria types that have shown remarkable ability in the attenuation of colitis can be carried out to accurately ascertain these concerns, as it will make for quick and easy translation for clinical trials.
6.4. Targeting and delivery of MVs to specific sites
Mechanisms must be explored and enhanced to ensure that MVs reach the specific sites of action in the gut and deliver their therapeutic cargo effectively. It is also important that the MVs are stably delivered at adequate therapeutic quantities to their target sites. This is a major challenge, as it has been reported that some probiotic-derived MVs may face rapid clearance and possible dilution effects in the GIT, which may impair their therapeutic efficacy.Citation155 The probiomimetics therapeutic system discussed earlier is quite advantageous and should be further explored since it limits the possibility of rapid clearance of the native MVs from the gut, leading to an increased concentration of MVs on the inflamed mucosal cells.Citation155 Although the nanoprobiotics team reported an insignificant overt systemic toxicity in the treatment, it was overcome by the integration of cytokine storm calm with the biotherapy, ultimately culminating in the development of a safe and effective bionanoplatform for the effective treatment of inflammation-mediated intestinal diseases.Citation170 Since the toxicity was successfully surmounted, it would be interesting to explore the potential inclusion of metformin in the engineered EcN MVs. In simpler terms, incorporating the anti-inflammatory drug metformin into the engineered EcN MVs could be a more potent alternative to administering each therapy on its own.
Therefore, future studies exploring these paths are encouraged in order to increase the bioavailability of MVs in the gut, thereby enhancing the therapeutic efficacy of these MVs.
6.5. The dual role of the commensal Bt-MVs in IBD
While some studies have reported that Bt-MVs can trigger the onset of colitis in a genetically susceptible host,Citation130 or may not be effective in inducing the expression of IL-10 in both colonic and blood DCs of IBD patients,Citation137 others have reported that the administration of Bt-MVs to DSS-colitis mice alleviated the symptoms of intestinal inflammation by upregulation of IL-10 production in colonic tissue and in splenocytes in mice.Citation164 Further studies on this commensal bacterium and its MVs are required to determine the pathways and conditions that stimulate these various activities.
6.6. MVs-miRnas interaction
Several studies have shown that miRNAs are significantly altered in colitis, and interaction of MVs with some of these miRNAs can either promote or reverse colitis in mice via different mechanisms. For instance, Cb-MVs restored miR-199a-3p expression,Citation154 treatment of MVs isolated from feces of colitis mice with miR-200b-3p rescued colitis in mice,Citation167 Fn-MVs facilitated downregulation of miR-574-5p expression exacerbating colitis.Citation134 These studies reveal that miRNAs could serve as potential targets for IBD diagnosis, progression, and therapy. Research focusing on determining the miRNAs implicated, mechanisms of action and interaction with MVs, and models for therapeutic applications are strongly advocated for.
6.7. MV-based IBD vaccines
The potential benefits of MV-based vaccines for IBD include effective immune response, targeted delivery, and improved safety. Future studies that focus on these benefits to develop vaccines from MVs against IBD are encouraged. Optimization of vaccine formulations, improving dosing regimens, and evaluating these vaccines’ long-term efficacy in preventing IBD flares and complications should be further considered.
7. Conclusion
Bacterial membrane vesicles have been described as major key players in the onset and progression of IBD, as well as in the treatment of the disease. Having adequate knowledge of the many factors that influence MV production and release is imperative for further studies in the area, particularly in the best approaches for manipulating MVs for the treatment of IBD. Many studies have reported that MVs from pathogenic bacteria induce strong pro-inflammatory responses that exacerbate inflammation, potentially resulting in IBD. Therapeutic agents that degrade these MVs in the gut lumen or block their release will be greatly needed to curtail these harmful effects. However, MVs from probiotics and some commensals have been shown to offer strong protection against the progression of IBD. It is therefore crucial that these MVs are further manipulated and effectively translated to different clinical trials of IBD treatment and management. Personalized therapy could even result from these since the makeup of the gut microbiota may show some slight uniqueness in each IBD patient. Lastly, there is also the possibility that MVs harbor specific molecules that could serve as biomarkers for IBD diagnosis and disease monitoring, enhancing their utility in IBD. The indispensable roles MVs play in IBD should be thoroughly considered, and a more profound insight into their mechanisms of action and interaction could become the next strategic area for notably reducing the epidemiology of IBD globally.
Disclosure statement
No potential conflict of interest was reported by the author(s).
Additional information
Funding
References
- Ng SC, Shi HY, Hamidi N, Underwood FE, Tang W, Benchimol EI, Panaccione R, Ghosh S, Wu JCY, Chan FKL, et al. Worldwide incidence and prevalence of inflammatory bowel disease in the 21st century: a systematic review of population-based studies. Lancet [Internet]. 2017;390(10114):2769–32. https://linkinghub.elsevier.com/retrieve/pii/S0140673617324480.
- Shi J, Xie Q, Yue Y, Chen Q, Zhao L, Evivie SE, Li B, Huo G. Gut microbiota modulation and anti-inflammatory properties of mixed lactobacilli in dextran sodium sulfate-induced colitis in mice. Food Funct [Internet]. 2021;12(11):5130–5143. http://xlink.rsc.org/?DOI=D1FO00317H.
- Shen Q, Huang Z, Yao J, Jin Y. Extracellular vesicles-mediated interaction within intestinal microenvironment in inflammatory bowel disease. J Adv Res [Internet]. 2022;37:221–233. https://linkinghub.elsevier.com/retrieve/pii/S2090123221001363.
- Shen Q, Xu B, Wang C, Xiao Y, Jin Y. Bacterial membrane vesicles in inflammatory bowel disease. Life Sci [Internet]. 2022;306:120803. https://linkinghub.elsevier.com/retrieve/pii/S0024320522005033.
- Dixon LJ, Kabi A, Nickerson KP, McDonald C. Combinatorial effects of diet and genetics on inflammatory bowel disease pathogenesis. Inflamm Bowel Dis [Internet]. 2015;21(4):912–922. https://academic.oup.com/ibdjournal/article/21/4/912-922/4579549.
- Mentella MC, Scaldaferri F, Pizzoferrato M, Gasbarrini A, GAD M. Nutrition, IBD and Gut Microbiota: A Review. Nutr [Internet]. 2020;12(4):944. https://www.mdpi.com/2072-6643/12/4/944.
- Yasmin F, Najeeb H, Shaikh S, Hasanain M, Naeem U, Moeed A, Koritala T, Hasan S, Surani S. Novel drug delivery systems for inflammatory bowel disease. World J Gastroenterol [Internet]. 2022;28(18):1922–1933. http://www.ncbi.nlm.nih.gov/pubmed/35664964.
- Mah C, Jayawardana T, Leong G, Koentgen S, Lemberg D, Connor SJ, Rokkas T, Grimm MC, Leach ST, Hold GL. Assessing the Relationship between the Gut Microbiota and Inflammatory Bowel Disease Therapeutics: A Systematic Review. Pathogens [Internet]. 2023;12(2):262. https://www.mdpi.com/2076-0817/12/2/262.
- Franzosa EA, Sirota-Madi A, Avila-Pacheco J, Fornelos N, Haiser HJ, Reinker S, Vatanen T, Hall AB, Mallick H, McIver LJ, et al. Gut microbiome structure and metabolic activity in inflammatory bowel disease. Nat Microbiol [Internet]. 2018; 4(2): 293–305. doi:10.1038/s41564-018-0306-4.
