Abstract
Six Aspergillus flavus isolates out of 17 fungal isolates were sampled from diverse food and organic matter in southwest Nigeria. All the A. flavus samples produced aflatoxin and cyclopiazonic acid. These six isolates constitute a mycobank of toxigenic species for analytical research involving the safety of food, feed and the general environment. Consumption of wholesome food materials is contingent upon accurate identification of fungal contaminants, detection and quantification of potential mycotoxins and subsequent removal and prevention of fungal contamination. Thorough investigations rest on proper maintenance of a reliable mycobank. This article introduces a mini toxigenic A. flavus bank, supported by pictorial illustrations, as a preliminary project for the establishment of a permanent culture collection centre in Nigeria.
Introduction
Being a tropical country, with attendant high temperature and humidity, Nigeria harbours a large number of heterogeneous filamentous fungi that exist heterotrophically (Bandh et al. Citation2012). Although some species/genera are more common and easily identifiable, fungal misidentification is always a possible impediment to scientific research. Being potential contaminants of food items in Nigeria (Fapohunda and Ogundero Citation1990; Simsekli et al. Citation1999; Sen and Asan Citation2001), it is important that more focus is given to their accurate identification in order to have a grasp of the intervention strategies necessary for food safety and security. Filamentous fungi are abundant in the soil and some species, such as within the genus Aspergillus, have been implicated in both animal and human illness and death due to the production of mycotoxins (e.g. aflatoxins). Aflatoxins are serious secondary metabolites that have two possible designations, B or G, which relate to fluorescence under exposure to long-wave ultraviolet (UV) light. B aflatoxins appear blue in colour, while G aflatoxins appear green in colour due to differences in their chemical structures (Verma Citation2004). B aflatoxins occur more frequently as contaminants, and are also believed to be more potent, than G aflatoxins (Bennett and Klich Citation2003). From a genomic perspective, the inability to produce G aflatoxins is reported to result from a deletion between the norB (aflF) and cypA (aflU) genes, upstream in the aflatoxin biosynthesis pathway (Ehrlich et al. Citation2004).
Many methods such as calorimetry (Li Citation2004) and chemical characterization (Rodrigues et al. Citation2009) have been used to identify filamentous fungi. Molecular investigations are also common practice; however, examinations of fungal morphology and microscopy are still integral to fungal identification. A common source of food contamination is the air, the bioload of which is always a reflection of the hygiene status of an environment; volatile organic compounds and mycotoxins may be implicated in such situations. The aims of this study were to identify common fungi in food and organic matter in southwest Nigeria; to ignite concerted awareness of the dangers of aflatoxin contamination in food and feed by examining the sampled fungi for aflatoxigenicity; and to establish a fungal databank for future scientific reference in Nigeria.
Materials and methods
Sampling and morphological identification
Samples of food, decaying leaves and logs of wood were collected from six towns in southwest Nigeria: Sagamu, Ilesa, Ilishan-Remo, Osogbo, Ogbomoso and Lagos. The sampled food items included yam, cassava, maize, bean, tomato and orange. Colonized host tissue was plated onto potato dextrose agar (PDA) and incubated at 28°C for 8 days. Streptomycin sulphate was added at 250 mg/L to prevent bacterial contamination. Pure fungal samples were then sent to the Southern Regional Research Center (United States Department of Agriculture) in New Orleans for morphological and genomic identification. To determine the identity of each fungal sample, the cultures were first screened microscopically to ascertain genus level so that the appropriate plating method could be performed. After 7 days of incubation in the dark, all colony diameters were measured and phenotypic characteristics were recorded based on both macroscopic and microscopic observations, which are often considered sufficient in the identification of some fungi (Diba et al. Citation2007). Identification to species level required the use of dichotomous keys, and photographs of certain morphological characteristics were taken using a Nikon Digital Sight DS-5M camera (Tokyo, Japan).
Aflatoxin detection and quantification for Aspergillus flavus samples
Cultures were three-point inoculated onto yeast extract sucrose agar (YES) plates and incubated in the dark at 25°C. One culture plate was inoculated for each isolate. After 7 days, each sample was extracted for toxin detection using thin layer chromatography (TLC). Entire plate contents were macerated and placed in a 250 mL Erlenmeyer flask with 50 mL acetone and 25 mL diH2O and shaken in an orbital shaker (25°C, 125 rpm) for at least 1 h. The liquid portion was filtered and then partitioned using 50 mL methylene chloride (CH2Cl2) whereby each sample mixture was inverted 3 times and then allowed to separate. The CH2Cl2-soluble phase was dried over sodium sulphate and further evaporated to dryness in vacuo. The CH2Cl2 extracts were then dissolved in 200 μL of acetone for analysis. Five microlitres of each sample was spotted onto a TLC plate (silica gel 60) and the plate was developed in a toluene/ethyl acetate/formic acid (5:4:1, v:v:v) solution. Both an aflatoxin standard (A9441, Sigma Chemical Co., St. Louis, MO, USA) and a cyclopiazonic acid (CPA) standard (C1530, Sigma Chemical Co.) were also spotted on the plate. Once dried, the plate was examined for aflatoxins under long-wave UV light (365 nm). Detection of CPA involved spraying of the TLC plate, first with a mixture of 1% dimethyl-aminobenzaldehyde in ethanol, followed by sulphuric acid:ethanol (1:1), then drying the spray solution on the plate with a heat gun. The presence of CPA shows on the plate as a purple spot.
