Candida species are common human fungal pathogens causing a wide range of clinical diseases, ranging from superficial infections to life-threatening systemic disease. Superficial infections include vaginal candidiasis which affects over 75% of women during their lifetime with 5% of women suffering debilitating recurrent infections.Citation1,Citation2 Life-threatening systemic Candida disease is the fourth most common nosocomial blood stream infection, affecting those undergoing chemotherapy, recovering from surgical procedures or major burns, transplant recipients and AIDs patients. The crude mortality rate associated with these infections is high, ranging from 46–75%, and current estimates suggest at least 400,000 life-threatening infections occur annually.Citation1,Citation3-6 Candida albicans is the predominant cause of invasive candidiasis, although in the last 3 decades there has been a rise in the incidence of non-albicans Candida species with Candida glabrata, Candida parapsilosis and Candida tropicalis being the other main agents causing disease. Of these, C. glabrata is the second most common cause of invasive candidiasis in the USA and Central and Northern Europe, and it has been associated with higher hospital costs.Citation5,Citation7-9 The basis of this increasing incidence of C. glabrata is not fully understood, however, it could be partially attributed to the higher innate tolerance C. glabrata displays to azole antifungals alongside its greater potential to develop drug resistance coincident with therapy.Citation10,Citation11
Murine models of infection are typically viewed as the gold standard for fungal virulence studies. However, although these models allow the host-pathogen interaction to be studied in vivo they do come with caveats associated with cost, legislation, and careful ethical considerations. Furthermore, with the development of large scale mutant libraries alternative, more ethically acceptable, models are required to identify interesting virulence targets while limiting the use of mice.Citation12,Citation13 Given the caveats associated with murine models of infection mini-host models, mainly invertebrates, have been explored as alternative models for fungal infection. These models include amoeba (Dictyostellium discoideum), nematodes (Caenorhabditis elegans), fruit fly (Drosophila melanogaster) and the greater wax moth larvae (Galleria mellonella).Citation14-16 G. mellonella, a lepidopteran, was first described as a mini-host for Candida species by Kavanagh and coworkers,Citation17,Citation18 and has received particular attention as an alternative host as it displays some important advantages. The G. mellonella larvae can be incubated at 37°C, allowing virulence to be studied at human body temperature, and an exact inoculum of the pathogen can be delivered by injection. Furthermore, the assays are inexpensive and simple to perform, allowing large numbers of larvae to be infected and thus increasing the statistical power of the assay. Finally some aspects of the G. mellonella immune response show similarities with the innate immune response of mammals.Citation14,Citation15,Citation19 Given these advantages the model has now been developed for a wide range of fungal pathogens, including several Candida species.Citation17,Citation18,Citation20-22
To study C. glabrata infection in mice immunosuppression is usually required, and fungal burdens and persistence are normally used as a parameter for virulence due to the absence of mortality.Citation23 This, along with the recent development of large scale mutant libraries, makes alternative models for studying C. glabrata virulence an attractive proposition. Initial reports on establishing the G. mellonella model for testing the virulence of Candida species only reported a low level of killing of larvae by C. glabrata.Citation18,Citation24,Citation25 However, we and othersCitation26,Citation27 have now shown that a faster rate of killing by C. glabrata is seen when using a higher pathogen concentration. In this work we provide the first detailed report on the ability of C. glabrata to grow and cause lethal infections in G. mellonella in a dose dependent manner. Furthermore, we have shown that this model can be used to assess the relative virulence of C. glabrata clinical isolates, and that the analysis of mutant strains demonstrates an overlap with results published using murine infection models. Finally, we have shown that antifungal efficacy in the G. mellonella model correlates with the in vitro susceptibility profile of C. glabrata. Therefore, the G. mellonella model can be used to study both C. glabrata virulence and antifungal efficacy.
To evaluate G. mellonella as a host model for C. glabrata infection we first infected larvae with the commonly used wild type reference strain ATCC200128 at a range of different inoculum levels (7.5 × 105, 1 × 106, 2.5 × 106, 5 × 106 and 7.5 × 106 cells/larva). For this, groups of 20 healthy larvae (0.25–0.35 g) were inoculated with 10 µl of cell suspension through injection into the haemocoel with a Hamilton syringe through the last left pro-leg. Following infection larvae were incubated in the dark at 37°C and survival, based on response to physical stimulation, was monitored daily for 7 d. Larvae inoculated with PBS were used as uninfected controls and resulted in no deaths (data not shown), and all assays were performed at least 3 times independently. The results from this clearly demonstrated that C. glabrata can kill the larvae in a dose dependent fashion (). For example, infection with 2.5 × 106 cells/larva gave a mean survival time of 3.45 ± 0.28 d compared with 1.63 ± 0.13 d with an infective dose of 7.5 × 106 cells/larva (P < 0.0001). The infective dose required for C. glabrata to kill G. mellonella larvae was however approximately 10-fold higher than the dose of C. albicans required to cause death, where an infective dose of 2 × 105 cells/larva C. albicans NGY15229 cells resulted in a mean survival time of 2.95 ± 0.21 d (data not shown) similar to previous reports.Citation17,Citation18,Citation22 Similar findings have been seen with other Candida species,Citation20-22 and for C. glabrata is perhaps in keeping with its differing virulence properties favoring stealth and evasion over aggressive invasion.Citation23,Citation30 Given the high dose of C. glabrata required to cause G. mellonella killing we also evaluated the survival of larvae inoculated with heat-killed yeast cells (incubated at 75°C for 20 min before use), to ensure that killing was not due to other factors such as toxic shock. No larval death was seen following infection with heat-killed cells at 5 × 106 cells/larva (data not shown); therefore the killing of larvae in this model is dependent on viable C. glabrata cells.