- Xu X, Ocansey DKW, Pei B, Zhang Y, Wang N, Wang Z, Mao F. Resveratrol alleviates DSS-induced IBD in mice by regulating the intestinal microbiota-macrophage-arginine metabolism axis. Eur J Med Res [Internet]. 2023;28(1):319. doi:10.1186/s40001-023-01257-6.
- Xu X, Ocansey DKW, Hang S, Wang B, Amoah S, Yi C, Zhang X, Liu L, Mao F. The gut metagenomics and metabolomics signature in patients with inflammatory bowel disease. Gut Pathog [Internet]. 2022;14(1):26. doi:10.1186/s13099-022-00499-9.
- Richard ML, Sokol H. The gut mycobiota: insights into analysis, environmental interactions and role in gastrointestinal diseases. Nat Rev Gastroenterol Hepatol [Internet]. 2019; https://www.nature.com/articles/s41575-019-0121-2.
- Stecher B, Conway T, Cohen P. The roles of inflammation, nutrient availability and the commensal microbiota in enteric pathogen infection. Microbiol Spectr [Internet]. 2015;3(3). doi:10.1128/microbiolspec.MBP-0008-2014.
- Olovo CV, Huang X, Zheng X, Xu M. Faecal microbial biomarkers in early diagnosis of colorectal cancer. J Cell Mol Med [Internet]. 2021;25(23):10783–10797. doi:10.1111/jcmm.17010.
- Cho I, Blaser MJ. The human microbiome: at the interface of health and disease. Nat Rev Genet [Internet]. 2012;13(4):260–270. doi:10.1038/nrg3182.
- Wiredu Ocansey DK, Hang S, Yuan X, Qian H, Zhou M, Valerie Olovo C, Zhang X, Mao F. The diagnostic and prognostic potential of gut bacteria in inflammatory bowel disease. Gut Microbes [Internet]. 2023;15(1). doi:10.1080/19490976.2023.2176118.
- Díaz‐Garrido N, Badia J, Baldomà L. Microbiota‐derived extracellular vesicles in interkingdom communication in the gut. J Extracell Vesicles [Internet]. 2021;10(13). doi:10.1002/jev2.12161.
- Kim OY, Park HT, Dinh NTH, Choi SJ, Lee J, Kim JH, Lee S-W, Gho YS. Bacterial outer membrane vesicles suppress tumor by interferon-γ-mediated antitumor response. Nat Commun [Internet]. 2017;8(1):626. doi:10.1038/s41467-017-00729-8.
- Brown L, Wolf JM, Prados-Rosales R, Casadevall A. Through the wall: extracellular vesicles in Gram-positive bacteria, mycobacteria and fungi. Nat Rev Microbiol [Internet]. 2015;13(10):620–630. doi:10.1038/nrmicro3480.
- Schwechheimer C, Kuehn MJ. Outer-membrane vesicles from Gram-negative bacteria: biogenesis and functions. Nat Rev Microbiol [Internet]. 2015;13(10):605–619. doi: 10.1038/nrmicro3525.
- Kulp A, Kuehn MJ. NIH Public Access. Biological functions and biogenesis of secreted bacterial outer membrane vesicles. Annual Review of Microbiology. 2010;64:163–184. doi:10.1146/annurev.micro.091208.073413.
- Toyofuku M, Nomura N, Eberl L. Types and origins of bacterial membrane vesicles. Nat Rev Microbiol [Internet]. 2019;17(1):13–24. doi:10.1038/s41579-018-0112-2.
- Knox KW, Vesk M, Work E. Relation between excreted lipopolysaccharide complexes and surface structures of a lysine-limited culture of escherichia coli. J Bacteriol [Internet]. 1966;92(4):1206–1217. doi:10.1128/jb.92.4.1206-1217.1966.
- DeVoe IW, Gilchrist JE. Pili on meningococci from primary cultures of nasopharyngeal carriers and cerebrospinal fluid of patients with acute disease. J Exp Med [Internet]. 1975;141(2):297–305. doi:10.1084/jem.141.2.297.
- Zavan L, Bitto NJ, Kaparakis-Liaskos M. Introduction, history, and discovery of bacterial membrane vesicles [Internet]. In: Kaparakis-Liaskos, M, Kufer, TA, editors. Bacterial membrane vesicles. Cham: Springer International Publishing; 2020. pp. 1–21. doi:10.1007/978-3-030-36331-4_1.
- Liu Y, Defourny KAY, Smid EJ, Abee T. Gram-positive bacterial extracellular vesicles and their impact on health and disease. Front Microbiol [Internet]. 2018;9:385261. doi:10.3389/fmicb.2018.01502/full.
- Engevik MA, Danhof HA, Ruan W, Engevik AC, Chang-Graham AL, Engevik KA, Shi Z, Zhao Y, Brand CK, Krystofiak ES, et al. Fusobacterium nucleatum secretes outer membrane vesicles and promotes intestinal inflammation. MBio [Internet]. 2021;12(2):10–128. doi:10.1128/mBio.02706-20.
- Liu L, Liang L, Yang C, Zhou Y, Chen Y. Extracellular vesicles of Fusobacterium nucleatum compromise intestinal barrier through targeting RIPK1-mediated cell death pathway. Gut Microbes [Internet]. 2021;13(1). doi:10.1080/19490976.2021.1902718.
- Wang X, Lin S, Wang L, Cao Z, Zhang M, Zhang Y, Liu R, Liu J. Versatility of bacterial outer membrane vesicles in regulating intestinal homeostasis. Sci Adv [Internet]. 2023;9(11). doi:10.1126/sciadv.ade5079.
- Hao H, Zhang X, Tong L, Liu Q, Liang X, Bu Y, Gong P, Liu T, Zhang L, Xia Y, et al. Effect of extracellular vesicles derived from lactobacillus plantarum Q7 on gut microbiota and ulcerative colitis in mice. Front Immunol [Internet]. 2021;12. doi:10.3389/fimmu.2021.777147/full.
- Tong L, Zhang X, Hao H, Liu Q, Zhou Z, Liang X, Liu T, Gong P, Zhang L, Zhai Z, et al. Lactobacillus rhamnosus GG derived extracellular vesicles modulate gut microbiota and attenuate inflammatory in DSS-induced colitis mice. Nutr [Internet]. 2021;13(10):3319. doi:10.3390/nu13103319.
- Derkus B, Emregul KC, Emregul E. A new approach in stem cell research—exosomes: their mechanism of action via cellular pathways. Cell Biol Int [Internet]. 2017;41(5):466–475. doi:10.1002/cbin.10742.
- Cao Y, Lin H. Characterization and function of membrane vesicles in Gram-positive bacteria. Appl Microbiol Biotechnol [Internet]. 2021;105(5):1795–1801. doi:10.1007/s00253-021-11140-1.
- Gill S, Catchpole R, Forterre P. Extracellular membrane vesicles in the three domains of life and beyond. FEMS Microbiol Rev [Internet]. 2019;43(3):273–303. https://academic.oup.com/femsre/article/43/3/273/5195520.
- Bose S, Aggarwal S, Singh DV, Acharya N. Extracellular vesicles: An emerging platform in gram-positive bacteria. Microb Cell [Internet]. 2020;7(12):312–322. doi:10.15698/mic2020.12.737.
- Tian C, Yang M-F, Xu H, Zhu M, Zhang Y, Yao J, Wang L-S, Liang Y, Li D. Emerging role of bacterial outer membrane vesicle in gastrointestinal tract. Gut Pathog [Internet]. 2023;15. https://www.semanticscholar.org/paper/cc3ede4de27020c6747534d2ad175125593556a8.
