Abstract
The European Renewable Energy Directive (RED) was produced by the European Parliament and the Council of the European Union to promote the uptake of energy from renewable resources by participating member states. It sets greenhouse gas (GHG) saving targets for renewable transport fuels and dictates the method by which this should be calculated. This study examines the life cycle assessment (LCA) methodology set out in the RED and compares it to attributional and consequential assessments. A case study of wheat-based bioethanol is used to illustrate how differences in methodology affect the GHG emission results. The results show that GHG emissions from wheat bioethanol are lowest when calculated under the RED, and highest when substitution credits are applied. The RED has been shown to not only combine attributional LCA and consequential LCA approaches but also to contain rules for specific co-products. For example, cereal residues are not allocated GHG emissions from cultivation, and exported electricity is awarded a credit based on similar electricity generation from the same source. It is suggested that these rules may be a reaction to concerns over indirect global land use change, and therefore these methodological anomalies in the RED place artificial biases in GHG emission results.
1. Introduction
In order to ensure that biofuels successfully reduce greenhouse gas (GHG) emissions from transport, it is vital that the emissions from the whole life cycle of producing and delivering them are favourable (Black et al., Citation2011). How this is measured is a serious and complex issue, as the emissions from a biofuel supply chain cannot be directly measured but require models to calculate the implications of biofuel production (Aylott, Higson, Evans, Hamer, & Mortimer, Citation2012). It now accepted that biomass is not ‘carbon neutral’ as first thought in renewable energy policies (Adams et al., Citation2013), as its provision requires the consumption of non-renewable resources during cultivation, harvesting, transport and processing (Royal Society, Citation2008). The increasing need to address the impacts of these stages has prompted the development of holistic methods of assessment. Life cycle assessment (LCA) has dominated environmental decision support (von Blottnitz & Curran, Citation2007), and has been used in current policy directives for the regulation of biofuels.
The Renewable Transport Fuel Obligation (RTFO, Bauen, Watson, & Howes, Citation2008) presented the first LCA-based methodology adopted for the regulation of GHG emissions from road transport, though this has now been displaced by the methodology described in the European Commission's Renewable Energy Directive (RED; EC, Citation2009). The RED also introduced GHG emission saving targets for biofuels, placing a minimum saving of 35% before January 2017, 50% after and 60% after January 2018 for installations that start on or after 1 January 2017. There are proposed changes to increase emission saving targets to 60% for plants initiating operation after 2014 (ICCT, Citation2012). The RED methodology aims to harmonize calculations, specifying what should be included in the calculations and how GHG emissions are apportioned between co-products. It is also proposed that these criteria and the calculation methodology will also apply to solid biomass fuels (Hagberg, Saarnholm, Gode, Ekvall, & Rydberg, Citation2009). A number of studies adopting the RED methodology have failed to note these rules in their entirety (Whittaker, Citation2013). For example, Menichetti and Otto (Citation2009) and Whitaker, Ludley, Rowe, Taylor, and Howard (Citation2010) mention that the RED allocates according to energy content; however in the rules this is only true for specific co-products. Other studies fail to adhere to the systems boundaries of the RED methodology by including extra emission sources such as farm machinery (Yan & Boies, Citation2013), or account for co-products incorrectly, particularly exported electricity (Martinez-Hernandez et al., Citation2013).
The LCA methodology is described as RED to be ‘partially’ consequential because it combines attributional and consequential LCA (ALCA and CLCA) approaches (Brander, Tipper, Hutchison, & Davis, Citation2009). ALCAs provide information about the direct GHG emissions that are directly attributed to the production and use of a product (Sanchez et al., Citation2012). It is recommended that biofuels are certified according to an ALCA approach because producers have immediate control over the direct emissions they cause (Nuffield Council on Bioethics, Citation2011). Alternatively, CLCAs examine effects of changes in products levels (Sanchez et al., Citation2012). Therefore, the impacts of biofuels are considered over a wider or even global context of producers and consumers (Nuffield Council on Bioethics, Citation2011). This approach is appropriate for policy-makers to assess the impacts of policies (Brander et al., Citation2009) but should not be used in consumption-based accounting as it includes credits for emissions that have not physically occurred (Brander & Wylie, Citation2011). It is important to ensure that the two approaches are not combined; otherwise misleading analyses are performed, with similarly misleading conclusions (Aylott et al., Citation2011). What is misleading, however, depends on the original goal. For scientific analysis there is a requirement to be accurate, whereas in policy, the goal is to be effective in directing the renewable energy sector to effectively reduce GHG emissions.
The aim of this paper is to analyse the LCA methodology applied in the RED GHG reporting guidelines and identify where CLCA and ALCA approaches are combined. The consequences of combining these approaches are discussed using a model case study of first-generation bioethanol production, while assessing the specific calculations methods contained within the RED. Many of the issues identified will also be applicable to second-generation biofuels; however there is a lack of good quality conversion process data and biofuel yields are more uncertain than for first-generation fuels.
2. Methodology
2.1. Case study details
The case study biofuel will be based on an existing example presented in the Biomass Environmental Assessment Tool (BEAT, version 2; AEA Technology & North Energy Associates, Citation2010). This model is used instead of Biograce (Biograce.net, Citation2012) as in BEAT the data sources used are transparent. The two sources show comparable yields of bioethanol and other co-products from one tonne of wheat grain. The final result is presented as ‘one GJ of fuel’.