Figure 1. Virulence of C. glabrata in G. mellonella. (A and B) Survival curves of G. mellonella infected with C. glabrata ATCC2001 at 7.5 × 105 (squares), 1 × 106 (triangles), 2.5 × 106 (upside-down triangles), 5 × 106 (diamonds) and 7.5 × 106 (circles) cells/larva at 37°C (A) and 30°C (B). At 37°C (A) all infective doses displayed significant differences (p < 0.05), whereas at 30°C (B) all doses displayed significant differences (p < 0.05) except between the 2 lowest infective doses (7.5 × 105 and 1 × 106 cells/larva). (C) Larvae were infected with C. glabrata ATCC2001 at 1 × 106, 2.5 × 106, and 5 × 106 cells/larva and at 2 and 6 hours post infection hemolymph was collected from larvae and OD405 determined to quantify melanin production. Larvae inoculated with PBS were used as a control, and asterisks denote a statistically significant difference to the PBS control (p < 0.05). (D) G. mellonella were infected with C. glabrata ATCC2001 at 2.5 × 106 cells/larva and fungal burdens determined at the time points indicated. Scatterplots depict combined results from 3 independent replicates using 9 larvae for each time point
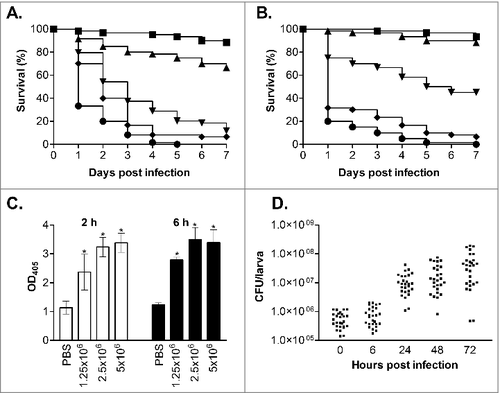
Temperature has been seen to impact on the outcome of G. mellonella infection for some fungal species, with both C. albicans and C. tropicalis displaying faster killing following incubation at 37°C rather than 30°C whereas killing rates following C. krusei infection were not affected by temperature.Citation21,Citation22,Citation31 To determine if temperature also impacts on the virulence of C. glabrata we compared the virulence of C. glabrata ATCC2001 at 30 and 37°C. At the intermediate infective doses of both 1 × 106 and 2.5 × 106 cells/larva C. glabrata virulence was significantly decreased at 30°C compared with 37°C (, P < 0.005). For example, with the 2.5 × 106 cells/larva dose the mean survival time at 30°C was 4.65 ± 0.33 d compared with 3.45 ± 0.28 d at 37°C. However, no significant impact of temperature was seen when higher infective doses were used, suggesting this limitation can be overcome. The increased mortality seen at higher temperatures with intermediate infective doses may be the result of the impact of temperature on both the growth and virulence properties of the pathogen, plus the effect of temperature on the immune response of the larvae.Citation32
Following infection with C. glabrata larvae quickly developed a brown-black colouration, indicative of the accumulation of melanin as part of the insect innate immune response. To quantify this hemolymph was collected from infected larvae, at 2 and 6 hours post infection, following established methods.Citation33 The extent of melanisation was then determined through measuring the hemolymph optical density at 405 nm, which correlates with its visual appearance and has been used previously to quantify laccase activity.Citation22 Through this the extent of melanisation was seen to be dependent on both the infective dose of C. glabrata and time post infection (). In addition to the activation of melanisation, previous work has demonstrated that the density of free hemocytes in the hemolymph is decreased following infection with C. albicans and other microbes.Citation21,Citation22,Citation24 This decrease was seen to correlate with susceptibility to infection, and is thought to be the result of nodulation or clumping of hemocytes, pathogens and melanised debris at the infection site.Citation19 Following infection with C. glabrata, at 2.5 × 106 cells/larva, we also saw a significant decrease in total hemocyte cell density, with levels falling from 8.5 × 106 ± 2.0 × 106 cells/ml for PBS inoculated control larvae to 4.6 × 106 ± 1.2 × 106 (54%; p < 0.01) and 2.7 × 106 ± 9.7 × 105 (33%; p < 0.005) at 2 and 6 hours post infection respectively. Overall therefore, with the activation of melanisation and the drop in free hemocyte density, it is clear that the larvae mount a defense response following C. glabrata infection.
