ABSTRACT
A methodological proposal was developed for the study of prehistoric siliceous artefacts which includes the use of smartphone photography as an analytical tool. Different kinds of materials (cherts, rock crystal and quartzite) were sampled from the lithic assemblage of La Calvera rock-shelter (Camaleño, Spain), chosen as a case study. Each sample was photographed with a smartphone and colour features were compared with portable and non-destructive standardised techniques including visible spectrophotometry, Raman, diffuse reflectance Fourier transform infrared spectroscopy and portable X-ray fluorescence. The obtained results suggest that smartphone image analysis is a reliable approach to characterise siliceous rocks and that it can be a valuable, cheap and fast method especially suitable for the first screening of large lithic assemblages.
1. Introduction
Siliceous lithic artefacts are a unique source of information about ancient populations, especially for the Prehistoric period. The study and identification of the origins of the raw materials used for the manufacture of lithic tools permits the reconstruction of socio-ecological dynamics linked to the mobility of human groups (Hess and Riede Citation2021; Machado et al. Citation2017; Ramacciotti, García-Puchol, Cortell-Nicolau et al. Citation2022). Several analytical techniques have been used to characterise siliceous artefacts and raw materials including, for example, Fourier transform infrared spectroscopy (FT-IR), portable X-ray fluorescence spectroscopy (pXRF), inductively coupled plasma optical emission spectroscopy (ICP-OES), inductively coupled plasma mass spectrometry (ICP-MS) and instrumental neutron activation analyses (INAA; Andersen et al. Citation2021; Chatzimpaloglou Citation2020; Delluniversità et al. Citation2019; Eixea et al. Citation2022; Newlander and Lin Citation2017; Ramacciotti et al. Citation2019; Ramacciotti, García-Puchol, Cortell-Nicolau et al. Citation2022). Nevertheless, many archaeological studies rely only on the analysis of macroscopic characteristics such as colour, translucency, or cortex presence to classify siliceous artefacts (Luedtke Citation1992). Although naked-eye examination permits a non-invasive and rapid examination of numerous samples, it is strongly conditioned by the experience and sensitivity of the researcher, and can lead to misleading or unreproducible results (Calogero Citation1992; Hess Citation1996; Milne, Hamilton, and Fayek Citation2009). However, there are several methods available for archaeologists to obtain data for artefacts colour properties, such as colour classification by Munsell Colour System charts (Chatzimpaloglou Citation2020; Hess and Riede Citation2021; Nazaroff, Baysal, and Çiftçi Citation2013; Newlander and Lin Citation2017). Munsell Colour charts allow a rapid and relatively straightforward characterisation of colour against standardised comparison charts, but this still risks influence from colour perception and environmental factors (Bloch et al. Citation2021). Furthermore, the attribution of a univocal colour code can be problematic in case of heterogeneous materials, being sometimes the primary chosen colour somewhat arbitrary. However, colour properties can be also measured by employing spectroscopic techniques, which measure the characteristics of the reflected light in the visible spectrum under controlled illumination conditions (Delluniversità et al. Citation2019; Zorzona-Indart, Lopez-Arce, and López-Polín Citation2021) providing reliable and robust data, but increasing costs with time-consuming operations and requiring training on the operators.
The present study shows a new methodological approach tested on La Calvera rock-shelter lithic assemblage (Diez-Castillo Citation1997), which includes the use of smartphone photography as an analytical tool. Smartphone-based methods have been developed in analytical chemistry for the colorimetric characterisation of different matrices such as human fluids or food (Rezazadeh et al. Citation2019; Sáez-Hernández, Antela, Mauri-Aucejo et al. Citation2022; Sáez-Hernández, Ruiz, Mauri-Aucejo et al. Citation2022). However, very few works deal with archaeological remains (Ramacciotti, Gallello, Lezzerini et al. Citation2022; Ramacciotti, Gallello, López-Montalvo et al. Citation2022; Sáez-Hernández, Antela, Gallello et al. Citation2022). Concerning siliceous artefacts, Marcos et al. (Citation2022) characterised the colour properties of the artefacts from Los Canes cave (Sierra del Cuera, Spain) by image analysis of camera pictures but the artefacts were powdered prior to being photographed, which is a major flaw since in many cases siliceous artefacts cannot be destroyed due to their archaeological value. Furthermore, in the above-quoted study, the direct use of RGB or HSL parameters, which characterise primaries colours (red, green and blue) or points in a colour space (hue, saturation and lightness respectively), the lack of information related to the illumination conditions and the employed device, as well as the absence of any comparison and control with standard methods for colour analysis, compromise the experimental reproducibility and do not permit the evaluation of the quality of the data.
In the present study, each sample was photographed with a smartphone in a controlled environment and colour data were obtained by image analysis. This allowed the comparison of results obtained by imaging with those from more standard and widely used techniques in order to evaluate the reliability and potential of the smartphone method for characterisation and provenance studies of siliceous artefacts.
2. La Calvera rock-shelter and its geological and archaeological contexts
La Calvera rock-shelter (Camaleño, Spain) is a small cavity excavated in a bluff where alternating strata of quartzite and limestone outcrop. The site is located in the south-eastern end of Picos de Europa National Park at 1180 m above the sea level, within the Cantabrian Mountains. The current relief of the Cantabrian zone is the result of the superposition of the Variscan and Alpine orogeneses of the Carboniferous and Tertiary periods, respectively (Marquínez and Adrados Citation2000). The Carboniferous represents the key period in the formation of the Picos de Europa Unit (Bahamonde, Vera, and Colmenero Citation1997), in which carbonate sedimentation began on the marine platform, accumulating up to 1000 metres of carbonate sediments which today, after subsequent orogenic periods, form the calcareous rocks of the Picos de Europa, that are of greatest magnitude in the National Park and occupy the main area of park.
From a geographical point of view, it can be considered part of the area of Peña Oviedo megalithic complex, which is characterised by the presence of dolmens and megalithic structures dated up to the second half of the sixth millennium BP (; Diez-Castillo Citation2010).