- Schwechheimer C, Sullivan CJ, Kuehn MJ. Envelope control of outer membrane vesicle production in gram-negative bacteria. Biochemistry [Internet]. 2013;52(18):3031–3040. doi:10.1021/bi400164t.
- Pathirana RD, Kaparakis-Liaskos M. Bacterial membrane vesicles: Biogenesis, immune regulation and pathogenesis. Cell Microbiol [Internet]. 2016;18(11):1518–1524. doi:10.1111/cmi.12658.
- Gan Y, Zhao G, Wang Z, Zhang X, Wu MX, Lu M. Bacterial membrane vesicles: physiological roles, infection immunology, and applications. Adv Sci [Internet]. 2023;10(25). doi:10.1002/advs.202301357.
- Deatherage BL, Lara JC, Bergsbaken T, Rassoulian Barrett SL, Lara S, Cookson BT. Biogenesis of bacterial membrane vesicles. Mol Microbiol [Internet]. 2009;72(6):1395–1407. http://www.ncbi.nlm.nih.gov/pubmed/19432795.
- Song T, Mika F, Lindmark B, Liu Z, Schild S, Bishop A, Zhu J, Camilli A, Johansson J, Vogel J, et al. A new Vibrio cholerae sRNA modulates colonization and affects release of outer membrane vesicles. Mol Microbiol [Internet]. 2008;70(1):100–111. http://www.ncbi.nlm.nih.gov/pubmed/18681937.
- Schwechheimer C, Rodriguez DL, Kuehn MJ. NlpI-mediated modulation of outer membrane vesicle production through peptidoglycan dynamics in Escherichia coli. Microbiologyopen [Internet]. 2015;4(3):375–389. doi:10.1002/mbo3.244.
- Ohara M, Wu HC, Sankaran K, Rick PD. Identification and characterization of a new lipoprotein, NlpI, in Escherichia coli K-12. J Bacteriol [Internet]. 1999;181(14):4318–4325. doi:10.1128/JB.181.14.4318-4325.1999.
- Tashiro Y, Sakai R, Toyofuku M, Sawada I, Nakajima-Kambe T, Uchiyama H, Nomura N. Outer membrane machinery and alginate synthesis regulators control membrane vesicle production in Pseudomonas aeruginosa. J Bacteriol [Internet]. 2009;191(24):7509–7519. doi:10.1128/JB.00722-09.
- McBroom AJ, Johnson AP, Vemulapalli S, Kuehn MJ. Outer membrane vesicle production by Escherichia coli is independent of membrane instability. J Bacteriol [Internet]. 2006;188(15):5385–5392. doi:10.1128/JB.00498-06.
- Hayashi J, Hamada N, Kuramitsu HK. The autolysin of Porphyromonas gingivalis is involved in outer membrane vesicle release. FEMS Microbiol Lett [Internet]. 2002;216(2):217–222. http://www.ncbi.nlm.nih.gov/pubmed/12435505.
- Sartorio MG, Pardue EJ, Feldman MF, Haurat MF. Bacterial outer membrane vesicles: from discovery to applications. Annu Rev Microbiol [Internet]. 2021;75(1):609–630. doi:10.1146/annurev-micro-052821-031444.
- McBroom AJ, Kuehn MJ. Release of outer membrane vesicles by Gram-negative bacteria is a novel envelope stress response. Mol Microbiol [Internet]. 2007;63(2):545–558. http://www.ncbi.nlm.nih.gov/pubmed/17163978.
- Aschtgen M-S, Lynch JB, Koch E, Schwartzman J, McFall-Ngai M, Ruby E, Christie PJ. Rotation of vibrio fischeri flagella produces outer membrane vesicles that induce host development. J Bacteriol [Internet]. 2016;198(16):2156–2165. doi:10.1128/JB.00101-16.
- Turnbull L, Toyofuku M, Hynen AL, Kurosawa M, Pessi G, Petty NK, Osvath SR, Cárcamo-Oyarce G, Gloag ES, Shimoni R, et al. Explosive cell lysis as a mechanism for the biogenesis of bacterial membrane vesicles and biofilms. Nat Commun [Internet]. 2016;7(1):11220. doi:10.1038/ncomms11220.
- Toyofuku M, Zhou S, Sawada I, Takaya N, Uchiyama H, Nomura N. Membrane vesicle formation is associated with pyocin production under denitrifying conditions in P seudomonas aeruginosa PAO 1. Environ Microbiol [Internet]. 2014;16(9):2927–2938. doi:10.1111/1462-2920.12260.
- Kadurugamuwa JL, Beveridge TJ. Bacteriolytic effect of membrane vesicles from Pseudomonas aeruginosa on other bacteria including pathogens: conceptually new antibiotics. J Bacteriol [Internet]. 1996;178(10):2767–2774. doi:10.1128/jb.178.10.2767-2774.1996.
- Toyofuku M, Cárcamo-Oyarce G, Yamamoto T, Eisenstein F, Hsiao C-C, Kurosawa M, Gademann K, Pilhofer M, Nomura N, Eberl L. Prophage-triggered membrane vesicle formation through peptidoglycan damage in Bacillus subtilis. Nat Commun [Internet]. 2017;8(1):481. doi:10.1038/s41467-017-00492-w.
- da Silva Barreira D, Lapaquette P, Novion Ducassou J, Couté Y, Guzzo J, Rieu A, Goldman GH. Spontaneous prophage induction contributes to the production of membrane vesicles by the gram-positive bacterium Lacticaseibacillus casei BL23. MBio [Internet]. 2022;13(5). doi:10.1128/mbio.02375-22.
- Resch U, Tsatsaronis JA, Le Rhun A, Stübiger G, Rohde M, Kasvandik S, Holzmeister S, Tinnefeld P, Wai SN, Charpentier E, et al. A two-component regulatory system impacts extracellular membrane-derived vesicle production in group a streptococcus. MBio [Internet]. 2016;7(6). doi:10.1128/mBio.00207-16.
- Dean SN, Leary DH, Sullivan CJ, Oh E, Walper SA. Isolation and characterization of Lactobacillus-derived membrane vesicles. Sci Rep [Internet]. 2019;9(1):877. doi:10.1038/s41598-018-37120-6.
- Toyofuku M, Schild S, Kaparakis-Liaskos M, Eberl L. Composition and functions of bacterial membrane vesicles. Nat Rev Microbiol [Internet]. 2023;21(7):415–430. doi:10.1038/s41579-023-00875-5.
- Schlatterer K, Beck C, Hanzelmann D, Lebtig M, Fehrenbacher B, Schaller M, Ebner P, Nega M, Otto M, Kretschmer D, et al. The mechanism behind bacterial lipoprotein release: phenol-soluble modulins mediate toll-like receptor 2 activation via extracellular vesicle release from staphylococcus aureus. MBio [Internet]. 2018;9(6). doi:10.1128/mBio.01851-18.
- Wang X, Eagen WJ, Lee JC. Orchestration of human macrophage NLRP3 inflammasome activation by Staphylococcus aureus extracellular vesicles. Proc Natl Acad Sci [Internet]. 2020;117(6):3174–3184. doi:10.1073/pnas.1915829117.
- Andreoni F, Toyofuku M, Menzi C, Kalawong R, Mairpady Shambat S, François P, Zinkernagel AS, Eberl L. Antibiotics stimulate formation of vesicles in staphylococcus aureus in both phage-dependent and -independent fashions and via different routes. Antimicrob Agents Chemother [Internet]. 2019;63(2). doi:10.1128/AAC.01439-18.
- Vermassen T, Andant P, Desvaux L. Cell-wall hydrolases as antimicrobials against staphylococcus species: focus on Sle1. Microorganisms. 2019;7(11):559. https://www.mdpi.com/2076-2607/7/11/559.