To follow the progress of infection we also determined the fungal burdens in G. mellonella, at 0, 6, 24, 48 and 72 h post infection, following inoculation with 2.5 × 106 cells/larva C. glabrata ATCC2001. For this 9 larvae were taken at each time point, briefly washed in 70% ethanol followed by sterile water, and then placed into 15 ml screw-cap tubes with 4 stainless steel balls (3 mm) and 1 ml PBS. The tissue was then homogenized through 3 rounds of shaking for 20 s at 4 m/s in a Fastprep-24 (MP Biomedicals). The resulting homogenate was then suspended in 14 ml PBS and serial dilutions prepared and inoculated onto YEPD-chloramphenicol (100 µg/ml) plates. Immediately following infection the detectable fungal burden was 5.8 × 105 ± 3.4 × 105 CFU/larvae, and initially remained fairly constant only reaching 8.5 × 105 ± 6 × 105 CFU/larvae at 6 h post infection. However, by 24 h, when larvae began to succumb to infection, fungal burdens had risen ∼20-fold to 1.2 × 107 ± 9.4 × 106 CFU/larvae and continued to rise at 48 and 72 h post infection (), demonstrating growth of the C. glabrata in the host.
In addition to testing the ability of C. glabrata ATCC2001 to cause lethal infection in G. mellonella we also screened a further 5 clinical isolates (BG2, Cg1184, Cg85/038, Cg11088A and NCPF3605),Citation34-37 including the other commonly used isolate BG2, at 3 infective doses (1.25 × 106, 2.5 × 106 and 5 × 106 cells/larva; ). All strains demonstrated a dose dependent response, and 4 of these strains (BG2, Cg1184, Cg85/038 and Cg11088A) demonstrated a very similar level of virulence to ATCC2001. Indeed when comparing the different infective doses the only significant difference was the slight increase in virulence seen with strain Cg1184 at the 5 × 106 cells/larva dose compared with BG2 (P < 0.005) and Cg11088A (P < 0.05). Therefore the majority of C. glabrata isolates tested show broadly similar levels of virulence in this model. The only key exception was the NCPF3605 strain which, at all infective doses tested, was clearly highly attenuated in virulence compared with all the other isolates (P < 0.0001) only causing 22.5% ± 9.6% killing of larvae by 7 d at the highest dose tested. A recent comparison of the growth of this strain and ATCC2001, at varying glucose concentrations, demonstrated distinct differences, with NCPF3605 displaying a faster growth rate but at the cost of entering stationary phase at a lower cell density.Citation38 General fitness defects may therefore impact on the virulence of C. glabrata in this model and, importantly, the G. mellonella model can be used to detect differences in the virulence potential of C. glabrata strains.
Figure 2. Virulence of C. glabrata clinical isolates in G. mellonella. Survival curves of G. mellonella infected with C. glabrata ATCC2001 (open squares), NCPF3605 (closed squares), BG2 (triangles), Cg1184 (upside-down triangles), Cg85/038 (diamonds), and Cg11088A (circles) at 1 × 106 (A), 2.5 × 106 (B), and 5 × 106 (C) cells/larva. At all doses strain NCPF3605 was highly attenuated compared with all other strains (p < 0.0001); plus at the highest dose (C) a slight but significant increase in virulence was seen for Cg1184 compared with BG2 (p < 0.005) and Cg11088A (p < 0.05)
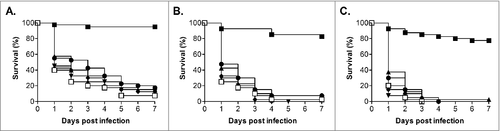
Auxotrophic markers are commonly used for selection during the genetic modification of Candida species. However, in C. albicans it is well recognized that ura3 auxotrophy causes virulence to be highly attenuated in the mouse model of infection, and that the level of expression of URA3 can also impact on virulence.Citation29,Citation39 In this work we have demonstrated that ura3 auxotrophy has a similar impact on the virulence of C. glabrata in the G. mellonella infection model. G. mellonella infected with 2. 5 × 106 cells/larva of wild type C. glabrata (ATCC2001) demonstrated a mean survival time of 2.90 ± 0.19 d compared with 6.39 ± 0.13 d (p < 0.0001) for those infected with a ura3 auxotroph (strain 2001UCitation40). Given the known issues with ura3 auxotrophy in C. albicans, most work in C. glabrata uses other auxotrophic markers that have been shown not to impact on virulence in mice.Citation23,Citation41 We therefore also screened his3, leu2 and trp1 single mutants (strains 2001H, 2001L and 2001T respectively) and a his3, leu2, trp1 triple mutant (strain 2001HTL).Citation41 Similar to the situation in a mouse model, loss of HIS3 or LEU2 did not impact on microbial virulence within the G. mellonella model (data not shown). However, in our model we did detect a subtle, but significant, attenuation of virulence in the trp1 single (mean survival time 3.67 ± 0.20 days, p = 0.0173) and his3, leu2, trp1 triple null mutant (mean survival time 3.87 ± 0.20 days, p = 0.0020) compared with the wild type strain ATCC2001 (mean survival time of 2.90 ± 0.19 days). It is tempting to attribute the lower virulence of the triple mutant to its loss of trp1; however, as we did not test this empirically we cannot rule out the possibility that the combined auxotrophies also impact on fitness and ultimately virulence. Overall, we would therefore suggest that when using this model it is important to ensure appropriate control strains, displaying the same auxotrophies, are used.