These monumental structures, which are among the archaeological evidence of the Vasco-Cantabrian region neolithisation (González Morales et al. Citation2004), were possibly aggregation places connected to the exploitation of the area for pastoral activities and were linked to open-air settlements such as those of the same Peña Oviedo and of La Calvera (La Campa), occupied from the second half of the sixth millennium BP to the Bronze Age (Diez-Castillo Citation2008, Citation2010). On the other hand, the survey excavations of La Calvera rock-shelter offered evidence of human activities prior to the introduction of the production economy and the appearance of the above-quoted megalithic complex, up to the ninth millennium BP (Diez-Castillo Citation2005). The stratigraphical sequence offered remains of hearts and charcoal, as well as of lithic industry, mainly composed by chips and flakes of dark grey chert and quartzite, similar to the rocks outcropping in the surroundings of the rock-shelter, although other chert types and fragments of rock crystal were found (Diez-Castillo Citation1997).
3. Materials and methods
3.1. Collected samples
Seventy-four samples were selected to carry out the archaeometric study of La Calvera rock-shelter lithic assemblage (the table is shown in the Supplementary Online Materials, SM1a).
Ten samples are chunks (RM01-05) of local grey chert raw material found in the rock-shelter and outcropping in its immediate surroundings ((a)). Twenty-eight flakes of grey chert were analysed and, though almost all of them have macroscopic features similar to the above-quoted raw materials ((b)), AR21 is different from the others, showing a lighter colour ((c)). Other chert types were collected from the lithic assemblage: two samples of whitish chert (AR09, AR23; (d)) and three samples of yellow chert (AR37, AR38.1-2; (e)). Fifteen fragments of quartzite ((f)) and five of rock crystal (i.e. translucent and colourless quartz macrocrystal; (g)) were collected from the lithic assemblage as well. Furthermore, in order to test the proposed method of colour analysis, eleven fragments of grey Domeño chert (Eixea, Villaverde, and Zilhão Citation2011) from a geological outcrop in Andilla (Valencia, Spain; Ramacciotti, García-Puchol, Cortell-Nicolau et al. Citation2022) were introduced in the sampling ((h)).
3.2. Colour analysis and imaging
Each sample was brushed with ultrapure water to remove remains of earth and incrustations prior to the analyses. Images of the samples were captured with a smartphone (Samsung Galaxy S7 Edge) to perform colour analysis. In order to validate the results, colour was also characterised by reflectance spectrophotometry in the visible region (Vis-SP) with a CM-26d portable spectrophotometer by Konica Minolta, calibrated with reference white and black to convert the signal to CIELAB colour space coordinates. Two up to five circular areas (d = 5 mm) were analysed and average values were taken into account. This last technique was considered as a reference method.
Flowchart for imaging process is shown in the . In order to carry out image analysis, each sample was photographed with a smartphone in a controlled environment with constant illumination and equal camera setting (ISO: 100, f: 1/350 s, T: 5500 K, focus: manual, zoom: 3x). The camera settings were chosen to obtain the best quality pictures and avoid artefacts in the given photography environment. Imaging of smartphone pictures was performed with MATLAB (version: R2019b) by MathWorks and Colorlab toolbox (Malo and Luque Citation2002). In some cases, such as for most of the studied quartzites and dark chert samples, background pixels could be kept out on the basis of RGB parameters. For example, for most chert samples setting a mask to exclude pixels with R lower than ∼200 was enough, while for quartzites B parameters was more effective (B lower than ∼200). However, in other ones (e.g.: whitish samples) the sample surface could not be isolated in this way and a rectangular mask was applied. RGB data for each pixel were then converted to the CIEXYZ colour space coordinates and finally to CIELAB ones by a reference white photographed in the same condition of the samples. Average L*, a* and b* were used which account for lightness, red–green and yellow–blue axes respectively (Fairchild Citation2004).
Both Vis-SP and imaging were performed on most of the collected samples (Supplementary Online Material, SM1b). However, rock crystals were not taken into account and some samples were excluded from Vis-SP analysis due to their very small size and shape which did not permit to adequately cover the measurement spot of the device.
3.3. Atomic and molecular spectroscopy
Atomic and molecular spectroscopy analyses were carried out directly on the surface of intact samples with pXRF, Raman and diffuse reflectance infrared Fourier transform spectroscopy (DRIFT). Some of the smallest samples could not be analysed by pXRF and DRIFT due to their large measurement area requirements (Supplementary Online Material, SM1b).
Multielement analysis was carried out by a S1 Titan pXRF by Bruker equipped with a Rh X-ray tube (50 kV) and X-Flash® SDD (resolution: 147 eV; FWHM: 5.9 keV). Geochem-trace application was employed to obtain the concentrations of Al, K, Ca, Fe, Ti and Zr, since most of the other potential elemental markers could not be detected by the spectrometer. Average concentrations from two up to five measurements for each sample were taken into account.
Raman and DRIFT were used to obtain information on the main minerals and compounds. Raman spectroscopy analysis was performed in the region between 2000 and 100 cm−1 with an i- Raman® Plus spectrometer (model: BWS465-785S) by B&W Tek coupled with a microscope (20x magnification) and laser wavelength of 785 nm (max power at laser port: 495 mW). Each sample was analysed in two up to four points with an integration time of 1.5 s, 10 repetitions and laser set at 15% of power. Spectra were subsequently averaged. A portable spectrometer 4300 Handheld FT-IR by Agilent Technologies was used for DRIFT spectroscopy analyses. The analysis consisted in 50 scans in the mid-infrared region (4000 - 650 cm- 1). Each sample was analysed two up to four times in different spots and spectra were averaged. Background was measured between each analysis. Resolution of 4 cm−1 was employed.
3.4. Data analysis
Statistical analysis was performed in R (version: 4.1.2; R Core Team Citation2021). Different principal component analysis (PCA) models were built to explore pXRF data, and both pXRF and imaging (L* and b*) ones. In each case, data were previously autoscaled (i.e., z-scores were used instead of raw data). Two cluster analysis (CA) models were built with the samples from La Calvera rock- shelter (except for rock crystal) using as variables previously autoscaled colour parameters (L* and b* from imaging) in the first, and concentrations from pXRF in the second (method: average linkage). The following R packages were employed for data analysis and visualisation: dendextend (version: 1.15.2; Galili Citation2015), factoextra (version: 1.0.7; Kassambara and Mundt Citation2020), ggplot2 (version: 3.3.5; Wickham Citation2009).
