ABSTRACT
Background: The claustrum is the most densely connected brain structure by volume and has extensive reciprocal connections with numerous cortical areas. It is divided into two embryologically distinct parts – dorsal and ventral claustrum. Despite numerous recent findings, much remains unknown about its structure and function.
Objective: The aim of our study was to observe and describe the ultrastructural characteristics of the different types of neurons in the dorsal claustrum of the cat and to discuss their possible functional significance.
Design: We used a total of 10 healthy young male cats. The obtained histological material was processed in the usual way for transmission electron microscopy.
Results: We observed four types of neurons in the dorsal claustrum of cats – large, medium-sized, small and ‘dwarf’ cells. While large and medium-sized neurons had a large electron-lucent nucleus with a wide cytoplasmic ring and an abundance of cellular organelles, small neurons had electron-lucent nuclei with varying degrees of dispersed and condensed chromatin and were poor in organelles. ‘Dwarf’ cells had the typical appearance of neurogliaform cells.
Conclusion: Based on their ultrastructural features, we recognized large and medium-sized neurons as efferent, or projection neurons. On the other hand, small neurons and neurogliaform ‘dwarf’ cells had the typical ultrastructure of inhibitory interneurons.
KEYWORDS:
Introduction
The claustrum is a thin sheet of neurons located between the neocortex and the striatum and is flanked by the fibers of the extreme and external capsule (Citation1–Citation6). The first description of this structure was made by the renowned French anatomist Vicq d’Azyr (Citation7–Citation9). The claustrum is the most densely connected brain structure by volume and has extensive reciprocal connections with numerous cortical areas, such as the motor, somatosensory, visual, auditory, prefrontal and limbic cortices (Citation1,Citation4,Citation10). In addition, the claustral neurons send efferent projections to other subcortical and allocortical structures, such as the striatum, the dorsal thalamic nuclei, the hippocampus and the subiculum (Citation11–Citation16). A number of more recent studies have also explored the afferent and efferent connectivity between the claustrum and the parahippocampal, somatosensory-motor and visual-motor cortices, as well as the prefrontal and entorhinal cortices (Citation17–Citation23).
The claustrum is subdivided into two embryologically distinct parts (Citation24). The dorsal claustrum, also known as insular claustrum, refers to the neurons located above the rhinal fissure and medial to the insular cortex and is derived from the lateral pallium (Citation5,Citation7,Citation9,Citation24,Citation25). The ventral claustrum, also termed endopiriform nucleus, is the name of the group of neurons situated below the rhinal fissure and medial to the piriform cortex and is derived from the ventral pallium (Citation3,Citation6,Citation25,Citation26).
Several studies described different types of neurons in the claustrum. Mamos et al. (Citation27) reported the presence of pyramidal, spindle-shaped and multipolar spiny neurons, as well as large and small multipolar aspiny neurons in the dorsal claustrum of cats. LeVay and Sherk (Citation28) described large spiny neurons, as well as typical Golgi type II interneurons with aspiny dendrites, along with a third type of cells similar to the Golgi type II neurons but with a wider dendritic tree. Multipolar spiny and aspiny neurons were also observed in the claustrum of the hedgehog (Citation13). In the primate claustrum, one study described the presence of pyramidal or spindle-shaped spiny neurons with one long apical dendrite, along with two types of aspiny neurons – large and small (Citation29). One electron microscopic study in the cat claustrum reported that the cytoplasm of small neurons was poor in cellular organelles, while large neurons contained an abundance of endoplasmic reticulum (ER), ribosomes and Golgi complex units (Citation30). Kubasik-Juraniec et al. (Citation31) reported that large and medium-sized cells in the dorsal claustrum were rich in cellular organelles and rough endoplasmic reticulum in particular and possessed a multitude of dendritic spines. They also observed small neurons lacking dendritic spines, which had large nuclei surrounded by a relatively thin cytoplasmic ring, poor in cellular organelles, mostly represented by free ribosomes and mitochondria. In another study, neurons in the dorsal claustrum of the cat were divided into three groups based on their size and their ultrastructural features were studied with the combined method of Fairen (Citation32).
Despite the obvious achievements of these studies, still much remains unknown about the structure of the claustrum. Therefore, the aim of our study was to observe and describe the ultrastructural characteristics of the different types of neurons in the dorsal claustrum of the cat and to discuss their possible functional significance.
Material and methods
We used a total of 10 healthy young male cats, weighing approximately 3–3.5 kg. All experiments were conducted with the approval of the University Committee on Animal Resources. All animals received humane care in compliance with the ‘Principles of laboratory animal care’ formulated by the National Society for Medical Research and the ‘Guide for the care and use of laboratory animals’ prepared by the National Institute of Health (NIH publication No. 86–23, revised 1996). The animals were anesthetized with an intraperitoneal injection of sodium pentobarbital (40 mg kg–1) and were then perfused according to the method of Palay et al. (Citation33). As a perfusion fixative, we used 3% glutaraldehyde in 0.1 M phosphate buffer, pH 7.4. After the perfusion was completed, the animals were decapitated and their heads were left overnight at 4°C. The brain was removed and blocks containing the region of interest, the dorsal claustrum, were obtained. Next, they were post-fixed in 2% osmium tetroxide in 0.1 M phosphate buffer, pH 7.4, for 2 h. After brief rinsing in the 0.1 M phosphate buffer, the samples were dehydrated in increasing concentrations of ethanol up to 100%, treated for 30 min with propylene oxide and embedded in Durcupan. Serial ultrathin sections were cut on a LKB ultratome and mounted on copper grids (75 mesh) and contrasted with 2.5% uranyl acetate, lead nitrate and sodium citrate. The sections were observed under a Hitachi U-11A transmission electron microscope (Hitachi, Ltd., Tokyo, Japan) using acceleration voltage of 100 kV. Micrographs were recorded on 8.25 cm X 10.16 cm Kodak electron image plates (Rochester, NY, USA) at magnifications ranging from ×6,000 to ×57,500.