To assess the utility of this model to screen defined mutants for virulence defects we also performed virulence assays with 14 deletion mutants, including 8 whose phenotype in a mouse model of infection has previously been reported. These included 12 mutants associated with C. glabrata stress responses (Δste50, Δste20, Δhog1, Δskn7, Δyap1, Δcta1, Δrim101, Δyps1, Δmsn2, Δmsn4, Δcst6 and Δslt2)Citation41-43 plus 2 glycosylation mutants (Δmnn2 and Δanp1)Citation44 that have previously been shown to be hypervirulent in mice. To increase the statistical power of the assay we used 50 larvae per replicate, and selected one infective dose (2.5 × 106 cells/larva) which would allow either an increase or decrease in virulence potential to be observed. Virulence was then compared with the relevant wild type strain (2001HTL, 2001H or HT6Citation41,43), selected based on the genetic background of the mutant. Through this analysis we identified 5 mutants that demonstrated a mild but significant attenuation in virulence, including Δste50, Δste20, Δhog1 in the Hog pathway, Δslt2 in the cell wall integrity pathway and Δskn7 involved in oxidative stress resistance (). The level of attenuation in these mutants was generally subtle, but shown to be significant through the benefit of being able to infect a large number of larvae to increase the statistical power of the assay. Of these mutants 4, Δste20, Δhog1, Δslt2 and Δskn7, have previously been tested in a mouse model of systemic infection where they also displayed a mild (Δste20, Δslt2 and Δskn7) to moderate (Δhog1) attenuation of virulence.Citation45-48 For STE50 this is the first report of its importance in virulence, and the phenotype displayed by the Δste50 mutant is in keeping with the overall importance of the Hog1 pathway. Furthermore, the large scale analysis of C. glabrata mutants in the Drosophila infection modelCitation13 also identified both the Hog1 and cell wall integrity pathways as playing a key role in virulence, therefore the importance of these pathways has been consistently demonstrated in 3 different infection models. The remaining mutants (Δyap1, Δcta1, Δrim101, Δyps1, Δmsn2, Δmsn4 and Δcst6; ) displayed no significant defect in virulence, and of these 2 (Δyap1 and Δcta1) have also previously been reported to display no virulence defect in mice.Citation49,Citation50 In addition to the stress response mutants we also screened 2 glycosylation mutants (Δmnn2 and Δanp1)Citation44 that have previously been shown to demonstrate increased virulence in a mouse model of infection. Intriguingly, these deletion mutants also displayed increased virulence in the G. mellonella model while their complemented strains demonstrated wild type virulence (). The molecular basis of this hypervirulence is currently not clear, but the mutants are known to be hyperadherent and potentially elicit a septic-shock like response.Citation44 This may therefore suggest that either similar components are involved in the recognition of the pathogen by G. mellonella or common adhesins play a role in both models. Overall, of the 14 mutants tested in this study 8 have previously been screened in mice and we have shown all to display comparable phenotypes in the G. mellonella model, plus confirm the importance of the Hog1 and cell wall integrity pathways in virulence. This correlation is very encouraging and suggests that this model has the potential to be used to screen for novel virulence factors in C. glabrata.
Table 1. Virulence of C. glabrata mutants in the G. mellonella model
To facilitate the future use of this model for comparing mutant strains, potentially through large scale screening efforts, we also calculated a larval virulence index (LVI) as a measure of virulence for the set of 14 mutants tested. For this we followed the methodology established for use with the Drosophila infection model.Citation13,Citation41 Survival curves were initially fitted to a Weibull distribution, then the time of 50% larval survival (LT50) determined and from this the LVI presented as the log2 ratio of mutant and corresponding wild type control (). The LT50 values determined for the different strains were, as expected, in very strong agreement with their mean survival times (Spearman's rho = 0.99, P < 0.00001). Furthermore, applying the cut-offs established by Brunke et al.Citation13 for increased or decreased virulence (virulence index ± 0.5), 6 of the 7 mutants we identified as displaying altered virulence through the traditional log rank tests were also highlighted by this approach. The mutant not highlighted, Δste20, was on the verge of detection with a LVI of −0.46 and was also the least attenuated in virulence through the traditional log rank test. Overall, therefore, this modeling approach gives a strong quantitative measure of virulence, and may facilitate the future use of this model in the large scale screening of available C. glabrata deletion libraries.Citation41
Finally, we also tested the efficacy of fluconazole, amphotericin B, and caspofungin against C. glabrata in the G. mellonella model and compared this to C. albicans. C. glabrata is well recognized for displaying a higher innate tolerance to azole antifungals than C. albicans.Citation10,Citation11 Consistent with this, although C. albicans NGY152 was highly sensitive to fluconazole in vitro the C. glabrata strain ATCC2001 displayed a high MIC of 32 µg/ml, whereas for caspofungin and amphotericin B both were acutely sensitive. We next tested the efficacy of these antifungals in the G. mellonella model at clinically relevant doses that did not cause toxicity in the model (data not shown). For this, larvae were infected with 1 × 105 cells/larva C. albicans NGY152 or 1.25 × 106 cells/larva C. glabrata ATCC2001 and antifungals administered 30 min post infection through a second 10 µl injection into the pro-leg adjacent to the site of initial infection. Untreated controls received a second injection of PBS. For G. mellonella infected with C. albicans, treatment with fluconazole at all concentrations tested (3, 6, and 12 mg/kg) promoted survival (; P < 0.0001). In contrast treatment with the same levels of fluconazole provided no protection against C. glabrata infection (). Amphotericin B at both 2 and 4 mg/kg also protected larvae against infection by C. albicans (; P < 0.0001), whereas only the highest concentration (4 mg/kg) provided significant protection against C. glabrata (; P < 0.0001). Finally, caspofungin also protected G. mellonella against C. albicans infection at all concentrations tested (, 1 mg/kg P < 0.005; 2 and 4 mg/kg P < 0.0001), whereas again only the higher doses of 2 and 4 mg/kg provided significant protection against C. glabrata infection (, P < 0.0001). We therefore saw a clear correlation between in vitro susceptibility and in vivo efficacy in this model for C. glabrata, consistent with previous studies demonstrating the potential of the G. mellonella model to be used in testing the toxicity and efficacy of antifungal agents for a range of fungal pathogens.Citation21,Citation22,Citation31,Citation51,Citation52 Interestingly, although amphotericin B and caspofungin did provide protection against C. glabrata, in both cases a higher dose of antifungal was required to achieve efficacy than for treatment of C. albicans. A similar profile was seen following infection with C. krusei and this was associated with the strain tested, although being susceptible, demonstrating reduced susceptibility compared with the C. albicans control strain.Citation22 In this work however, the C. glabrata and C. albicans strains used demonstrated very similar susceptibility profiles toward amphotericin B and caspofungin. The basis of this subtle but consistent in vivo susceptibility shift is therefore not clear. It could simply be due to the requirement of a tenfold higher infective dose for C. glabrata compared with C. albicans, which may result in the need for a higher drug concentration, or alternatively it could be suggestive of C. glabrata displaying a higher in vivo tolerance toward antifungals.