The systems boundaries of BEAT are shown in . In BEAT, and Biograce, it is assumed that heat and power for hydrolysis, fermentation, distillation and dehydration phases are supplied through a natural-gas-powered combined heat and power (CHP) unit, the properties of which are listed in . As a result of utilizing this plant there is a net excess of 24 kWh/GJ bioethanol. Two scenarios are presented for this, the first with straw removal and the second with straw incorporation. Differences in the carbon dynamics of these two systems are not examined here as they have been elsewhere (Whittaker et al., Citation2014). Nitrous oxide emissions from straw incorporation are also included according to the IPCC (De Klein et al., Citation2006). For consistency with the RED, the manufacture of farm machinery is excluded from the analysis.
Table 1. Properties of the combined heat and power boilers assumed in this study.
Another case study, where wheat straw is used a source of fuel for the CHP plant, is also included. The CHP properties will be based on a specific straw-based CHP used in in a similar study (Punter et al., Citation2004). It is assumed that removing 0.9 tonnes of straw/tonne bioethanol can satisfy the heat and power requirements of the bioethanol plant. This corresponds to 60% of the removal straw yield, based on an average wheat yield of 3.5 tonnes/ha (Nix, Citation2011) and a yield of 2.3 tonnes bioethanol/ha (AEA Technology & North Energy Associates, Citation2010). According to the Phyllis Database (ECN, Citationn.d.), the average lower heating value of straw is 18.11 GJ/tonne. The straw-based CHP boiler would lead to an excess of 39.9 kWh/GJ bioethanol ().
Co-products from the bioethanol supply chain include wheat straw, dry distiller's grain and solubles (DDGS) and electricity. According to the BEAT model, approximately 56 kg of straw and 43 kg of DDGS are produced per GJ bioethanol (26.8 GJ/tonne). The final GHG emissions from the supply chain are attributed to bioethanol according to the following methods (a) under the RED methodology (b) avoiding allocation by using the substitution method, (c) allocating by energy content (d) allocating by economic values. provides the parameters applied during allocation. A substitution credit of 3074 kg CO2 eq./tonne DDGS is assumed based on a substitution ratio of 0.59 tonne soy meal/tonne DDGS and a GHG emission of 5.78 kg CO2 eq./tonne soy meal (Brander & Wylie, Citation2011). The substitution credit for exported electricity is based on grid electricity (0.491 kg CO2 eq./kWh (DEFRA & DECC, Citation2012). The credits awarded to exported electricity under the RED are calculated following the methodology used in Biograce.
Table 2. Allocation parameters assumed in this study.
2.2. The RED calculation methodology
The ISO Standards state the first steps of performing an LCA involve identifying the goal and scope and system boundaries of a study (CEN, Citation2006a). To facilitate this process the RED provides Equation (1) in Part C or Annex V. The equation lists the emission sources that must be accounted for, including crop cultivation, extraction of raw material, transport, processing, final distribution and combustion.
Equation 1. Greenhouse gas emissions from the production and use of transport fuels, biofuels and bioliquids (EC, Citation2009).
where E = total emissions from the use of the fuel, eec = emissions from the extraction or cultivation of raw materials, el = annualized emissions from carbon stock changes caused by land-use change, ep = emissions from processing, etd = emissions from transport and distribution, eu = emissions from the fuel in use, esca = Emission saving from soil carbon accumulation via improved agricultural management, eccr = emission saving from carbon capture and geological storage and eee = emissions saving from excess electricity from cogeneration.
The RED provides supporting documentation listing specific calculation rules that are associated with Equation (1). These are as follows:
2.2.1. Allocation of co-products: except from residues
In note 17, it states that co-products should be allocated by energy content; however in note 18 it specifies that:
Wastes, agricultural crop residues, including straw, bagasse, husks, cobs and nut shells, and residues from processing, including crude glycerine (glycerine that is not refined), shall be considered to have zero life-cycle greenhouse gas emissions up to the process of collection of those materials.
Therefore, in the case study selected, straw is not attributed with GHG emissions from cultivation. The RED does not offer any guidance on what differentiates a co-product from a ‘residue from processing’, though it provides a hint that unrefined glycerine is a waste, suggesting that refined glycerine would be a co-product. Whether this assumption is true or could be extrapolated to other co-products is unclear. This is reported to be an important area of clarification, particularly in biomass systems where co-product and waste definitions cause large differences in the results, such as in pellet (Hagberg et al., Citation2009) and biogas plants (Manninen et al., Citation2013).
2.2.2. Allocation of co-products: the exception of electricity
In the RED exported electricity is treated separately to other co-products. Credits are awarded for exported electricity depending on what that exported electricity was generated from. It states in Annex 5, point 16:
Emission saving from excess electricity from cogeneration shall be taken into account in relation to the excess electricity produced by fuel production systems that use cogeneration except where the fuel used for the cogeneration is a co-product other than an agricultural crop residue.
Therefore, exported electricity credits are not awarded if a co-product, other than a residue, is used for electricity production. In this case electricity credits would be awarded to electricity generated from straw.
Following from previous rule, the methodology requires that credits awarded to exported electricity are as follows: ‘excess electricity shall be taken to be equal to the amount of greenhouse gas that would be emitted when an equal amount of electricity was generated in a power plant using the same fuel as the cogeneration unit’. This means that the exported electricity credit will be based on the emissions from using the same fuel in a power plant to produce electricity. In the case studies used here, this means that when natural gas is used in the CHP plant the credit will be based on natural gas-generated electricity. If straw is used in the CHP plant then the exported electricity credit will be based avoiding dedicated straw-based electricity.