4. Results and discussion
4.1. Colour analysis
The results of colour analysis for imaging are reported in .
Table 1. Average values and standard deviations of CIELAB colour space parameters obtained by imaging of smartphone photos.
Raw materials chunks and local grey cherts show similar values for the three colour parameters with L* ranging between 30.4 and 47, a* between 1.7 and 4.4 and b* between −9.8 and −3.1. All the other cherts from La Calvera show higher L*, being AR21 (light grey) the darkest one (L* = 67.1) and the whitish the brightest ones (L* is 87.9 and 91.7 for AR09 and AR23 respectively), while the yellowish cherts have intermediate values (L* = 72.9−79.5). For all these, a* values are from −1.0 (AR09) up to 7.8 (AR38.1). These cherts are also characterised by higher b* (b* = 8.0–33.4), indicating higher degree of yellowness. In particular, yellowish chert artefacts are the highest for this parameter. Concerning dark quartzites, they show intermediate values of L* compared to the dark cherts and the others (L* = 38.6–66.7), while a* ranges between 1.1 and 7.3. As regards b*, quartzites show in most of the cases positive values (from −2.6 to 13.7). Domeño chert fragments, introduced to test the method, have in most of the cases slightly higher lightness (L* = 37.6–64.2) than grey local cherts and higher b* as well (b* = 0.0–9.3), while a* ranges between 3.2 and 5.2. Comparing the results obtained by imaging and by Vis-SP () shows that the variance of L*, a* and b* in each sample is relevantly higher for imaging than for Vis-SP analysis ( and ). Furthermore, imaging gives slightly higher values for L* and a*, and slightly lower values for b* than Vis-SP.
Table 2. Average values and standard deviations of CIELAB colour space parameters obtained by Vis-SP.
(a) shows the heatmaps of the three colour parameters on the surface of sample AR03. The scale is based on mean and standard deviation, and each pixel is coloured according to its z-score. As can be observed, surface irregularities determine relevant deviations from the average, caused by the different light reflection angle ((a)). This issue can be partially avoided with Vis-SP by working with small measurement areas and choosing the most suitable spots. This fact can explain the different variance for the three CIELAB parameters obtained by the two methods.
Figure 4. Results of colour analysis: (a) Heatmap of L*, a* and b* for AR03 (see for mean, µ, and standard deviation, σ); (b) comparison between colour analysis carried out by Vis-SP and imaging; (c) scatter plot for L* vs b* obtained by imaging of smartphone pictures.
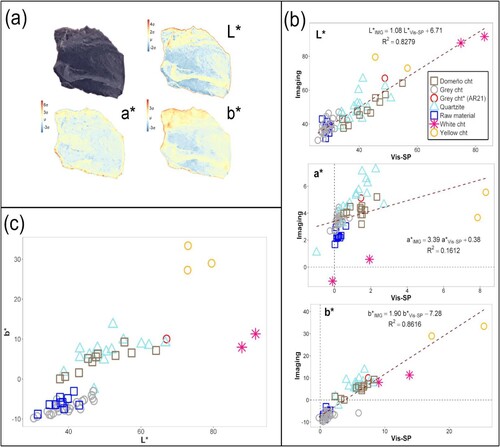
Although imaging data from the three parameters deviate from those obtained by the reference technique ((b)), L* and b* are highly correlated, being R2 equal to 0.83 and 0.86, respectively. On the other hand, a* data show a low correlation (R2 = 0.16), possibly due to the presence of outliers (i.e., white and yellow cherts). The high correlations indicate that the differences among the classes of samples highlighted by imaging are actually linked to their own colour features, suggesting that smartphone photography could be a useful method to obtain objective colour data in order to carry out a first classification that can provide useful information for raw material provenance studies.
Based on the aforementioned observations, the samples were plotted in a L* vs b* scatterplot ((c)). The grey chert artefacts from La Calvera fall together with the chunks of raw material, indicating that the two class of samples have very similar colour features, while the yellow and white cherts are characterised by the highest levels for b* and L* respectively and are plotted far from all the other samples. The other grey chert (AR21) has intermediate L* and b*. Finally, the grey Domeño chert from Andilla, introduced as control samples, and the quartzite flakes are plotted between AR21 and the other grey cherts from La Calvera.
4.2. DRIFT and Raman spectroscopy
Characteristic DRIFT ((a)) and Raman ((b,c)) spectra for each rock type are shown. The most relevant features for DRIFT are the Reststrahlen bands for quartz at ∼1158 and ∼788 cm−1 (Parish, Swihart, and Li Citation2013). The wide unresolved band between ∼3700 and ∼2700 cm−1 can be attributed to stretching vibrations of OH, possibly linked to the presence of clay phases (Yeasmin et al. Citation2017). Except for rock crystal, in which the OH band is barely visible, the different chert types from La Calvera cannot be clearly differentiated on the basis of mid-IR spectral characteristics. On the other hand, Domeño chert samples show bands close to 2880 and 2520 cm−1, and an inverted band close to 1500 cm−1, which are linked to the presence of carbonate minerals (Arrizabalaga et al. Citation2014; De Lorenzi Pezzolo, Colombi, and Mazzocchin Citation2018).
Figure 5. DRIFT (a) and Raman (b and c) spectra for the different types of samples. Spectra were scaled by standard normal variate (SNV) and they are offset to avoid overlapping.