Figure 3. Antifungal efficacy against C. albicans and C. glabrata in the G. mellonella model. Survival curves of G. mellonella infected with 1 × 105 cells/larva C. albicans NGY152 (A, C and E), or 1.25 × 106 cells/larva C. glabrata ATCC2001 (B, D and F). (A and B) Fluconazole treatment at 0 (squares), 3 (triangle), 6 (diamonds) or 12 mg/kg (circles). (C, D, E and F) Amphotericin B or Caspofungin treatment at 0 (squares), 1 (triangle), 2 (diamonds) or 4 mg/kg (circles)
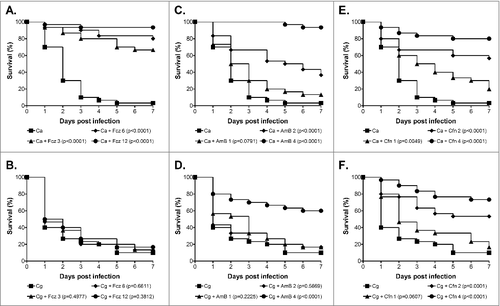
Infection models using G. mellonella are generally gaining acceptance and have now been established for a range of fungal pathogens. As previously discussed these models present some advantages through being more ethically acceptable, inexpensive allowing the use of more test subjects to increase the statistical power of the assay, alongside the easy manipulation of larvae and ability to assay at 37°C. There are however some disadvantages such as no complete genome sequence and the lack of genetic tractability in G. mellonella, plus an inherent level of variability in the quality of larvae from suppliers. Finally, as with any infection model, it is unlikely that all virulence attributes involved in mammalian infection will demonstrate similar importance in the G. mellonella system. Overall however we would conclude that G. mellonella is an attractive and simple model for following C. glabrata infection. High doses are initially required to cause an infection, but once established a simple to follow lethal infection coupled with growth of the pathogen and a detectable host response is seen. Furthermore, through the mutants tested, we saw a good level of correlation with murine models suggesting that this system has the potential to be used to screen for novel virulence factors in this important pathogen. Finally, as has been seen with other fungal pathogens, this system can clearly be used for the in vivo evaluation of antifungal agents.
Disclosure of potential conflicts of interest
No potential conflicts of interest were disclosed.
Acknowledgments
We thank Sukrit Silas (Stanford University) for providing information on the application of the modeling approach.
Funding
This work was supported in part by the Wellcome Trust Strategic Award for Medical Mycology and Fungal Immunology 097377/Z/11/Z.
