ABSTRACT
This study aimed to investigate in vitro and in vivo the probiotic characteristics of lactic acid bacteria (LAB) isolated from Korean traditional fermented foods. Caenorhabditis elegans (C. elegans) was used for analytical assays of fertility, chemotaxis, life-span, worm-killing and bacterial colonization in the intestinal lumen of the worm. All 35 strains of LAB reduced fertility and slowed development in the worms. The worm-killing assay showed that LAB significantly increased the lifespan (P < 0.05) and reduced the susceptibility to virulent PA14; however, the heat-killed LAB did not. The bacterial colonization assay revealed that LAB proliferated and protected the gut of the worm against infection by Pseudomonas aeruginosa PA14. In addition, specific LAB Pediococcus acidilactici(P. acidilactici DM-9), Pediococcus brevis (L. brevis SDL1411), and Pediococcus pentosaceus (P. pentosaceus SDL1409) strains showed acid resistance (66–91%), resistance to pepsin (64–67%) and viability in simulated intestinal fluid (67–73%) based on in vitro probiotic analyses. Taken together, these results suggest that C. elegans may be a tractable model for screening efficient probiotics.
Introduction
Caenorhabditis elegans are free-living nematodes that reside in the soil and rotten fruits, where they feed on bacteria [Citation1,Citation2]. When used for in vitro studies, they are normally cultured on a lawn of Escherichia coli (E. coli OP50) on which they feed, leading to the presence of bacterial cells in pharynx and the intestine, which frequently appears distended; it has been widely applied as an experimental system for biological studies due to its transparency, short reproductive cycle, ease of cultivation, and suitability for genetic analysis [Citation2].
C. elegans has been used as a model animal in a variety of experiments, including susceptibility testing for antibiotics and human pathogen experiments, because of numerous advantages over other experimental animal models. In probiotic-based research, there are few studies using non-mammalian hosts. Studies on the nematode C. elegans have previously indicated that isolates of both Bifidobacterium and Lactobacillus spp. are beneficial to the health and lifespan of the worm [Citation3,Citation4]; feeding C. elegans with Lactobacillus has been proven to protect against the foodborne pathogens Enterococcus faecalis and Staphylococcus aureus [Citation5]. Furthermore, symbiotic lactobacilli appear to be involved in intestinal development.
Lactic acid bacteria (LAB) are rod shaped non-spore-forming gram-positive, microaerophiles and facultative anaerobes bacteria [Citation6]. The enhancement of life span based on probiotics were first proclaimed by Metchnikoff who conveyed that Bulgarian farmers practiced in consuming fermented milk with Lactobacilli experienced improved health and longevity, which leads to antiaging effect [Citation7]. Recently, several research groups were focused on finding novel Lactobacillus based probiotics and the functional properties as a potential probiotic candidates [Citation8,Citation9]. Unfortunately, studies on the enhancing life span and defense against specific pathogens based on the influence of probiotics are limited because of a lack of appropriate experimental models to validate host longevity [Citation10]. The Mechanisms underlying the probiotic effects of Lactic acid bacteria are also unclear. In this respect, studies on C. elegans have delivered valuable perceptions into the functional aspects of anti-aging and defense against specific pathogen (P. aeruginosa) and have led to the exploration of the multiple genetic pathways that determine longevity [Citation11,Citation12]. In addition, lactic acid bacteria are widely used and produced commercially, and they are mainly consumed in the form of fermented foods or dairy products [Citation13]. These products have a positive impact on the immune system and overall health. LAB can elicit various immune responses by affecting specific receptors in the intestinal tract or immune cells of the host [Citation6,Citation7].
Pseudomonas aeruginosa is an opportunistic Gram-negative pathogen that infects immunocompromized individuals, for instance patients with cancer or AIDS, or patients with cystic fibrosis [Citation14,Citation15]. The pathogenic factors of P. aeruginosa mainly based on lipopolysaccharide (LPS), flagella endotoxin and extracellular toxin [Citation16]. Most of the Gram-positive pathogens depends on, lipoteichoic acid, which induce pro-inflammatory cytokines mediates by Toll-like receptor 4 is a protein (TLR-2) [Citation17,Citation18].But Non-pathogenic Gram-positive bacteria, such Pediococcus, Enterococcus, Lactobacilli, persuade a dissimilar pattern of cytokines in peripheral blood cells[Citation19–Citation21]. The mechanism of lactobacillus mainly based on inhibition of peritoneal macrophage apoptosis by infection with Salmonella typhimurium [Citation22], studies on invitro and invio have reported that Lactobacilli pocess antagonistic effects against urinary tract infecting (UTI) pathogens [Citation23,Citation24].
Doenjang (Korean soy bean paste) is a traditional Korean food made from fermented soybeans. Doenjang has many health-friendly ingredients, including anti-carcinogenic flavonoids and other beneficial vitamins, minerals, and phytoestrogens [Citation25]. Moreover, the soybeans used for making soybean paste contain linoleic acid and linolenic acid, which have positive effects on cardiovascular diseases and contain a high level of lysine, which is one of the essential amino acids. Thus, this food is known as nutritional source [Citation26].
Lactic Acid Bacteria was isolated and identified from Korean soy bean paste further screened for in-vitro probiotics characteristics. Further the C. elegans model was applied to study the effects of lactic acid bacteria against Pseudomonas aeruginosa PA14. Specifically, we aimed to study the effects of LAB on the reproductive ability, colonization and life span of C. elegans.
Materials and methods
Bacterial strains and growth media
A total of 35 indigenous LAB (Lactic acid bacteria) strains () isolated from the Korean fermented soybean paste, were provided by Soonchang Jang Ryu Saupso Company-Korea. Based on different colony morphology using MRS (de Man, Rogosa and Sharpe) selective media and further identified on the bases of 16S rRNA sequencing using universal primers (27F-5ʹ AGA GTT TGA TCM TGG CTC AG 3ʹ and 1492R-5ʹ GGT TAC CTT GTT ACG ACT 3ʹ). The LAB, Pseudomonas aeruginosa PA14 and Escherichia coli OP50 bacterial strains were stored at – 80°C in De Man, Rogosa & Sharpe (MRS, BD Biosciences, San Jose, CA, USA) medium. Trypticase soy broth (TSB, BD Biosciences, San Jose, CA, USA) was used to cultivate Pseudomonas aeruginosa PA14 and Escherichia coli OP50, which were obtained from the US Food Fermentation Laboratory Culture Collection (Wyndmoor, PA., USA) supplemented with 30% glycerol. Each culture was streaked from frozen stocks onto TSA and MRS (BD Biosciences) and incubated at 37°C for 24 h.
Table 1. Bacterial strains used in this study All 35 LAB (lactic acid bacterial) strains were isolated from Korean fermented soybean paste.
Screening for probiotic properties in LAB
Tolerance to low pH
The tolerance to low pH of selected lactic acid bacterial strains was determined using the method described by Mokoena [Citation27], with some modifications. Briefly, the active strains grown in MRS broth were inoculated (1.5%) in 10 ml of fresh MRS broth, incubated at 37°C for 24 h and then centrifuged at 6000 × g for 15 min. The pellets were suspended in sterile phosphate-buffered saline (PBS; GIBCO, USA) containing 9 g/L NaCl (Sigma-Aldrich, South Korea), 9 g/L Na2HPO4⋅2H2O and 1.5 g/L KH2PO4 adjusted to a pH of 2.5. The mixture were incubated at 37°C for 4 h, and samples were taken at time 0 and after 4 h. These samples were serially diluted in sterile saline (0.85% NaCl) solution and plated on MRS agar; the viable cells were determined after incubation at 37°C for 24 h, and the percentage of survival of the bacteria was calculated as follows: % survival = [CFU of viable cells survived/CFU of initial viable cells inoculated] x 100.
Tolerance to pepsin
To test the bacterial tolerance to pepsin, a simulated gastric juice was prepared by aadding 3 mg/mL pepsin (Sigma-Aldrich, South Korea) to sterile saline solution (0.85% NaCl, w/v) and adjusting the pH to 2.5. The fluid was inoculated with active cultures at an inoculum size of 1% (v/v) and incubated at 37°C for 4 h. The viable cells were determined at an initial (T1) and final incubation time (T2) by the spread plate method by Oh et al [Citation28]. The percentage survival of the bacteria was calculated as follows: % survival = [CFU of viable cells survived/CFU of initial viable cells inoculated x 100].
Resistance to bile salts and pancreatin
The resistance of the lactobacilli to bile salts and pancreatin was tested as described by Oh et al [Citation28], with some modifications. Briefly, 0.3% (w/v) bile salts (Sigma-Aldrich, South Korea) and 1 mg/mL pancreatin (Sigma-Aldrich, South Korea) were dissolved in sterile saline solution (0.85% NaCl, w/v) adjusted to pH 8.0. The fluid was inoculated with 1% (v/v) LAB cultures and incubated at 37°C for 6 h. The viable cells were determined before and after incubation by the spread plate method. The percentage survival of the bacteria was calculated as follows: % survival = [CFU of survivors/CFU of inoculum x 100].