Results
The fixed material from cat brain was dissected and the claustrum was identified as a thin sheet of grey matter, extending 12–14 mm in oro-caudal direction and 9–11 mm in dorsoventral direction. The frontal pole of the claustrum was found at the level of the rhinal sulcus, approximately 20 mm in front of the interaural line and had the shape of a teardrop (). On slides stained according to the method of Nissl (according to the standard protocol) the claustrum was easily differentiated from the surrounding structures (). Using transmission electron microscopy, we observed the following four types of neurons in the dorsal claustrum of cats: large, medium-sized, small and ‘dwarf’ cells.
Fig. 1. An overview of the dorsal claustrum in cat. (a) A section through the brain showing the site (black arrow) from which the material for electron microscopy was obtained. (b) Histological control image of a Nissl stain showing the localization of the dorsal claustrum. Scale bar −500 µm.
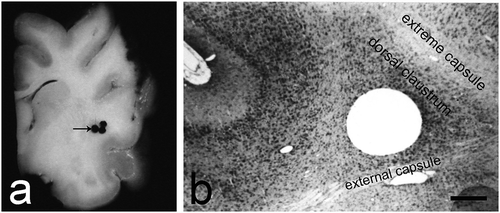
Large neurons
The size of large neurons varied between 21 and 40 µm and the nuclear-cytoplasmic ratio was approximately 1:2. We sub-divided these neurons into four types based on their shape: multipolar, fusiform, cylindrical, and neurons of irregular shape.
Multipolar large neurons
Large multipolar neurons were the most often encountered type (). They had a round to elliptical cellular body and size 20–30 µm. The nucleus was located approximately in the middle of the cell and was surrounded by a wide cytoplasmic ring. The nuclear membrane had an arch-shaped course and formed small invaginations containing a multitude of polysomes. The nuclear pores were uniformly arranged. The nuclear chromatin was uniformly dispersed and we noted an accumulation of condensed chromatin at certain locations under the nuclear membrane. The compact nucleoli had a spherical shape and were eccentrically located; their mean size was 2–2.5 µm. The ribonucleoprotein (RNP) granules and fibrils were arranged in a trabecular pattern in the uniform shape of the so-called nucleolonemma (). The highest number of fibrillar centers (FC) was observed in this type of neurons. They comprised almost the entire nucleolus and had a size of approximately 0.2–0.3 µm, thus forming a typical reticular nucleolus. Nucleolus-associated heterochromatin was scarce. Perichromatin granules were prominent and uniformly dispersed in the border zones of the heterochromatin deposits.
Fig. 2. Multipolar and fusiform large neurons in the dorsal claustrum in cat. (a) Multipolar large neuron with an oval nucleus (N). n – nucleolus; D – dendrite; black arrowhead – glial cell. Scale bar − 3 μm. (b) Multipolar large neuron with an oval nucleus, electron-lucent nucleus (N). D – dendrites. Scale bar − 5.5 μm. (c) Multipolar large neuron with a typical Nissl body. Long black arrows – axo-somatic synaptic contacts; short black arrow – Golgi complex; black arrowhead – lysosomes. Scale bar − 2 μm. (d) Multipolar large neuron with prominent Golgi complex comprising cisterns and small electron-lucent vesicles (black arrows). Scale bar − 1.4 μm. (e) Fusiform large neuron with an oval nucleus (N) and an electron-dense nucleolus (n) containing fibrillar centers. The nuclear membrane forms invaginations of various sizes (long black arrows). Scale bar − 5.5 μm. (f) Fusiform large neuron with a slightly elongated nucleus (N). Long black arrows – invaginations of the nuclear membrane; short black arrows – axo-somatic synaptic contacts. Scale bar − 5.5 μm.
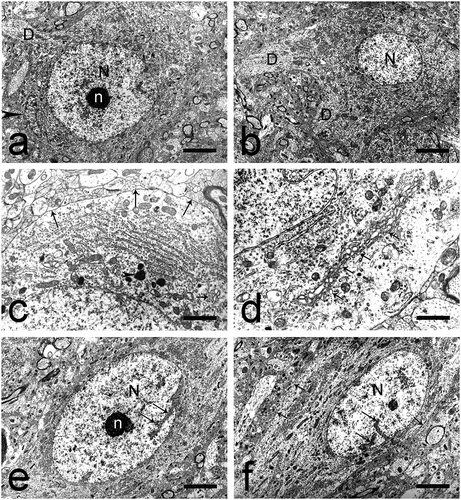
The cytoplasm was rich in cellular organelles. This abundance of cellular organelles gave the cytoplasm a higher electron density, in contrast to the electron-lucent nucleus. The granular endoplasmic reticulum was well represented and formed typical Nissl bodies in close proximity to the nuclear membrane. In the periphery of the cytoplasm we noted multiple free ribosomes and a small number of polysomes (). Mitochondria were particularly abundant and had a spherical shape and size 0.2–0.4 µm. Microtubules were also observed, mainly at the points of emerging of the cellular outgrowths. The Golgi complex was well developed, situated around the nucleus with cisterns with smooth wall of various length, width and number and small lucent vesicles (). Lysosomes and multivesicular bodies were sometimes noted around the units of the Golgi complex. Lysosomes presented as electron-dense bodies of different shape and size of approximately 0.2–0.3 µm. Submembranous cisterns were often observed, oriented parallel to the plasmalemma, with different number and length; some of them were fenestrated. Numerous axo-somatic synapses were described on the cellular surface of this type of neurons.
Fusiform large neurons
Fusiform neurons were an often recognized type and had longitudinal size of 18–22 µm and transverse size of 13–15 µm (). The nucleus was large, electron-lucent and oval-shaped and located in the middle of the cell. It was surrounded by a wide cytoplasmic ring. The nuclear membrane had an arch-shaped course and formed thin and shallow invaginations towards the karyoplasm. Euchromatin was the predominant type. Heterochromatin deposits were observed inside the karyoplasm and in small patches under the nuclear membrane. Accumulations of nucleolus-associated chromatin were also reported. The nucleolus was centrally located, with a reticular pattern and presence of FC.