References
- Brown GD, Denning DW, Gow NA, Levitz SM, Netea MG, White TC. Hidden killers: human fungal infections. Sci Transl Med 2012; 4:165rv13; PMID:23253612; https://doi.org/https://doi.org/10.1126/scitranslmed.3004404
- Sobel JD. Vulvovaginal candidosis. Lancet 2007; 369:1961-71; PMID:17560449; https://doi.org/https://doi.org/10.1016/S0140-6736(07)60917-9
- Leroy O, Gangneux JP, Montravers P, Mira JP, Gouin F, Sollet JP, Carlet J, Reynes J, Rosenheim M, Regnier B, et al. Epidemiology, management, and risk factors for death of invasive Candida infections in critical care: a multicenter, prospective, observational study in France (2005-2006). Crit Care Med 2009; 37:1612-8; PMID:19325476; https://doi.org/https://doi.org/10.1097/CCM.0b013e31819efac0
- Perlroth J, Choi B, Spellberg B. Nosocomial fungal infections: epidemiology, diagnosis, and treatment. Med Mycol 2007; 45:321-46; PMID:17510856; https://doi.org/https://doi.org/10.1080/13693780701218689
- Pfaller M, Neofytos D, Diekema D, Azie N, Meier-Kriesche HU, Quan SP, Horn D. Epidemiology and outcomes of candidemia in 3648 patients: data from the Prospective Antifungal Therapy (PATH Alliance(R)) registry, 2004–2008. Diagn Microbiol Infect Dis 2012; 74:323-31; PMID:23102556; https://doi.org/https://doi.org/10.1016/j.diagmicrobio.2012.10.003
- Pfaller MA, Diekema DJ. Epidemiology of invasive mycoses in North America. Crit Rev Microbiol 2010; 36:1-53; PMID:20088682; https://doi.org/https://doi.org/10.3109/10408410903241444
- Diekema D, Arbefeville S, Boyken L, Kroeger J, Pfaller M. The changing epidemiology of healthcare-associated candidemia over three decades. Diagn Microbiol Infect Dis 2012; 73:45-8; PMID:22578938; https://doi.org/https://doi.org/10.1016/j.diagmicrobio.2012.02.001
- Moran C, Grussemeyer CA, Spalding JR, Benjamin DK, Jr, Reed SD. Comparison of costs, length of stay, and mortality associated with Candida glabrata and Candida albicans bloodstream infections. Am J Infect Control 2010; 38:78-80; PMID:19836856; https://doi.org/https://doi.org/10.1016/j.ajic.2009.06.014
- Quindos G. Epidemiology of candidaemia and invasive candidiasis. A changing face. Rev Iberoam Micol 2014; 31:42-8; PMID:24270071; https://doi.org/https://doi.org/10.1016/j.riam.2013.10.001
- Pfaller MA, Diekema DJ. Epidemiology of invasive candidiasis: a persistent public health problem. Clin Microbiol Rev 2007; 20:133-63; PMID:17223626; https://doi.org/https://doi.org/10.1128/CMR.00029-06
- Pfaller MA, Diekema DJ, Gibbs DL, Newell VA, Barton R, Bijie H, Bille J, Chang SC, da Luz Martins M, Duse A, et al. Geographic variation in the frequency of isolation and fluconazole and voriconazole susceptibilities of Candida glabrata: an assessment from the ARTEMIS DISK Global Antifungal Surveillance program. Diagn Microbiol Infect Dis 2010; 67:162-71; PMID:20338711; https://doi.org/https://doi.org/10.1016/j.diagmicrobio.2010.01.002
- Amorim-Vaz S, Delarze E, Ischer F, Sanglard D, Coste AT. Examining the virulence of Candida albicans transcription factor mutants using Galleria mellonella and mouse infection models. Front Microbiol 2015; 6:367; PMID:25999923; https://doi.org/https://doi.org/10.3389/fmicb.2015.00367
- Brunke S, Quintin J, Kasper L, Jacobsen ID, Richter ME, Hiller E, Schwarzmuller T, d'Enfert C, Kuchler K, Rupp S, et al. Of mice, flies–and men? Comparing fungal infection models for large-scale screening efforts. Dis Model Mech 2015; 8:473-86; PMID:25786415; https://doi.org/https://doi.org/10.1242/dmm.019901
- Chamilos G, Lionakis MS, Lewis RE, Kontoyiannis DP. Role of mini-host models in the study of medically important fungi. Lancet Infect Dis 2007; 7:42-55; PMID:17182343; https://doi.org/https://doi.org/10.1016/S1473-3099(06)70686-7
- Desalermos A, Fuchs BB, Mylonakis E. Selecting an invertebrate model host for the study of fungal pathogenesis. PLoS Pathog 2012; 8:e1002451; PMID:22319439; https://doi.org/https://doi.org/10.1371/journal.ppat.1002451
- Maccallum DM. Hosting infection: experimental models to assay Candida virulence. Int J Microbiol 2012; 2012:363764; PMID:22235206; https://doi.org/https://doi.org/10.1155/2012/363764
- Brennan M, Thomas DY, Whiteway M, Kavanagh K. Correlation between virulence of Candida albicans mutants in mice and Galleria mellonella larvae. FEMS Immunol Med Microbiol 2002; 34:153-7; PMID:12381467; https://doi.org/https://doi.org/10.1111/j.1574-695X.2002.tb00617.x
- Cotter G, Doyle S, Kavanagh K. Development of an insect model for the in vivo pathogenicity testing of yeasts. FEMS Immunol Med Microbiol 2000; 27:163-9; PMID:10640612; https://doi.org/https://doi.org/10.1111/j.1574-695X.2000.tb01427.x
- Browne N, Heelan M, Kavanagh K. An analysis of the structural and functional similarities of insect hemocytes and mammalian phagocytes. Virulence 2013; 4:597-603; PMID:23921374; https://doi.org/https://doi.org/10.4161/viru.25906