Determination of antibiotic susceptibility
The susceptibility of the lactic acid bacteria towards antibiotics was tested as reported earlier by Oh et al [Citation28]. All the antibiotics were purchased from Sigma Inc.-USA. The lactic acid bacteria were tested against the cell wall-targeting antibiotics 10 μg ampicillin (Am), 10 μg penicillin (Pe) and 30 μg vancomycin (Va); the protein synthesis-inhibiting antibiotics 15 μg erythromycin (Er), 2 μg clindamycin (Cl), 30 μg tetracycline (Te), 30 μg kanamycin (Ka), 30 μg chloramphenicol (Ch) and 10 μg gentamicin (Ge); and the nucleic acid-targeting antibiotic 25 μg streptomycin (St) using the disc diffusion method. In addition 0.005mg/mL. The MRS agar plates were examined for the zones of inhibition after incubation at 37°C for 24 h.
Antibacterial effects of LAB isolates in vitro
The antibacterial effects of eight selected LAB isolates on six common food borne pathogens or spoilage organism’s at 37°C were investigated using the agar diffusion assay described above. Targeted indicator organisms and their respective media used were as follows: P. aeruginosa (PA14) (Nutrient Broth (NB), DifcoTM), L. brevis SDL1411 (L05), P. acidilatici DM9 (L21) and P. pentosaceus SDL1409 (L33), Enterococcus faecium CK-5 (L03) Enterococcus faecium SC54 (L02), Lactobacillus plantatrum JDFM44 (L09), Leuconstoc citreum SC53 (L12), Pediococcus acidilatic SDL1414 (L17), Pediococcus acidilatic DM9 (L21), Pediococcus pentosaceus MAC11 (L22), Weissella koreensis JBNU2 (L31) (MRS, DifcoTM). All strains were cultured aerobically for 24 h at 37°C, from which Cell free supernatant (CFS) was prepared and tested against P. aeruginosa (PA14). Following inoculation, plates were incubated at 37°C for 24 h, respectively. Inhibition zones were measured.
Thermal stability of antimicrobial peptides produced by LAB isolates
Based on identified LAB species, isolation source and the size of inhibition zones, nine LAB isolates were chosen for thermal stability tests. The pH adjusted CFS described above were treated at 90°C for both 10 and 30 min. pH adjusted CFS without any heat treatments served as a controls. Residual antimicrobial activity of heat-treated CFS was determined by the agar diffusion bioassay compared to the control using P. aeruginosa (PA14) as the indicator bacteria.
Digestive enzyme stability of antimicrobial peptides produced by LAB isolates
Based on identified LAB species, isolation source and the size of inhibition zones, nine LAB isolates were chosen for enzyme stability tests. The pH adjusted CFS with < 10Kda (Abcam ab-93,349) peptide separated using spin column, which were treated at 3 mg/mL pepsin + (pH 2.5) and incubated at 37°C for 4 h, 1 mg/mL bile + pancreatin+ (pH 8.0) and incubated at 37°C for 6 h. The pH adjusted proteins were freeze dried, without any treatments served as a controls. Residual antimicrobial activity of enzyme – treated CFS was determined by the agar diffusion bioassay compared to the control using P. aeruginosa (PA14) as the indicator bacteria.
Nitric oxide production in Lactobacillus
Qualitative analysis
In addition Lactobacillus (L5, L21 and L33) biosynthesis of Nitric Oxide were evaluated by staining with 1, 2-diaminoantraquinone sulfate (DAAD11582-5G), 50 μg/ml; at 37°C for 30 min) subsequently the bacteria washed from stained observed in confocal microscope [Citation30]. DAA is a colored compound with a maximum of absorption at about 540 nm (fluorescence images obtained using 488 nm and emission measured between 505 and 750 nm – green channel) (Sup.1).
Quantitative analysis
(d) Modified MRS broth (Sharpe.” to test for the production of Nitric oxide from arginine by lactobacilli): MRS broth+ 0–3% (w/v) L-argininc monohydrochloride. After 12 and 24 h incubation at 37 o C likewise during 3rd, 6th and 9th day of C. elegens treated with Lactobacillus (L5, L21 and L33). The concentration of NO in sodium nitroprusside solutions was estimated by calibration curve plotted with the use of fresh solutions of NaNO2 (Sigma Aldrich 237,213) in concentrations of 0–100 μM. Nitrites, NO oxidation products under aerobic conditions, were measured spectrophotometrically (540 nm) using Griess reagent (Sigma-Aldrich G4410) [Citation31].
Nematode and stage of development
The experiment were executed mainly based on [Citation27]. The eggs from strain N2 C. elegans were isolated and treated with hypochlorite, and further incubated 20oC for 20 h in S-buffer [Citation28]. The nematodes were maintained on 35 mm diameter NGM (Nematode Growing Medium) agar plates seeded with 50 μL E. coli OP50 and stored at 20 o C. C. elegans (N2) strains were obtained from the Caenorhabditis Genetic Center (CGC), Minnesota. A harmonized population of L1 arrested worms were then placed on NGM agar plates seeded with LAB. Five stage [L1 – after egg 5 stage of egg development and immediately after hatching Nervous, Reproductive and coelomocyte system developmental stage; L2- few cell division after L1 stage leads to possess developed reproductive and nervous system (L2 stage also known as dauer larva; L3- posse matured reproductive system; L4- young adult posse fully developed reproductive system and Adult- hence completing its 3-day reproductive life cycle] [Citation29]. In that L4 nematode were picked manually and moved to a new plate. The worms were then moved twice a day to prevent overcrowding until egg laying ceased. The progeny were counted 3 days after removal of the parents. The experiment was performed in triplicate.
Nematode synchronizing
The young adult worms hatched from eggs were treated with sterile water containing 0.5 M NaOH and 0.5% bleaching solution (sodium hypochlorite and sodium hydroxide) for synchronization. The assay was performed as described by Mokoena [Citation30]. After vortex mixing at 2 min intervals, worms were washed three times in M9 buffer (3.0 g KH2PO4, 6.0 g Na2HPO4, 0.5 g NaCl, 1.0 g NH4Cl, H2O/1 L, sterilized at 121°C) via centrifugation (1200 × g for 200 sec). To prepare worms for all assays, approximately 3000 synchronized eggs were hatched in M9 buffer at 20°C overnight. The L1 larvae were subsequently transferred to nematode growth medium (NGM) agar with a lawn of E. coli OP50 and incubated at 25°C for 48 h to reach the L4 stage.
Chemotaxis assay
The chemotaxis assay was adapted from Martin et al., 2017. Briefly, the bacteria (10 µl of OP50) were seeded separately with worms at the initial growth (L4) stage after incubation. Fifty young adult stage worms were washed twice with S-basal buffer and placed in the centre of a (5 cm) plate wherein 20 µl of 0.5 M sodium azide solution had been added to two sites on the plate with a circle marked around the origin to ensure that non-motile worms would be ignored. A two-quadrant system was used to monitor the movement of the worms, which were equidistant from the test and control samples, regardless of which side of the origin they began. In this system, the worms respond to an odorant, which can delay or force them to take a more circuitous route. The number of worms at both sites was checked after 90 min at 25°C and then incubated further at 4°C. Chemotaxis assays were performed in triplicate for each condition. For each experiment, a chemotaxis index (CI) was calculated using the following formula:
Chemotaxis index (CI) = [number of worms in the LAB bacteria strain – number of worms in OP50 (control)/total number of worms applied]. A + 1.0 score indicates maximal attraction towards the target and represents 100% of the worms arriving in the quadrants containing the chemical target. An index of −1.0 is evidence of maximal repulsion.
C. elegans fertility assay
L4 young adult (N2) worms were transferred to new NGM plates containing 50 μL of the target bacterial strain cultured in TS and MRS broth. Worms were transferred daily until they no longer produced progeny. The NGM plates containing eggs were incubated at 20°C for another 48 h, and the number of progeny was counted for each adult worm tested at the end of 3 days. The fertility of worms was monitored using wild-type L2 [Citation31].
Lifespan assays
The longevity of C. elegans was measured using a previously described method by Smolentsevae et al [Citation32]. In the experiment, 50 μL of the target bacterial strain cultured in TS and MRS broth at 25°C for 24 h was spread on a 35 mm diameter NGM agar plate and incubated overnight at 37°C. Each plate was seeded with 30 L4 stage young adults of C. elegans grown in E. coli OP50. All plates were incubated at 25°C and enumerated for dead worms every 24 h. Live worms were transferred onto fresh NGM agar plates seeded with the target bacterial strain every 2 days. The experiment was performed in triplicate for each strain. Each plate was monitored until all the worms had died. To compare the effects of Pseudomonas aeruginosa PA14 alone to the effects of LAB () against Pseudomonas aeruginosa PA14, worms were first grown with OP50, and the mean life span (MLS) was calculated by applying a previously described formula [Citation33].