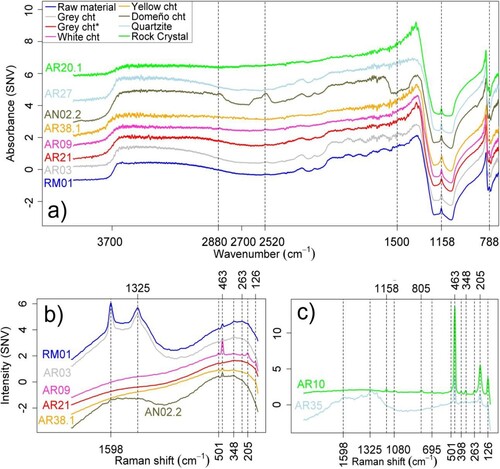
Raman spectra for samples of the different types were shown in (b,c). Most of the bands shown by the samples correspond to quartz vibrational modes (around 126, 205, 348, 398, 463, 695, 805, 1080 and 1158 cm−1; Chukanov and Vigasina Citation2020). However, it should be pointed out that quartz bands are more numerous and intense in rock crystals and in several quartzite samples than in chert, possibly due the higher crystallinity of these samples. The band at 501 cm−1 could mark the presence of moganite (Capel Ferrón et al. Citation2015) and is present with variable intensities in the different kinds of cherts. Except for AR21, all the grey chert samples show the typical D (∼1325 cm−1) and G (∼1598 cm−1) bands for carbonaceous materials (Sforna, van Zuilen, and Philippot Citation2014). Quartzite samples show also features in this region, though mostly unresolved, suggesting the possible presence of similar compounds, maybe characterised by more amorphous material (Sforna, van Zuilen, and Philippot Citation2014).
4.3. Multielement analysis
Elemental concentrations obtained by pXRF analyses are reported in the Supplementary Online Material (SM3). In order to explore the analytical results and interpret them, PCA was carried out and the first two PC were taken into account, explaining 54.6% and 15.6% of the overall variance respectively ().
Most local grey chert artefacts fall close to the chunks of raw materials and can be distinguished from the other cherts ((a)), including the light grey sample (AR21), and to rock crystal due to the high PC1 scores of the latter. Indeed, the local grey cherts artefacts are characterised by higher levels of Al, K, Ti, Fe and Zr than the other cherts, as suggested by loadings plot ((b)) and corroborated by the observation of the results (see the Supplementary Online Materials, SM4). Quartzite samples have scores similar to the local grey cherts, though they are scattered on both the axes. Finally, Domeño cherts from Andilla, introduced as test set for the method, can be distinguished from La Calvera materials due to higher PC1 and PC2 scores, especially linked to their high levels of calcium, which is positively correlated with both the PCs ((c)), consistently with the presence of carbonates highlighted by DRIFT.
4.4. Critical evaluation of the method
The tested smartphone photography approach permitted to obtain a wide range of useful information for the study of the different lithic materials employed at La Calvera rock-shelter.
Colour analysis of smartphone photos offered data which could have been used to discriminate among different rock types. The good quality of L* and b* parameters is suggested by the high correlations to those obtained with a Vis-SP, although it has been observed that imaging gave slightly higher values for L* and lower one for b* than the reference technique. Imaging data permitted to discriminate among the different cherts, in particular among the three grey chert types which were analysed: the local dark grey chert, the light grey chert (AR21) and the Domeño chert from Andilla, introduced to test the method (). It should be pointed out that imaging can be applied on all the chert and quartzite samples, regardless to their size and shape. This is an important aspect since other techniques like pXRF, DRIFT and Vis-SP require a minimum surface area to carry out proper analyses, and X-ray fluorescence spectroscopy results can be also influenced by sample thickness (Williams, Taylor, and Orr Citation2020). Thus, imaging could be applied to challenging remains such as microdebitage. Furthermore, it is non-destructive, and also cheaper and more user friendly than laboratory devices.
Focussing on the materials from La Calvera rock-shelter, as can be observed in the CA (), three groups are mainly present according to smartphone imaging ((a)): local grey chert and chunks of raw materials fall in Group 1, most of the quartzite samples and grey chert AR21 cluster in a second group (Group 2) and, finally, the other allochthonous cherts fall in the third group (Group 3), in which they are divided in two sub-clusters. The clustering on the basis of pXRF data ((b)) is quite similar: Group 1 is mainly composed by local grey cherts and chunks of raw materials, and Group 2 by the non-local cherts, while most of the quartzite samples are in the remaining clusters together with few local grey cherts. Raman spectroscopy gave similar results, distinguishing the different lithologies, as well as between local and non-local cherts ((b,c)).
These results suggest that smartphone pictures data could have been used as a previous step to identify the main groups in order to carry out a further in-depth characterisation on a representative subset. This would be particularly useful especially while working on large lithic assemblages or to select samples for destructive analyses, and could be easily implemented in the procedure of photographic documentation. Furthermore, colour data can be used as variables together with chemical ones in data analysis. Indeed, the comparison between pXRF PCAs with and without smartphone data suggests that colour properties could increase the resolution of the model in order to carry out a provenance study (see the Supplementary Online Material, SM3).
4.5. Preliminary provenance hypothesis
The information obtained by smartphone imaging is in line with the study carried out by Columbu et al. (Citation2023). In fact, the most common rock in the assemblage is a dark grey chert outcropping close to the site, found as chunks of raw materials and flakes in the rock-shelter. The possibility to distinguish this chert type from the other dark cherts from Cantabrian Range relies mainly on petrographic and mineralogical characterisation (Herrero-Alonso et al. Citation2021), the results of which suggest an attribution to Barcaliente Fm. chert (Columbu et al. Citation2023).
Similarly for quartzite, which is the second most common lithotype along the archaeological sequence. The area of La Calvera rock-shelter is characterised by the presence of different quartzite and quarzitic conglomerate outcrops (Rodríguez Fernández et al. Citation1994) and, as previously suggested (Prieto et al. Citation2021), a petrographic characterisation was carried out to determine the source but the precise type of rock could not be identified (Columbu et al. Citation2023).
The scarce occurrence of other chert types suggests a non-local supply. Concerning yellow chert (AR37, AR38.1-2), macroscopic features and elemental data are compatible with Piloña chert, outcropping in eastern Asturias about 50 km north-west to La Calvera rock-shelter (Tarriño-Vinagre et al. Citation2013; Tarriño-Vinagre, Elorrieta, and García-Rojas Citation2015). This chert was identified also in other Mesolithic sites of Picos de Europa area (León, Spain; Neira Campos, Fuertes Prieto, and Herrero Alonso Citation2016). As regards, the light grey (AR21) and white (AR09, AR23) cherts, any identification on the basis of the present data would be highly speculative.