Quantification of aflatoxins involved high-performance liquid chromatography (HPLC) analysis whereby dried sample residues were suspended in 1000 μL acetonitrile before transfer of 500 μL to 2 mL, 0.45 μm nylon filter centrifuge tubes (Spin-X; Corning Inc., Corning, NY, USA) and centrifugation at 14,000 rpm for 1 min. Aliquot samples (10 μL) of filtered extract were then analysed by HPLC according to a previously described procedure (Sobolov and Dorner Citation2002). HPLC analyses were performed with a Waters 2695 HPLC combined with a Waters 2475 fluorescence detector (Milford, MA, USA). Post-column derivatization was performed with a Photochemical Reactor for Enhanced Detection (Aura Industries Inc., New York, NY, USA) system. AFB1 detection wavelengths were 365 nm (excitation) and 474 nm (emission). Sample extracts (10 μL) were injected for separation through a Waters Nova-Pak C18 (3.9 mm × 150 mm; 5 μm) reverse-phase column (Milford, MA, USA). The analytical column was protected by a guard column containing the same packing. Column temperature was maintained at 38°C, and the elution flow rate was 0.8 mL/min with mobile phase solvent consisting of water:methanol:n-butanol (1400:720:15, v/v/v). Retention time for AFB1 was 20.1 min. A calibration curve with high linearity (R 2 = 0.9998) was constructed for AFB1 from a series of diluted standards.
Molecular analysis and identification
For each isolate, approximately 400–600 μL of fungal spore suspensions (in 0.01% Triton) were utilized to inoculate 75 mL of potato dextrose broth in a 250 mL Erlenmeyer flask. Flasks were placed in an orbital shaker (30°C, 135 rpm) for 24–48 h. Once substantial spore germination occurred, the resulting mycelia were vacuum filtered through a Büchner funnel using Miracloth to retain the mycelial tissue, and a wash was performed with sterile water. Mycelial tissue (200 mg) was collected into a 1.5 mL microcentrifuge tube, and a DNA extraction protocol was followed using the MasterPure Yeast Purification kit (Epicentre Biotechnologies; Madison, WI, USA). Optical density readings were performed on extracted genomic DNA using a Nano Drop (ND-1000) spectrophotometer (Wilmington, DE, USA), and dilutions (if necessary) were made in preparation for Polymerase chain reaction (PCR) amplification of a portion of the internal transcribed spacer (ITS) region (∼200 bp). For the PCR amplification reaction, 0.5 μL of ITS1 (TCCGTAGGTGAACCTGCGG) and ITS2 (GCTGCGTTCTTCATCGATGC) were mixed with 12.5 μL of GoTaq Colorless Mastermix (Promega; Madison, WI, USA), 1 μL of genomic DNA and 10.5 μL of nuclease-free water. PCR cycling conditions included a 2-min initial denaturation at 95°C, followed by 40 cycles of denaturation at 95°C for 30 s, annealing at 58°C for 30s and extension at 72°C for 30 s. A final extension was added at 72°C for 7 min. To test the success of PCR amplification, electrophoresis was performed using 5 μL of DNA on a 1.5% agarose gel in Tris-acetate-EDTA (TAE) buffer. DNA amplicons were purified using a QIAquick PCR purification kit (Qiagen Sciences; Germantown, MD, USA) before submission to Eton Bioscience for sequencing (San Diego, CA, USA). Nucleotide sequences were cleaned and trimmed using Sequencher version 5.0 (Gene Codes Corporation; Ann Arbor, MI, USA) and then the sequences were BLAST searched for molecular confirmation/identification. For the strains morphologically identified as Aspergillus flavus, diagnostic PCR amplification of the norB-cypA (aflF-aflU) region was performed using the primers and protocol of Ehrlich et al. (Citation2004). Based on deletions in the region between the norB (aflF) and cypA (aflU) genes, upstream in the aflatoxin gene cluster, it is possible to determine morphotype and aflatoxin-producing potential of A. flavus. For example, an amplicon size of ∼1000 bp is indicative of an A. flavus morphotype that is capable of producing only B aflatoxins and large sclerotia; an amplicon size of ∼300 bp is indicative of an A. flavus morphotype that is reported to also produce only B aflatoxins and has small sclerotia. Species/morphotypes that produce both B and G aflatoxins have a larger span of this intergenic region such as Aspergillus parasiticus, Aspergillus nomius and some A. flavus (∼1800 bp).
Results and discussion
Morphological identification and species description
Five genera were discerned in the initial screen: Aspergillus, Penicillium, Fusarium, Trichoderma and Mucor. For the aspergilli, the cultures were plated/studied using the techniques described in Identification of Common Aspergillus Species (Klich (Citation2002). For Penicillium, the culture was plated and identified using the techniques found in Pitt's (Citation2000) A Laboratory Guide to Common Penicillium Species. The Fusarium, Trichoderma and Mucor isolates were plated onto both PDA and water agar and incubated at 25°C. The Fusarium isolate was referenced using The Genus Fusarium (Booth Citation1977) and the Mucor and Trichoderma isolates were referenced using the Compendium of Soil Fungi (Domsch et al. Citation1993). Seventeen isolates were sampled and identified from a total of 40 substrates (, ). Of these, six (35%) were identified as A. flavus based on morphological characteristics. The other morphologically identified species, which are now serving as part of fungal databanks at both Babcock University and Fountain University, include Aspergillus fumigatus, Aspergillus terreus, Aspergillus niger, Penicillium minioluteum, Fusarium semitectum, Trichoderma harzianum and Mucor hiemalis f.sp. luteus.
Table 1. Identification (ID) of sampled fungi based on morphology and ITS sequence analysis
Figure 1. Macromorphology images for each isolate using various media (top image), with corresponding micromorphology based on 40× magnification (bottom image). Images are shown in numerical order from SF1 (top left) to SF18 (bottom right).