Bacterial colonization assay
The influence of selected Lactobacillus strains on colonization in C. elegans was determined using the bacterial colonization assay described by Antunes et al [Citation35], with some modifications. On the first day, lactic acid bacteria were seeded on NGM plates containing worms. On the 3rd, 5th, 7th, and 9th day of incubation, the total number of colonies present in the gut of the young adult (L4) stage worm was determined using the following procedure: Five worms were washed in 5 μl drops of M9 buffer to inhibit pharyngeal pumping and expulsion. The washed nematodes were placed in a 1.5 ml centrifuge tube containing 50 μl of PBS buffer with 1% Triton X-100 and mechanically disrupted using a mortar and pestle. Worm lysates were then diluted in PBS buffer and plated on MRS agar at 37°C. LAB colonies were quantified and used to calculate the number of bacteria per nematode. The procedure was repeated using P. aeruginosa PA14 as a comparison with lactic acid bacteria [Citation35].
Life span assay of C. elegens with heat killed bacteria
Initially E. coli OP50 fed C. elegens, were subsequently infected with P. aeruginosa PA14, which results in a combination of OP50-PA14: worms were transferred onto PA14-seeded NGM plate after 1st day of incubation in OP50-seeded NGM plate and enumerate dead worms each day to 10th day. OP50-PA14; secondly worms were transferred onto PA14-seeded NGM plate after 1st day of incubation, further seeded with heat-killed (80°C for 10 min) E. coli OP50 in NGM plate and dead worms were enumerate each day till 10th day. Likewise the Lactic acid bacteria (The LAB was selected for the experiment based on the colonization assay) seeded NGM plates were then seeded with PA14, from 1st day – 10th day of incubation the life span of the worm were enumerated, in addition the heat killed LAB were tested similarly against PA14. Each experiment for each strain administrated 30 worms and repeated twice and ploted the results using OASIS II program
Statistical analysis
Results are reported as the mean of at least 2 independent experiments ± standard error (s.e.m). In the life span assay and the killing assay, the OASIS2 (Online Application for Survival Analysis 2) program was used for statistical analysis [Citation38]. Differences in the survival of C. elegans in the infection assays were determined using Graph Pad Prism version 5.00 [Citation39] (www.graphpad.com).
Results
In vitro probiotic properties of LAB
Among the 35 total lactic acid bacterial strains, based on the lifespan assay, a drastic reduction in the survival ability of LAB in the C. elegans model with respect to the pathogen P. aeruginosa PA14 could indicate less chances of surviving further harsh conditions [Citation40]. However, only 3 selective isolates (L. brevis, P. latici and P. pentosaceus) showed promising results; hence, these three strains were further tested for resistance against acid (pH 2.5), pepsin (pH 2.5), Bile + Pancreatin (pH 8.0), antibiotics resistance with different mode of action and antimicrobial activity (, ).
Table 2. Acid resistance of lactic acid bacteria in phosphate-saline buffer (pH 2.5). Values are expressed in mean ± standard deviation (n = 3) Different superscripts (a, b, c, d, e, f, g, h, i, j, k, l, m, n, o, p, q, r, s, t, u) represent significantly different values (P < 0.05) .
Table 3. Probiotic characteristics of the selected Lactic acid bacterial strain.
Acid tolerance
All the LAB isolates were subjected to a 2.5 pH. Among the 35 LAB were presented in (), Lactobacillus brevis SDL1411 (L05) showed > 90% survival abilities in low pH, Enterococcus faecium SC54 (L12), Lactobacillus plantatrum JDFM44 (L09), Leuconstoc citreum SC53 (L12), Pediococcus acidilatic SDL1414 (L17), Pediococcus acidilatic DM9 (L21), Pediococcus pentosaceus MAC11 (L22), Weissella koreensis JBNU2 (L31), Pediococcus pentosaceus SDL1409 (L33) exhibited 60–75%, Enterococcus faecalis MAD-13 (L01), Enterococcus faecium CK-5 (L03), Enterococcus lactis SCL1421 (L04), Lactobacillus brevis SDL1408 (L06), Lactobacillus curvatus JBNU38 (L07), Lactobacillus pentosus SC48 (L08), Lactobacillus rhamnosus JDFM33 (L10), Lactobacillus rhamnosus JDFM6 (L11), Leuconstoc mesenteroides JBNU10 (L13), Leuconstoc paramesenteroides SC46 (L14), Pediococcus acidilatic SCL1420 (L15), Pediococcus acidilatic SKL1418 (L16), Pediococcus acidilatic SDL1406 (L18), Pediococcus acidilatic SDL1405(L19), Pediococcus acidilatic SDL1402(L20), Pediococcus pentosaceus SDL1416 (L23), Pediococcus pentosaceus SDL1415 (L24), Pediococcus pentosaceus SDL1401(L25), Streptococcus thermophiles SCML337 (L26), Streptococcus thermophiles SCML300 (L27), Weissella cibaria SCCB2306 (L28), Weissella confuse SCSB2320 (L29), Weissella confuse SCKB2318 (L30). The strains showing < 50% () survival capability were omitted from further analysis. Since the extreme decline in their survival ability could specify lesser chances of surviving further harsh conditions [Citation30]. However, the remaining 9 strains were tested for their resistance against pepsin.
Resistance to pepsin
Nine LAB were tested for their resistance to pepsin. Our results showed that L. brevis SDL1411 (L05), P. acidilatici DM9 (L21) and P. pentosaceus SDL1409 (L33) showed the highest survival ability in pepsin (> 60%) while Enterococcus faecium CK-5 (L03) Enterococcus faecium SC54 (L12), Lactobacillus plantatrum JDFM44 (L09), Leuconstoc citreum SC53 (L12), Pediococcus acidilatic SDL1414 (L17), Pediococcus acidilatic DM9 (L21), Pediococcus pentosaceus MAC11 (L22), Weissella koreensis JBNU2 (L31), showed the least survival ability (< 41%) these least survival abilities were therefore excluded from subsequent experiments. Our results showed that all three (L. brevis, P. latici and P. pentosaceus) strains showed the highest survival ability in pepsin (> 64%), as shown in .
Resistance to bile salts and pancreatin
In this study, the LAB isolates showed varying levels of resistance to bile salts and 1 mg/mL pancreatin after 4 h of exposure (). Six LAB strains were found to be less tolerant (< 24%) to the simulated intestinal fluid after 4 h of incubation. L. brevis SDL1411 (L05), P. acidilatici DM9 (L21) and P. pentosaceus SDL1409 (L33) showed strong resistance to bile salts and pancreatin with survival abilities of 68.00%, 67.00% and 73.17% respectively. Among the Pediococcus species, only P. acidilatici DM9 (L21) and P. pentosaceus SDL1409 (L33) showed high resistance against simulated intestinal conditions respectively.
Antibiotic susceptibility
The breakpoints for testing lactobacilli are not reported in the European Committee on Antimicrobial Susceptibility Testing (EUCAST) guidelines, and the procedure proposed by the CLSI (ISO/IDF standard for antimicrobial susceptibility testing) is not adapted to the growth requirements of all Lactobacillus species. Hence our interpretation of the results was based on the breakpoint values suggested by FEDAP (EFSA Panel on Additives and Products or Substances used in Animal Feed – European Food Safety Authority, 2012) [Citation41] for ampicillin, tetracycline, erythromycin, streptomycin, gentamicin and chloramphenicol. For kanamycin, vancomycin, clindamycin and penicillin we adopted the breakpoints of tetracycline and erythromycin, respectively. In addition 0.001mg/mL concentration was tested according to against selected nine LAB strains as per the European Food Safety Authority (EFSA), 2012, Panel on Additives and Products or Substances used in Animal Feed (FEEDAP). All three LAB strains tested in this study were found to be sensitive to ampicillin, a cell wall-inhibiting antibiotic. Likewise, they were sensitive to the nucleic acid-targeting antibiotic streptomycin. However, among the cell wall-targeting antibiotics, they were resistant to penicillin. Additionally, they were resistant to the rest of the protein synthesis-inhibiting antibiotics (tetracycline, kanamycin, erythromycin, gentamicin, clindamycin and chloramphenicol) (). There was no change in the results with higher concentration 0.001mg/mL of the respective antibiotics against LAB.
Antibacterial effects of LAB isolates in vitro
In this study, the CFS and < 10Kda peptide of 9 LAB isolates showed varying levels of antimicrobial activity against P. aeruginosa (). Three LAB [L. brevis SDL1411 (L05), P. acidilatici DM9 (L21) and P. pentosaceus SDL1409 (L33)] strains were found to be strong bactericidal activity (19 – 25 mm) Enterococcus faecium CK-5 (L03), Lactobacillus plantatrum JDFM44 (L09), Leuconstoc citreum SC53 (L12), Pediococcus acidilatic SDL1414 (L17),, Pediococcus pentosaceus MAC11 (L22), Weissella koreensis JBNU2 (L31), showed the least survival ability (< 15 mm) respectively. Low molecular weight (< 10 Kda) peptide showed enhanced antimicrobial activity due to high in low molecular weight peptides respectively.