The GHG emissions for natural gas-generated electricity are relatively straightforward to calculate. This value is often used to represent marginal electricity generation, as combined cycle gas turbine (CCGT) plants are relatively cheap and quick to build and start up (DECC, Citation2012), can respond quickly to fluctuating demand and are the most efficient mode of producing electricity (IEA, Citation2010). A typical conversion efficiency for a CCGT plant is 50% (Brown & McLeavey-Reville, Citation2011), though this could reach 64% by 2020 (IEA, Citation2010). The average efficiency is assumed to be 54.5% (North Energy Associates, Citation2010). The electricity credits for this are presented in .
Table 3. Electricity emission factors for natural gas- and straw-based electricity based on the assumptions in Section 2.1.
The Elean power station in Ely is currently the only operational straw-powered power station in the UK. The 38 MW plant consumes 200,000 tonnes/straw/year (EPR, Citation2011). To generate a theoretical estimate for the substitution credit of such electricity, it is assumed that this plant receives all straw via road delivery, with an average round-trip transport distance of 160 km (50 miles, plus return journey). Under the RED calculation rules straw is not allocated upstream of GHG emissions from cultivation, and it has a ‘GHG intensity of production’ of zero. As the RED excludes machinery manufacture (note 1) it is assumed that plant construction is not included. Also, as the RED assumes that combustion of biomass has a GHG emission of zero (note 13), non-GHG combustion emissions are also excluded. Refuting this there are reported non-CO2 emissions of CH4 and N2O from biofuel combustion (Hagberg et al., Citation2009). It is assumed that the conversion efficiency of the power plant is 25%, and 56.7 GJ of natural gas for start-up is required (AEA Technology & North Energy Associates, Citation2010). The GHG emissions from straw-based electricity are also provided in .
3. Results
shows the results from the case study example calculated according to the four methodologies applied in this study. For context, when straw is used the GHG emissions from the processing step are reduced from 14.8 to 2.8 kg CO2/GJ. The net effect of this () in the context of the full life cycle ranges between a saving of 33.9 kg CO2 eq./GJ (substitution vs. straw left onsite) or an increase of 1.6 kg CO2 eq./GJ (energy allocation vs. straw removed). The actual result depends on the upstream emissions from the cultivation stage, which in turn depend on the fate of the straw. The results in show the results for each accounting methodology if straw was removed or incorporated. shows the breakdown of GHG emissions between each stage that is allocated to bioethanol, plus GHG emissions allocated to straw, DDGS and electricity. In the two allocation methods, straw removal means that GHG emissions from cultivation will be proportioned between the grain and straw, and the GHG emissions allocated to bioethanol are reduced. If straw is incorporated into the soil or used as a fuel source within the bioethanol system then allocation does not occur, and the overall emissions from cultivation are entirely attributed to bioethanol. If straw is used as a power source in the plant, then compared to an alternative fate of incorporation there is a benefit of 9.7 kg CO2/GJ (allocation by energy) to 11.8 kg CO2/GJ (allocation by price).
Figure 2. Breakdown of sources of GHG emissions from bioethanol production from wheat according to the LCA methods studied here.
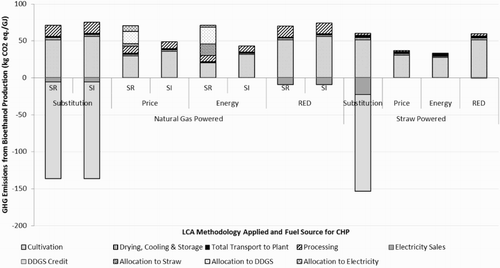
Table 4. Results generated according to the four LCA methodologies examined in this study.
Table 5. Results from the study depending on straw removal or incorporation in the reference system.
Alternatively, if straw was removed for other uses the GHG benefits from diverting it for fuelling the bioethanol plant has very different effects depending on the allocation method used. shows how GHG emissions are allocated to straw differently between allocation by price and energy. When allocation is carried out according to energy content, using straw for a fuel source actually increases the GHG emissions attributed to bioethanol by 1.6 kg CO2 eq./GJ compared to removing it for other uses. This result is due to marginally higher cultivation and transport emissions (). This is not seen when allocating by price due to the bias of emissions towards grain, and a benefit of 5.6 kg CO2/GJ is seen. This does not account for any impacts on competing industries that would otherwise had utilized the same straw.
Much higher GHG savings are achieved in both natural gas- and straw-powered systems when calculated via the substitution method. This is due to the credits awarded to DDGS and exported electricity. The GHG emission savings are reduced by 28 and 32 kg CO2/GJ when straw is used in the boiler, depending on if straw was otherwise incorporated or removed. These large increases are due to a combination of reduced fossil fuel for processing as well as a larger net export of electricity from the straw-based CHP plant. As allocation does not take place in the substitution method the only difference between incorporating straw and utilizing it for fuel is the N2O emissions from soil and emissions from transportation.
The GHG savings result generated by the RED is much lower than that calculated by the allocation and substitution methods. From , it can be seen that the GHG emissions from cultivation are the same as in the substitution method. This is higher than the two allocation examples. Unlike the substitution method, credits are only awarded to exported electricity. As the RED accounting rules state that residues from processing are not allocated GHG emissions the other processing stages, as well as the cultivation stage, are entirely attributed to bioethanol. Due to the calculation rules specified in Section 2.2, utilizing straw in the boiler decreases the GHG emissions from bioethanol production by 2.1 and 6.2 kg CO2/GJ depending on whether straw was otherwise incorporated or removed. The reason for this small improvement in GHG emissions (compared to the other LCA methods) is that the RED attributes a credit to exported electricity based on straw-generated electricity, which is virtually negligible.