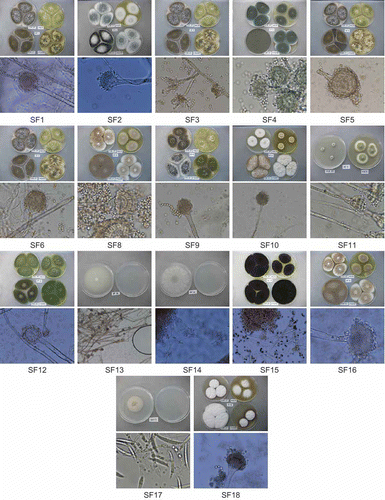
Aspergillus flavus (SF1, SF3, SF5, SF6, SF9 and SF12) is a filamentous fungus with worldwide distribution. According to Rodrigues et al. (Citation2009), the aspergilli from subgenus Circumdati, section Flavi may be grouped in either of two ways: the aflatoxigenic species (A. flavus, A. parasiticus and A. nomius) that are serious agricultural contaminants and the non-aflatoxigenic species (A. oryzae, Aspergillus tamarii and Aspergillus sojae) that offer significant industrial contributions (e.g. food fermentation). Despite its association with aflatoxin production, A. flavus is also capable of being non-aflatoxigenic. For example, two commercially available biocontrol strains exist in the United States, both A. flavus, that offer control/prevention of contamination by native toxigenic strains in agricultural fields (Brown et al. Citation1991; Abbas et al. Citation2006). Aspergillus flavus may exist as either of two morphotypes which relate to sclerotium size. Large- (L-) strain A. flavus produces moderate quantities of sclerotia greater than 400 μm in diameter, while small- (S-) strain A. flavus produces many sclerotia smaller than 400 μm in diameter. Morphotype testing may involve the use of V-8 medium (Cotty Citation1989) for plating individual strains, but it may also involve PCR amplification of the norB-cypA (aflF-aflU) region (Ehrlich et al. Citation2004). The S-strain morphotype of A. flavus is reported to produce higher concentrations of aflatoxins than the L-strain morphotype (Cotty Citation1989). Aspergillus flavus grows better with water activity (aw) between 0.86 and 0.96 (Vujanovic et al. Citation2001). The optimum temperature for A. flavus to grow is 37°C, but fungal growth has been observed at temperatures ranging from 12°C to 47°C. Such a wide range of temperatures contributes to its pathogenicity in humans. Aspergillus flavus seems to be the main aetiological agent of osteoarticular infection and rhinosinusitis, more likely to be recovered from the upper respiratory tract than any other Aspergillus species (Kennedy et al. Citation1997; Panda et al. Citation1998). As recently as 2009, the teleomorphic state for A. flavus was discovered and reported to be Petromyces, which is the genus name given to all teleomorphs in section Flavi (Malloch and Cain Citation1972; Horn BW, Moore GG, et al. Citation2009; Horn BW, Ramirez-Prado JH, Citation2009, Citation2011).
Aspergillus fumigatus (SF2, SF4 and SF18) is taxonomically placed in subgenus Fumigati, section Fumigati. It is an airborne, saprophytic and filamentous fungus found mostly in hospitals, since it is an opportunistic pathogen of immunocompromised individuals (Latgé Citation1999). Aspergillus fumigatus is a leading cause of death among the rapidly expanding population of immunocompromised patients through chronic cavitary pulmonary aspergillosis and aspergilloma (Denning et al. Citation2003). Aspergillus infections of humans and animals have grown in importance over recent years; however, most of the studies have focused on A. fumigatus, the most prevalent clinical pathogen in the genus. This is because of the production of numerous small airborne conidia which can be dispersed by air movements. Atmosphere composition has a great impact on mould growth, with humidity being the most important variable (Gibson et al. Citation1994). Based on morphological characteristics, SF18 is very slow to sporulate, SF2 exhibits moderate sporulation and SF4 is an aggressive sporulator. The teleomorphic state for section Fumigati is known as Neosartorya, and this section's most recent teleomorph discovery, for A. fumigatus, is named Neosartorya fumigata (O'Gorman et al. Citation2009).
Aspergillus niger (SF15) is a ubiquitous filamentous ascomycete regarded as a member of the ‘black aspergilli’ group (subgenus Circumdati, section Nigri) due to the colour of its spores. It has been implicated in inciting opportunistic infections in humans, even though the US Food and Drug Administration generally regards it as safe (GRAS). It is also critical in industrial processing such as citric acid production and hydrolytic enzyme synthesis (Baker Citation2006). Although no sexual state has been determined for this species, the production of sclerotia gives potential for the formation of cleistothecia within a sclerotium-like stroma as with Petromyces (Geiser Citation2009).
Aspergillus terreus (SF8, SF10 and SF16) has been described as the only species in subgenus Nidulantes, section Terrei, with rare related taxa such as A. terreus var. aureus and A. terreus var. africanus. It is a medically difficult mould as it has been indicted in the formation of aspergilloma in humans, and there is a dearth of information regarding the mechanism of action for its resistance to amphotericin B (AMB) and its ability to cause infection (Balajee Citation2009). In fact, reports indicate that lower susceptibility to AMB is a means to distinguish between otherwise similar A. terreus and A. fumigatus infections (Baddley et al. Citation2003). It is industrially important because it produces lorastatin (Bizukojc and Ledakowicz Citation2010), a meviloninoic acid that can be used to synthesize itaconic acid which lowers serum cholesterol (López et al. Citation2005). Aspergillus terreus is also used as a metal biosorbent for the treatment of metallurgical effluents (Dias et al. Citation2002). Aspergillus terreus shares phylogenetic association with the teleomorphic genus Fennellia, but no sexual structures have been observed (Geiser Citation2009).