Rock crystal is instead a possible local raw material. Indeed, macrocrystal quartz outcrops in the southern areas of Picos de Europa Fm. and in eastern Asturias, where it is commonly found in Mesolithic contexts (Arias et al. Citation2009; Neira Campos, Fuertes Prieto, and Herrero Alonso Citation2016).
Resuming, our approach employing smartphone imaging suggests a prevalence of local raw materials, especially dark grey chert and quartzite which outcrop in the surroundings of the site, as well as few cobbles of rock crystal, as confirmed by mineralogical and petrographic studies (Columbu et al. Citation2023). Allochthonous rocks are instead very scarce. This supply pattern is consistent with those observed in other sites of the area during the Mesolithic (Arias et al. Citation2009; Herrero-Alonso, Fuertes-Prieto, and Neira-Campos Citation2020).
5. Conclusions
The present study permitted to test for the first time the use of smartphone photography as an analytical tool for the characterisation of colour features of siliceous artefacts from a Prehistoric site. Smartphone imaging proved to be a reliable method to obtain colour data on this kind of material, as indicated by the comparison with the results from a reference technique (Vis-SP). It permitted to observe differences among rock classes, obtaining similar information to the standardised techniques such as Raman spectroscopy and pXRF, suggesting that this approach should be tested also on other types of rocks. These results indicate that colour analysis of smartphone photos could be a fast, reliable and cheap method to screen large lithic assemblages and to select representative samples for further investigation or destructive analyses.
ysta_a_2240175_sm8592.docx
Download MS Word (171.4 KB)Disclosure statement
No potential conflict of interest was reported by the author(s).
Additional information
Funding
Notes on contributors
Mirco Ramacciotti
Mirco Ramacciotti got a PhD at the University of Valencia (Spain) in 2022 and is currently a postdoctoral researcher of the Department of Prehistory, Archaeology and Ancient History of the same institution. He works with the ArchaeChemis research unit (https://www.uv.es/archaechemis), carrying out research on archaeological lithic and lithoid materials by the means of archaeometric methods.
Gianni Gallello
Gianni Gallello has completed his PhD at the University of Valencia (Spain) where he founded ArchaeChemis (2014) and he is head of the group. He was granted by the European Commission with a Marie Curie individual fellowship (MATRIX project), hosted by the University of York (Department of Archaeology and Department of Environment and Geography) and he is now Distinguished Researcher “Beatriz Galindo” at the Department of Prehistory, Archaeology and Ancient History of the University of Valencia. In the last few years, his research activity has been focused on the chemical analysis of a wide range of archaeological materials such as sediments, human remains, stones, mortars, ceramics, etc. He has developed new methodological proposals through the employment of novel sampling strategies, Rare Earth Elements, major and trace element analysis and multivariate statistics.
Stefano Columbu
Stefano Columbu is associate professor in Cagliari University. He holds courses in “Mining Georesources” and “Mineralogical Petrographic Applications for the Environment and Cultural Heritage” in Master's Degree in Geological Sciences and Technologies, where he is president of the Internship Commission. The scientific activity was mainly aimed at: i) geochemical-mineralogical-petrographic characterisation, decay and provenance of geomaterials (stones and mortars) from monuments of historical-cultural significance of Italian, Spanish, Portuguese areas, belonging to the Nuragic, Punic, Roman, Paleochristian, Arab-Islamic and early medieval times; ii) petroarchaeometric studies of archaeological lithic artifacts (e.g. Eneolithic splinter axes, obsidian artefacts, etc.) found in Sardinian prehistoric settlements and millstones from the North African Roman age; iii) compositional analysis of natural pozzolans and their chemical reactivity with lime and hydraulic limes binders through experimentation with newly synthesised mortars with low environmental impact.
Dario Fancello
Dario Fancello is a researcher and laboratory technician at the University of Cagliari. Since receiving his PhD in Geology in 2016, he has participated to research projects in different fields spanning from metamorphic petrology to geochemistry, from ore geology to environmental mineralogy, and finally archaeometry and geological heritage. This wide-ranging interest in the various branches of geology results in a heterogeneous scientific production, mainly consisting of papers published in indexed and peer-reviewed international journals. He has participated and contributed with oral presentations to numerous national and international congresses in the geological field and beyond. He is specialised in analytical techniques such as XRD, XRF, EMP and LA-ICP-MS, but recently he's also interested in field surveying using photogrammetry and laser scanners.
Agustín Diez-Castillo
Agustín Diez-Castillo got a PhD in History at the University of Cantabria in 1996 with a dissertation about the prehistoric peopling in the western valleys of Cantabria. He moved to Berkley (California) for four years, thanks to a scholarship of the Fundación Marcelino Botín, where he worked at the Department of Anthropology and at the Archaeological Research Facility with prof. Margaret Conkey. He came to the Department of Prehistory, Archaeology and Ancient History of the University of Valencia in 2000, thanks to the Reincorporation Program for Doctors and Technologists Abroad. His main research lines are landscape archaeology, cultural contacts between last hunters-gatherers communities and first farmers in the Cantabrian area as well as in the Mediterranean one and the employment of new technologies in cultural heritage study, such as the development of the Archaeological Information System SIDGEIPA.
Oreto García-Puchol
Oreto García-Puchol got her PhD in History from the University of Valencia in 2002. From 2010 to 2015 she was a “Ramon y Cajal” researcher at the Department of Prehistory, Archaeology and Ancient History (University of Valencia), where she is currently a Professor integrated in the PREMEDOC Research Group. She specializes in socioecological dynamics during the recent Prehistory in the Western Mediterranean. Her research interests include the Mesolithic, Neolithic transition, cultural transmission, lithic technology, the emergence of social hierarchies, Mesolithic and Neolithic funerary practices and 3D Archaeology. She has directed archaeological fieldworks in the Mediterranean basin of Iberia, including sites like Falguera Rock Shelter, Pastora Cave and Cocina Cave.