The cytoplasm exhibited high electron density due to the presence of a multitude of cellular organelles. The granular endoplasmic reticulum was well represented and formed typical Nissl bodies. Free ribosomes and polysomes were particularly abundant. Mitochondria were uniformly dispersed within the cytoplasm and had a spherical shape and size 0.2–0.3 µm. We also noted a few elongated mitochondria with size 0.4–0.6 µm. The Golgi complex was represented by short smooth cisterns and vacuoles, surrounded by small electron-lucent vesicles. Microtubules, multivesicular bodies and lipofuscin granules were also described. A large number of axonal endings were noted on the cellular surface, most often participating in the formation of asymmetrical axo-somatic synapses ().
Cylindrical large neurons
These neurons had longitudinal size of 38–40 µm and transverse size of 12–16 µm (). The nucleus was large and electron-lucent and had a markedly elongated shape. It was observed in the middle of the cell and was surrounded by a wide cytoplasmic ring. The nuclear membrane arched at the two poles of the nucleus. Deep invaginations of its two membranes were described, which gave the nucleus a lobulated appearance (). The nuclear pores were uniformly arranged. Chromatin was finely dispersed.
Fig. 3. Cylindrical large neurons and large neurons of irregular shape in the dorsal claustrum in cat. (a) Cylindrical large neuron with a bean-shaped nucleus (N). The nuclear membrane forms deep invaginations (long black arrows), giving the nucleus a lobulated appearance. Scale bar − 5.5 μm. (b) Cylindrical large neuron containing a typical nucleolus-resembling structure (NucRS), recognized as a nematosome. Typical Nissl bodies are also seen. Scale bar − 1.2 μm. (c) Cylindrical large neuron containing a nematosome with an electron-dense central portion and electron-lucent peripheral portion. Long black arrows – axo-somatic synaptic contacts. Scale bar − 1.3 μm. (d) A special type of nematosome with an electron-lucent central portion built of amorphous material and a large number of filaments. Scale bar − 1.1 μm. (e) Large neuron of irregular shape. The nuclear membrane forms deep invaginations (long black arrows), giving the nucleus (N) a lobulated appearance. Black arrowhead – glial cell. Scale bar − 5.5 μm.
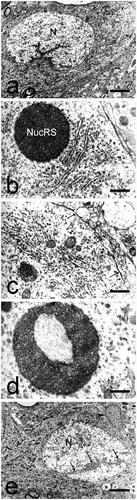
The cytoplasm was well represented but contained a relatively poor number of cellular organelles. The granular endoplasmic reticulum was represented by a small number of distended or flattened cisterns and we did not observe their typical arrangement in Nissl bodies. Mitochondria were relatively few in number. The Golgi complex was abundant and located close to the nuclear membrane. Microtubules, electron-dense bodies and lipofuscin granules of various shapes were noted. Nematosomes were seen in the cytoplasm of all cylindrical neurons. They had an oval shape and a mean diameter of 0.7–1.1 µm but lacked a limiting membrane (). They were built of densely packed granules and fine filaments. Often, free ribosomes were associated with these structures. These were similar to the pars fibrosa of the nucleolus but the number, size and distribution of the granules was different (larger granules formed an electron-dense portion, while smaller ones formed an electron-lucent portion) ()). In other nematosomes, we observed an electron-lucent central portion built of amorphous material and a large number of filaments ()).
Large neurons of irregular shape
These neurons exhibited many various shapes and sizes (). The shape of the nucleus was not strictly defined. The two layers of the nuclear membrane formed invaginations of various depths. Some of them were particularly deep and gave the nucleus a lobulated appearance. Finely dispersed chromatin was the predominant type ().
An abundance of cellular organelles was noted in the cytoplasm. The granular endoplasmic reticulum was represented by typical Nissl bodies. The number of mitochondria was moderate. Their shape was either spherical or elongated. A scarce number of cisterns of the Golgi complex were noted. Electron-dense bodies of spherical shape, numerous microtubules and neurofilaments were described. Asymmetrical axo-somatic synapses on the cellular surface of these neurons were rarely seen.
Medium-sized neurons
The size of medium-sized neurons varied between 16 and 21 µm. We sub-divided these neurons into two types based on their shape: elliptical neurons and neurons of irregularly elongated shape.
Elliptical medium-sized neurons
These were the more often observed of the two types of medium-sized neurons (). Their nucleus was large and electron-lucent, centrally or slightly eccentrically located. The nuclear membrane had a uniform arch-shaped course and formed shallow invaginations towards the karyoplasm, filled with polysomes. Euchromatin was the predominant type. The nucleolus was centrally located and had a reticular structure.
Fig. 4. Medium-sized neurons in the dorsal claustrum in cat. (a) Medium-sized neuron of elliptical shape with an elongated electron-lucent nucleus (N) and a centrally located nucleolus (n) with reticular structure. Scale bar − 3 μm. (b) Cilium in a medium-sized elliptical neuron (black arrowheads). Scale bar − 1.4 μm. (c) Cilium in a medium-sized elliptical neuron (black arrowheads). Scale bar − 1.6 μm. (d) Medium-sized neuron of irregular shape with a large, electron-lucent nucleus (N). The nuclear membrane forms wide shallow invaginations (long black arrows). The nucleolus (n) is relatively large, eccentrically located, with a reticular structure. Scale bar − 4 μm.
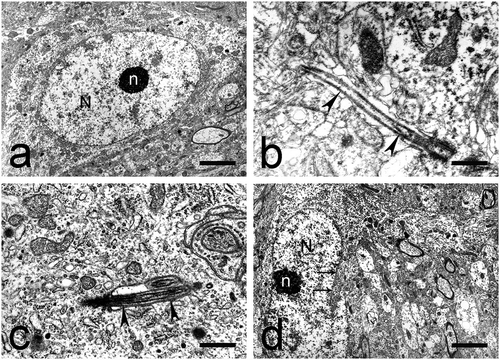
The cytoplasm formed a relatively narrow ring around the nucleus. There was a moderate number of cellular organelles. The granular endoplasmic reticulum consisted of small Nissl bodies. Ribosomes were particularly prominent. We noted a moderate number of round or slightly elongated mitochondria. The Golgi complex was represented by a small number of separate units. A moderate number of microtubules were noted, located most often parallel to the nucleus. Part of them continued their course towards the outgrowths ()). Axo-somatic synapses on the cellular surface of these neurons were not seen. Often, we reported the presence of cilia protruding from the cellular surface ().