4. Discussion
The results of the study show that the accounting rules contained within the RED methodology mean that it does not truly follow either an ALCA or a CLCA approach. Instead the RED contains a mixture of ALCA and CLCA methods, such as awarding substitution credits to exported electricity while allocating co-products by energy content. The impact of this is that it generates very different GHG emission results to the other LCA methods. The calculations show that the GHG emission savings for cereal-based bioethanol are lower when calculated according to the RED. A key question is whether this is lower than expected if calculated via an ALCA method, and whether the RED method is adopting a ‘conservative method’ in order to incentivize other feedstocks for bioethanol, as discussed in the following section.
The issue of mixed-LCA methods is not restricted to the RED. Other methodologies such as the Publicly Available Standard 2050 (BSI, Citation2011) and the original RTFO also have a combined approach (Whittaker et al., Citation2011). Similarly, agricultural and food certification schemes suffer from differences in approaches and methodologies (Keller, Milà i Canals, King, Lee, & Clift, Citation2013). Across LCA studies, there is apparent confusion over the use of system expansion in ALCA, including discrepancies where either average or marginal data are used to derive substitution credits. In many studies one can find a combination of allocation and system expansion being adopted (Menichetti & Otto, Citation2009). Brander and Wylie (Citation2011) suggest that system expansion should not be used in consumption-based ALCA as it accounts for emissions that have not physically occurred. The results of this study show very large differences in the results when allocation and substitution methods are adopted.
A particular methodological exception in the RED is that allocation and system expansion is not performed according to conventional methods; instead specific rules apply to specific co-products. Elsewhere the RED methodology has been described to contain obscurities that may lead to misleading results compared to other life cycle principles (Hagberg et al., Citation2009). In this study such obscurities refers to allocation rules regarding cereal residues, ‘residues from processing’ and the source-specific electricity credits. It could be said that these specific rules are not ISO-compliant and arbitrary. Refuting this, allocation is inherently arbitrary (Ekvall & Finnveden, Citation2001), and the ISO Standards do state that ‘There is no single method for conducting LCA. Organizations have the flexibility to implement LCA as established in this International Standard, in accordance with the intended application and the requirements of the organization’ (CEN, Citation2006a). Therefore, some arbitrary elements in life cycle models may be unavoidable, and LCAs will always vary according to the original goal and scope of the study, and on the intended use of the results (Ekvall & Weidema, Citation2004).
Unfortunately, by confusing ALCA and CLCA methods in GHG regulation it means that the goal and scope of the study are not addressed. There is, however, little guidance in the ISO Standards for developing a specific ALCA or CLCA study. A consequence of this may be the irregularity seen in the LCA methods. The RED itself states that to account for co-products via system expansion is a method best suitable for policy analysis but maybe not for ‘regulation of individual economic operators and individual consignments of transport fuels’ (EC, Citation2009), yet does not adapt this in their own methods. They may have referred to the process of expanding the system to include the functions of the co-products and not strictly substitution credits which seek to apply credits to account for displacement of products (Brander & Wylie, Citation2011).
The main confusion arises when the ISO Standards state that allocation should be avoided if possible. They suggest that if possible, the unit process should be divided into two or more sub-processes, or alternatively the product system can be expanded to include the additional functions related to the co-products (CEN, Citation2006b). If this is not possible, the inputs and outputs of the system should be allocated between co-products in a way that reflects the underlying physical relationships between them. If again not possible, allocation according to other relationships, such as economic value, is recommended (CEN, Citation2006b). In summary, the ISO standards appear to favour substitution credits, than prioritize physical allocation over economic allocation. This may be why regulation-based LCA methodologies use substitution credits, even when this is not appropriate for their goal and scope.
4.1. Suggested origin of the rules
The specific rules in the RED methodology may have arisen due to original concerns over biofuel sustainability. Upon drafting the RED, the European Commission was encouraged to introduce some effective sustainability criteria for bioliquids and ensure commercial availability of second-generation biofuels (EC, Citation2009). The current criteria forbid the production of biofuels on biodiverse, protected or endangered lands and states that land conversion should not lead to a net increase in GHG emissions. Concerns over potentially devastating consequences of indirect global land use change (ILUC) has meant that the use of ‘non-food’ and ‘non-land’ resources has been encouraged in the Directive. It does this by doubling the contribution made by biofuels produced from wastes, residues and non-food cellulosic material to the targets. This may be quadrupled for some biomass resources (e.g. wheat straw) in future revisions of the RED (ICCT, Citation2012). This way, if a Member State produces 5% of renewable fuels from food crops and 1.25% from lignocellulosic wastes, the 1.25% contribution is quadrupled and that country has met their 10% biofuel contribution target (ICCT, Citation2012).