Agustín Pastor
Agustín Pastor has been Full Professor at the Department of Analytical Chemistry of the University of Valencia since 2007. During his career, he has dealt with the development of analytical methods such as chromatography and spectrometry and he has managed several projects in the field of analytical chemistry. He has been also the head of ArchaeChemis research unit since 2014.
M. Luisa Cervera
M. Luisa Cervera is Full Professor at the Department of Analytical Chemistry of the University of Valencia. During her career, she has dealt with the development of analytical methods for the detection of contaminants in the environment and for food sciences. Recently, she has led the project “Smartphone and Green Analytical Chemistry” (PROMETEO 2019-056) related to the development of analytical methods by the means of smartphone devices.
References
- Andersen, C., C. Dunlap, M. Freeman, and B. W. Hicks. 2021. “Imaging and Analysis of Near-Infrared Fluorescence from Tiger Chert and Native American Tiger Chert Artifacts.” Journal of Archaeological Science: Reports 35:102722. https://doi.org/10.1016/j.jasrep.2020.102722.
- Arias, P., P. Fernández, C. Marcos, and I. Rodríguez. 2009. “The Elusive Flint: Raw Materials and Lithic Technology in the Mesolithic of Eastern Asturias, Spain.” In Mesolithic Horizons, edited by S. McCartan, R. Schulting, G. Warren, and P. Woodman, 860–866. Oxford: Oxbow Books.
- Arrizabalaga, I., O. Gómez-Laserna, J. Aramendia, G. Arana, and J. M. Madariaga. 2014. “Applicability of a Diffuse Reflectance Infrared Fourier Transform Handheld Spectrometer to Perform in Situ Analyses on Cultural Heritage Materials.” Spectrochimica Acta Part A: Molecular and Biomolecular Spectroscopy 129:259–267. https://doi.org/10.1016/j.saa.2014.03.096.
- Bahamonde, J. R., C. Vera, and J. R. Colmenero. 1997. “Geometría y facies del margen progradante de una plataforma carbonatada carbonífera (Unidad de Picos de Europa, Zona Cantábrica).” Revista de la Sociedad Geológica de España 10:163–182.
- Bloch, L. C., J. D. Hosen, E. C. Kracht, M. J. LeFebvre, C. J. Lopez, R. Woodcock, and W. F. Keegan. 2021. “Is It Better to be Objectively Wrong or Subjectively Right?: Testing the Accuracy and Consistency of the Munsell Capsure Spectrocolorimeter for Archaeological Applications.” Advances in Archaeological Practice 9:132–144. https://doi.org/10.1017/aap.2020.53.
- Calogero, B. L. 1992. “Lithic Misidentification.” Man in the Northeast 43:87–90.
- Capel Ferrón, C., L. León-Reina, S. Jorge-Villar, J. M. Compaña, M. A. G. Aranda, J. T. López Navarrete, V. Hernández, et al. 2015. “Combined Raman Spectroscopic and Rietveld Analyses as a Useful and Nondestructive Approach to Studying Flint Raw Materials at Prehistoric Archaeological Sites.” Archaeological and Anthropological Sciences 7:235–243. https://doi.org/10.1007/s12520-014-0189-0.
- Chatzimpaloglou, P. 2020. “A Geoarchaeological Methodology for Sourcing Chert Artefacts in the Mediterranean Region: A Case Study from Neolithic Skorba on Malta.” Geoarchaeology 35:897–920. https://doi.org/10.1002/gea.21813.
- Chukanov, N. V., and M. F. Vigasina. 2020. Vibrational (Infrared and Raman) Spectra of Minerals and Related Compounds, Springer Mineralogy. Cham: Springer International Publishing. https://doi.org/10.1007/978-3-030-26803-9
- Columbu, S., D. Fancello, G. Gallello, M. Ramacciotti, and A. Diez-Castillo. 2023. “Multi-Analytical Techniques to Define the Mineralogical and Petrophysical Characteristics and Provenance of Siliceous Lithic Findings: The Case Study of La Calvera Rock Shelter (Cantabria, Spain).” Minerals 13 (5): 666. https://doi.org/10.3390/min13050666.
- Delluniversità, E., I. M. Muntoni, I. Allegretta, M. Tarantini, A. Monno, P. Maiorano, A. Girone, M. Morsilli, R. Terzano, and G. Eramo. 2019. “Development of a Multiparametric Characterisation Protocol for Chert Investigation and Application on the Gargano Promontory Mines.” Archaeological and Anthropological Sciences 11:6037–6063. https://doi.org/10.1007/s12520-019-00875-8.
- De Lorenzi Pezzolo, A., M. Colombi, and G. A. Mazzocchin. 2018. “Where Did Roman Masons Get Their Material From? A Preliminary DRIFTS/PCA Investigation on Mortar Aggregates from X Regio Buildings in the Veneto Area (NE Italy) and Their Potential Sources.” Environmental Science and Pollution Research 25:28798–28807. https://doi.org/10.1007/s11356-018-2202-0.
- Diez-Castillo, A. 1997. “Utilización de los recursos en la Marina y Montaña cantábricas: una prehistoria ecológica de los valles del Deva y Nansa.” Illunzar 3:23–190.
- Diez-Castillo, A. 2005. “El Contacto Entre Cazadores - Recolectores y Agricultores en los Valles Occidentales de Cantabria.” In Actas Del III Congreso Del Neolítico En La Península Ibérica: Santander, 5 a 8 de Octubre de 2003, edited by P. Arias Cabal, R. Ontañon Peredo, and C. García-Moncó Piñeiro, 425–434. Santander: Universidad de Cantabria.
- Diez-Castillo, A. 2008. “Las excavaciones en la zona arqueológica de la Peña Oviedo. Las campañas de 2000 a 2003.” In Actuaciones arqueologicas en Cantabria: 2000- 2003. Gobierno de Cantabria. Consejeria de cultura, turismo y deporte, edited by R. Ontañon Peredo, 101–106. Santander: Gobierno de Cantabria.