Medium-sized neurons of irregular shape
The size of these neurons varied between 16 and 18 µm (). Their nucleus was large, had an irregular shape and occupied almost the entire body of the cell. The nuclear membrane formed shallow invaginations, filled with granular endoplasmic reticulum. Chromatin was uniformly dispersed; we noted the presence of accumulation of heterochromatin, which gave the nucleus a spotted appearance. The nucleolus was relatively large, eccentrically located and had a reticular structure.
The cytoplasm was scarce and poor in cellular organelles. A relatively larger number of them were situated at the point of emerging of the dendrites. The granular endoplasmic reticulum was represented by diffusely situated, short, non-branching cisterns. Small Nissl bodies were rarely seen. Free ribosomes and polysomes were described in between those structures. The number of mitochondria and lysosomes was moderate. Few microtubules and a small number of units of Golgi complex were observed. The surface of this type of neurons was covered by a relatively small number of terminal boutons.
Small neurons
The size of small neurons varied between 10 and 16 µm. We sub-divided these neurons into three types based on their shape: round neurons, elliptical neurons and elongated neurons.
Round small neurons
Most often, this type of neurons had size 12–14 µm (). They had a spherical, centrally located nucleus. The nuclear membrane had a non-uniform arch-shaped course and formed deep invaginations, which gave the nucleus a highly lobulated appearance. Condensed chromatin was the predominant type. It was located below the nuclear membrane (submembrane-associated chromatin) and among the karyoplasm, in the perinucleolar zones. A typical, oval-shaped, eccentrically located nucleolus was observed. Small electron-dense zones were seen within the nucleolus (nucleolar interstitium). FC were especially prominent. The nucleolus-associated chromatin was present in the form of large accumulations (0.1–0.3 µm) (). Rod-shaped intranuclear inclusions were seen in the nuclei of some cells (). Some of these rods consisted of fine granular material in one end and of parallelly positioned fine fibrils in the other (). Other rods were built of bundles of filaments which traversed almost the entire nucleus ()). A third type of rods exhibited a crystal-like structure ().
Fig. 5. Round and elliptical small neurons in the dorsal claustrum in cat. (a) Round small neuron with a large, electron-lucent nucleus (N). The nuclear membrane forms deep invaginations (long black arrows). The nucleolus (n) is eccentrically located, with prominent fibrillar centers. A rod-shaped intranuclear inclusion (black arrowhead) is also seen. Short black arrow – electron-dense inclusion in the cytoplasm. Scale bar − 4.5 μm. (b) Rod-shaped intranuclear inclusion (black arrowheads) built of fine granular material and parallel fine fibrils. Scale bar − 1.3 μm. (c) Rod-shaped intranuclear inclusions (black arrowheads) built of bundles of filaments. N – nucleus. Scale bar − 4.5 μm. (d) Rod-shaped intranuclear inclusion (black arrowheads) with a crystal-like structure. Scale bar − 1.3 μm. (e) Round small neuron with a large, electron-lucent nucleus (N) and a centrally located nucleolus (n). The nuclear membrane forms deep invaginations (long black arrows). Tight junctions (short black arrows) are seen between two neurons and one neuron and one glial cell (black arrowhead). Scale bar − 4.5 μm. (f) Elliptical small neuron with an oval-shaped, electron-lucent nucleus (N). The nuclear membrane forms a small number of invaginations (long black arrows). The nucleolus (n) is centrally located, has an oval shape and a compact structure. Short black arrows – tight junctions. Scale bar − 3 μm.
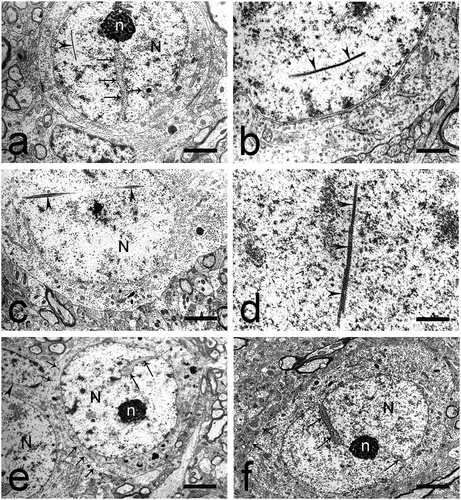
The cytoplasm was represented by a thin cytoplasmic ring and contained scarce organelles. The granular endoplasmic reticulum was described as short, poorly branching cisterns, covered by ribosomes. Small typical Nissl bodies were seen in some cells. Polysomal chains were rarely observed, most often surrounding the nucleus. The mitochondria were few in number. Their length was between 0.1 and 0.2 µm. The Golgi complex was represented by parallelly arranged arch-shaped cisterns and a small number of vesicles with smooth walls. Few lysosomes were also seen, with size 0.1–0.4 µm, as well as a small number of microtubules and neurofilaments.
A characteristic feature of these cells was the presence of submembranous cisterns (). The external surface of the cellular membrane almost always contacted with the membrane of a glial cell or with the membrane of other neurons of the same type. Tight junctions were described at numerous sites between the cellular membranes (). Axo-somatic contacts were not seen on the membranes of these neurons.
Elliptical small neurons
The size of these neurons varied between 10 and 11 µm (). They had an oval-shaped, electron-lucent, centrally located nucleus. The nuclear membrane formed a small number of invaginations, filled with the cisterns of the granular endoplasmic reticulum. Chromatin was diffusely distributed within the karyoplasm. Small accumulations of condensed chromatin were also noted. The nucleolus was centrally located and had an oval shape. Its structure was more compact as opposed to the other types of small neurons.
The cytoplasm formed a narrow cytoplasmic ring and exhibited high electron density due to the relative abundance of organelles. The granular endoplasmic reticulum formed small typical Nissl bodies. Numerous free ribosomes and polysomes were also seen. The mitochondria were spherical or slightly elongated and were particularly abundant. Separate units of Golgi complex were seen surrounding the nucleus. Lysosomes were very few. A small number of terminal boutons, forming axo-somatic synapses, terminated on the surface of this type of cells.