- Dineshkumar K, Aparna V, Wu L, Wan J, Abdelaziz MH, Su Z, Wang S, Xu H. Bacterial bug-out bags: outer membrane vesicles and their proteins and functions. J Microbiol [Internet]. 2020;58(7):531–542. doi:10.1007/s12275-020-0026-3.
- Yu Y, Wang X, Fan G. Versatile effects of bacterium-released membrane vesicles on mammalian cells and infectious/inflammatory diseases. Acta Pharmacol Sin [Internet]. 2017;39(4):514–533. https://www.semanticscholar.org/paper/9a0f9369ab7f811db5dc161c47d5c99fe1d375a6.
- Briaud P, Carroll RK, Richardson AR. Extracellular vesicle biogenesis and functions in gram-positive bacteria. Infect Immun. 2020;88(12):10–128. doi:10.1128/IAI.00433-20.
- Kim JH, Lee J, Park J, Gho YS. Gram-negative and Gram-positive bacterial extracellular vesicles. Semin Cell Dev Biol [Internet]. 2015;40:97–104. https://linkinghub.elsevier.com/retrieve/pii/S1084952115000336.
- Park JH, Song S, Kim S, Kim M, Kim K-S. Optimizing conditions for the production of bacterial extracellular vesicles of vibrio vulnificus and analysis of the inner small RNA compositions. J Microbiol Biotechnol [Internet]. 2024;34(1):29–38. doi:10.4014/jmb.2310.10002.
- Mao H, Gong T, Sun Y, Yang S, Qiao X, Yang D. Bacterial growth stage determines the yields, protein composition, and periodontal pathogenicity of Porphyromonas gingivalis outer membrane vesicles. Front Cell Infect Microbiol. 2023;13. doi:10.3389/fcimb.2023.1193198/full.
- Aytar Çelik P, Derkuş B, Erdoğan K, Barut D, Blaise Manga E, Y Y, Pecha S, A Ç. Bacterial membrane vesicle functions, laboratory methods, and applications. Biotechnol Adv [Internet]. 2022;54:107869. https://linkinghub.elsevier.com/retrieve/pii/S0734975021001750.
- Li M, Zhou H, Yang C, Wu Y, Zhou X, Liu H, Wang Y. Bacterial outer membrane vesicles as a platform for biomedical applications: an update. J Control Release [Internet]. 2020;323:253–268. https://linkinghub.elsevier.com/retrieve/pii/S0168365920302455.
- Chen DJ, Osterrieder N, Metzger SM, Buckles E, Doody AM, DeLisa MP, Putnam D. Delivery of foreign antigens by engineered outer membrane vesicle vaccines. Proc Natl Acad Sci [Internet]. 2010;107(7):3099–3104. doi:10.1073/pnas.0805532107.
- Wang X, Ni J, You Y, Feng G, Zhang S, Bao W, Hou H, Li H, Liu L, Zheng M, et al. SNX10‐mediated LPS sensing causes intestinal barrier dysfunction via a caspase‐5‐dependent signaling cascade. Embo J [Internet]. 2021;40(24): doi:10.15252/embj.2021108080.
- Zavan L, Bitto NJ, Johnston EL, Greening DW, Kaparakis‐Liaskos M. Back cover: helicobacter pylori growth stage determines the size, protein composition, and preferential cargo packaging of outer membrane vesicles. Proteomics [Internet]. 2019;19(1–2). doi:10.1002/pmic.201800209.
- Sharif E, Eftekhari Z, Mohit E. The effect of growth stage and isolation method on properties of ClearColi™ Outer Membrane Vesicles (OMVs). Curr Microbiol [Internet]. 2021;78(4):1602–1614. doi:10.1007/s00284-021-02414-y.
- Pérez‐Cruz C, Briansó F, Sonnleitner E, Bläsi U, Mercadé E. RNA release via membrane vesicles in Pseudomonas aeruginosa PAO1 is associated with the growth phase. Environ Microbiol [Internet]. 2021;23(9):5030–5041. doi:10.1111/1462-2920.15436.
- Turner L, Bitto NJ, Steer DL, Lo C, D’Costa K, Ramm G, Shambrook M, Hill AF, Ferrero RL, Kaparakis-Liaskos M. Helicobacter pylori outer membrane vesicle size determines their mechanisms of host cell entry and protein content. Front Immunol [Internet]. 2018;9. doi:10.3389/fimmu.2018.01466/full.
- Lynch JB, Schwartzman JA, Bennett BD, McAnulty SJ, Knop M, Nyholm SV, Ruby EG, Silhavy TJ. Ambient pH alters the protein content of outer membrane vesicles, driving host development in a beneficial symbiosis. J Bacteriol [Internet]. 2019;201(20). doi:10.1128/JB.00319-19
- Johnston EL, Guy‐Von Stieglitz S, Zavan L, Cross J, Greening DW, Hill AF, Kaparakis‐Liaskos M. The effect of altered pH growth conditions on the production, composition, and proteomes of Helicobacter pylori outer membrane vesicles. Proteomics [Internet]. 2023. doi:10.1002/pmic.202300269.
- Zhang X, Wang Y, Fan R, Zhang L, Li Z, Zhang Y, Zheng W, Wang L, Liu B, Quan C, et al. Quantitative proteomic analysis of outer membrane vesicles from fusobacterium nucleatum cultivated in the mimic cancer environment. Microbiol Spectr. 2023;11(4):11. doi:10.1128/spectrum.00394-23.
- Jeon H, Oh MH, Jun SH, Kim SI, Choi CW, Kwon HI, Na SH, Kim YJ, Nicholas A, Selasi GN, et al. Variation among Staphylococcus aureus membrane vesicle proteomes affects cytotoxicity of host cells. Microb Pathog [Internet]. 2016;93:185–193. doi:10.1016/j.micpath.2016.02.014.
- Reimer SL, Beniac DR, Hiebert SL, Booth TF, Chong PM, Westmacott GR, Zhanel GG, Bay DC. Comparative analysis of outer membrane vesicle isolation methods with an escherichia coli tolA mutant reveals a hypervesiculating phenotype with outer-inner membrane vesicle content. Front Microbiol [Internet]. 2021;12. doi:10.3389/fmicb.2021.628801/full.
- Klimentová J, Stulík J. Methods of isolation and purification of outer membrane vesicles from gram-negative bacteria. Microbiol Res [Internet]. 2015;170:1–9. https://linkinghub.elsevier.com/retrieve/pii/S0944501314001153.
- Bitto NJ, Zavan L, Johnston EL, Stinear TP, Hill AF, Kaparakis-Liaskos M, Edelmann MJ. Considerations for the analysis of bacterial membrane vesicles: methods of vesicle production and quantification can influence biological and experimental outcomes. Microbiol Spectr. 2021;9(3):e01273–21. doi:10.1128/Spectrum.01273-21.
- Begić M, Josić D. Biofilm formation and extracellular microvesicles—The way of foodborne pathogens toward resistance. Electrophoresis [Internet]. 2020;41(20):1718–1739. doi:10.1002/elps.202000106.
- He X, Li S, Yin Y, Xu J, Gong W, Li G, Qian L, Yin Y, He X, Guo T, et al. Membrane vesicles are the dominant structural components of ceftazidime-induced biofilm formation in an oxacillin-sensitive MRSA. Front Microbiol [Internet]. 2019;10. doi:10.3389/fmicb.2019.00571/full.
- van Hoek ML. Biofilms. Virulence [Internet]. 2013;4(8):833–846. doi:10.4161/viru.27023.