Penicillium minioluteum (SF11) is taxonomically placed in the subgenus Biverticillium, section Simplicium and series Miniolutea. Identification to species level is mostly based on macroscopic morphology and microscopic features according to de Hoog et al. (Citation2000). Nearly all penicillia are filamentous fungi; the only non-filamentous species is known as Pencillium marneffei which is thermally dimorphic and more commonly associated with infections of immunocompromised humans (Singh et al. Citation1999; Wong et al. Citation2001). Pencillium minioluteum is not considered pathogenic to humans, being more commonly found in soils and also in very damp environments as a biodeteriorant (Pitt Citation1979). Penicillium infections do occur in immunocompromised hosts, most times resulting in disease that is generically referred to as penicilliosis. Penicillium has been isolated from patients with keratitis, endophthalmitis, otomycosis, necrotizing esophagitis, pneumonia, endocarditis, peritonitis and urinary tract infections, with corneal infections being post-traumatic (Deshpande and Koppikar Citation1999). In addition to their infectious potential, some species of Penicillium are capable of producing mycotoxins (Pitt et al. Citation2000). For example, Penicillium verrucosum produces a mycotoxin, ochratoxin A, which is nephrotoxic and carcinogenic. The production of the toxin usually occurs in cereal grains in cold climates (Pitt Citation2000). Some Penicillium species have teleomorphs included in genera Eupenicillium, Talaromyces, Hamigera and Trichocoma (Pitt and Hocking Citation2009).
Fusarium semitectum (SF17) is taxonomically placed in section Arthrosporiella and can be either one of two varieties: var. semitectum or var. majus. Species within the genus Fusarium mostly cause plant diseases, but with the ability of certain species to secrete nephrotoxic mycotoxins such as trichothecenes, deoxynivalenol and fumonisins, Fusarium can also incite hyalohyphomycosis, or fusariosis, leading to morbidities in man and livestock (Dalcero et al. Citation1998; Meky et al. Citation2001; Awad et al. Citation2008). Teleomorph genera related to the Fusaria include Calonectria, Gibberella and Nectria, although research indicates that F. semitectum may be more closely associated with the teleomorph genus, Gibberella (Kerényi et al. Citation2004).
Trichoderma harzianum (SF14) is a fungal species, in the order Hypocreales and family Hypocreaceae, which has attained importance as a biocontrol agent over the years, favourably substituting for chemical pesticides. Trichoderma species may use different mechanisms for control of phytopathogens which include mycoparasitism, outcompeting pathogenic fungi for nutrients, secretion of antibiotics and/or secretion of fungal cell wall-degrading enzymes (Kubicek and Harman Citation1998; Harman et al. Citation2004; Reino et al. Citation2008). Trichoderma is very effective against some notable plant pathogens such as Pythium (Seidl et al. Citation2006). A correlation was established between different types of genotypes, enzyme production and biocontrol activity of Trichoderma when tested against Fusarium solani, Rhizoctonia solani, Fusarium oxysporum, Macrophomina phaseolina and Sclerotinia sclerotiorum (Haggag and Mohamed Citation2011). The teleomorphic genus for Trichoderma is known as Hypocrea (Samuels et al. Citation2011).
Mucor hiemalis f.sp. luteus (SF13) is a zygomycete in the order Mucorales and family Mucoraceae. The colonies grow very fast, starting cottony to fluffy, white to creamy, becoming blackish, which signifies the development of sporangia at 28°C. It is a filamentous fungus found in soil, plants or decaying fruits and vegetables. Mucor spp. may cause infections in man, frogs, amphibians, cattle and swine. In both immunocompromised and diabetic hosts, it incites a form of zygomycosis that results in the growth of hyphae within the brain, sinuses and lungs (Chandra and Woodgyer Citation2002). Invaded tissues have blood supply terminated (Ellis Citation1998). Most Mucor species are unable to grow at 37°C, so the strains isolated from human infections are usually one of few thermotolerant Mucor spp. (Larone Citation1995; Sutton et al. Citation1998; Stewart et al. Citation1999; de Hoog et al. Citation2000). A current anamorph–teleomorph connection is unknown for this species, since no sexual dimorphism has been reported (Schipper Citation1973).
Aspergillus flavus aflatoxin assay
It is not common for toxigenic A. flavus to express both aflatoxin and CPA (Gallagher et al. Citation1978; Cvetnic and Pepelnjak Citation1995). However, in this experiment, all A. flavus S- strain isolates (SF1, SF3, SF5, SF6 and SF9), sampled from different substrates, produced both aflatoxins B and G, as well as CPA ( and ). This result agrees with the recent report of Hua et al. (Citation2012) in which they found all aflatoxigenic isolates sampled, from pistachio orchards in California, produced both AFB1 and CPA. Isolate SF12 produces copious B aflatoxins and CPA, but no G aflatoxins, which is expected of L- strain A. flavus. shows the aflatoxin concentrations obtained for each isolate through HPLC analysis. Except for SF12, the other A. flavus isolates are G dominant, producing more than double the amount of G1 compared to B1 aflatoxins.
Table 2. Aflatoxin chemotype profiles for sampled Aspergillus flavus isolates
Figure 3. TLC plate showing the presence of CPA for each Aspergillus flavus isolate sampled. This is the same TLC plate as shown in , except that this plate has been sprayed with a mixture of 1% dimethyl-aminobenzaldehyde in ethanol, followed by sulphuric acid:ethanol (1:1), then drying the spray solution on the plate with a heat gun.
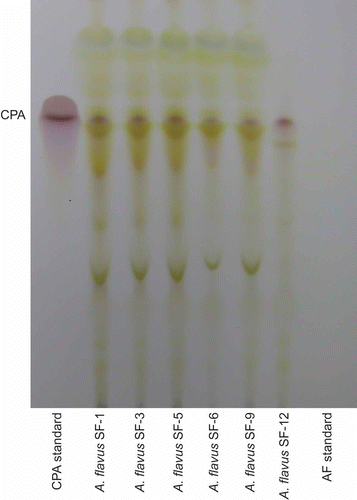
Figure 2. TLC plate showing fluorescence of B and G aflatoxins under long-wave UV illumination (365 nm). The aflatoxin bands are noted on the right side of the image. These bands correspond to the AF standard lane noted at the bottom right of the image. The CPA standard, which does not fluoresce under UV, is noted on the bottom left lane. Between both standard lanes are the Aspergillus flavus isolates sampled.