Heat stability of antimicrobial peptides (< 10Kda peptide)
The < 10Kda peptide produced by the nine selected LAB isolates were heat-treated at 90ºC for 30 and 60 min, respectively. Peptides were thermal stable at these heat conditions above as their inhibitory effects against P. aeruginosa were retained (). However, < 10Kda from Enterococcus faecium CK-5 (L03), Lactobacillus plantatrum JDFM44 (L09), Leuconstoc citreum SC53 (L12), Pediococcus acidilatic SDL1414 (L17),, Pediococcus pentosaceus MAC11 (L22), Weissella koreensis JBNU2 (L31) were less sensitive to 90°C for 30 and 60 min displaying either smaller or no inhibition zones compared to the control. But LAB Isolates [L. brevis SDL1411 (L05), P. acidilatici DM9 (L21) and P. pentosaceus SDL1409 (L33)] showed strong antimicrobial activity against P. aerogenasa and retained their anti – pseudomonas activity subsequent to exposure to 90°C for 60 min.
Digestive enzyme stability of antimicrobial peptides
The enzymatic stability of peptide produced from nine LAB isolates reveled that among the isoaltes L. brevis SDL1411 (L05), P. acidilatici DM9 (L21) and P. pentosaceus SDL1409 (L33) showed moderate sensitivity (17–20 mm) against P. aerogenasa with enzyme treated peptided, but subsequently other six LAB Enterococcus faecium CK-5 (L03), Lactobacillus plantatrum JDFM44 (L09), Leuconstoc citreum SC53 (L12), Pediococcus acidilatic SDL1414 (L17), Pediococcus pentosaceus MAC11 (L22), Weissella koreensis JBNU2 (L31) were found to be sensitive with digestive enzymes hence it lost the anti-pseudomonas activity
Nitric oxide production from LAB
The Production of nitric acid (NO) was estimated qualitatively (Sup. 1) and quantitatively determined in L5, L21, and L33 ()
NO generation had be stimulated by dietary supplementation with substrate lactobacilli in vivo. The NO (p < 0.001) content were also estimated in modified media, NO contents in C. elegens supplemented with probiotics showed increase in NO nitrate, had significantly higher at 9th day nitrite levels (, Sup.1).
Chemotaxis assay
Feeding nematodes with 35 Lactobacilli strains resulted in increases in the average life span of the nematodes compared to ther life span when fed with E. coli OP50 strain (. Between the cell pellet and broth culture of LAB, the chemotaxis assay indicates that the worms were maximally attracted towards the broth culture ()) and showed lesser attraction towards the pellet and solvents.
Figure 1. (a) Selection of preferred LAB among 35 strains (either broth or cell pellet) as food to C. elegans. (a) Cell pellet of LAB strains collected by micro-centrifuge and used as bacterial food in NGM plates with worms (n = 150 per plate). From chemotaxis assay results, worms showed a high preference for 4 LAB strains and low preference for 31 LAB strains compared with solvent (butanol and octanol). (b) Chemotaxis assay Broth of LAB strains used as food in NGM plates with worms (n = 150 per plate). A total of Worms showed high preference for 33 LAB strains and low preference for 2 LAB (L1 and L2) strains. Preference was obtained from the choice index as a numerical value (Y axis) and the threshold value from bacterial strains as the horizontal axis (X axis). (c) Fertility in C. elegans fed on various lactic acid bacteria. To explore effects of lactic acid bacteria on the fertility of nematodes, we used lactic acid bacteria as food instead of E. coli OP50 normally used in experimental conditions. The results indicate that worms fed LAB (L01-L35) had low reproduction and developmental rates compared with worms fed control E. coli OP50.
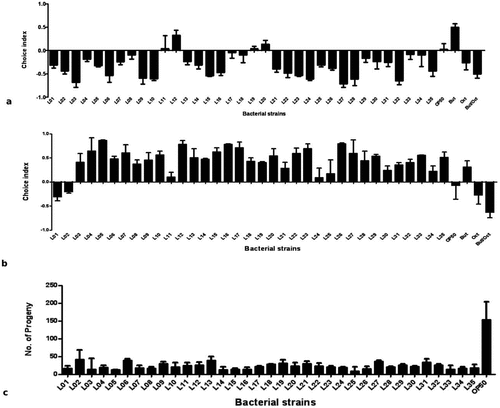
Figure 2. Life span and killing of C. elegans fed on various lactic acid bacteria and pathogens. (a)E. coli OP50 as their basic food for 24 h in NGM plates and then transferred onto P. aeruginosa (PA14)-seeded NGM plates, and the number of dead worms was enumerated each day until the 8th day of incubation. (b-j) Initially, the worms were fed for 24 h with lactic acid bacteria, which were seeded on NGM plates, and later, the worms were transferred onto PA14-seeded NGM plates (b) L2: Enterococcus faecium SC54, (c) L5: Lactobacillus brevis SDL1411, (d) L9: Lactobacillus plantatrum JDFM44, (e) L12: Leuconostoc citreum SC53, (f) L17: Pediococcus acidilatic SDL1414, (g) L21: Pediococcus acidilatici DM-9, (h) L22: Pediococcus pentosaceus MAC-11, (i) L31: Weissella koreensis JBNU2, and (j) L33: P. pentosaceus SDL 1409. In each experiment, 30 worms were administered, repeated twice and plotted using OASIS II. (a1-z2) Initially, the worms were fed for 24 h with lactic acid bacteria, which were seeded on NGM plates, and later, the worms were transferred onto PA14-seeded NGM plates (a1) L1: Enterococcus faecalis SC54, (b1) L3: Enterococcus faecium CK-5, (c1) L4: Enterococcus lactis SCL1421, (d1) L6: Enterococcus lactis SCL1421, (e1) L7: Lactobacillus curvatus JBNU38, (f1) L8: Lactobacillus pentosus SC48, (g1) L10: Lactobacillus rhamnosus JDFM33, (h1) L11: Lactobacillus rhamnosus JDFM6, (i1) L13: Leuconstoc mesenteroides JBNU10, (j1) L14: Leuconstoc paramesenteroides SC46, (k1) L15: Pediococcus acidilatic SCL1420 (l1) l16: Pediococcus acidilatic SKL1418, (m1) L18: Pediococcus acidilatic SDL1406, (n1) L19: Pediococcus acidilatic SDL1405, (o1) L20: Pediococcus acidilatic SDL1402, (p1) L23: Pediococcus pentosaceusSDL1416, (q1) L24: Pediococcus pentosaceus SDL1415, (r1) L25: Pediococcus pentosaceus SDL1401, s1) L26: Streptococcus thermophiles SCML337, (t1) L27: Streptococcus thermophiles SCML300, (w1) L28: Weissella cibaria SCCB2306, (x1) L29: Weissella cibaria SCCB2320, (y1) L30: Weissella confusa SCKB2318, (z1) L32 Weissella cibariaSCCB2307 and (z2) L34: Lactobacillus plantatrum SDL1413, (z2) L35: Lactobacillus arizonensis SC25. In each experiment, 30 worms were administered, repeated twice and plotted using OASIS II.
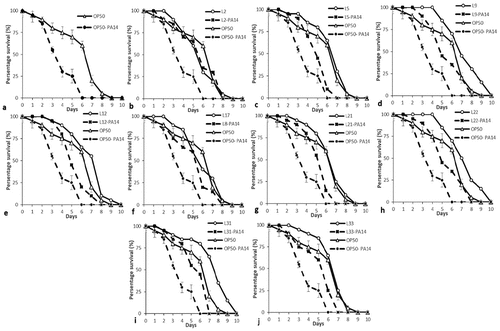
Fertility assay
To increase the fertility of nematodes, LAB strains were fed to worms in NGM plate, and their effects were compared with those of E. coli OP50. The results indicated that worms fed the 35 different LAB strains showed lower fertility compared with worms fed the OP50 strain ()). To examine if there were any beneficial effects of feeding LAB to worms compared to OP50 and the survival rate of worms fed on LAB were compared with that of nematodes fed on P. aeruginosa PA14. OP50 did not prolong the worms’ longevity as much as LAB did ()). We observed only negligible change in the body sizes of the nematodes fed on P. aeruginosa and LAB compared with those of control worms during the first 3 days after the food source was changed (Data not shown). On the other hand, 80% of the worms fed OP50 remained alive after 5 days. The pathogenicity of P. aeruginosa against C. elegans has been reported elsewhere, slow killing of C. elegans by PA14 involves the colonization and proliferation of pathogen in the host gut. The widespread problems associated with PA14 is principally due to the production of a series of virulence factors including protease, elastase, pyocyanin, and alginate [Citation1,Citation16] and was confirmed in this study; the life span of worms fed with newly isolated LAB strains from fermented soy bean paste were significantly different from the life spans of those grown on E. coli OP50 ()).