- Cooke AC, Florez C, Dunshee EB, Lieber AD, Terry ML, Light CJ, Schertzer JW, Ellermeier CD. Pseudomonas quinolone signal-induced outer membrane vesicles enhance biofilm dispersion in Pseudomonas aeruginosa. mSphere [Internet]. 2020;5(6). doi:10.1128/mSphere.01109-20.
- Manning AJ, Kuehn MJ. Contribution of bacterial outer membrane vesicles to innate bacterial defense. BMC Microbiol [Internet]. 2011;11(1):258. doi:10.1186/1471-2180-11-258.
- Kulkarni HM, Nagaraj R, Jagannadham MV. Protective role of E. coli outer membrane vesicles against antibiotics. Microbiol Res [Internet]. 2015;181:1–7. https://linkinghub.elsevier.com/retrieve/pii/S0944501315001342.
- Lee J, Lee E-Y, Kim S-H, Kim D-K, Park K-S, Kim KP, Kim Y-K, Roh T-Y, Gho YS. Staphylococcus aureus extracellular vesicles carry biologically active β-lactamase. Antimicrob Agents Chemother. 2013;57(6):2589–2595. doi:10.1128/AAC.00522-12.
- Schaar V, Nordström T, Mörgelin M, Riesbeck K. Moraxella catarrhalis outer membrane vesicles carry β-lactamase and promote survival of streptococcus pneumoniae and haemophilus influenzae by inactivating amoxicillin. Antimicrob Agents Chemother. 2011;55(8):3845–3853. doi:10.1128/AAC.01772-10.
- Azam AH, Tanji Y. Bacteriophage-host arm race: an update on the mechanism of phage resistance in bacteria and revenge of the phage with the perspective for phage therapy. Appl Microbiol Biotechnol [Internet]. 2019;103(5):2121–2131. doi:10.1007/s00253-019-09629-x.
- YashRoy RC. Outer membrane vesicles of gram-negative bacteria: nanoware for combat against microbes and macrobes [Internet]. In: Ficai, A, Grumezescu, AM, editors. Nanostructures for Antimicrobial Therapy. Elsevier; 2017. pp. 341–367. doi:10.1016/B978-0-323-46152-8.00015-9.
- Reyes-Robles T, Dillard RS, Cairns LS, Silva-Valenzuela CA, Housman M, Ali A, Wright ER, Camilli A, DiRita VJ. Vibrio cholerae outer membrane vesicles inhibit bacteriophage infection. J Bacteriol [Internet]. 2018;200(15). doi:10.1128/JB.00792-17.
- Park AJ, Surette MD, Khursigara CM. Antimicrobial targets localize to the extracellular vesicle-associated proteome of Pseudomonas aeruginosa grown in a biofilm. Front Microbiol [Internet]. 2014;5. doi:10.3389/fmicb.2014.00464.
- Tran F, Boedicker JQ, Becker A. Plasmid characteristics modulate the propensity of gene exchange in bacterial vesicles. J Bacteriol [Internet]. 2019;201(7). doi:10.1128/JB.00430-18.
- Domingues S, Nielsen KM. Membrane vesicles and horizontal gene transfer in prokaryotes. Curr Opin Microbiol [Internet]. 2017;38:16–21. https://linkinghub.elsevier.com/retrieve/pii/S136952741630193X.
- Carvalho AL, Fonseca S, Miquel‐Clopés A, Cross K, Kok K, Wegmann U, Gil‐Cardoso K, Bentley EG, Al Katy SHM, Coombes JL, et al. Bioengineering commensal bacteria‐derived outer membrane vesicles for delivery of biologics to the gastrointestinal and respiratory tract. J Extracell Vesicles [Internet]. 2019;8(1). doi:10.1080/20013078.2019.1632100.
- Bitto N, Kaparakis-Liaskos M. The therapeutic benefit of bacterial membrane vesicles. Int J Mol Sci Int. 2017;18(6):1287. http://www.mdpi.com/1422-0067/18/6/1287.
- Cai W, Kesavan DK, Wan J, Abdelaziz MH, Su Z, Xu H. Bacterial outer membrane vesicles, a potential vaccine candidate in interactions with host cells based. Diagn Pathol [Internet]. 2018;13(1):95. doi:10.1186/s13000-018-0768-y.
- Toyofuku M, Morinaga K, Hashimoto Y, Uhl J, Shimamura H, Inaba H, Schmitt-Kopplin P, Eberl L, Nomura N. Membrane vesicle-mediated bacterial communication. ISME J [Internet]. 2017;11(6):1504–1509. https://www.nature.com/articles/ismej201713.
- Caruana JC, Walper SA. Bacterial membrane vesicles as mediators of microbe – microbe and microbe – host community interactions. Front Microbiol [Internet]. 2020;11:11. doi:10.3389/fmicb.2020.00432/full.
- Vasilyeva NV, Tsfasman IM, Suzina NE, Stepnaya OA, Kulaev IS. Secretion of bacteriolytic endopeptidase L5 of Lysobacter sp. XL1 into the medium by means of outer membrane vesicles. FEBS J [Internet]. 2008;275(15):3827–3835. doi:10.1111/j.1742-4658.2008.06530.x.
- Caruana JC, Walper SA. Bacterial membrane vesicles and their applications as vaccines and in biotechnology. 2020. https://www.semanticscholar.org/paper/9b556ffbbfa484bc5995a57f00c6b10fcdef1783.
- O’Donoghue EJ, Krachler AM. Mechanisms of outer membrane vesicle entry into host cells. Cell Microbiol [Internet]. 2016;18(11):1508–1517. doi:10.1111/cmi.12655.
- Kaparakis-Liaskos M, Ferrero RL. Immune modulation by bacterial outer membrane vesicles. Nat Rev Immunol [Internet]. 2015;15(6):375–387. doi:10.1038/nri3837.
- Weiner A, Mellouk N, Lopez-Montero N, Chang Y-Y, Souque C, Schmitt C, Enninga J, Luo Z-Q. Macropinosomes are key players in early shigella invasion and vacuolar escape in epithelial cells. PLOS Pathog [Internet]. 2016;12(5):e1005602. doi:10.1371/journal.ppat.1005602.
- Olofsson A, Nygård Skalman L, Obi I, Lundmark R, Arnqvist A, Kuehn M, Hultgren SJ. Uptake of Helicobacter pylori vesicles is facilitated by clathrin-dependent and clathrin-independent endocytic pathways. MBio [Internet]. 2014;5(3). doi:10.1128/mBio.00979-14.
- Cañas M-A, Fábrega M-J, Giménez R, Badia J, Baldomà L. Outer membrane vesicles from probiotic and commensal Escherichia coli activate NOD1-mediated immune responses in intestinal epithelial cells. Front Microbiol [Internet]. 2018;9:498. http://www.ncbi.nlm.nih.gov/pubmed/29616010.
- Rewatkar PV, Parton RG, Parekh HS, Parat M-O. Are caveolae a cellular entry route for non-viral therapeutic delivery systems? Adv Drug Deliv Rev [Internet]. 2015;91:92–108. https://linkinghub.elsevier.com/retrieve/pii/S0169409X15000058.
- Chatterjee D, Chaudhuri K. Association of cholera toxin with Vibrio cholerae outer membrane vesicles which are internalized by human intestinal epithelial cells. FEBS Lett [Internet]. 2011;585(9):1357–1362. http://www.ncbi.nlm.nih.gov/pubmed/21510946.