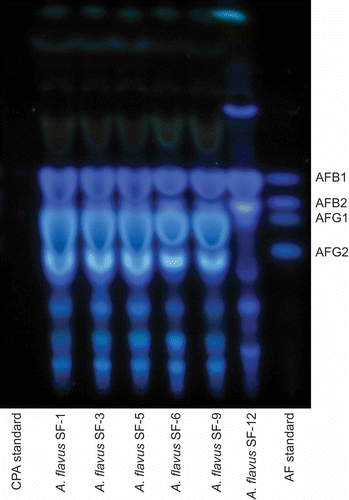
Molecular identification
All sequences have been deposited in GenBank under accession numbers JX442515–JX442537 (). BLAST analysis of the sequenced ITS region confirmed that isolates SF1, SF3, SF5, SF6, SF9 and SF12 are A. flavus, and their 210 bp sequences are identical since no polymorphisms were detected when aligned. Interestingly, BLAST analysis also returned 100% similarity to a section of the same sequence in Penicillium janthinellum, a species that produces secondary metabolites which happen to fluoresce, such as the aflatoxins, when exposed to UV light (Gallagher et al. Citation1980). The results of the norB-cypA investigation indicate that most of the A. flavus isolates (SF1, SF5, SF6 and SF9) are S- strains that produce amplicons of the same size (∼1800 bp) as A. parasiticus and A. nomius. This supports the TLC and HPLC toxin analyses which show these isolates producing both B and G aflatoxins (). Some researchers believe A. flavus-like strains that produce B and G aflatoxins to be Aspergillus minisclerotigenes (Pildain et al. Citation2008); however, we do not have sufficient data to make such a claim. Interestingly, one A. flavus isolate (SF3) has the toxin profile of a B and G producer, but the sequenced amplicon for its norB-cypA region suggests it is an S-strain that would produce only B aflatoxins (∼300 bp). Isolate SF12 has an amplicon band size of ∼1000 bp, supporting morphological identification as an L- strain as well as its chemotype identification as a B aflatoxin producer (). ITS BLAST results for SF2 and SF18 indicate they are teleomorphs from section Fumigati known as Neosartorya udagawae. Neosartorya udagawae, first isolated from soil in a Brazilian sugar cane field, is believed to be responsible for several cases of aspergillosis, but its pathogenicity is hindered by a lack of thermotolerance compared to A. fumigatus (Sugui et al. Citation2010). Isolate SF4 is BLAST confirmed A. fumigatus, and its 212 bp sequence shows three polymorphic sites when aligned with SF2 and SF18 (which have identical sequences). For isolates SF8, SF10 and SF16, their 214 bp sequence BLAST results confirm the isolates as A. terreus, but 100% similarity also exists for a species in a completely different section, Aspergillus tubingensis, which is affiliated with the black fungi (section Nigri) such as A. niger. All three sampled A. terreus isolates have identical sequences when aligned. When the ITS sequence was BLAST analysed for SF15, identity was confirmed as A. niger. This 211 bp sequence aligns with the A. terreus sequences, but there are many polymorphic sites that distinguish them. The 210 bp ITS sequence for SF11, which was morphologically identified as P. minioluteum, was confirmed through BLAST query, and 90% identity was shared with only one teleomorph genus, Talaromyces. SF17 was identified as F. semitectum based on its morphology, but according to its 300 bp ITS sequence the top BLAST results include multiple species within the genus Fusarium. However, Fusarium incarnatum shares this region with 100% sequence similarity, and this species name is considered synonymous with F. semitectum so its molecular identification is essentially confirmed. Fusarium incarnatum is a plant pathogen but is also seen as a potential human pathogen (Azor et al. Citation2009). The ITS sequence for SF17 shares 100% identity with a sequence from the teleomorph genus, Gibberella. SF14 was identified as T. harzianum through morphological characteristics, and BLAST results for the 224 bp ITS query reveal 99% identity with Hypocrea lixii. The genus name Hypocrea represents the teleomorphic state for Trichoderma (anamorph), and since both T. harzianum and H. lixii are considered synonymous organisms, morphological identity is confirmed. Finally, SF13 was identified as M. hiemalis f.sp. luteus based on morphology, but molecular comparison of its ITS region reveals only 92% identity to accessioned M. hiemalis ITS sequences. BLAST analysis of the 245 bp region amplified gave the top result of Rhizomucor variabilis being the identity of the organism with a maximum identity of 99%. Both are found in the same fungal family, but the organisms are not synonymous. Rhizomucor variabilis is a fungus capable of rare but serious mucormycoses in humans. Morphologically it is very similar to M. hiemalis, and phylogenetic investigations show this organism to be more closely related to Mucor spp. than species of Rhizomucor, so researchers are seeking to rename the organism as Mucor irregularis (Hemashettar et al. Citation2011).
Table 3. GenBank accession numbers for ITS and aflF-aflU sequences
The fact that highly toxigenic isolates of A. flavus (35%) were present among the sampled fungi further raises the alert on dangers from consumption of mouldy food and feed in southwest Nigeria. It is worth noting that most open-air vended agricultural products are exposed to poor hygiene, a condition that enhances mould growth, aflatoxin production and consumption with the attendant morbidity. Discoveries of three new aflatoxin-producing species belonging to Aspergillus section Flavi (Aspergillus mittae, Aspergillus sergii and Aspergillus transmontanensis), all in Portuguese almonds (Soares et al. Citation2012), lends credence to the possibility of active speciation for aflatoxigenic aspergilli, particularly in the tropics with high temperatures. This is critical to making Nigeria and other sub-Saharan African countries an endemic zone for aflatoxins, in spite of recent but isolated attempts at biological control (Abbas et al. Citation2011; Probst et al. Citation2011). The prevalence of A. flavus in the sampled materials is indicative of the level of danger an average consumer is exposed to in Nigeria. More concerted efforts need to be made to avail the local farmers and commodity traders with results of research efforts aimed at reducing the impact of aflatoxin contamination and consumption of items such as peanuts, maize, beans, spices and other agricultural products. Beyond aflatoxins, sampling of certain species such as A. flavus, A. fumigatus, F. incarnatum and R. variabilis that have clinical associations as potential human and animal pathogens is a serious matter to address. The fact that these organisms are sampled from areas affiliated with foods further supports the necessity for aggressive study and prevention of fungal contamination. By establishing a permanent culture collection centre, southwest Nigeria, as well as the rest of the country, could access isolates already identified using globally validated procedures, thus guaranteeing reliability in food and feed safety, disease control, industrial use and environmental quality control.