C. elegans life span assay
The OP50 fed C. elegens indicates life span up to 8th days ()), but their life span was shortened to 6th days when treated with P. aeruginosa ()). The average life span of the 35 different LAB strains individually fed nematodes compared to the life span of nematode fed with P. aeruginosa (). Based on the results obtained, pretreated P. aeruginosa with post treated with LAB strains showed increase in life span (). Among the 35 LAB isolates, nine strains showed strong acid tolerance at pH 2.5 () with range of (62.79 – 91.45%). Based on the acid tolerance and life span assay (), selectively 9 strains were proceed for probiotic characterization. Among the selected 9 strains, only L. brevis SDL1411 (L05), P. acidilatici DM9 (L21) and P. pentosaceus SDL1409 (L33) showed strong antimicrobial activity against P. aeruginosa () and the selected three strains (L05, L21 and L33) showed efficient probiotic properties, such as Pepsin (pH 2.5) stability (), Bile + Pancreatin (pH 8.0) stability (), and lower antibiotic resistance towards most of the antibiotics (). Hence the selected efficient LAB (L05, L21 and L33) strains where further analyzed for heat killed bacterial life span assay in comparison with OP50 and P. aeruginosa PA14 ()
Figure 3. Bacterial colonization in C. elegans. Evaluation of LAB colonization (numbers of log CFU/nematode) in the nematode intestine. (a, b, c) Representation of pre-exposure to LAB or P. aeruginosa PA14. The habituation of LAB in the worm showed a minor degree of inhibition with the increase in the number of P. aeruginosa PA14 in the intestine of nematodes at the seventh and ninth days of incubation. (a) L5: Lactobacillus brevis SDL 1411 (b) L21: Pediococcus acidilatici DM – 9 (c) L33: Pediococcus pentosaceus SDL 1409. All results are presented as the means± standard error mean.
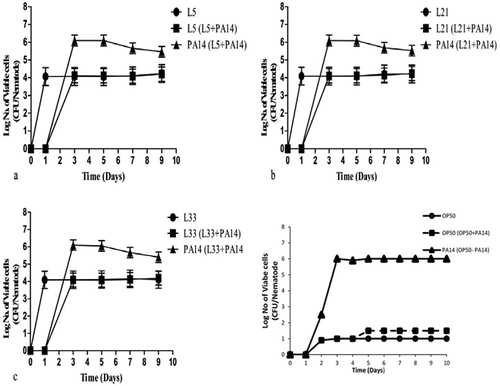
Figure 4. Lifespan and killing of C. elegans fed on heat-killed lactic acid bacteria and pathogens. (a) Young adult stage worms were transferred onto PA14-seeded NGM plates after 24 h incubation, and later, the worms were transferred onto E. coli OP50-seeded NGM plates; the dead worms were enumerated each day until the 8th day of incubation. (D): Worms were transferred onto PA14-seeded NGM plates, which were pre-exposed with heat-killed E. coli OP50 for 24 h on NGM plates; later, the dead worms were enumerated each day until the eighth day. (B-H) Lactic acid bacteria-seeded NGM plates and PA14-seeded NGM plates after 1-day incubation on LAB-seeded NGM plates. (b) L5: Lactobacillus brevis SDL 1411, (c) L21: Pediococcus acidilatici DM – 9, (d) L33: Pediococcus pentosaceus SDL 1409. Each experiment administered 30 worms and was repeated twice, and the results were plotted using OASIS II program. (Each experiment groups labelled “D” – heat-killed bacteria in experiment).
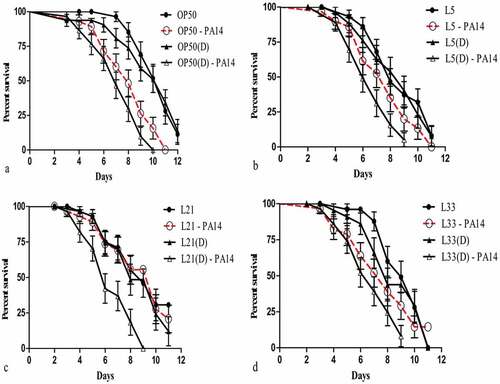
Nematode gut colonization ability of LAB
Although LAB strains in cultured broth may act as a major food source for worms, the L4 to young adult stage of the worms failed to lay eggs, and the L1 to L3 or L4 stages of the worms did not grow well compare to worms fed with OP50 ()). However, the results indicated longevity and protective effects of LAB on nematodes, which show that most of the LAB strains have beneficial effects on the nematode when challenged with P. aeruginosa PA14 ()). In these experiments, the nematodes were initially grown with LAB strains (pre-exposed) before being transferred to NGM plates with the PA14 strain comparatively the vise-versa. Post-exposure resulted in increased life span and LAB colonization in the intestine of C. elegans ()).
Life span assay of C. elegens with heat killed bacteria
The OP50 fed C. elegens indicates life span up to 8th days ()), but their life span was shortened to 6th days when treated with P. aeruginosa ()). Intestinal colonization experiment with heat-killed lactic acid bacteria were conducted to determine the detailed factors for increasing nematode longevity and protection against pathogens. As a result, the initial number of P. aeruginosa recovered from those worms during the initial stage (3-day-old L4 stage to young adult stage worms) was less than the number recovered from worms infected on the 7th day ()). The 7-day-old nematodes were fed separately with three different bacteria (L. brevis SDL1411 (L05), P. acidilatici DM9 (L21) and P. pentosaceus SDL1409 (L33) () and ). The results show that worms fed with any of the three tested strains, namely, L. brevis SDL1411 (L05), P. acidilatici DM9 (L21) and P. pentosaceus SDL1409 (L33) from 3 days old were clearly resistant to subsequent P. aeruginosa infection compared with C. elegans fed with OP50 before the Pseudomonas infection ()). However, the number of Pseudomonas cells recovered from worms fed LAB was the same as that recovered from worms grown on OP50 ()). Hence, the results reveal that compared with heat-killed bacteria, most of the live bacteria express bioactive compounds that provide protection and longevity ()). Apart from the selected LAB, other bacterial strains showed different levels of probiotic effects ()). The positive effects of LAB were brought about by their beneficial nature compared to OP50. The survival rate of worms fed on LAB was higher than that of nematodes fed on heat-killed P. aeruginosa PA14 ()), but OP50 did not prolong the worms’ longevity as much as LAB ()).
Discussion
Food and nutrition are known to be essential for survival and life. There is a particularly high interest in highly nutritious foods and functional foods [Citation23,Citation25,Citation26,Citation30,Citation42,Citation43].
It is known that soybean paste, a traditional Korean fermented food, is rich in soybean protein, organic acids, amino acids, and minerals, all of which are beneficial to health; has antioxidant, anti-obesity, anti-mutagenic and fibrinolytic properties; and harbours many beneficial bacteria as probiotics [Citation43]. The probiotic effect of lactic acid bacteria on C. elegans, which is a widely known in vivo model. However, there are no studies that used C. elegans or applied various experimental methods to confirm the effect gradually.
In this study, we investigated the probiotic effect of 35 lactic acid bacteria strains isolated from soybean paste using C. elegans, a model animal. Among 35 strains isolated from Korean traditional foods, the bioactive compounds secreted from certain Lactobacillus spp. inhibit both gram-positive and gram-negative bacteria [Citation14]. In the present study, the life span assay in C. elegans, indirectly showed that three selective Lactobacillus spp. isolates from Korean traditional foods have strong antimicrobial activity against P. aeruginosa. Antimicrobial molecules modulate the composition of the microbiota and influence the host immune system [Citation44]. Hence, Lactobacillus spp. that possess antimicrobial activity can boost the host defence response towards pathogens. In this study, the worm was used as a simple in vivo defence response model. This model has been accepted as an alternative, efficient host model that resembles other animal models and has been used to study evolutionarily conserved aspects of innate immunity [Citation45].
Probiotic lactobacilli present in fermented foods may have positive impacts on health [Citation60]. Before an efficient probiotic bacterium is confirmed, it has to be tested for its biological impact on the host during gut passage [Citation60]. The physiologic activity of probiotics should be able to withstand the stress environment imposed by digestive enzymes (Pepsin, pancreatin), bile salts, and acidity (2.0 pH) [Citation61]. Resistance to these stresses is now considered the basic criteria for screening potential probiotic strains [Citation62]. Acid resistance is also a prerequisite for the use of efficient probiotics as dietary supplements [Citation63]. Most LAB strains survive for a long time in highly acidic environments without large reductions in viable bacterial counts [Citation64]. However, the capacity of lactobacilli to survive in low pH remains debatable [Citation64]. For example, L. delbrueckii subsp. bulgaricus strain has very poor survival rates when exposed to low pH [Citation64].