This highlights the current state of concerns in the biofuel sector, where most questions on sustainability are directed towards ‘first generation’ biofuels (Mohr & Raman, Citation2013). Residues are a special type of product that arise in agricultural and forestry systems, as there is an option to harvest them or leave them onsite. The RED's approach of not allocating emissions from the cultivation stages to straw means that it can be acquired at zero GHG ‘cost’. This also means that the GHG emissions from first-generation biofuels are less favourable. Such a rule may originate from the Commission's promotion of utilizing residues rather than food sources for biofuel production. It is debatable whether this approach to incentivizing the use of residues is correct (Kindred, Mortimer, Sylvester-Bradley, Brown, & Woods, Citation2008). Allocating zero GHG emissions to wastes and crop residues puts them equal in terms of GHG attractiveness as feedstock, which is misleading (Aylott et al., Citation2012). The RED also specifies that upstream processing emissions are to be allocated to co-products but not ‘residues from processing’. It is clear here that some clarification is required to distinguish between the two. It can also be predicted that these LCA accounting methodology issues will also affect second-generation biofuels that utilize cereal residues, or those that utilize residues from processing as a fuel source during conversion.
4.2. Allocation issues
The goal of an ALCA is to account for the environmental impacts producing one unit of production from a specific pathway. The system boundaries should include any GHG emissions that are directly caused by the life cycle of the product studied, specifically those that are controlled by the operator (Brander et al., Citation2009; Sanchez et al., Citation2012). Therefore, in ALCA, upstream GHG emissions should be allocated between co-products, as this directly attributes environmental impacts to the final product, or producer. Allocation has been described as the major weakness of bioenergy LCAs due to the high sensitivity of the results to allocation decisions (Aylott et al., Citation2012; Gnansounou, Dauriat, Villegas, & Panichelli, Citation2009; Mendoza, van Ruijven, Vad, & Wardenaar, Citation2008). The results of this study demonstrate that allocation methods can affect the GHG emission savings calculations (Kaufman, Meier, Sinistore, & Reinemann, Citation2010).
There are various methods for allocation in LCA studies, but none without debate. Allocation by energy content could be described as being easy to apply and consistent (Ekvall & Finnveden, Citation2001). A disadvantage of this method is that the calorific value is not relevant to every co-product in biomass systems. Also, basing allocation on this metric is questionable when not every co-product is utilized as an energy source.
Economic drivers can be considered to be relevant to business decision-making, and more recently policy drivers for GHG mitigation are implemented through financial incentives (Bauen et al., Citation2008; Reijnders, Citation2008). It can be argued that price allocation is more consistent in a regulatory context as it implies the need for industry to take ownership of GHG emissions based on economic relationships (Aylott et al., Citation2012). Out of all the allocation methods, allocation by price may be more widely applicable to all co-products (Singh et al., Citation2010; Weidema, Citation2003), and in those instances when co-products have a low or zero value (i.e. they are a waste) the system will automatically respond by allocating few or no upstream emissions to them. A disadvantage of price allocation is that values are subject to change and can be difficult to determine, particularly if shadow prices (pre-processed) need to be calculated. It may be possible, however, that GHG reporting within biofuel companies will eventually be aligned and integrated with annual financial reports, allowing them to re-calculate GHG emission savings and respond in the same way they would to economic changes.
Interestingly, the RTFO applies an economic threshold to determine whether a co-product is indeed a co-product or ‘by-product’. This is defined as a co-product that represents less than 15% of the farm or factory gate value (RFA, Citation2010). When a product is a ‘by-product’ it is assumed that its consumers have ‘little influence on the sustainability of the production processes for the original product’ and do not need to report on the sustainability of their origin (RFA, Citation2010). True co-products are then allocated by energy content according to the RED.
The goal of a CLCA is to account for the environmental impacts due to a change in production of a given product. This increases the scope of the analysis to encompass market responses to changes in patterns of production (Nuffield Council on Bioethics, Citation2011), and must consider indirect effects on other markets or where resources are constrained (Brander et al., Citation2009; Sanchez et al., Citation2012). The use of substitution credits should therefore be confined to CLCAs, as they aim to describe responses to change. Exported electricity is another co-product that is often awarded credits for avoided electricity. Sanchez et al. (Citation2012) suggest this is acceptable for ALCA, though only when using average rather than marginal data. The meaningfulness of such a credit would be questionable however. The RED applies credits based on the source of the electricity. Therefore if electricity that is generated from straw is exported to the grid then the same ‘amount’ of straw-based electricity is displaced elsewhere. Again, the logic of this is questionable. This rule has been demonstrated to dampen the benefits to the bioethanol plant when utilizing renewable sources in-house ().
It is possible that this electricity credit issue will affect GHG reporting for second-generation biofuels, particularly lignocellulosic ethanol production: as these are envisaged to be able to utilize the lignin stream as a fuel source for heat and electricity demands to reduce primary energy requirements for processing (Aden et al., Citation2002; Slade, Bauen, & Shah, Citation2009). As lignin is a ‘residue from processing’ any exported electricity would not receive a credit under the RED. The lignin stream represents approximately one-third of the input biomass stream (Wooley et al., Citation1999); this methodological effect could also compromise the expected GHG emission savings from second-generation biofuels compared to a purely ALCA approach.