Elongated small neurons
This was the most rarely observed type of small neurons, with size approximately 10 µm (). They had a large, electron-lucent, slightly eccentrically located nucleus. Chromatin was finely dispersed within the karyoplasm. Nucleolus-associated chromatin was also described. On some sections, heterochromatin was noted among the elements of the nucleolonemma.
Fig. 6. Elongated small neurons and neurogliaform (‘dwarf’) cells in the dorsal claustrum in cat. (a) Elongated small neuron with a large, electron-lucent nucleus (N). The nuclear membrane forms various invaginations (long black arrow). The nucleolus (n) is centrally located with prominent nucleolus-associated chromatin. The Golgi complex is represented by small cisterns and electron-lucent vesicles (short black arrow). Black arrowhead – cilium protruding from the cellular surface. Scale bar − 4.5 μm. (b) Elongated small neuron with a large, electron-lucent nucleus (N). The nuclear membrane forms extensive invaginations which form several branches (long black arrows). The nucleolus (n) is centrally located with prominent nucleolus-associated chromatin. Scale bar − 4.5 μm. (c) Terminal boutons, forming symmetrical axo-somatic synaptic contacts (long black arrows). N – nucleus; n – nucleolus. Scale bar − 1.3 μm. (d) Two neurogliaform (‘dwarf’) cells with electron-lucent nuclei (N) and centrally located nucleoli (n) with reticular structure. Scale bar − 5.5 μm. (e) Neurogliaform (‘dwarf’) cell with an irregularly-shaped nucleus (N) owing to the numerous invaginations of the nuclear membrane (long black arrows). n – nucleolus. Scale bar − 4.5 μm. (f) Axo-somatic synaptic contact on the surface of a neurogliaform (‘dwarf’) cell (short black arrow). N – nucleus. Scale bar − 1.6 μm.
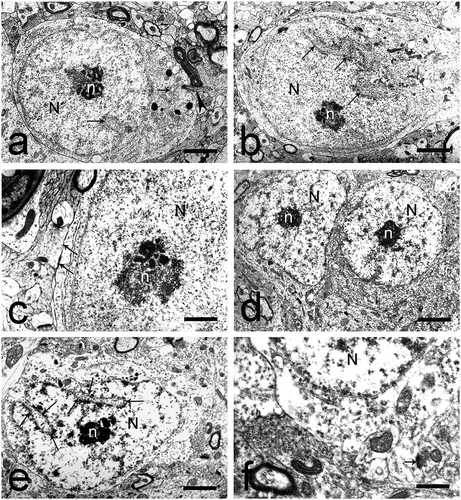
The electron-lucent cytoplasm formed a narrow cytoplasmic ring and was poor in cellular organelles. The granular endoplasmic reticulum formed small Nissl bodies and was seen as short, poorly-branching cisterns, covered by ribosomes. Free ribosomes and polysomes were few in number. There were a small number of mitochondria, among which we noted a few large lysosomes. Microtubules were very few. The Golgi complex was poorly developed. Cilia were often seen protruding from the surface of this type of neurons (). Terminal boutons, forming symmetrical synaptic contacts were noted on the surface of these cells ().
Small (neurogliaform) cells or ‘dwarf’ cells
These were the smallest type of cells observed in the dorsal claustrum of the cat. They had an oval body and size 5–10 µm (). Their nucleus had various shape – crescent, oval, very often irregular (). The nuclear membrane formed numerous invaginations of various depth. Condensed chromatin was the predominant type and was seen in the form of accumulations under the nuclear membrane and around the nucleolus. The nucleolus was relatively large.
The cytoplasm formed a narrow ring around the nucleus and was poor in cellular organelles. The granular endoplasmic reticulum was described as short cisterns, among which we noted free ribosomes and polysomes. There were a few spherical or slightly elongated mitochondria. Lysosomes of various shape were also noted. We saw as many as two separate units of Golgi complex in each neuron, surrounded by small vesicles. Axo-somatic synapses were described terminating on the surface of this type of cells ().
Discussion
In the present study, we studied the neurons of the dorsal claustrum and classified them into three groups based on their size and shape. Based on their ultrastructural features, we were able to deduce that large and medium-sized neurons most often represent projection or ‘relay’ neurons, which have a high metabolic activity and synthetic function and send efferent information from the claustrum. Small neurons and ‘dwarf’ cells, on the other hand, exhibited the structural features of inhibitory interneurons, with low metabolic activity and synthetic function. These data further support and supplement the findings acquired through the combined method of Fairen in the dorsal claustrum of cats in our previous studies (Citation32).
In our ultrastructural study we observed and described the characteristic features of the cell nuclei and the type and distribution of the cellular organelles. An interesting ultrastructural observation was the presence of nematosomes. This structure was first described by Le Beux (Citation34) in the substantia nigra of the cat and in some rat neurons. Later, nematosomes were described in the cerebral cortex, cerebellum, trigeminal ganglia, spinal ganglia, etc. (Citation35). Nematosomes are nucleolus-like structures, found in neural and non-neural tissues whose function is not entirely clear (Citation36). Many theories have been proposed regarding the function of nematosomes, including a site of RNA-storage (a ‘cytoplasmic nucleolus’) or an inactive microtubule-organizing center (Citation35). In our study, these structures were described in cylindrical large neurons, associated with a number of free ribosomes. This seems to confirm the hypothesis that they are indeed an RNA-storage site. However, taking into account the rich cytoskeleton in these cells, they could yet play a role in the turnover of components of the cytoskeleton as the so-called microtubule-organizing center (Citation35). Further proof in this direction could be the study of Ramer et al. (Citation36) who suggested that nematosomes are built of filamentous actin.