References
- Abbas , HK , Zablotwicz , RM , Bruns , HA and Abel , CA. 2006 . Biocontrol of aflatoxin in corn by inoculation with non-aflatoxigenic Aspergillus flavus isolates . Biocontrol Sci Technol , 16 ( 5 ) : 437 – 439 .
- Abbas , HK , Zablotwiocz , RM , Horn , BW , Phillips , NA , Johnson , J , Jin , X and Abel , CA. 2011 . Comparison of major biocontrol strains of non-aflatoxigenic Aspergillus flavus for the reduction of aflatoxins and cyclopiazonic acid in maize . Food Addit Contam Part A: Chem Anal Control Expo Risk Assess , 28 ( 2 ) : 198 – 205 .
- Awad , WA , Ghareeb , K , Böhm , J , Razzazi , EM , Hellweg , P and Zentek , J. 2008 . The impact of the Fusarium toxin deoxynivalenol (DON) on poultry . Int J Poultry Sci , 7 ( 9 ) : 827 – 842 .
- Azor , M , Gené , J , Cano , J , Manikandan , P , Venkatapathy , N and Guarro , J. 2009 . Less-frequent Fusarium species of clinical interest: correlation between morphological and molecular identification and antifungal susceptibility . J Clin Microbiol , 47 ( 5 ) : 1463 – 1468 .
- Baddley , JW , Pappus , PG , Smith , AC and Moser , SA. 2003 . Epidemiology of Aspergillus terreus at a university hospital . J Clin Microbiol , 41 ( 12 ) : 5525 – 5529 .
- Baker , SE. 2006 . Aspergillus niger genomics: past, present, and into the future . Med Mycol , 44 (Suppl. 1) : S17 – S21 .
- Balajee , SA. 2009 . Aspergillus terreus complex . Med Mycol , 47 (Suppl. 1) : S42 – S46 .
- Bandh , SA , Kamili , AN , Ganai , BA , Saleem , S , Lone , BA and Nissa , H. 2012 . First qualitative survey of filamentous fungi in Dal Lake, Kashmir . J Yeast Fungal Res , 3 ( 1 ) : 7 – 11 .
- Bennett , JW and Klich , M. 2003 . Mycotoxins . Clin Microbiol Rev , 16 ( 3 ) : 497 – 516 .
- Bizukojc , M and Ledakowicz , S. 2010 . The morphological and physiological evolution of Aspergillus terreus mycelium in the submerged culture and its relation to the formation of secondary metabolites . World J Microbiol Biotechnol , 26 ( 1 ) : 41 – 54 .
- Booth , C. 1977 . The genus Fusarium , Kew , England : Commonwealth Mycological Institute .
- Brown , RL , Cotty , PJ and Cleveland , TE. 1991 . Reduction in aflatoxin content of maize by atoxigenic strains of Aspergillus flavus . J Food Prot , 54 ( 8 ) : 623 – 626 .
- Chandra , S and Woodgyer , A. 2002 . Primary cutaneous zygomycosis due to Mucor circinelloides . Aust J Dermatol , 43 ( 1 ) : 39 – 42 .
- Cotty , PJ. 1989 . Virulence and cultural characteristics of two Aspergillus flavus strains pathogenic on cotton . Phytopathology , 79 ( 7 ) : 808 – 814 .
- Cvetnic , Z and Pepelnjak , S. 1995 . Aflatoxin-producing potential of Aspergillus flavus and Aspergillus parasiticus isolated from samples of smoke-dried meat . Nahrung , 39 ( 4 ) : 302 – 307 .
- Dalcero , A , Magnoli , C , Luna , M , Ancasi , G , Reynoso , MM , Chiacchiera , S , Miazzo , R and Palacio , G. 1998 . Mycoflora and naturally occurring mycotoxins in poultry feeds in Argentina . Mycopathologia , 141 ( 1 ) : 37 – 43 .
- de Hoog , GS , Guarro , J , Gené , J and Figueras , MJ. 2000 . Atlas of clinical fungi , 2nd , Vol. 1 , Utrecht , The Netherlands : Centraalbureau voor Schimmelcultures .
- Denning , DW , Riniotis , K , Dobrashian , R and Sambatakou , H. 2003 . Chronic cavitary and fibrosing pulmonary and pleural aspergillosis: case series, proposed nomenclature change, and review . Clin Infect Dis , 37 (Suppl. 3) : 265 – 280 .
- Deshpande , SD and Koppikar , GV. 1999 . A study of mycotic keratitis in Mumbai . Indian J Pathol Microbiol , 42 ( 1 ) : 81 – 87 .
- Dias , MA , Lacerda , IC , Pimentel , PF , De Castro , HF and Rosa , CA. 2002 . Removal of heavy metals by an Aspergillus terreus strain immobilized in a polyurethane matrix . Lett Appl Microbiol , 34 ( 1 ) : 46 – 50 .
- Diba , K , Kordbacheh , P , Mirhendi , SH , Rezaie , S and Mahmoudii , M. 2007 . Identification of Aspergillus species using morphological characteristics . Pak J Med Sci , 23 ( 6 ) : 867 – 872 .