- Diez-Castillo, A. 2010. “Nuevas tecnologías y viejas piedras: un repaso al megalitismo en Cantabria.” Illunzar 7:7–35.
- Eixea, A., C. Roldán, V. Villaverde, I. Días, I. Prudêncio, R. Marques, D. Russo, K. Gméling, G. Cavallo, and S. Murcia. 2022. “Geochemical and Petrographic Analyses on Middle and Upper Palaeolithic Cherts from the Central Region of Mediterranean Iberia.” Lithic Technology 48 (2): 1–24. https://doi.org/10.1080/01977261.2022.2097811.
- Eixea, A., V. Villaverde, and J. Zilhão. 2011. “Aproximación al aprovisionamiento de materias primas líticas en el yacimiento del Paleolítico medio del Abrigo de la Quebrada (Chelva, Valencia).” Trabajos de Prehistoria 68:65–78. https://doi.org/10.3989/tp.2011.11059.
- Fairchild, M. D. 2004. Color Appearence Model. 2nd ed. Chirchester: John Wiley and Sons Ltd.
- Galili, T. 2015. “dendextend: An R Package for Visualizing, Adjusting and Comparing Trees of Hierarchical Clustering.” Bioinformatics 31:3718–3720. https://doi.org/10.1093/bioinformatics/btv428.
- González Morales, M., L. G. Straus, A. Díez-Castillo, and J. Ruiz Cobo. 2004. “Postglacial Coast & Inland: The Epipaleolithic-Mesolithic-Neolithic Transitions in the Vasco-Cantabrian Region.” Munibe (Antropologia-Arkeologia) 56:61–78.
- Herrero-Alonso, D., N. Fuertes-Prieto, and A. Neira-Campos. 2020. “Management of Lithic raw Materials in the “Mesolithic with Geometrics” (Northern of Iberian Peninsula): Chaînes Opératoires and Territory.” Journal of Archaeological Science: Reports 29:102093. https://doi.org/10.1016/j.jasrep.2019.102093.
- Herrero-Alonso, D., A. Tarriño-Vinagre, E. Fernández-Martínez, N. Fuertes-Prieto, and A. Neira-Campos. 2021. “Black Chert and Radiolarite: Knappable Lithic raw Materials in the Prehistory of the Cantabrian Mountains (North Spain).” Archaeological and Anthropological Sciences 13:113. https://doi.org/10.1007/s12520-021-01340-1.
- Hess, S. C. 1996. “Chert Provenance Analysis at the Mack Canyon Site, Sherman County, Oregon: An Evaluative Study.” Geoarchaeology 11:51–81. https://doi.org/10.1002/(SICI)1520-6548(199601)11:1<51::AID-GEA3>3.0.CO;2-9.
- Hess, T., and F. Riede. 2021. “The Use of Lithic Raw Materials at the Early Mesolithic Open- Air Site Feuersteinacker (Vogelsbergkreis, Germany).” Geoarchaeology 36:252–265. https://doi.org/10.1002/gea.21828.
- Kassambara, A., and F. Mundt. 2020. “factoextra: Extract and Visualize the Results of Multivariate Data Analyses.” R package version 1.0.7. https://CRAN.R-project.org/package=factoextra
- Luedtke, B. E. 1992. An Archaeologist’s Guide to Chert and Flint, Archaeological Research Tools. Los Angeles.: Institute of Archaeology, University of California.
- Machado, J., F. J. Molina, C. M. Hernández, A. Tarriño, and B. Galván. 2017. “Using Lithic Assemblage Formation to Approach Middle Palaeolithic Settlement Dynamics: El Salt Stratigraphic Unit X (Alicante, Spain).” Archaeological and Anthropological Sciences 9:1715–1743. https://doi.org/10.1007/s12520-016-0318-z.
- Malo, J., and M. J. Luque. 2002. COLORLAB: A Color Processing Toolbox for Matlab. Universitat de València. http://www.uv.es/vista/vistavalencia/software.html.
- Marcos, C., M. de Uribe- Zorita, P. Fernández, P. Álvarez- Lloret, J. Vallejo- Llano, and P. Arias. 2022. “Diversification of Lithic Raw Materials Used by Mesolithic Inhabitants of Los Canes Cave (Sierra del Cuera, Eastern Asturias, Spain), and Quartz Crystallite Size of Chert as an Essential Indicator Parameter of its Provenance.” Geoarchaeology 21930:902–922. https://doi.org/10.1002/gea.21930.
- Marquínez, J., and L. Adrados. 2000. “La geología y el relieve de los Picos de Europa.” Naturalia Cantabricae 1:3–19.
- Milne, S. B., A. Hamilton, and M. Fayek. 2009. “Combining Visual and Geochemical Analyses to Source Chert on Southern Baffin Island, Arctic Canada.” Geoarchaeology 24:429–449. https://doi.org/10.1002/gea.20273.
- Nazaroff, A. J., A. Baysal, and Y. Çiftçi. 2013. “The Importance of Chert in Central Anatolia: Lessons from the Neolithic Assemblage at Çatalhöyük, Turkey.” Geoarchaeology 28:340–362. https://doi.org/10.1002/gea.21446.
- Neira Campos, A., N. Fuertes Prieto, and D. Herrero Alonso. 2016. “The Mesolithic with Geometrics South of the ‘Picos de Europa’ (Northern Iberian Peninsula): The Main Characteristics of the Lithic Industry and raw Material Procurement.” Quaternary International 402:90–99. https://doi.org/10.1016/j.quaint.2015.10.065.
- Newlander, K., and Y. Lin. 2017. “Integrating Visual and Chemical Data to Source Chert Artifacts in the North American Great Basin.” Journal of Archaeological Science: Reports 11:578–591. https://doi.org/10.1016/j.jasrep.2016.12.037.
- Parish, R. M., G. H. Swihart, and Y. S. Li. 2013. “Evaluating Fourier Transform Infrared Spectroscopy as a Non-Destructive Chert Sourcing Technique.” Geoarchaeology 28:289–307. https://doi.org/10.1002/gea.21437.