- Gago S, Garcia-Rodas R, Cuesta I, Mellado E, Alastruey-Izquierdo A. Candida parapsilosis, Candida orthopsilosis, and Candida metapsilosis virulence in the non-conventional host Galleria mellonella. Virulence 2014; 5:278-85; PMID:24193303; https://doi.org/https://doi.org/10.4161/viru.26973
- Mesa-Arango AC, Forastiero A, Bernal-Martinez L, Cuenca-Estrella M, Mellado E, Zaragoza O. The non-mammalian host Galleria mellonella can be used to study the virulence of the fungal pathogen Candida tropicalis and the efficacy of antifungal drugs during infection by this pathogenic yeast. Med Mycol 2013; 51:461-72; PMID:23170962; https://doi.org/https://doi.org/10.3109/13693786.2012.737031
- Scorzoni L, de Lucas MP, Mesa-Arango AC, Fusco-Almeida AM, Lozano E, Cuenca-Estrella M, Mendes-Giannini MJ, Zaragoza O. Antifungal efficacy during Candida krusei infection in non-conventional models correlates with the yeast in vitro susceptibility profile. PLoS One 2013; 8:e60047; PMID:23555877; https://doi.org/https://doi.org/10.1371/journal.pone.0060047
- Jacobsen ID, Brunke S, Seider K, Schwarzmuller T, Firon A, d'Enfert C, Kuchler K, Hube B. Candida glabrata persistence in mice does not depend on host immunosuppression and is unaffected by fungal amino acid auxotrophy. Infect Immun 2010; 78:1066-77; PMID:20008535; https://doi.org/https://doi.org/10.1128/IAI.01244-09
- Bergin D, Brennan M, Kavanagh K. Fluctuations in haemocyte density and microbial load may be used as indicators of fungal pathogenicity in larvae of Galleria mellonella. Microbes Infect 2003; 5:1389-95; PMID:14670452; https://doi.org/https://doi.org/10.1016/j.micinf.2003.09.019
- Junqueira JC, Fuchs BB, Muhammed M, Coleman JJ, Suleiman JM, Vilela SF, Costa AC, Rasteiro VM, Jorge AO, Mylonakis E. Oral Candida albicans isolates from HIV-positive individuals have similar in vitro biofilm-forming ability and pathogenicity as invasive Candida isolates. BMC Microbiol 2011; 11:247; PMID:22053894; https://doi.org/https://doi.org/10.1186/1471-2180-11-247
- Borghi E, Andreoni S, Cirasola D, Ricucci V, Sciota R, Morace G. Antifungal resistance does not necessarily affect Candida glabrata fitness. J Chemother 2014; 26:32-6; PMID:24091025; https://doi.org/https://doi.org/10.1179/1973947813Y.0000000100
- Santos R, Costa C, Mil-Homens D, Romao D, de Carvalho CC, Pais P, Mira NP, Fialho AM, Teixeira MC. The multidrug resistance transporters CgTpo1_1 and CgTpo1_2 play a role in virulence and biofilm formation in the human pathogen Candida glabrata. Cell Microbiol 2016; 19:e12686; https://doi.org/https://doi.org/10.1111/cmi.12686
- Dujon B, Sherman D, Fischer G, Durrens P, Casaregola S, Lafontaine I, De Montigny J, Marck C, Neuveglise C, Talla E, et al. Genome evolution in yeasts. Nature 2004; 430:35-44; PMID:15229592; https://doi.org/https://doi.org/10.1038/nature02579
- Brand A, MacCallum DM, Brown AJ, Gow NA, Odds FC. Ectopic expression of URA3 can influence the virulence phenotypes and proteome of Candida albicans but can be overcome by targeted reintegration of URA3 at the RPS10 locus. Eukaryot Cell 2004; 3:900-9; PMID:15302823; https://doi.org/https://doi.org/10.1128/EC.3.4.900-909.2004
- Brunke S, Hube B. Two unlike cousins: Candida albicans and C. glabrata infection strategies. Cell Microbiol 2013; 15:701-8; PMID:23253282; https://doi.org/https://doi.org/10.1111/cmi.12091
- Mylonakis E, Moreno R, El Khoury JB, Idnurm A, Heitman J, Calderwood SB, Ausubel FM, Diener A. Galleria mellonella as a model system to study Cryptococcus neoformans pathogenesis. Infect Immun 2005; 73:3842-50; PMID:15972469; https://doi.org/https://doi.org/10.1128/IAI.73.7.3842-3850.2005
- Kavanagh K, Reeves EP. Exploiting the potential of insects for in vivo pathogenicity testing of microbial pathogens. FEMS Microbiol Rev 2004; 28:101-12; PMID:14975532; https://doi.org/https://doi.org/10.1016/j.femsre.2003.09.002
- Fuchs BB, O'Brien E, Khoury JB, Mylonakis E. Methods for using Galleria mellonella as a model host to study fungal pathogenesis. Virulence 2010; 1:475-82; PMID:21178491; https://doi.org/https://doi.org/10.4161/viru.1.6.12985
- Cormack BP, Falkow S. Efficient homologous and illegitimate recombination in the opportunistic yeast pathogen Candida glabrata. Genetics 1999; 151:979-87; PMID:10049916
- Haynes KA, Westerneng TJ. Rapid identification of Candida albicans, C. glabrata, C. parapsilosis and C. krusei by species-specific PCR of large subunit ribosomal DNA. J Med Microbiol 1996; 44:390-6; PMID:8636954; https://doi.org/https://doi.org/10.1099/00222615-44-5-390