Previous studies showed that the capacity of LAB and Pediococcus to survive at low pH were variable, even within the same species. In this study, the selected three strains exhibited good tolerance to pH 2.5 with significant 60% cell count ((a)). Previous studies support the testing of bacteria for resistance to gastric and intestinal conditions. However, few of these studies included testing for digestive enzymes [Citation65]. Other relevant studies on probiotics examined combined effects, for example, of pepsin and pH or pancreatic enzyme and bile salts [Citation66]. Notably, well-studied probiotics were subjected to individual and multiple stress-based assays and were found to be highly resistant to bile salts and digestive enzymes under an acidic pH of 2.0 [Citation67]. The relevant physiological concentrations of human bile ranges from 0.3% [Citation68]. Assessing the bile resistance of LAB strains is imperative during the selection of proficient probiotics [Citation68]. Since most bile- and pancreatic enzyme-tolerant strains are highly beneficial as a remedy for lactose intolerance. A significant variability in pepsin tolerance has been observed among different LAB species. 1, 2-diaminoantraquinone sulfate (DAA) with nitric oxide is water insoluble, this dye makes it possible to visualize the real NO distribution in native bacterial cells [Citation69]. Although NO molecules have high permeability through the membranes of lactobacillus and intracellular structures due to their small sizes. This result indicates that bacterial NO is generally beneficial to worms [Citation70] indicates NO Promotes heat – tolerance (stress tolerance) in C. elegans [Citation71–Citation73].
Because the evolution of multi-drug-resistant pathogens may have developed from the antibiotic resistance genes (transmissible resistance) from probiotic strains, this has become a serious safety issue [Citation73]. Some studies reported that Lactobacillus had high levels of resistance to ampicillin vancomycin and streptomycin [Citation59]. Similar results were confirmed by our study. Most of the Lactobacillus strains were kanamycin and vancomycin resistant but were susceptible to protein synthesis-inhibiting antibiotics. Further studies are needed to estimate the antibiotic resistance on the basis of genetic stability of the examined strains. Immunocompromised patients generally are more vulnerable to infection with pathogens and have a higher incidence of opportunistic infections. However, there is no published evidence that consumption of probiotics that contain Lactobacillior Bifidobacteria increases the risk of opportunistic infection among such individuals. In addition, 2 clinical studies have been conducted to assess the safety of probiotics in small groups of specific immunocompromised patients (e.g. patients with HIV infection), and the findings of these studies support the safety of probiotics consumed by such groups [Citation74–Citation76] Immunocompromised treated with – (Pediacoccus pentosaceus, Leuconostoc mesenteroides, Lactobacillus paracasei subspecies paracasei F19, Lactobacillus plantarum, LGG, L. johnsonii LA 1, VSL 3, L. plantarum 299V (liver transplant), S. boulardii (HIV) – Absence of toxicity were determined [Citation77–Citation79] previously likewise pregnant women – (LGG, LC705, Bb99, and Propionibacterium freudenreichii spp shermanii) Absence of toxicity [Citation80], Inflammatory bowel disease – (Lactobaccillus acidophilus LA-5, Lactobacillus delbrueckii subsp. bulgaricus LBY-27, Bifidobacterium animalis subsp. lactis BB-12, Streptococcus thermophilus STY-31, LGG) – Absence of toxicity [Citation81] Hospitalized adults – LGG, BioK+ -Absence of toxicity [Citation82].
In the current study, C. elegans killing assay were performed by transferring 30 young adult to L4 stage worms onto a lawn of lactobacillus species for 24 h at 25°C and then transferring them to P. aeruginosa PA14. The results revealed that all the tested Lactobacillus spp. elicited negligible changes in the physiological characteristics of C. elegans, including size, compared with those of worms given E. coli OP50 as a control (data not shown) for 24 h. Remarkably, the viability of C. elegans was significantly enhanced. When they were pre-exposed to the non-pathogenic E. coli OP50 strain [Citation46]. The chemotaxis assay has been used to reveal the behavioural characteristics of nematodes in the field of neurobiology [Citation47]. In this study, the nematodes showed low preference for the cell pellet of LAB and high preference for the broth (liquid) culture. This finding may be due to the attraction of nematodes to the diacetyl group, which is one of the secondary metabolites produced by lactic acid bacteria [Citation48]. In addition, the behavioural characteristics of nematodes during the chemotaxis assay clearly showed that the LAB strains are non-toxic to the nematodes [Citation49]. Based on these results, it is evident that LAB act as growth enhancers when they are used as food supplements for nematodes.
Results obtained from the fertility assays show that LAB reduced the reproductive ability but enhanced the life span development of the nematode, and showed a protective effect against the tested pathogen, P. aeruginosa PA14. Coenzyme Q (CoQ) is an important coenzyme in aerobic respiration, and mainly due to calorie restriction, it plays a role in increasing the lifespan of C. elegans. Similar life span enhancement based on LAB in C. elegans was reported by Martin [Citation50]. Coenzyme Q (CoQ) or ubiquinone (2, 3-dimethoxy-5-methyl-6-multiprenyl-1, 4- benzoquinone) molecules are located in the hydrophobic domain of the phospholipid bilayer of cellular membranes [Citation51]. Ubiquinone (or coenzyme Q [CoQ]), an isoprenylated benzoquinone lipid important for electron transport in aerobic respiration, is another possible factor to explain our findings. Since nematodes fed an E. coli mutant that was defective in the ability to synthesize CoQ were reported have an decreased life span [Citation52], it is possible that the LAB do have a full set of enzymes to synthesize CoQ and that this resulted in the extension of life span. In addition, Ishi et al. observed that the life span of worms could be prolonged by adding CoQ10 into the medium for the worms and discussed the possible role of ubiquinone as an antiaging substance (Ishii et al., 2004). Likewise the Coenzyme Q production leads to extending the lifespan of Drosophila. CoQ has been linked to the lifespan extension. Ishii and co-workers reported that dietary supplement of CoQ10 extended the lifespan of C. elegans by releasing oxidative stress [Citation53]. In contrast, another study showed that wild type C. elegans fed with CoQ-depleted E. coli extended their lifespan [Citation54]. Worms heterozygous for clk-1, a homolog of yeast COQ7 that is required for CoQ biosynthesis, showed extended lifespan as well [Citation27]. In mammals, although CoQ has been administrated for therapeutic use in human heart failure and ischemic heart disease [Citation55], its relationship with lifespan has, like in worms, been controversial. Kalen and co-workers detected an age-associated loss of CoQ in homogenized human tissues, while a constant level of CoQ with age was reported from homogenates of rat brains and lungs [Citation55, Citation56, Citation57]. The biosynthesis of CoQ in S. cerevisiae is a complex process. Nine genes which are designated as COQ1–COQ9 have been identified for this series of enzymatic process in S. cerevisiae [Citation58]. If LAB is producing CoQ enzyme, then it leads to increased life span of C. elegens. Similar to S. cerevisiae the CoQ gene has not been identified. Previously documented study’s also provided sufficient proof, about the reduction in fertility due to LAB feeding is based on balanced diet and as an added advantage, when compared with OP50 fed C. elegens the LAB fed C. elegens showed increased life span.
The results from bacterial colonization and killing assays that applied heat-killed LAB pellet revealed that the life span and fertility were increased in the nematodes fed with heat-killed LAB cell pellets, which indirectly prove the protective nature of the LAB and the heat stability of the bioactive compounds. In addition, when the cell pellet-treated nematodes were fed with P. aeruginosa PA14, a dramatic reduction in pathogen colonization when compared with the control (not fed with heat-treated cell pellets). Likewise, there was a steep increase in selected LAB strains (L5- L. brevis SDL 1411; L21- P. acidilatici DM – 9; L33- P. pentosaceus SDL 1409) that were initially fed to C. elegans 24 h before being fed with P. aeruginosa PA14. This finding proves the efficacy of LAB strains against the pathogen. This defensive mechanism may be due to the probiotic efficiency of the LAB26. In addition, some of the previously reported secondary metabolites that enhance the immune modulating activity may also act as defensive factors [Citation59].
In summary, the work presented here demonstrates, P. aeruginosa PA14 significantly reduced the life span of C. elegans through pathogenicity. However, L. brevis (L5), P. acidilatici (L21) and P. pentosaceus (L33) increased the life span of the C. elegans pathogenicity model. The antibacterial activity exhibited by these isolates may be useful for controlling undesirable microbiota either in the food system or in the GI tract. Our results demonstrate promising probiotic characteristics for LAB by their survival in gut-simulated fluids and gut colonization abilities. However, the multiple-antibiotic resistance exhibited by most of the LAB requires further investigation. Among the strains, those with intrinsic antibiotic resistance may be used as probiotics as they cannot transfer resistant genes to pathogenic bacteria. Nevertheless, in the future, it is essential to match the potential application with the functional properties of these probiotic candidates. Moreover, the L. brevis (L5), P. acidilatici (L21) and P. pentosaceus (L33) strains demonstrated potential probiotic features. The combination of in vitro platforms coupled with in vivo screening in the simple model organism, C. elegans, is a powerful tool to characterize industrially relevant bacterial isolates.