- Sharpe SW, Kuehn MJ, Mason KM, Weiser JN. Elicitation of epithelial cell-derived immune effectors by outer membrane vesicles of nontypeable haemophilus influenzae. Infect Immun. 2011;79(11):4361–4369. doi:10.1128/IAI.05332-11.
- Jäger J, Keese S, Roessle M, Steinert M, Schromm AB. Fusion of L egionella pneumophila outer membrane vesicles with eukaryotic membrane systems is a mechanism to deliver pathogen factors to host cell membranes. Cell Microbiol [Internet]. 2015;17(5):607–620. doi:10.1111/cmi.12392.
- Bomberger JM, MacEachran DP, Coutermarsh BA, Ye S, O’Toole GA, Stanton BA, Ausubel FM. Long-distance delivery of bacterial virulence factors by pseudomonas aeruginosa outer membrane vesicles. PLOS Pathog [Internet]. 2009;5(4):e1000382. doi:10.1371/journal.ppat.1000382.
- Kuipers ME, Hokke CH, Smits HH, Nolte-‘t Hoen ENM. Pathogen-derived extracellular vesicle-associated molecules that affect the host immune system: an overview. Front Microbiol [Internet]. 2018;9. doi:10.3389/fmicb.2018.02182/full.
- Qu M, Zhu H, Zhang X. Extracellular vesicle-mediated regulation of macrophage polarization in bacterial infections. Front Microbiol [Internet]. 2022;13. doi:10.3389/fmicb.2022.1039040.
- Diaz-Garrido N, Badia J, Baldomà L. Modulation of dendritic cells by microbiota extracellular vesicles influences the cytokine profile and exosome cargo. Nutr. 2022;14(2):344. https://www.mdpi.com/2072-6643/14/2/344.
- Soderblom T, Oxhamre C, Wai SN, Uhlen P, Aperia A, Uhlin BE, Richter-Dahlfors A. Effects of the Escherichia coli toxin cytolysin a on mucosal immunostimulation via epithelial Ca2+ signalling and Toll-like receptor 4. Cell Microbiol [Internet]. 2005;7(6):779–788. doi:10.1111/j.1462-5822.2005.00510.x.
- Zhao K, Deng X, He C, Yue B, Wu M, McCormick BA. Pseudomonas aeruginosa outer membrane vesicles modulate host immune responses by targeting the toll-like receptor 4 signaling pathway. Infect Immun. 2013;81(12):4509–4518. doi:10.1128/IAI.01008-13.
- Bitto NJ, Cheng L, Johnston EL, Pathirana R, Phan TK, Poon IKH, O’Brien‐Simpson NM, Hill AF, Stinear TP, Kaparakis‐Liaskos M. Staphylococcus aureus membrane vesicles contain immunostimulatory DNA, RNA and peptidoglycan that activate innate immune receptors and induce autophagy. J Extracell Vesicles [Internet]. 2021;10(6). doi:10.1002/jev2.12080.
- Kaparakis M, Turnbull L, Carneiro L, Firth S, Coleman HA, Parkington HC, Le Bourhis L, Karrar A, Viala J, Mak J, et al. Bacterial membrane vesicles deliver peptidoglycan to NOD1 in epithelial cells. Cell Microbiol [Internet]. 2010;12(3):372–385. doi:10.1111/j.1462-5822.2009.01404.x.
- Thay B, Damm A, Kufer TA, Wai SN, Oscarsson J, Blanke SR. Aggregatibacter actinomycetemcomitans outer membrane vesicles are internalized in human host cells and trigger NOD1- and NOD2-dependent NF-κB activation. Infect Immun. 2014;82(10):4034–4046. doi:10.1128/IAI.01980-14.
- Diaz-Garrido N, Fábrega MJ, Vera R, Giménez R, Badia J, Baldomà L. Membrane vesicles from the probiotic Nissle 1917 and gut resident Escherichia coli strains distinctly modulate human dendritic cells and subsequent T cell responses. J Funct Foods [Internet]. 2019;61:61. doi:10.1016/j.jff.2019.103495.
- Bielaszewska M, Marejková M, Bauwens A, Kunsmann-Prokscha L, Mellmann A, Karch H. Enterohemorrhagic Escherichia coli O157 outer membrane vesicles induce interleukin 8 production in human intestinal epithelial cells by signaling via Toll-like receptors TLR4 and TLR5 and activation of the nuclear factor NF-κB. Int J Med Microbiol [Internet]. 2018;308(7):882–889. http://www.ncbi.nlm.nih.gov/pubmed/29934223.
- Ma L, Shen Q, Lyu W, Lv L, Wang W, Yu M, Yang H, Tao S, Xiao Y, Claesen J. Clostridium butyricum and its derived extracellular vesicles modulate gut homeostasis and ameliorate acute experimental colitis. Microbiol Spectr. 2022;10(4). doi:10.1128/spectrum.01368-22.
- Ye L, Wang Y, Xiao F, Wang X, Li X, Cao R, Zhang J, Zhang T. F. prausnitzii-derived extracellular vesicles attenuate experimental colitis by regulating intestinal homeostasis in mice. Microb Cell Fact [Internet]. 2023;22(1):235. doi:10.1186/s12934-023-02243-7.
- Macia L, Nanan R, Hosseini-Beheshti E, Grau GE. Host- and microbiota-derived extracellular vesicles, immune function, and disease development. Int J Mol Sci Int. 2019;21(1):107. https://www.mdpi.com/1422-0067/21/1/107.
- Villard A, Boursier J, Andriantsitohaina R. Microbiota‐derived extracellular vesicles and metabolic syndrome. Acta Physiol [Internet]. 2021;231(4). doi:10.1111/apha.13600.
- Tian C, Yang M, Xu H, Zhu M, Zhang Y, Yao J, Wang L, Liang Y, Li D. Emerging role of bacterial outer membrane vesicle in gastrointestinal tract. Gut Pathog [Internet]. 2023;15(1):20. doi:10.1186/s13099-023-00543-2.
- Qi Y, Wu H, Yang Z, Zhou Y, Jin L, Yang M, Wang F. New insights into the role of oral microbiota dysbiosis in the pathogenesis of inflammatory bowel disease. Dig Dis Sci [Internet]. 2022;67(1):42–55. doi:10.1007/s10620-021-06837-2.
- Hickey CA, Kuhn KA, Donermeyer DL, Porter NT, Jin C, Cameron EA, Jung H, Kaiko GE, Wegorzewska M, Malvin NP, et al. Colitogenic bacteroides thetaiotaomicron antigens access host immune cells in a sulfatase-dependent manner via outer membrane vesicles. Cell Host Microbe [Internet]. 2015;17(5):672–680. https://linkinghub.elsevier.com/retrieve/pii/S1931312815001602.
- Thapa HB, Kohl P, Zingl FG, Fleischhacker D, Wolinski H, Kufer TA, Schild S, Josenhans C. Characterization of the inflammatory response evoked by bacterial membrane vesicles in intestinal cells reveals an RIPK2-dependent activation by enterotoxigenic escherichia coli vesicles. Microbiol Spectr. 2023;11(4):11. doi:10.1128/spectrum.01115-23.
- Kunsmann L, Rüter C, Bauwens A, Greune L, Glüder M, Kemper B, Fruth A, Wai SN, He X, Lloubes R, et al. Virulence from vesicles: Novel mechanisms of host cell injury by Escherichia coli O104: H4 outbreak strain. Sci Rep [Internet]. 2015;5(1):13252.https://www.nature.com/articles/srep13252.