In the present study, we described primary cilia protruding from the surface of both projection neurons and interneurons. They are present in most brain cells, including neurons, neural stem cells and astrocytes (Citation37). The primary cilium is thought to act as an antenna accepting information from the extracellular space in the form of various signals and then transmitting them into the cell (Citation38). So far, neural cilia have been reported in various regions of the brain, including the spinal cord (Citation39), lateral geniculate nucleus (Citation40), ventral tegmental area (Citation41), cerebellum (Citation42), striatum (Citation43), hypothalamus (Citation44), retinal neurons (Citation45) and neocortex (Citation46), among others. Herein, we report for the first time the presence of primary cilia on the surface of medium-sized claustral neurons, which are normally recognized as efferent neurons, as well as on small neurons, which are considered to be interneurons. This observation confirms that claustral neurons most likely integrate numerous signals from the extracellular space, together with the input which they receive from other brain structures, which is yet another testament to their complex function.
Kubasik-Juraniec et al. (Citation31) described three types of neurons in the dorsal claustrum of the cat. Large and medium-sized cells formed three quarters of the cell population and were fairly similar in their ultrastructure. The shape of their perikaryon was described as oval, round, fusiform or triangular. The cytoplasm of these cells was reported to be rich in cellular organelles and rough endoplasmic reticulum in particular. The proximal dendrites of medium-sized cells were short and relatively wide at the base, while those originating from the large cells were often thick and with a short base. Both types of neurons possessed spines on their distal dendrites. These neurons are similar to the large and medium-sized neurons found in the present study. The third type of neurons were small cells with large nuclei surrounded by a relatively thin cytoplasmic ring, which was not rich in cellular organelles. In most cases, the cellular organelles were represented by free ribosomes and mitochondria. The dendrites originating from that type of neurons were long and thin. The authors considered these cells to be intrinsic claustral neurons. These neurons can be compared to the small neurons described in the present study.
In addition to electron microscopy, some recent studies have used immunohistochemistry to identify cell types depending on the expression of different proteins (Citation47). Immunohistochemistry can provide additional information about the internal circuits in the claustrum by revealing the neurotransmitters used by claustral neurons (Citation47). Such studies have revealed the presence of neurons positive for parvalbumin, calretinin, calbindin, neuronal nitric oxide synthase (nNOS) and other substances (Citation24,Citation48). Despite having some characteristic features of large spiny neurons, it has been suggested that these cells are in fact interneurons (Citation48). In addition, the spatial distribution of claustral neurons as assessed by immunohistochemical studies appears to be rather homogenous. In contrast, studies on the rodent claustrum have revealed a clear distinction between parvalbumin- and calbindin-immunoreactive neurons (Citation49). The former are observed predominantly in the dorsal claustrum, while the latter are located in the ventral claustrum. Calretinin-positive neurons, on the other hand, are diffusely distributed in the dorsal and ventral claustrum of the rat (Citation50). In addition, some neurotransmitters, such as GABA, have been described in both cat and rodents (Citation51). This shows that claustral neurons from different mammals may participate in diverse local circuits.
In recent years, neurogliaform cells or ‘dwarf cells’ have been recognized as a type of local GABA-ergic inhibitory interneurons that have been described in the neocortex, striatum, hippocampus, habenula of the thalamus and others (Citation52–Citation55). Herein, we also describe this type of cells in the dorsal claustrum of cats. Armstrong et al. (Citation54) claimed that despite their wide distribution, the main characteristic features of neurogliaform cells in the different parts of the brain are identical, which allowed us to draw comparisons between the ‘dwarf’ cells described in our study and cells of the same family in other neural structures. Despite their small size, neurogliaform cells have numerous stellate dendrites and an extensively branching axonal arbor which forms a dense plexus around the body of the cell (Citation52,Citation56). Apart from GABA, these cells have been associated with production and expression of neuropeptide Y, alpha-actinin-2 and neuronal nitric oxide synthase (Citation52,Citation54,Citation56). Jackson et al. (Citation18) described neuropeptide Y-positive neurofibrillar cells in the claustrum, responsible for a long-lasting inhibition of the prefrontal cortex from the claustrum. Our observations on their ultrastructural features confirmed that they are indeed interneurons. We hypothesized that ‘dwarf’ cells are most probably responsible for inter-regulation at the level of the claustrum by synchronizing neuronal oscillatory activity. However, this does not exclude the hypothesis of Jackson et al. (Citation18); it could be possible that neurofibrillar cells in the claustrum realize their inhibitory effect on the prefrontal cortex by first affecting the activity of the large and medium-sized efferent claustral neurons.
The dorsal claustrum and its subdivisions are incorporated in a number of sensory chains inside the central nervous system. The visual claustrum in the cat receives input from several zones in the visual cortex (areas 17, 18 and 19 and the lateral suprasylvian visual area) and sends information back to the same areas (Citation4,Citation25,Citation57–Citation59). It is organized retinotopically (Citation28). A large part of the claustral neurons are binocular (i.e. receive information from both eyes) and are sensitive to elongated stimuli, moving at a moderate speed (Citation4,Citation25). They are selective to some of the features of those stimuli, such as orientation, but indifferent to others, such as direction of motion (Citation25). The cortical neurons which project to the visual claustrum, on the other hand, tend to be monocular (i.e. receive input from only one eye) and are direction-sensitive (Citation25,Citation60). The auditory zone of the claustrum is organized topographically and projects to the primary auditory cortex while receiving input from the higher-order associative auditory cortex (Citation4,Citation25,Citation61). The cells of this zone prefer long latency stimuli from either ear and have broad tuning with marked preference for noisy stimuli (Citation4,Citation58,Citation61). Goll et al. (Citation4) also proposed that these characteristic traits of the visual and auditory claustrum likely mean that it is responsible for registering the sudden appearance of surprising stimuli. The somatosensory zone of the cat claustrum is connected with the primary somatosensory cortex, the primary motor cortex and the parietal motor cortex (Citation11,Citation25,Citation58,Citation62). The biggest representation area in the somatosensory zone is that of the foreleg at the expense of the facial area which is significantly smaller (Citation4,Citation25,Citation62).