Author Contributions
RC., B.J.P., D.H.O., J.G.C and E.B.M., designed the experiments. R.C., B.J.P., D.H.O., J.G.C., E.B.M., S.H., carried out the experiments. D.H.O., and B.J.P. provided expertise and guidance. R.C., J.G.C., B.J.P. wrote the manuscript.
Supplemental Material
Download JPEG Image (296.2 KB)Acknowledgments
We thank Mr. Park-Yong IK (Kangwon National University, Central laboratory) for technical support for CLSM analysis. This research work was supported by a grant from the BrainKorea (BK) 21 Plus Project (Grant No. 22A20153713433) Funded by the Korean Government,Republic of Korea.. The part of this work was supported by Korea Research Fellowship Program through the National Research Foundation of Korea (NRF) funded by the Ministry of Science, in Young Researchers Program (2018007551) (http://www.nrf.re.kr/eng/main)
Disclosure statement
No potential conflict of interest was reported by the authors.
Supplementary material
Supplementary data for this article can be accessed here.
Additional information
Funding
References
- Samuel BS, Rowedder H, Braendle C, et al. Caenorhabditis elegans responses to bacteria from its natural habitats. Proc Natl Acad Sci. 2016; 113(27):E3941–E3949.
- Schulenburg H, Félix MA. The natural biotic environment of Caenorhabditis elegans. Genetics. 2017;206(1):55–86.
- Ramani AK, Calarco JA, Pan Q, et al. Genome-wide analysis of alternative splicing in Caenorhabditis elegans. Genome Res. 2011;21(2):342–348.
- Dhanashree SR, Krishnaswamy B, Kammara R. Bifid shape is intrinsic to Bifidobacterium adolescentis. Front Microbiol. 2017;8.
- El-Bestawy EA, Gohar YM, Wefky SM. Antimicrobial phenolic compounds from Enterococcus faecium S29 (EU 158188): characterization and production optimization. Int J Environ Sci Technol. 2017;14(3):497–508.
- Szatraj K, Szczepankowska AK, Chmielewska‐Jeznach M. Lactic acid bacteria‐promising vaccine vectors: possibilities, limitations, doubts. J Appl Microbiol. 2017;123(2):325–339.
- Shen Y, Kim DH, Chon JW, et al. Nutritional effects and antimicrobial activity of kefir (Grains). J Milk Sci Biotechnol. 2018;36(1):1–13.
- Vinderola G, Gueimonde M, Gomez-Gallego C, et al. Correlation between in vitro and in vivo assays in selection of probiotics from traditional species of bacteria. Trends Food Sci Technol. 2017;68:83–90.
- Gheziel C, Russo P, Arena MP, et al. Evaluating the probiotic potential of lactobacillus plantarum strains from algerian infant feces: towards the design of probiotic starter cultures tailored for developing countries. Probiotics Antimicrob Proteins. 2018;19:1–11.
- Park MR, Ryu S, Maburutse BE, et al. Probiotic Lactobacillus fermentum strain JDFM216 stimulates the longevity and immune response of Caenorhabditis elegans through a nuclear hormone receptor. Sci Rep. 2018;8(1):7441.
- Gruber J, Ng LF, Poovathingal SK, et al. Deceptively simple but simply deceptive–caenorhabditis elegans lifespan studies: considerations for aging and antioxidant effects. FEBS Lett. 2009;583(21):3377–3387.
- Grimmig B, Kim SH, Nash K, et al. Neuroprotective mechanisms of astaxanthin: a potential therapeutic role in preserving cognitive function in age and neurodegeneration. Geroscience. 2017;39(1):19–32.
- Fardet A, Rock E. In vitro and in vivo antioxidant potential of milks, yoghurts, fermented milks and cheeses: a narrative review of evidence. Nutr Res Rev. 2018;31(1):52–70.
- Kim D, Zeng MY, Núñez G. The interplay between host immune cells and gut microbiota in chronic inflammatory diseases. Exp Mol Med. 2017;49(5):e339.
- Freeman AF, Cuellar-Rodriguez JM. Infections in the immunocompromised host. In: Clinical Immunology. 5th ed. Elsevier; 2019. p. 523–534.
- Cornforth DM, Dees JL, Ibberson CB, et al. Pseudomonas aeruginosa transcriptome during human infection. Proceedings of the National Academy of Sciences; 2018; 201717525.
- Grin PM, Dwivedi DJ, Chathely KM, et al. Low-density lipoprotein (LDL)-dependent uptake of gram-positive lipoteichoic acid and gram-negative lipopolysaccharide occurs through LDL receptor. Sci Rep. 2018;8(1):10496.
- Fonceca AM, Zosky GR, Bozanich EM, et al. Accumulation mode particles and LPS exposure induce TLR-4 dependent and independent inflammatory responses in the lung. Respir Res. 2018;19(1):15.
- Ho YH, Huang YT, Lu YC, et al. Effects of gender and age on immune responses of human peripheral blood mononuclear cells to probiotics: a large scale pilot study. J Nutr Health Aging. 2017;21(5):521–526.
- Ladha G, Jeevaratnam K. Probiotic potential of pediococcus pentosaceus LJR1, a bacteriocinogenic strain isolated from rumen liquor of goat (capra aegagrus hircus). Food Biotechnol. 2018;32(1):60–77.
- Kawashima T, Ikari N, Kouchi T, et al. The molecular mechanism for activating IgA production by pediococcus acidilactici K15 and the clinical impact in a randomized trial. Sci Rep. 2018;8(1):5065.
- Trikha R, Rishi P, Tewari R. Remediation of intramacrophageal shigella dysenteriae type 1 by probiotic lactobacilli isolated from human infants’ stool samples. Indian J Med Res. 2017;145(5):679.
- Toualbia M, Bouras AED, Koiche M, et al. Isolation, identification and characterization of Lactobacillus plantarum from camel milk and its antagonist effect against diarrheal bacteria. Emirates J Food Agric. 2018;30:283–287.
- Vizoso Pinto MG, Franz CM, Schillinger U, et al. Lactobacillus spp. with in-vitro probiotic properties from human faeces and traditional fermented products. Int J Food Microbiol. 2006;109:205–214.
- Bok-Rae KIM Research on food tourism by foreign visitors in seoul. Conference on Chinese Food Culture; 2015; p. 313).
- Song DY, Park JE, Shim JE, et al. Trends in the major dish groups and food groups contributing to sodium intake in the Korea national health and nutrition examination survey 1998-2010. Korean J Nutr. 2013;46(1):72–85.
- Mokoena MP, Mutanda T, Olaniran AO. Perspectives on the probiotic potential of lactic acid bacteria from African traditional fermented foods and beverages. Food Nutr Res. 2016;60(1):29630.
- Oh A, Daliri EBM, Oh DH. Screening for potential probiotic bacteria from Korean fermented soybean paste: in vitro and Caenorhabditis elegans model testing. LWT-Food Sci Technol. 2018;88(132–138):1.
- Kojima H, Nakatsubo N, Kikuchi K, et al. Detection and imaging of nitric oxide with novel fluorescent indicators: diaminofluoresceins. Anal Chem. 1998;70(13):2446–2453.
- Yarullina DR, Ilinskaya ON. Detection of NO-synthase activity of lactobacilli by fluorescent staining. Microbiology. 2007;76(4):503–505.
- Wong A, Boutis P, Hekimi S. Mutations in the clk-1 gene of Caenorhabditis elegans affect developmental and behavioral timing. Genetics. 1995;139(3):1247–1259.
- Gao AW, Chatzispyrou IA, Kamble R, et al. A sensitive mass spectrometry platform identifies metabolic changes of life history traits in C. elegans. Sci Rep. 2017;7(1):2408.
- Available from: www.wormatlas.org/ver1/handbook/anatomyintro/anatomyintro.htm
- Mokoena MP, Mutanda T, Olaniran AO. Perspectives on the probiotic potential of lactic acid bacteria from African traditional fermented foods and beverages. Food Nutr Res. 2016;60(1):29630.
- Bito T, Matsunaga Y, Yabuta Y, et al. Vitamin B12 deficiency in Caenorhabditis elegans results in loss of fertility, extended life cycle, and reduced lifespan. FEBS Open Bio. 2013;3:112–117.
- Smolentseva O, Gusarov I, Gautier L, et al. Mechanism of biofilm-mediated stress resistance and lifespan extension in C. Sci Rep. 2017;7(1):7137.
- Wu D, Rea SL, Yashin AI, et al. Visualizing hidden heterogeneity in isogenic populations of C. elegans. Exp Gerontol. 2006;41(3):261–270.