- Rolhion N, Barnich N, Claret L, Darfeuille-Michaud A. Strong decrease in invasive ability and outer membrane vesicle release in crohn’s disease-associated adherent-invasive escherichia coli strain LF82 with the yfgL gene deleted. J Bacteriol [Internet]. 2005;187:2286–2296. doi:10.1128/JB.187.7.2286-2296.2005.
- Wei S, Zhang J, Wu X, Chen M, Huang H, Zeng S, Xiang Z, Li X, Dong W. Fusobacterium nucleatum extracellular vesicles promote experimental colitis by modulating autophagy via the miR-574-5p/CARD3 axis. Inflamm Bowel Dis [Internet]. 2023;29(1):9–26. https://academic.oup.com/ibdjournal/article/29/1/9/6674006.
- Pavkova I, Klimentova J, Bavlovic J, Horcickova L, Kubelkova K, Vlcak E, Raabova H, Filimonenko V, Ballek O, Stulik J. Francisella tularensis outer membrane vesicles participate in the early phase of interaction with macrophages. Front Microbiol [Internet]. 2021;12:748706. doi:10.3389/fmicb.2021.748706/full.
- Tulkens J, Vergauwen G, Van Deun J, Geeurickx E, Dhondt B, Lippens L, De Scheerder M-A, Miinalainen I, Rappu P, De Geest BG, et al. Increased levels of systemic LPS-positive bacterial extracellular vesicles in patients with intestinal barrier dysfunction. Gut [Internet]. 2020;69(1):191–193. doi:10.1136/gutjnl-2018-317726.
- Durant L, Stentz R, Noble A, Brooks J, Gicheva N, Reddi D, O’Connor MJ, Hoyles L, McCartney AL, Man R, et al. Bacteroides thetaiotaomicron-derived outer membrane vesicles promote regulatory dendritic cell responses in health but not in inflammatory bowel disease. Microbiome [Internet]. 2020;8(1):88. doi:10.1186/s40168-020-00868-z.
- Palmela C, Chevarin C, Xu Z, Torres J, Sevrin G, Hirten R, Barnich N, Ng SC, Colombel J-F. Adherent-invasive Escherichia coli in inflammatory bowel disease. Gut [Internet]. 2018;67(3):574–587. doi:10.1136/gutjnl-2017-314903.
- Perna A, Hay E, Contieri M, De Luca A, Guerra G, Lucariello A. Adherent‐invasive Escherichia coli (AIEC): Cause or consequence of inflammation, dysbiosis, and rupture of cellular joints in patients with IBD? J Cell Physiol [Internet]. 2020;235(6):5041–5049. doi:10.1002/jcp.29430.
- Zheng L, Duan S-L, Dai Y-C, S-C W. Role of adherent invasive Escherichia coli in pathogenesis of inflammatory bowel disease. World J Clin Cases [Internet]. 2022;10(32):11671–11689. https://www.wjgnet.com/2307-8960/full/v10/i32/11671.htm.
- Rueter C, Bielaszewska M. Secretion and delivery of intestinal pathogenic escherichia coli virulence factors via outer membrane vesicles. Front Cell Infect Microbiol. 2020;10:91. doi:10.3389/fcimb.2020.00091/full.
- Kameli N, Borman R, López-Iglesias C, Savelkoul P, Stassen FRM. Characterization of feces-derived bacterial membrane vesicles and the impact of their origin on the inflammatory response. Front Cell Infect Microbiol [Internet]. 2021;11:11. doi:10.3389/fcimb.2021.667987/full.
- Barbara G, Barbaro MR, Fuschi D, Palombo M, Falangone F, Cremon C, Marasco G, Stanghellini V. Inflammatory and microbiota-related regulation of the intestinal epithelial barrier. Front Nutr [Internet]. 2021;8:718356. doi:10.3389/fnut.2021.718356/full.
- Srivastava A, Gupta J, Kumar S, Kumar A. Gut biofilm forming bacteria in inflammatory bowel disease. Microb Pathog [Internet]. 2017;112:5–14. https://linkinghub.elsevier.com/retrieve/pii/S0882401017309580.
- Palandurkar GS, Kumar S. Biofilm’s impact on inflammatory bowel diseases. Cureus [Internet]. 2023; https://www.cureus.com/articles/183491-biofilms-impact-on-inflammatory-bowel-diseases
- Baumgartner M, Lang M, Holley H, Crepaz D, Hausmann B, Pjevac P, Moser D, Haller F, Hof F, Beer A, et al. Mucosal biofilms are an endoscopic feature of irritable bowel syndrome and ulcerative colitis. Gastroenterology [Internet]. 2021;161(4):1245–1256.e20. doi:10.1053/j.gastro.2021.06.024.
- Swidsinski A, Weber J, Loening-Baucke V, Hale LP, Lochs H. Spatial organization and composition of the mucosal flora in patients with inflammatory bowel disease. J Clin Microbiol [Internet]. 2005;43(7):3380–3389. doi:10.1128/JCM.43.7.3380-3389.2005.
- Kameli N, Becker HEF, Welbers T, Jonkers DMAE, Penders J, Savelkoul P, Stassen FR. Metagenomic profiling of fecal-derived bacterial membrane vesicles in crohn’s disease patients. Cells [Internet]. 2021;10(10):2795. https://www.mdpi.com/2073-4409/10/10/2795.
- Heo M, Park YS, Yoon H, Kim N-E, Kim K, Shin CM, Kim N, Lee DH. Potential of gut microbe-derived extracellular vesicles to differentiate inflammatory bowel disease patients from healthy controls. Gut Liver [Internet]. 2023;17(1):108–118. doi:10.5009/gnl220081.
- Kang C, Ban M, Choi E-J, Moon H-G, Jeon J-S, Kim D-K, Park S-K, Jeon SG, Roh T-Y, Myung S-J, et al. Extracellular vesicles derived from gut microbiota, especially akkermansia muciniphila, protect the progression of dextran sulfate sodium-induced colitis. PLOS One [Internet]. 2013;8(10):e76520. doi:10.1371/journal.pone.0076520.
- Landgraf P, Rusu M, Sheridan R, Sewer A, Iovino N, Aravin A, Pfeffer S, Rice A, Kamphorst AO, Landthaler M, et al. A mammalian microRNA expression atlas based on small RNA library sequencing. Cell [Internet]. 2007;129(7):1401–1414. doi:10.1016/j.cell.2007.04.040.
- Liu D, Saikam V, Skrada KA, Merlin D, Iyer SS. Inflammatory bowel disease biomarkers. Med Res Rev [Internet]. 2022;42(5):1856–1887. doi:10.1002/med.21893.
- Polytarchou C, Hommes DW, Palumbo T, Hatziapostolou M, Koutsioumpa M, Koukos G, van der Meulen-de Jong AE, Oikonomopoulos A, van Deen WK, Vorvis C, et al. MicroRNA214 is associated with progression of ulcerative colitis, and inhibition reduces development of colitis and colitis-associated cancer in mice. Gastroenterology [Internet]. 2015;149(4):981–992.e11. doi:10.1053/j.gastro.2015.05.057.
- Ma L, Lyu W, Song Y, Chen K, Lv L, Yang H, Wang W, Xiao Y. Front Cover: Anti-Inflammatory Effect of Clostridium butyricum -Derived Extracellular Vesicles in Ulcerative Colitis: Impact on Host microRNAs Expressions and Gut Microbiome Profiles. Mol Nutr Food Res [Internet]. 2023;67(13). doi:10.1002/mnfr.202200884.
- Kuhn T, Koch M, Fuhrmann G. Probiomimetics—novel lactobacillus ‐mimicking microparticles show anti‐inflammatory and barrier‐protecting effects in gastrointestinal models. Small [Internet]. 2020;16(40). doi:10.1002/smll.202003158.