4.3. Introducing ILUC
Currently GHG reporting methodologies do not include GHG emissions from indirect land use change in the system boundaries of their GHG calculations as it is difficult to calculate, predict and validate (EC, Citation2010). There is some indication, however, that the RED intends on including this in further revisions (EC, Citation2009; ICCT, Citation2012). Modelling the GHG emissions that arise due to ILUC requires a coupled modelling framework to estimate interactions in agricultural and energy markets, predict production volumes and prices, and estimate risks of conversion of land (Bauen et al., Citation2008). Models can be based on economics (Hiederer et al., Citation2010), patterns of land use change (Bauen et al., Citation2010) or statistics (Kim & Dale, Citation2011). It is therefore highly possible that ILUC factors will range considerably, increasing uncertainty in GHG reporting. Despite this, ILUC should not be exclusive to GHG reporting for biofuels, but part of a wider, global framework that protects carbon-rich and biodiverse lands from destruction (Nuffield Council on Bioethics, Citation2011). Considering that ILUC is more suited to CLCA, impacts of ILUC may need to be accounted for through some other mechanism. As the RED intends to include ILUC in future GHG regulation calculations it suggests it is simplifying the debate to how land is valued, managed and governed (Mohr & Raman, Citation2013), and confusing the role that regulation of biofuels has in this world-wide issue. It may be possible that applying ‘penalties’ for ILUC could go some way towards the RED's goal to promote the uptake of non-food resources in the bioenergy sector; however careful consideration would be required to assess the effectiveness of such penalties in reducing GHG emissions, and maybe the two should be kept separate. The European Commission is investigating the issue of ILUC in separate policy streams; therefore it is possible that it will be addressed within a wider and international approach.
5. Conclusion
Without set rules there are various options to how an LCA could be performed, and it is possible that two studies that examine the same functional unit could both comply with the ISO standards, but provide different results. Attempts to prescribe a set or rules show it is challenging to develop a universally accepted method. There are, however, logical structures to ALCA and CLCA approaches. The RED has been shown to not only combine ALCA and CLCA approaches but also to contain rules for specific co-products. For example, cereal residues are not allocated GHG emissions from cultivation. This may be a result of concerns over ILUC, which is a consequential issue. This rule places cereal residue-based bioethanol at an advantage. Another rule in the RED is that exported electricity is credited according to its source. The results of this study show that this reduces the net benefit of utilizing straw as a fuel source in the bioethanol plant. Reasons for this rule are less clear. A possible concern about these rules is that they place artificial biases in GHG emission results. Key improvements and justifications of methodological decisions are needed, along with precise definitions of co-products, by-products and wastes as different interpretations of these will affect the results. Clear definitions are necessary as differences in interpretations will lead to conflicting results and lack of certainty in GHG regulation.
It is recommended that substitution credits are avoided in ALCA, and for consistency the same allocation method should be applied to each co-product. The RED has adopted allocation by energy content, which has been demonstrated to calculate lower GHG emissions result compared to allocation by price (Gnansounou et al., Citation2009; Yan & Boies, Citation2013). This is also shown in this study. Allocation via economic value should be compatible across systems, and reflects the behaviour of market system while acknowledging that value can be derived from co-products. This method also automatically adapts when wastes with zero value are produced.
Acknowledgements
This study was performed after completing a PhD at the University of Bath, during which some of the ideas and analyses presented here were based. I would like to thank the reviewers for their very constructive comments.
References
- Adams, P. W., Bows, A., Gilbert, P., Hammond, J., Howard, D., Lee, R., … Whitaker, J. (2013). Understanding greenhouse gas balances of bioenergy systems (Supergen Bioenergy Hub Report). Supergen Bioenergy Hub.
- Aden, A., Ruth, M., Ibsen, K., Jechura, J., Neeves, K., Sheehan, J., … Lukas, J. (2002). Lignocellulosic biomass to ethanol process design and economics utilizing co-current dilute acid prehydrolysis and enzymatic hydrolysis current and futuristic scenarios (Technical Report No. NREL/TP-510–32438). National Renewable Energy Laboratory.
- AEA Technology & North Energy Associates. (2010). BEAT2 (biomass environmental assessment tool) v2.1: User guide (No. 4). Oxford: Author.
- Alberichi, S., & Hamelinck, C. (2010). Annotated example of a GHG calculation using the EU Renewable Energy Directive methodology (No. Project Number: PEGENL083540). Ecofys.
- Aylott, M., Higson, A., Evans, G., Hamer, A., & Mortimer, N. (2011). What is the most appropriate LCA method for measuring greenhouse gas emissions from bioenergy? Biofuels, Bioproducts and Biorefining, 5(2), 122–124. doi:10.1002/bbb.282
- Aylott, M., Higson, A., Evans, G., & Mortimer, N. (2012). Measuring the energy and greenhouse gas balances of biofuels and bio-based chemicals using LCA (No. NNFCC 12–023). York: NNFCC.
- Bauen, A., Chudziak, C., Vad, K., & Watson, P. (2010). A causal descriptive approach to modelling the GHG emissions associated with the indirect land use impacts of biofuels. Final report. A study for the UK Department for Transport. E4Tec.
- Bauen, A., Watson, P., & Howes, J. (2008). Carbon reporting within the renewable transport fuel obligation – methodology. E4Tec.
- Biograce.net. (2012). BIOGRACE. Retrieved May 17, 2012, from http://www.biograce.net/content/ghgcalculationtools/overview
- Black, M., Whittaker, C., Hosseini, S. A., Diaz-Chavez, R., Woods, J., & Murphy, R. J. (2011). Life cycle assessment and sustainability methodologies for assessing industrial crops, processes and end products. Industrial Crops and Products, 34(2), 1332–1339. doi:10.1016/j.indcrop.2010.12.002
- Brander, M., Tipper, R., Hutchison, C., & Davis, G. (2009). Consequential and attributional approaches to LCA: A guide to policy makers with specific reference to greenhouse gas LCA of biofuels (Techical Paper No. TP-090403-A). Ecometrica.