Conclusion
In the present study, we observed and described in detail the characteristic traits of four different types of neurons found in the dorsal claustrum of the cat – large, medium-sized, small and ‘dwarf’ cells. Based on their ultrastructural features, we recognized large and medium-sized neurons as efferent, or projection neurons. They are the main anatomical substrate of the claustrocortical circuit and are densely connected with nearly all areas of the cortex, including the visual, auditory, somatosensory and prefrontal cortex. Small neurons and neurogliaform ‘dwarf’ cells bear the typical ultrastructure of inhibitory interneurons which are responsible for the local circuit organization at the level of the claustrum. In addition, neurogliaform cells may initiate long-lasting inhibition of the prefrontal cortex by modulating the function of efferent claustral neurons. The present manuscript shares new details about subcellular structures such as the nematosomes and the primary cilia, which are described for the first time in claustral neurons.
Disclosure statement
No potential conflict of interest was reported by the authors.
References
- Brown SP, Mathur BN, Olsen SR, et al. New breakthroughs in understanding the role of functional interactions between the neocortex and the claustrum. J Neurosci. 2017;37(45):1–12.
- Crick FC, Koch C. What is the function of the claustrum? Philos Trans R Soc Lond B Biol Sci. 2005;360(1458):1271–1279.
- Edelstein LR, Denaro FJ. The claustrum: a historical review of its anatomy, physiology, cytochemistry and functional significance. Cell Mol Biol. 2004;50:675–702.
- Goll Y, Atlan G, Citri A. Attention: the claustrum. Trends Neurosci. 2015;38(8):486–495.
- Hinova-Palova DV, Edelstein L, Landzhov BV, et al. Parvalbumin-immunoreactive neurons in the human claustrum. Brain Struct Funct. 2014a;219(5):1813–1830.
- Pirone A, Cozzi B, Edelstein L, et al. Topography of Gng2- and NetrinG2-expression suggests an insular origin of the human claustrum. PLoS One. 2012;7(9):e44745.
- Hinova-Palova DV, Edelstein L, Landzhov B, et al. Topographical distribution and morphology of NADPH-diaphorase-stained neurons in the human claustrum. Front Syst Neurosci. 2014b;8:96.
- Johnson JI, Fenske BA, Jaswa AS, et al. Exploitation of puddles for breakthroughs in claustrum research. Front Syst Neurosci. 2014;8:78.
- Landzhov B, Hinova-Palova D, Edelstein L, et al. Comparative investigation of neuronal nitric oxide synthase immunoreactivity in rat and human claustrum. J Chem Neuroanat. 2017;86:1–14.
- Torgerson CM, Irimia A, Goh SY, et al. The DTI connectivity of the human claustrum. Hum Brain Mapp. 2015;36(3):827–838.
- Clascá F, Avendaño C, Román-Guindo A, et al. Innervation from the claustrum of the frontal association and motor areas: axonal transport studies in the cat. J Comp Neurol. 1992;326(3):402–422.
- Day-Brown JD, Slusarczyk AS, Zhou N, et al. Synaptic organization of striate cortex projections in the tree shrew: A comparison of the claustrum and dorsal thalamus. J Comp Neurol. 2017;525(6):1403–1420.
- Dinopoulos A, Papadopoulos GC, Michaloudi H, et al. Claustrum in the hedgehog (Erinaceus europaeus) brain: cytoarchitecture and connections with cortical and subcortical structures. J Comp Neurol. 1992;316(2):187–205.
- Mathur BN. The claustrum in review. Fron Syst Neurosci. 2014;8:48.
- Usunoff K, Schmitt O, Lazarov N, et al. Efferent projections of the claustrum to the dorsal and ventral striatum, substantia nigra, ventral tegmental area and parabrachial nuclei. Retrograde tracing study in rat. Compt Rend Acad Buld Sci. 2008;61(6):817–825.
- Vertes RP, Hoover WB. Projections of the paraventricular and paratenial nuclei of the dorsal midline thalamus in the rat. J Comp Neurol. 2008;508(2):212–237.
- Agster KL, Tomás Pereira I, Saddoris MP, et al. Subcortical connections of the perirhinal, postrhinal, and entorhinal cortices of the rat. II. efferents. Hippocampus. 2016;26(9):1213–1230.
- Jackson J, Kamani M, Zemelman B, et al. Inhibitory control of prefrontal cortex by the claustrum. Neuron. 2018;99:1029–1039.
- Smith JB, Alloway KD. Functional specificity of claustrum connections in the rat: interhemispheric communication between specific parts of motor cortex. J Neurosci. 2010;30(50):16832–16844.
- Smith JB, Alloway KD. Interhemispheric claustral circuits coordinate sensory and motor cortical areas that regulate exploratory behaviors. Front Syst Neurosci. 2014;8:93.
- Kitanishi T, Matsuo N. Organization of the claustrum-to-entorhinal cortical connection in mice. J Neurosci. 2017;37(2):269–280.
- Tomás Pereira I, Agster KL, Burwell RD. Subcortical connections of the perirhinal, postrhinal, and entorhinal cortices of the rat. I. afferents. Hippocampus. 2016;26(9):1189–1212.
- Watson GDR, Smith JB, Alloway KD. Interhemispheric connections between the infralimbic and entorhinal cortices: the endopiriform nucleus has limbic connections that parallel the sensory and motor connections of the claustrum. J Comp Neurol. 2017;525(6):1363–1380.
- Hinova-Palova DV, Edelstein LR, Paloff AM, et al. Parvalbumin in the cat claustrum: ultrastructure, distribution and functional implications. Acta Histochem. 2007;109(1):61–77.
- Smythies J, Edelstein L, Ramachandran V, editors. The claustrum. Structural, functional and clinical neuroscience. Cambridge, Massachusetts: Academic Press; 2014.
- Buchanan KJ, Johnson JI. Diversity of spatial relationships of the claustrum and insula in branches of the mammalian radiation. Ann N Y Acad Sci. 2011;1225(S1):E30–E63.
- Mamos L, Narkiewicz O, Moryś J. Neurons of the claustrum in the cat; a Golgi study. Acta Neurobiol Exp (Wars). 1986;46(4):171–178.
- LeVay S, Sherk H. The visual claustrum of the cat. I. Structure and connections. J Neurosci. 1981;1(9):956–980.
- Brand S. A serial section Golgi analysis of the primate claustrum. Anat Embryol (Berl). 1981;162(4):475–488.