- Antunes CA, Clark L, Wanuske MT, et al. Caenorhabditis elegans star formation and negative chemotaxis induced by infection with corynebacteria. Microbiology. 2016;162(1):84–93.
- Drovandi CC, Moores MT, Boys RJ, et al. Section I: computational statistics. Comput Stat Data Anal. 2018;118:1–138.
- GraphPad Software, Inc.,. San Diego. Available from: http://www.graphpad.com
- Patel RM, Denning PW. Therapeutic use of prebiotics, probiotics, and postbiotics to prevent necrotizing enterocolitis: what is the current evidence? Clin Perinatol. 2013;40(1):11–25.
- EFSA. Panel on additives and products or substances used in animal feed (FEEDAP). guidance on the assessment of bacterial susceptibility to antimicrobials of human and veterinary importance. EFSA J. 2012;10(6):2740.
- Carollo V. Dietary interventions to improve healthy status in pig intensive farm conditions: a morpho-functional study of target organs. Milan (Italy): Università degli Studi di Milano; 2013.
- Tamang JP, Shin DH, Jung SJ, et al. Functional properties of microorganisms in fermented foods. Front Microbiol. 2016;7:578.
- Cabreiro F, Gems D. Worms need microbes too: microbiota, health and aging in Caenorhabditis elegans. EMBO Mol Med. 2013;5(9):1300–1310.
- Irazoqui JE, Urbach JM, Ausubel FM. Evolution of host innate defence: insights from C. elegans and primitive invertebrates. Nat Rev Immunol. 2010;10(1):47.
- Mokoena MP. Lactic acid bacteria and their bacteriocins: classification, biosynthesis and applications against uropathogens: a mini-Review. Molecules. 2017;22(8):1255.
- Kabluchko TV, Bomko TV, Nosalskaya TN, et al. Survival of microorganisms from modern probiotics in model conditions of the intestine. Аннали Мечниківського інституту. 2017:1(1):28–33.
- Gupta M, Kumar Bajaj B. Selection criteria for probiotics and potential of cereal based food products as novel probiotic-carriers. Curr Nutr Food Sci. 2016;12(3):157–174.
- Bertelsen RJ, Jensen ET, Ringel-Kulka T. Use of probiotics and prebiotics in infant feeding. Best Pract Res Clin Gastroenterol. 2016;30(1):39–48. 2016.
- Reyes-Nava LA, Garduño-Siciliano L, Estrada-de los Santos P, et al. Use of bile acids as a selection strategy for lactobacillus strains with probiotic potential. J Food Nutr Disord. 2016;5:1000187(1–10).
- Liévin-Le Moal V, Servin AL. Anti-infective activities of lactobacillus strains in the human intestinal microbiota: from probiotics to gastrointestinal anti-infectious biotherapeutic agents. Clin Microbiol Rev. 2014;27(2):167–199.
- Melgar-Lalanne G, Rivera-Espinoza Y, Méndez AIR, et al. In vitro evaluation of the probiotic potential of halotolerant lactobacilli isolated from a ripened tropical Mexican cheese. Probiotics Antimicrob Proteins. 2013;5(4):239–251.
- Succi M, Tremonte P, Pannella G, et al. Pre-cultivation with selected prebiotics enhances the survival and the stress response of Lactobacillus rhamnosus strains in simulated gastrointestinal transit. Front Microbiol. 2017;8:1067.
- Abushelaibi A, Al-Mahadin S, El-Tarabily K, et al. Characterization of potential probiotic lactic acid bacteria isolated from camel milk. LWT-Food Sci Technol. 2017;79:316–325.
- Gusarov I, Gautier L, Smolentseva O, et al. Bacterial nitric oxide extends the lifespan of C. elegans. Cell. 2013;152(4):818–830.
- Darling KE, Evans TJ. Effects of nitric oxide on Pseudomonas aeruginosa infection of epithelial cells from a human respiratory cell line derived from a patient with cystic fibrosis. Infect Immun. 2003;71(5):2341–2349.
- Halaschek-Wiener J, Khattra JS, McKay S, et al. Analysis of long-lived C. elegans daf-2 mutants using serial analysis of gene expression. Genome Res. 2005;15(5):603–615.
- Samuelsson B, Morgenstern R, Jakobsson PJ. Membrane prostaglandin E synthase-1: a novel therapeutic target. Pharmacol Rev. 2007;59(3):207–224.
- Štšepetova J, Taelma H, Smidt I, et al. Assessment of phenotypic and genotypic antibiotic susceptibility of vaginal Lactobacillus sp. J Appl Microbiol. 2017;123(2):524–534.
- Nami Y, Haghshenas B, Abdullah N, et al. Probiotics or antibiotics: future challenges in medicine. J Med Microbiol. 2015;64(2):137–146.
- Wolf BW, Wheeler KB, Ataya DG, et al. Safety and tolerance of Lactobacillus reuteri supplementation to a population infected with the human immunodeficiency virus. Food Chem Toxicol. 1998;36:1085–1094.
- Cunningham-Rundles S, Ahrne´ S, Bengmark S, et al. Probiotics and immune response. Am J Gastroenterol. 2000;95:S22–S5.
- Husni RN, Gordon SM, Washington JA, et al. Lactobacillus bacteremia and endocarditis: review of 45 cases. Clin Infect Dis. 1997;25(5):1048–1055.
- Rayes N, Seehofer D, Hansen S, et al. Early enteral supply of lactobacillus and fiber versus selective bowel decontamination: a controlled trial in liver transplant recipients. Transplantation. 2002;74:123–127.
- Rayes N, Seehofer D, Theruvath T, et al. Supply of pre- and probiotics reduces bacterial infection rates after liver transplantation-a randomized, double-blind trial. Am J Transplant. 2005;5:125–130.
- Saint-Marc T, Rossello-Prats L, Touraine JL. Efficacy of saccharomyces boulardii in the treatment of diarrhea in AIDS. Ann Med Interne (Paris). 1991;142:64–65.
- Boyle RJ, Ismail IH, Kivivuori S, et al. Lactobacillus GG treatment during pregnancy for the prevention of eczema: a randomized controlled trial. Allergy. 2011;66(4):509–516.
- Azad MB, Coneys JG, Kozyrskyj AL, et al. Probiotic supplementation during pregnancy or infancy for the prevention of asthma and wheeze: systematic review and meta-analysis. Bmj. 2013;347:f6471.
- Maziade PJ, Pereira P, Goldstein EJ. A decade of experience in primary prevention of clostridium difficile infection at a community hospital using the probiotic combination Lactobacillus acidophilus CL1285, Lactobacillus casei LBC80R, and Lactobacillus rhamnosus CLR2 (Bio-K+). Clin Infect Dis. 2015;60(suppl_2):S144–S147.
- Zhang J, Holdorf AD, Walhout AJ. C. elegans and its bacterial diet as a model for systems-level understanding of host–microbiota interactions. Curr Opin Biotechnol. 2017;46:74–80.
- Sasakura H, Mori I. Behavioral plasticity, learning, and memory in C. elegans. Curr Opin Neurobiol. 2013;23(1):92–99.
- Im Choi J, Yoon KH, Kalichamy SS, et al. A natural odor attraction between lactic acid bacteria and the nematode Caenorhabditis elegans. ISME J. 2016;10(3):558–567.
- Schwarz RF, Branicky R, Grundy LJ, et al. Changes in postural syntax characterize sensory modulation and natural variation of C. elegans locomotion. PLoS Comput Biol. 2015;11(8):e1004322.
- Martin R, Miquel S, Benevides L, et al. Functional characterization of novel Faecalibacterium prausnitzii strains isolated from healthy volunteers: a step forward in the use of F. prausnitzii as a next-generation probiotic. Front Microbiol. 2017;8:1226.
- Battino M, Ferri E, Gorini A, et al. Natural distribution and occurrence of coenzyme Q homologues. Membr Biochem. 1990;9(3):179–190.
- Larsen PL, Clarke CF. Extension of life-span in Caenorhabditis elegans by a diet lacking coenzyme Q. Science. 2002;295(5552):120–123.
- Ishii N, Senoo-Matsuda N, Miyake K, et al. Coenzyme Q 10 can prolong C. elegans lifespan by lowering oxidative stress. Mech Ageing Dev. 2004;125(1):41–46.
- Larsen PL, Clarke CF. Extension of life-span in Caenorhabditis elegans by a diet lacking coenzyme Q. Science. 2002;295(5552):120–123.
- Pepe S, Marasco SF, Haas SJ, et al. Coenzyme Q 10 in cardiovascular disease. Mitochondrion. 2007;7:S154–S167.
- Beyer RE. An analysis of the role of coenzyme Q in free radical generation and as an antioxidant. Biochem Biol. 1992;70(6):390–403.
- Kawamukai M. Biosynthesis and bioproduction of coenzyme Q10 by yeasts and other organisms. Biotechnol Appl Biochem. 2009;53(4):217–226.