- Brander, M., & Wylie, C. (2011). The use of substitution in attributional life cycle assessment. Greenhouse Gas Measurement and Management, 1(3–4), 161–166. doi:10.1080/20430779.2011.637670
- Brown, M., & McLeavey-Reville, C. (2011). Driving a resource efficiency power generation sector in europe. Edinburgh: Delta Energy and Environment.
- BSI. (2011). PAS 2050:2011 specification for the assessment of the life cycle greenhouse gas emissions of goods and services. London: Author.
- CEN. (2006a). BS EN ISO 14040:2006. Environmental management – life cycle assessment – principles and framework. (British Standard No. BS EN ISO 14040:2006). Brussels: European Committee for Standardisation.
- CEN. (2006b). BS EN ISO 14044:2006. Environmental management – life cycle assessment – requirements and guidelines (British Standard No. BS EN ISO 14044:2006). Brussels: European Committee for Standardisation.
- DECC. (2012). Valuation of energy use and greenhouse gas (GHG) emissions: Background documentation. London: Author.
- DEFRA, & DECC. (2012). 2012 guidelines to defra/DECC's GHG conversion factors for company reporting: Methodology paper for emission factors. London.
- De Klein, C. A. M., Novoa, R. S. A., Ogle, S., Smith, K. A., Rochette, P., Wirth, T. C., … Rypdal, K. (2006). N2O emissions from managed soils, and CO2 emissions from lime and urea application. Chapter 11. 2006 IPCC Guidelines for National Greenhouse Gas Inventories.
- EC. (2009). Directive 2009/28/EC of the European Parliament and of the council of 23 April 2009 on the promotion of the use of energy from renewable sources and amending and subsequently repealing Directives 2001/77/EC and 2003/30/EC (No. 2009/28/EC). Author.
- EC. (2010). Report from the commission on indirect land-use change related to biofuels and bioliquids (No. COM(2010) 811 final). Author.
- ECN. (n.d.). Phyllis, database for biomass and waste. Retrieved February 4, 2011, from http://www.ecn.nl/phyllis/
- Ekvall, T., & Finnveden, G. (2001). Allocation in ISO 14041 – a critical review. Journal of Cleaner Production, 9(3), 197–208. doi:10.1016/S0959-6526(00)00052-4
- Ekvall, T., & Weidema, B. P. (2004). System boundaries and input data in consequential life cycle inventory analysis. The International Journal of Life Cycle Assessment, 9(3), 161–171. doi:10.1007/BF02994190
- EPR. (2011). Overview | ely | assets | energy power resources. Retrieved September 1, 2011, from http://www.eprl.co.uk/assets/ely/overview.html
- EUCAR, CONCAWE, & JRC. (2006). Well-to-wheels analysis of future automotive fuels and powertrains in the European context (No. Version 2b).
- Gnansounou, E., Dauriat, A., Villegas, J., & Panichelli, L. (2009). Life cycle assessment of biofuels: Energy and greenhouse gas balances. Bioresource Technology, 100(21), 4919–4930. doi:10.1016/j.biortech.2009.05.067
- Hagberg, L., Saarnholm, E., Gode, J., Ekvall, T., & Rydberg, T. (2009). LCA calculations on Swedish wood pellet production chains – according to the Renewable Energy Directive (No. IVL Report B1873). Stockholm: Swedish Environmental Research Institute.
- Hiederer, R., Ramon, F., Capitani, C., Koeble, R., Bludjea, V., Gomez, O., … Marelli, L. (2010). Biofuels: A new methodology to estimate GHG emissions from global land use change: A methodology involving spatial allocation of agricultural land demand and estimation of CO2 and N2O emissions. Luxembourg: Joint Research Centre.
- ICCT. (2012). Proposed amendments to EU Fuel Quality and Renewable Energy Directives (Policy Update). Author.
- IEA. (2010). Gas-Fired Power (Technology Brief). International Energy Agency, Energy Technology Network.
- Kaufman, A. S., Meier, P. J., Sinistore, J. C., & Reinemann, D. J. (2010). Applying life-cycle assessment to low carbon fuel standards – how allocation choices influence carbon intensity for renewable transportation fuels. Energy Policy, 38(9), 5229–5241. doi:10.1016/j.enpol.2010.05.008
- Keller, E. J., Milà i Canals, L., King, H., Lee, J., & Clift, R. (2013). Agri-food certification schemes: How do they address greenhouse gas emissions? Greenhouse Gas Measurement and Management, 3(3–4), 85–106. doi:10.1080/20430779.2013.840200
- Kim, S., & Dale, B. E. (2011). Indirect land use change for biofuels: Testing predictions and improving analytical methodologies. Biomass and Bioenergy, 35(7), 3235–3240. doi:10.1016/j.biombioe.2011.04.039
- Kindred, D. R., Mortimer, N., Sylvester-Bradley, R., Brown, G., & Woods, J. (2008). Understanding and managing uncertainties to improve biofuel GHG emissions calculations (Project Report No. 435 Part 2). London: Home Grown Cereals Authority.