- Prieto, A., I. Yusta, M. García- Rojas, A. Arrizabalaga, and J. Baena Preysler. 2021. “Quartzite Procurement in Conglomerates and Deposits: Geoarchaeological Characterization of Potential Catchment Areas in the Central Part of the Cantabrian Region, Spain.” Geoarchaeology 36:490–510. https://doi.org/10.1002/gea.21838.
- Ramacciotti, M., G. Gallello, M. Lezzerini, S. Pagnotta, A. Aquino, L. Alapont, J. Antonio Martín Ruiz, and A. Pérez-Malumbres Landa. 2022. “Smartphone Application for Ancient Mortars Identification Developed by a Multi-Analytical Approach.” Journal of Archaeological Science: Reports 43:103433. https://doi.org/10.1016/j.jasrep.2022.103433.
- Ramacciotti, M., G. Gallello, E. López-Montalvo, A. Morales-Rubio, and C. Roldán. 2022. “Aplicación de smartphone en el análisis colorimétrico de réplicas de pinturas rupestres.” In XIII Congreso Nacional Del Color: Terrassa, 29-30 Juny - 1 Juliol, 2022. Presented at the XIII Congreso Nacional del Color, edited by F. J. Burgos Fernández, and M. Vilaseca Ricart, 61–64. Universitat Politècnica de Catalunya.
- Ramacciotti, M., G. Gallello, A. Pastor, A. Diez Castillo, and O. García Puchol. 2019. “Chert Nucleus and Cortex Characterization for Archaeological Provenance Study Tested in the Prebaetic System Region (Valencian Community, Spain).” Lithic Technology 44:166–180. https://doi.org/10.1080/01977261.2019.1618043.
- Ramacciotti, M., O. García-Puchol, A. Cortell-Nicolau, G. Gallello, A. Morales- Rubio, and A. Pastor. 2022. “Moving to the land: First archaeometric study of chert procurement at Cueva de la Cocina (Eastern Iberia).” Geoarchaeology 37:544–559. https://doi.org/10.1002/gea.21903.
- R Core Team. 2021. R: A Language and Environment for Statistical Computing.R Foundation for Statistical Computing, Vienna, Austria. URL https://www.R-project.org/.
- Rezazadeh, M., S. Seidi, M. Lid, S. Pedersen-Bjergaard, and Y. Yamini. 2019. “The Modern Role of Smartphones in Analytical Chemistry.” TrAC Trends in Analytical Chemistry 118:548–555. https://doi.org/10.1016/j.trac.2019.06.019.
- Rodríguez Fernández, L. R., P. Barba, N. Heredia, and G. Gallastegui. 1994. Memoria de la hoja no. 81 (Potes), Mapa geológico de España E. 1:50.000. Segunda seria - Primera edición. Madrid.: Istituto Tecnologico Geominero de España.
- Sáez-Hernández, R., K. U. Antela, G. Gallello, M. L. Cervera, and A. R. Mauri-Aucejo. 2022. “A smartphone-based innovative approach to discriminate red pigments in roman frescoes mock-ups.” Journal of Cultural Heritage 58:156–166. https://doi.org/10.1016/j.culher.2022.10.003.
- Sáez-Hernández, R., K. U. Antela, A. R. Mauri-Aucejo, A. Morales-Rubio, and M. L. Cervera. 2022. “Smartphone-based Colorimetric Study of Adulterated Tuna Samples.” Food Chemistry 389:133063. https://doi.org/10.1016/j.foodchem.2022.133063.
- Sáez-Hernández, R., P. Ruiz, A. R. Mauri-Aucejo, V. Yusa, and M. L. Cervera. 2022. “Determination of Acrylamide in Toasts Using Digital Image Colorimetry by Smartphone.” Food Control 141:109163. https://doi.org/10.1016/j.foodcont.2022.109163.
- Sforna, M. C., M. A. van Zuilen, and P. Philippot. 2014. “Structural Characterization by Raman Hyperspectral Mapping of Organic Carbon in the 3.46 Billion-Year-old Apex Chert, Western Australia.” Geochimica et Cosmochimica Acta 124:18–33. https://doi.org/10.1016/j.gca.2013.09.031.
- Tarriño-Vinagre, A., E. Duarte Matías, D. Santamaría Álvarez, L. Martínez Fernández, J. Fernández de la Vega Medina, P. Suárez Ferruelo, V. Rodríguez Otero, E. Forcelledo Arena, and M. de la Rasilla Vives. 2013. “El Sílex de Piloña. Caracterización de una nueva fuente de materia prima lítica en la Prehistoria de Asturias.” In F. Javier Fortea Pérez: Universitatis Ovetensis Magister. Estudios en homenaje, edited by Marco de la Rasilla Vives, and F. Javier Fortea Pérez, 115–132. Oviedo: Ménsula Ediciones.
- Tarriño-Vinagre, A., I. Elorrieta, and M. García-Rojas. 2015. “Flint as raw Material in Prehistoric Times: Cantabrian Mountain and Western Pyrenees Data.” Quaternary International 364:94–108. https://doi.org/10.1016/j.quaint.2014.10.061.
- Wickham, H. 2009. ggplot2: Elegant Graphics for Data Analysis. New York, NY: Springer New York. https://doi.org/10.1007/978-0-387-98141-3
- Williams, R., G. Taylor, and C. Orr. 2020. “pXRF Method Development for Elemental Analysis of Archaeological Soil.” Archaeometry 62:1145–1163. https://doi.org/10.1111/arcm.12583.
- Yeasmin, S., B. Singh, C. T. Johnston, and D. L. Sparks. 2017. “Evaluation of pre-Treatment Procedures for Improved Interpretation of mid Infrared Spectra of Soil Organic Matter.” Geoderma 304:83–92. https://doi.org/10.1016/j.geoderma.2016.04.008.
- Zorzona-Indart, A., P. Lopez-Arce, and L. López-Polín. 2021. “Archaeological Chert Artifacts from Atapuerca Sites (Burgos, Spain): Characterization, Causes of Decay and Selection of Compatible Consolidating Product.” Cons. Património 20:20–35.