- Haynes KA, Westerneng TJ, Fell JW, Moens W. Rapid detection and identification of pathogenic fungi by polymerase chain reaction amplification of large subunit ribosomal DNA. J Med Vet Mycol 1995; 33:319-25; PMID:8544085; https://doi.org/https://doi.org/10.1080/02681219580000641
- Zhou PB, Thiele DJ. Isolation of a metal-activated transcription factor gene from Candida glabrata by complementation in Saccharomyces cerevisiae. Proc Natl Acad Sci U S A 1991; 88:6112-6; PMID:2068090; https://doi.org/https://doi.org/10.1073/pnas.88.14.6112
- Reding-Roman C, Hewlett M, Duxbury S, Gori F, Gudelj I, Beardmore R. The unconstrained evolution of fast and efficient antibiotic-resistant bacterial genomes. Nat Ecol Evol 2017; 1:0050; https://doi.org/https://doi.org/10.1038/s41559-016-0050
- Staab JF, Sundstrom P. URA3 as a selectable marker for disruption and virulence assessment of Candida albicans genes. Trends Microbiol 2003; 11:69-73; PMID:12598128; https://doi.org/https://doi.org/10.1016/S0966-842X(02)00029-X
- Kitada K, Yamaguchi E, Arisawa M. Cloning of the Candida glabrata TRP1 and HIS3 genes, and construction of their disruptant strains by sequential integrative transformation. Gene 1995; 165:203-6; PMID:8522176; https://doi.org/https://doi.org/10.1016/0378-1119(95)00552-H
- Schwarzmuller T, Ma B, Hiller E, Istel F, Tscherner M, Brunke S, Ames L, Firon A, Green B, Cabral V, et al. Systematic phenotyping of a large-scale Candida glabrata deletion collection reveals novel antifungal tolerance genes. PLoS Pathog 2014; 10:e1004211; PMID:24945925; https://doi.org/https://doi.org/10.1371/journal.ppat.1004211
- Roetzer A, Klopf E, Gratz N, Marcet-Houben M, Hiller E, Rupp S, Gabaldon T, Kovarik P, Schuller C. Regulation of Candida glabrata oxidative stress resistance is adapted to host environment. FEBS Lett 2011; 585:319-27; PMID:21156173; https://doi.org/https://doi.org/10.1016/j.febslet.2010.12.006
- Weig M, Haynes K, Rogers TR, Kurzai O, Frosch M, Muhlschlegel FA. A GAS-like gene family in the pathogenic fungus Candida glabrata. Microbiology 2001; 147:2007-19; PMID:11495979; https://doi.org/https://doi.org/10.1099/00221287-147-8-2007
- West L, Lowman DW, Mora-Montes HM, Grubb S, Murdoch C, Thornhill MH, Gow NA, Williams D, Haynes K. Differential virulence of Candida glabrata glycosylation mutants. J Biol Chem 2013; 288:22006-18; PMID:23720756; https://doi.org/https://doi.org/10.1074/jbc.M113.478743
- Calcagno AM, Bignell E, Rogers TR, Canedo M, Muhlschlegel FA, Haynes K. Candida glabrata Ste20 is involved in maintaining cell wall integrity and adaptation to hypertonic stress, and is required for wild-type levels of virulence. Yeast 2004; 21:557-68; PMID:15164359; https://doi.org/https://doi.org/10.1002/yea.1125
- Miyazaki T, Inamine T, Yamauchi S, Nagayoshi Y, Saijo T, Izumikawa K, Seki M, Kakeya H, Yamamoto Y, Yanagihara K, et al. Role of the Slt2 mitogen-activated protein kinase pathway in cell wall integrity and virulence in Candida glabrata. FEMS Yeast Res 2010; 10:343-52; PMID:20214686; https://doi.org/https://doi.org/10.1111/j.1567-1364.2010.00611.x
- Saijo T, Miyazaki T, Izumikawa K, Mihara T, Takazono T, Kosai K, Imamura Y, Seki M, Kakeya H, Yamamoto Y, et al. Skn7p is involved in oxidative stress response and virulence of Candida glabrata. Mycopathologia 2010; 169:81-90; PMID:19693686; https://doi.org/https://doi.org/10.1007/s11046-009-9233-5
- Srivastava VK, Suneetha KJ, Kaur R. The mitogen-activated protein kinase CgHog1 is required for iron homeostasis, adherence and virulence in Candida glabrata. FEBS J 2015; 282:2142-66; PMID:25772226; https://doi.org/https://doi.org/10.1111/febs.13264
- Chen KH, Miyazaki T, Tsai HF, Bennett JE. The bZip transcription factor Cgap1p is involved in multidrug resistance and required for activation of multidrug transporter gene CgFLR1 in Candida glabrata. Gene 2007; 386:63-72; PMID:17046176; https://doi.org/https://doi.org/10.1016/j.gene.2006.08.010
- Cuellar-Cruz M, Briones-Martin-del-Campo M, Canas-Villamar I, Montalvo-Arredondo J, Riego-Ruiz L, Castano I, De Las Penas A. High resistance to oxidative stress in the fungal pathogen Candida glabrata is mediated by a single catalase, Cta1p, and is controlled by the transcription factors Yap1p, Skn7p, Msn2p, and Msn4p. Eukaryot Cell 2008; 7:814-25; PMID:18375620; https://doi.org/https://doi.org/10.1128/EC.00011-08
- Kelly J, Kavanagh K. Caspofungin primes the immune response of the larvae of Galleria mellonella and induces a non-specific antimicrobial response. J Med Microbiol 2011; 60:189-96; PMID:20947665; https://doi.org/https://doi.org/10.1099/jmm.0.025494-0
- Li DD, Deng L, Hu GH, Zhao LX, Hu DD, Jiang YY, Wang Y. Using Galleria mellonella-Candida albicans infection model to evaluate antifungal agents. Biol Pharm Bull 2013; 36:1482-7; PMID:23995660; https://doi.org/https://doi.org/10.1248/bpb.b13-00270