- Manninen, K., Koskela, S., Nuppunen, A., Sorvari, J., Nevalainen, O., & Siitonen, S. (2013). The applicability of the renewable energy directive calculation to assess the sustainability of biogas production. Energy Policy, 56, 549–557. doi:10.1016/j.enpol.2013.01.040
- Martinez-Hernandez, E., Ibrahim, M. H., Leach, M., Sinclair, P., Campbell, G. M., & Sadhukhan, J. (2013). Environmental sustainability analysis of UK whole-wheat bioethanol and CHP systems. Biomass and Bioenergy, 50, 52–64. doi:10.1016/j.biombioe.2013.01.001
- Mendoza, A., van Ruijven, T., Vad, K., & Wardenaar, T. (2008, January). The allocation problem in bio-electricity chains (MSc thesis, Industrial Ecology). Leiden.
- Menichetti, E., & Otto, M. (2009). Energy balance and greenhouse Gas emissions of biofuels from a life cycle perspective. In R. W. Howarth & S. Bringezu (Eds.), Biofuels: Environmental consequences and interactions with changing land use. Proceedings of the scientific committee on problems of the environment (SCOPE) international biofuels project rapid assessment (pp. 81–109). Ithaca, NY: Gummersbach Germany, Cornell University.
- Mohr, A., & Raman, S. (2013). Lessons from first generation biofuels and implications for the sustainability appraisal of second generation biofuels. Energy Policy, 63, 114–122. doi:10.1016/j.enpol.2013.08.033
- Nix, J. (2011). The john Nix farm management pocketbook (41st ed.). Leicestershire: The Anderson Centre.
- North Energy Associates. (2010). Jatropha workbook (Version NNFCC 10–016). Retrieved from http://www.nnfcc.co.uk/tools/oilseed-rape-workbook?sectorKey=crops-wood-waste&typeKey=technical-reports-tools
- Nuffield Council on Bioethics. (2011). Biofuels: Ethical issues. London.
- Punter, G., Rickeard, D., Larive, J. F., Edwards, R., Mortimer, N., Horne, R., … Woods, J. (2004). Well-to-wheel evaluation for production of ethanol from wheat. A report by the LowCVP fuels working group, WTW sub-group (No. FWG-P-04–024).
- Reijnders, L. (2008). Transport biofuels – a life-cycle assessment approach. CAB Reviews: Perspectives in Agriculture, Veterinary Science, Nutrition and Natural Resources, 3(71), 1–8. doi: 10.1079/PAVSNNR20083071
- RFA. (2010). Carbon and sustainability reporting within the renewable transport fuel obligation: Technical guidance part one (3.2). London: Author.
- Royal Society. (2008). Sustainable biofuels: Prospects and challenges (Policy Document No. 01/08). Author.
- Sanchez, S. T., Woods, J., Akhurst, M., Brander, M., O'Hare, M., Dawson, T. P., … Malpas, R. (2012). Accounting for indirect land-use change in the life cycle assessment of biofuel supply chains. Journal of The Royal Society Interface, 9(71), 1105–1119. doi:10.1098/rsif.2011.0769
- Singh, A., Pant, D., Korres, N. E., Nizami, A.-S., Prasad, S., & Murphy, J. D. (2010). Key issues in life cycle assessment of ethanol production from lignocellulosic biomass: Challenges and perspectives. Bioresource Technology, 101(13), 5003–5012. doi:10.1016/j.biortech.2009.11.062
- Slade, R., Bauen, A., & Shah, N. (2009). The greenhouse gas emissions performance of cellulosic ethanol supply chains in Europe. Biotechnology for Biofuels, 2(1), 15. doi:10.1186/1754-6834-2-15
- UK Power. (2014). Electricity running cost calculator | electricity prices | electricity costs. Retrieved July 15, 2014, from http://www.ukpower.co.uk/tools/running_costs_electricity#.U8U651JY_Fk
- Von Blottnitz, H., & Curran, M. A. (2007). A review of assessments conducted on bio-ethanol as a transportation fuel from a net energy, greenhouse gas, and environmental life cycle perspective. Journal of Cleaner Production, 15(7), 607–619. doi:10.1016/j.jclepro.2006.03.002
- Weidema, B. (2003). Market information in life cycle assessment (Environmental Project No. 863 2003). Danish Environmental Protection Agency.
- Whitaker, J., Ludley, K. E., Rowe, R., Taylor, G., & Howard, D. C. (2010). Sources of variability in greenhouse gas and energy balances for biofuel production: A systematic review. GCB Bioenergy, 2(3), 99–112. doi:10.1111/j.1757-1707.2010.01047.x
- Whittaker, C. (2013). The importance of life cycle assessment methodology in the regulation of biofuels (PhD thesis). University of Bath, Bath.
- Whittaker, C., Borrion, A. L., Newnes, L., & McManus, M. (2014). The renewable energy directive and cereal residues. Applied Energy, 122, 207–215. doi:10.1016/j.apenergy.2014.01.091
- Whittaker, C., McManus, M. C., & Hammond, G. P. (2011). Greenhouse gas reporting for biofuels: A comparison between the RED, RTFO and PAS2050 methodologies. Energy Policy, 39(10), 5950–5960. doi:10.1016/j.enpol.2011.06.054
- Wooley, R., Ruth, M., Sheehan, J., Ibsen, K., Majdeski, H., & Galvez, A. (1999). Lignocellulosic biomass to ethanol process design and economics utilizing co-current dilute acid prehydrolysis and enzymatic hydrolysis current and futuristic scenarios (Technical Report No. NREL/TP-580–26157). National Renewable Energy Laboratory.
- Yan, X., & Boies, A. M. (2013). Quantifying the uncertainties in life cycle greenhouse gas emissions for UK wheat ethanol. Environmental Research Letters, 8(1), 015024. doi:10.1088/1748-9326/8/1/015024