- Domsch , KH , Gams , W and Anderson , T-H. 1993 . Compendium of soil fungi. Vol. 1 , Eching , Germany : IHW .
- Ehrlich , KC , Chang , P-K , Yu , J and Cotty , PJ. 2004 . Aflatoxin biosynthesis cluster gene cypA is required for G aflatoxin formation . Appl Environ Microbiol , 70 ( 11 ) : 6518 – 6524 .
- Ellis , DH. 1998 . “ Zygomycetes ” . In Topley and Wilson's microbiology and microbial infections , 9th , Edited by: Collier , L , Balows , A and Sussman , M . 247 – 277 . London , UK : Edward Arnold .
- Fapohunda , SO and Ogundero , VW. 1990 . Physiology of fungi associated with fast foods in Nigeria . Int Biodeterior , 26 ( 1 ) : 23 – 32 .
- Gallagher , RT , Latch , GCM and Keogh , RG. 1980 . The Janthitrems: fluorescent tremorgenic toxins produced by Penicillium janthinellum isolates from ryegrass pastures . Appl Environ Microbiol , 39 ( 1 ) : 272 – 273 .
- Gallagher , RT , Richard , JL , Stahr , HM and Cole , RJ. 1978 . Production of cyclopiazonic acid by aflatoxigenic and non-aflatoxigenic strains of Aspergillus flavus . Mycopathologia , 66 ( 1–2 ) : 31 – 36 .
- Geiser , DM. 2009 . Sexual structures in Aspergillus: morphology, importance and genomics . Med Mycol , 47 (Suppl. 1) : S21 – S26 .
- Gibson , AM , Baranyi , J , Pitt , JI , Eyles , MJ and Roberts , TA. 1994 . Predicting fungal growth: the effect of water activity on Aspergillus flavus and related species . Int J Food Microbiol , 23 ( 3–4 ) : 419 – 431 .
- Haggag , WM and Mohamed , MES. 2011 . Biodiversity, biological and molecular investigations of biocontrol by the genus Hypocrea/Trichoderma spp . Eur J Sci Res , 65 ( 2 ) : 281 – 292 .
- Harman , GE , Howell , CR , Viterbo , A , Chet , I and Lorito , M. 2004 . Trichoderma species: opportunistic, avirulent plant symbionts . Nat Rev Microbiol , 2 ( 1 ) : 43 – 56 .
- Hemashettar , BM , Patil , RN , O'Donnell , K , Chaturvedi , V , Ren , P and Padhye , AA. 2011 . Chronic rhinofacial mucormycosis caused by Mucor irregularis (Rhizomucor variabilis) in India . J Clin Microbiol , 49 ( 6 ) : 2372 – 2375 .
- Horn , BW , Moore , GG and Carbone , I. 2009 . Sexual reproduction in Aspergillus flavus . Mycologia , 101 ( 3 ) : 423 – 429 .
- Horn , BW , Moore , GG and Carbone , I. 2011 . Sexual reproduction in the aflatoxin-producing fungus Aspergillus nomius . Mycologia , 103 ( 1 ) : 174 – 183 .
- Horn , BW , Ramirez-Prado , JH and Carbone , I. 2009 . Sexual reproduction and recombination in the aflatoxin-producing fungus Aspergillus parasiticus . Fungal Genet Biol , 46 ( 2 ) : 169 – 175 .
- Hua , SST , Mcalpin , CE , Chang , P-K and Sarreal , SBL. 2012 . Characterization of aflatoxigenic and non-aflatoxigenic Aspergillus flavus isolates from pistachio . Mycotoxin Res , 28 ( 1 ) : 67 – 75 .
- Kennedy , CA , Adams , GL , Neglia , JP and Giebink , GS. 1997 . Impact of surgical treatment on paranasal fungal infections in bone marrow transplant patients . Otolaryngol Head Neck Surg , 116 (6, Part 1) : 610 – 616 .
- Kerényi , Z , Moretti , A , Waalwijk , C , Oláh , B and Hornok , L. 2004 . Mating type sequences in asexually reproducing Fusarium species . Appl Environ Microbiol , 70 ( 8 ) : 4419 – 4423 .
- Klich , MA. 2002 . Identification of common Aspergillus species , Utrecht , The Netherlands : Centraalbureau voor Schimmelcultures .
- Kubicek , CP and Harman , GE , eds. 1998 . Trichoderma and Gliocladium. Vol. 1, Basic biology, taxonomy and genetics , 278 London , , UK : Taylor & Francis .
- Larone , DH. 1995 . Medically important fungi – a guide to identification , 3rd , Washington , DC : ASM Press .
- Latgé , JP. 1999 . Aspergillus fumigatus and aspergillosis . Clin Microbiol Rev , 12 ( 2 ) : 310 – 350 .
- Li , Y. 2004 . Calorimetric method for the study of fungi on buildings materials [dissertation] , Lund , , [Lund(Sweden)] : Institute of Technology .
- López , JLC , Pérez , JAS , Sevilla , JMF , Porcel , EMR and Chisti , Y. 2005 . Pellet morphology, culture rheology and lovastatin production in cultures of Aspergillus terreus . J Biotechnol , 116 ( 1 ) : 61 – 77 .
- Malloch , DM and Cain , RF. 1972 . The Trichocomataceae: ascomycetes with Aspergillus, Paecilomyces, and Penicillium imperfect states . Can J Botany , 50 ( 12 ) : 2613 – 2628 .
- Meky , FA , Hardie , LJ , Evans , SW and Wild , CP. 2001 . Deoxynivalenol-induced immunomodulation of human lymphocyte proliferation and cytokine production . Food Chem Toxicol , 39 ( 8 ) : 827 – 836 .
- O'Gorman , CM , Fuller , HT and Dyer , PS. 2009 . Discovery of a sexual cycle in the opportunistic fungal pathogen Aspergillus fumigatus . Nature , 457 ( 7228 ) : 471 – 474 .