- Kang EA, Choi H-I, Hong SW, Kang S, Jegal H-Y, Choi EW, Park B-S, Kim JS. Extracellular vesicles derived from kefir grain lactobacillus ameliorate intestinal inflammation via regulation of proinflammatory pathway and tight junction integrity. Biomedicines Int [Internet]. 2020;8(11):522.
- Liang L, Yang C, Liu L, Mai G, Li H, Wu L, Jin M, Chen Y. Commensal bacteria-derived extracellular vesicles suppress ulcerative colitis through regulating the macrophages polarization and remodeling the gut microbiota. Microb Cell Fact [Internet]. 2022;21(1):88. doi:10.1186/s12934-022-01812-6.
- Fábrega M-J, Rodríguez-Nogales A, Garrido-Mesa J, Algieri F, Badía J, Giménez R, Gálvez J, Baldomà L. Intestinal anti-inflammatory effects of outer membrane vesicles from escherichia coli nissle 1917 in DSS-experimental colitis in Mice. Front Microbiol [Internet]. 2017;8. doi:10.3389/fmicb.2017.01274/full.
- Choi JH, Moon CM, Shin T-S, Kim EK, McDowell A, Jo M-K, Joo YH, Kim S-E, Jung H-K, Shim K-N, et al. Lactobacillus paracasei-derived extracellular vesicles attenuate the intestinal inflammatory response by augmenting the endoplasmic reticulum stress pathway. Exp Mol Med [Internet]. 2020;52(3):423–437. http://www.nature.com/articles/s12276-019-0359-3.
- Liang D, Liu C, Li Y, Wu C, Chen Y, Tan M, Su W. Engineering fucoxanthin-loaded probiotics’ membrane vesicles for the dietary intervention of colitis. Biomaterials [Internet]. 2023;297:122107. https://linkinghub.elsevier.com/retrieve/pii/S0142961223001151.
- Shen Y, Torchia MLG, Lawson GW, Karp CL, Ashwell JD, Mazmanian SK. Outer membrane vesicles of a human commensal mediate immune regulation and disease protection. Cell Host Microbe [Internet]. 2012;12(4):509–520. https://linkinghub.elsevier.com/retrieve/pii/S1931312812002752.
- Rodovalho VDR, Luz BD, Rabah H, Do Carmo FLR, Folador EL, Nicolas A, Jardin J, Briard-Bion V, Blottière H, Lapaque N, et al. Extracellular vesicles produced by the probiotic propionibacterium freudenreichii CIRM-BIA 129 mitigate inflammation by modulating the NF-κB pathway. Front Microbiol [Internet]. 2020;11:11. doi:10.3389/fmicb.2020.01544/full.
- Alpdundar Bulut E, Bayyurt Kocabas B, Yazar V, Aykut G, Guler U, Salih B, Surucu Yilmaz N, Ayanoglu IC, Polat MM, Akcali KC, et al. Human gut commensal membrane vesicles modulate inflammation by generating m2-like macrophages and myeloid-derived suppressor Cells. J Immunol [Internet]. 2020;205(10):2707–2718.https://journals.aai.org/jimmunol/article/205/10/2707/107686/Human-Gut-Commensal-Membrane-Vesicles-Modulate.
- Fonseca S, Carvalho AL, Miquel-Clopés A, Jones EJ, Juodeikis R, Stentz R, Carding SR. Extracellular vesicles produced by the human gut commensal bacterium Bacteroides thetaiotaomicron elicit anti-inflammatory responses from innate immune cells. Front Microbiol [Internet]. 2022;13:1050271. doi:10.3389/fmicb.2022.1050271/full.
- Suzuki T. Regulation of intestinal epithelial permeability by tight junctions. Cell Mol Life Sci [Internet]. 2013;70(4):631–659. doi:10.1007/s00018-012-1070-x.
- Chang X, Wang S-L, Zhao S-B, Shi Y-H, Pan P, Gu L, Yao J, Z-S L, Bai Y. Extracellular vesicles with possible roles in gut intestinal tract homeostasis and IBD. Mediators Inflamm [Internet]. 2020;2020:1–14. https://www.hindawi.com/journals/mi/2020/1945832/.
- Shen Q, Huang Z, Ma L, Yao J, Luo T, Zhao Y, Xiao Y, Jin Y. Extracellular vesicle miRnas promote the intestinal microenvironment by interacting with microbes in colitis. Gut Microbes [Internet]. 2022;14(1). doi:10.1080/19490976.2022.2128604.
- Hu R, Lin H, Li J, Zhao Y, Wang M, Sun X, Min Y, Gao Y, Yang M. Probiotic Escherichia coli Nissle 1917-derived outer membrane vesicles enhance immunomodulation and antimicrobial activity in RAW264.7 macrophages. BMC Microbiol [Internet]. 2020;20(1):268. doi: 10.1186/s12866-020-01953-x.
- Müller L, Kuhn T, Koch M, Fuhrmann G. Stimulation of probiotic bacteria induces release of membrane vesicles with augmented anti-inflammatory activity. ACS Appl Bio Mater [Internet]. 2021;4(5):3739–3748. doi:10.1021/acsabm.0c01136.
- Li J, Sun M, Liu L, Yang W, Sun A, Yu J, Liu D, Zhao W, Cheng M, He Z, et al. Nanoprobiotics for remolding the pro-inflammatory microenvironment and microbiome in the treatment of colitis. Nano Lett [Internet]. 2023;23(18):8593–8601. doi:10.1021/acs.nanolett.3c02408.
- Liu Y, Liao F. Vaccination therapy for inflammatory bowel disease. Hum Vaccin Immunother [Internet]. 2023;19(2). doi:10.1080/21645515.2023.2259418.
- Daley A, Randall R, Darsley M, Choudhry N, Thomas N, Sanderson IR, Croft NM, Kelly P. Genetically modified enterotoxigenic Escherichia coli vaccines induce mucosal immune responses without inflammation. Gut [Internet]. 2007;56(11):1550–1556. doi:10.1136/gut.2006.112805.
- Krishnan N, Kubiatowicz LJ, Holay M, Zhou J, Fang RH, Zhang L. Bacterial membrane vesicles for vaccine applications. Adv Drug Deliv Rev [Internet]. 2022;185:114294. https://linkinghub.elsevier.com/retrieve/pii/S0169409X22001843.
- Nakao R, Kobayashi H, Iwabuchi Y, Kawahara K, Hirayama S, Ramstedt M, Sasaki Y, Kataoka M, Akeda Y, Ohnishi M. A highly immunogenic vaccine platform against encapsulated pathogens using chimeric probiotic Escherichia coli membrane vesicles. NPJ Vaccines [Internet]. 2022;7(1):153. doi:10.1038/s41541-022-00572-z.
- Aitken JM, Phan K, Bodman SE, Sharma S, Watt A, George PM, Agrawal G, Tie ABM. A mycobacterium species for crohn’s disease? Pathology [Internet]. 2021;53:818–823. https://linkinghub.elsevier.com/retrieve/pii/S0031302521002348.
- Lee J-J, Abdullah M, Liu J, Carvalho IA, Junior AS, Moreira MAS, Mohammed H, DeLisa MP, McDonough SP, Chang Y-F. Proteomic profiling of membrane vesicles from Mycobacterium avium subsp. paratuberculosis: Navigating towards an in silico design of a multi-epitope vaccine targeting membrane vesicle proteins. J Proteomics [Internet]. 2024;292:105058. https://linkinghub.elsevier.com/retrieve/pii/S1874391923002476.