- Norita M, Hirata Y. Some electron microscope findings of the claustrum of the cat. Arch Histol Jpn. 1976;39(1):33–49.
- Kubasik-Juraniec J, Dziewiatkowski J, Moryś J, et al. Ultrastructural organization of the visual zone in the claustrum of the cat. Folia Morphol (Warsz). 1998;57(4):287–299.
- Hinova-Palova D, Iliev A, Edelstein L, et al. Electron microscopic study of Golgi-impregnated and gold-toned neurons and fibers in the claustrum of the cat. J Mol Histol. 2018 (in press).
- Palay SL, McGee-Russell SM, Gordon S, et al. Fixation of neural tissues for electron microscopy by perfusion with solutions of osmium tetroxide. J Cell Biol. 1962;12(2):385–410.
- Le Beux YJ. An ultrastructural study of a cytoplasmic filamentous body, termed nematosome, in the neurons of the rat and cat substantia nigra. The association of nematosomes with the other cytoplasmic organelles in the neuron. Z Zellforsch Mikrosk Anat. 1972;133(3):289–325.
- Ockleford CD, Nevard CH, Indans I, et al. Structure and function of the nematosome. J Cell Sci. 1987;87:27–44.
- Ramer MS, Cruz Cabrera MA, Alan N, et al. A new organellar complex in rat sympathetic neurons. PLoS ONE. 2010;5(5):e1000872.
- Lee JE, Gleeson JG. Cilia in the nervous system: linking cilia function and neurodevelopmental disorders. Curr Opin Neurol. 2011;24(2):98–105.
- Green JA, Mykytyn K. Neuronal primary cilia: an underappreciated signaling and sensory organelle in the brain. Neuropsychopharmacology. 2014;39(1):244–245.
- Duncan D, Williams V, Morales R. Centrioles and cilia-like structures in spinal gray matter. Tex Rep Biol Med. 1963;21:185–187.
- Karlsson U. Three-dimensional studies of neurons in the lateral geniculate nucleus of the rat. I. Organelle organization in the perikaryon and its proximal branches. J Ultrastruct Res. 1966;16(5):429–481.
- Bayer VE, Pickel VM. Ultrastructural localization of tyrosine hydroxylase in the rat ventral tegmental area: relationship between immunolabeling density and neuronal associations. J Neurosci. 1990;10(9):2996–3013.
- Del Cerro MP, Snider RS. The Purkinje cell cilium. Anat Rec. 1969;165(2):127–130.
- Wolfrum G, Nitsch C. High frequency of ciliated neuropeptide Y immunoreactive neurons in rat striatum. Cell Tissue Res. 1992;267(1):199–202.
- Vigh-Teichmann I, Vigh B, Aros B. Ciliated perikarya, “peptidergic” synapses and supraependymal structures in the guinea pig hypothalamus. Acta Biol Acad Sci Hung. 1980;31(1–3):373–394.
- Boycott BB, Hopkins JM. A neurofibrillar method stains solitary (primary) cilia in the mammalian retina: their distribution and age-related changes. J Cell Sci. 1984;66:95–118.
- Mandl L, Megele R. Primary cilia in normal human neocortical neurons. Z Mikrosk Anat Forsch. 1989;103(3):425–430.
- Baizer JS. The neurochemical organization of the claustrum. In: Smythies JR, Edelstein LR, Ramachandran VS, editors. The Claustrum. Structural, functional and clinical neuroscience. Cambridge, Massachusetts: Academic Press; 2014. p. 85–118.
- Rahman FE, Baizer JS. Neurochemically defined cell types in the claustrum of the cat. Brain Res. 2007;1159:94–111.
- Druga R, Chen S, Bentivoglio M. Parvalbumin and calbindin in the rat claustrum: an immunocytochemical study combined with retrograde tracing frontoparietal cortex. J Chem Neuroanat. 1993 Nov-Dec;6(6):399–406.
- Druga R, Salaj M, Barinka F, et al. Calretinin immunoreactivity in the claustrum of the rat. Front Neuroanat. 2015;8:160.
- Narkiewicz O, Nitecka L, Mamos L, et al. The pattern of the GABA-like immunoreactivity in the claustrum. Folia Morphol (Warsz). 1988;47(1–4):21–30.
- Overstreet-Wadiche L, McBain CJ. Neurogliaform cells in cortical circuits. Nat Rev Neurosci. 2015;16(8):458–468.
- Ibáñez-Sandoval O, Tecuapetla F, Unal B, et al. A novel functionally distinct subtype of striatal neuropeptide Y interneuron. J Neurosci. 2011;31(46):16757–16769.
- Armstrong C, Krook-Magnuson E, Soltesz I. Neurogliaform and ivy cells: a major family of nNOS expressing GABAergic neurons. Front Neural Circuits. 2012;6:23.
- Weiss T, Veh RW. Morphological and electrophysiological characteristics of neurons within identified subnuclei of the lateral habenula in rat brain slices. Neurosci. 2011;172:74–93.
- Price CJ, Cauli B, Kovacs ER, et al. Neurogliaform neurons form a novel inhibitory network in the hippocampal CA1 area. J Neurosci. 2005;25(29):6775–6786.
- Jayaraman A, Updyke BV. Organization of visual cortical projections to the claustrum in the cat. Brain Res. 1979;178(1):107–115.
- Olson CR, Graybiel AM. Sensory maps in the claustrum of the cat. Nature. 1980;288(5790):479–481.
- Squatrito S, Battaglini PP, Galletti C, et al. Autoradiographic evidence for projections from cortical visual areas 17, 18, 19 and the Clare-Bishop area to the ipsilateral claustrum in the cat. Neurosci Lett. 1980;19(3):265–269.
- Sherk H, LeVay S. Visual claustrum: topography and receptive field properties in the cat. Science. 1981;212(4490):87–89.
- Beneyto M, Prieto JJ. Connections of the auditory cortex with the claustrum and the endopiriform nucleus in the cat. Brain Res Bull. 2001;54(5):485–498.
- Minciacchi D, Granato A, Antonini A, et al. Mapping subcortical extrarelay afferents onto primary somatosensory and visual areas in cats. J Comp Neurol. 1995;362(1):46–70.