- Panda , NK , Sharma , SC , Chakrabarti , A and Mann , SB. 1998 . Paranasal sinus mycoses in north India . Mycoses , 41 ( 7–8 ) : 281 – 286 .
- Pildain , MB , Frisvad , JC , Vaamonde , G , Cabral , D , Varga , J and Samson , RA. 2008 . Two novel aflatoxin-producing Aspergillus species from Argentenian peanuts . Int J Syst Evol Microbiol , 58 ( Pt 3 ) : 725 – 735 .
- Pitt , JI. 1979 . The genus Penicillium and its teleomorphic states Eupenicillium and Talaromyces , 422 New York , NY : Academic Press .
- Pitt , JI. 2000 . A laboratory guide to common Penicillium species , North Ryde , NSW : Food Science Australia .
- Pitt , JI , Basílico , JC , Abarca , ML and López , C. 2000 . Mycotoxins and toxigenic fungi . Med Mycol , 38 (Suppl. 1) : S41 – S46 .
- Pitt , JI and Hocking , AD. 2009 . “ Penicillium and related genera ” . In Pitt JI, Hocking AD, editors. Fungi and food spoilage , 3rd , 519 New York , NY : Springer .
- Probst , C , Bandyopadhyay , R , Price , LE and Cotty , PJ. 2011 . Identification of atoxigenic Aspergillus flavus isolates to reduce aflatoxin contamination of maize in Kenya . Plant Dis , 95 ( 2 ) : 212 – 218 .
- Reino , JL , Guerrero , RF , Hernández-Galán , R and Collado , IG. 2008 . Secondary metabolites from species of the biocontrol agent Trichoderma . Phytochem Rev , 7 ( 1 ) : 89 – 123 .
- Rodrigues , P , Venâncio , A , Kozakiewicz , Z and Lima , N. 2009 . A polyphasic approach to the identification of aflatoxigenic and non‐aflatoxigenic strains of Aspergillus section Flavi isolated from Portuguese almonds . Int J Food Microbiol , 129 ( 2 ) : 187 – 193 .
- Samuels , GJ , Ismaiel , A , De Souza , J and Chaverri , P. 2011 . Trichoderma stromaticum and its overseas relatives . Mycol Progr , 11 ( 1 ) : 215 – 254 .
- Schipper , MAA. 1973 . A study on variability in Mucor hiemalis and related species . Studies Mycol , 4 : 1 – 40 .
- Seidl , V , Schmoll , M , Scherm , B , Balmas , V , Seiboth , B , Migheli , Q and Kubicek , CP. 2006 . Antagonism of Pythium blight of zucchini by Hypocrea jecorina does not require cellulase gene expression but is improved by carbon catabolite derepression . FEMS Microbiol Lett , 257 ( 1 ) : 145 – 151 .
- Sen , B and Asan , A. 2001 . Airborne fungi in vegetable growing areas of Edirne, Turkey . Aerobiologia , 17 ( 1 ) : 69 – 75 .
- Simsekli , Y , Gücin , F and Asan , A. 1999 . Isolation and identification of indoor airborne fungal contaminants of food production facilities and warehouses in Bursa, Turkey . Aerobiologia , 15 ( 3 ) : 225 – 231 .
- Singh , PN , Ranjana , K , Singh , YI , Singh , KP , Sharma , SS , Kulachandra , M , Nabakumar , Y , Chakrabarti , A , Padhye , AA and Kaufman , L . 1999 . Indigenous disseminated Penicillium marneffei infection in the state of Manipur, India: report of four autochthonous cases . J Clin Microbiol , 37 ( 8 ) : 2699 – 2702 .
- Soares , C , Rodrigues , P , Peterson , SW , Lima , N and Venâncio , A. 2012 . Three new species of Aspergillus section Flavi isolated from almonds and maize in Portugal . Mycologia , 104 ( 3 ) : 682 – 697 .
- Sobolov , VS and Dorner , JW. 2002 . Cleanup procedure for determination of aflatoxins in major agricultural commodities by liquid chromatography . J AOAC Int , 85 ( 3 ) : 642 – 645 .
- Stewart , NJ , Munday , BL and Hawkesford , T. 1999 . Isolation of Mucor circinelloides from a case of ulcerative mycosis of platypus (Ornithorhynchus anatinus), and a comparison of the response of Mucor circinelloides and Mucor amphibiorum to different culture temperatures . Med Mycol , 37 ( 3 ) : 201 – 206 .
- Sugui , JA , Vinh , DC , Nardone , G , Shea , YR , Chang , YC , Zelazny , AM , Marr , KA , Holland , SM and Kwon-Chung , KJ. 2010 . Neosartorya udagawae (Aspergillus udagawae), an emerging agent of Aspergillosis: how different is it from Aspergillus fumigatus? . J Clin Microbiol , 48 ( 1 ) : 220 – 228 .
- Sutton , DA , Fothergill , AW and Rinaldi , MG , eds. 1998 . Guide to clinically significant fungi , 1st , 471 Baltimore , MD : Williams & Wilkins .
- Verma , RJ. 2004 . Aflatoxin cause DNA damage . Int J Human Genet , 4 ( 4 ) : 231 – 236 .
- Vujanovic , V , Smoragiewicz , W and Krzysztyniak , K. 2001 . Airborne fungal ecological niche determination as one of the possibilities for indirect mycotoxin risk assessment in indoor air . Environ Toxicol , 16 ( 1 ) : 1 – 8 .
- Wong , SSY , Wong , KH , Hui , WT , Lee , SS , Lo , JYC , Cao , L and Yuen , KY. 2001 . Differences in clinical and laboratory diagnostic characteristics of Penicilliosis Marneffei in human immunodeficiency virus (HIV)- and non-HIV-infected patients . J Clin Microbiol , 39 ( 12 ) : 4535 – 4540 .